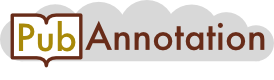
FA10@AikoHIRAKI:FA1102
Pias3-dependent SUMOylation controls mammalian cone photoreceptor differentiation
Abstract
Selective expression of retinal cone opsin genes is essential for color vision, but the mechanism mediating this process is poorly understood. Both vertebrate rod and medium wavelength–sensitive (M) cone photoreceptors differentiate by repression of a short wavelength–sensitive (S) cone differentiation program. We found that Pias3 acts in mouse cone photoreceptors to activate expression of M opsin and repress expression of S opsin, with the transcription factors Trβ2 and Rxrγ mediating preferential expression of Pias3 in M cones. Finally, we observed that Pias3 directly regulated M and S cone opsin expression by modulating the cone-enriched transcription factors Rxrγ, Rorα and Trβ1. Our results indicate that Pias3-dependent SUMOylation of photoreceptor-specific transcription factors is a common mechanism that controls both rod and cone photoreceptor subtype specification, regulating distinct molecular targets in the two cell types.
Main
Vertebrate image-forming retinal photoreceptors are comprised of rod and cone subtypes. Rod photoreceptors are activated by dim light, whereas cone photoreceptors are activated by bright light, and the two photoreceptor subtypes differ considerably in function and gene expression. Most vertebrates possess multiple cone opsin genes, whose maximum spectral absorbance are tuned to different wavelengths and are expressed in different cone photoreceptor cells. Correct expression of cone opsin subtypes is critical for normal color vision, which is essential for many ecologically important behaviors1,2,3,4. Mice have two cone opsin genes, Opn1sw and Opn1mw, that are maximally sensitive to short and medium wavelengths of light, respectively, and that are expressed in opposing, partially mosaic gradients along the dorsoventral axis of the retina. Three major subtypes of cone photoreceptors are found in the mouse: S cones, M cones and mixed cones that express both opsin genes5,6,7,8.
The molecular pathways that guide rod and cone cell fate specification differ considerably. Although the homeodomain factors Otx2 and Crx are required for differentiation of both rods and cones9,10, the two photoreceptor classes largely use mutually exclusive sets of transcription factors to guide their differentiation. In developing rods, the rod-specific transcription factors Nrl and Nr2e3 bind to both rod- and cone-specific promoters and active expression of rod-specific genes while repressing expression of S cone–specific genes11,12,13,14. Developing M cone photoreceptors likewise differentiate by repressing S opsin expression and activating M opsin expression. Loss of function of the nuclear hormone receptor Trβ2, which is selectively expressed in developing cone photoreceptors and at lower levels in mature cones15, results in a loss of M opsin expression and a corresponding upregulation of S opsin16, whereas loss of function of Rxrγ results in ectopic expression of S opsin in all cones without affecting M opsin expression17. Injection of 3,5,3′-triiodothyronine (T3), the physiological ligand for Trβ2, leads to activation of M opsin expression and repression of S opsin expression, and it has been proposed that T3 regulates cone opsin specification by altering Trβ2 activity18. Both the ligand binding and DNA binding domains of Trβ2 are required for regulation of cone opsin expression19,20. Furthermore, Trβ2 is bound to the promoters of both Opn1mw and Opn1sw in vivo, implying that unidentified regulatory factors must also control Trβ2-dependent regulation of cone opsin gene expression, allowing it to directly repress S opsin expression and activate M opsin expression21.
We previously found that Pias3-dependent SUMOylation of Nr2e3 is essential for mediating repression of S cone–specific genes in rods22. Here, we found that Pias3-dependent SUMOylation has a similar role in regulating cone photoreceptor subtype specification.
Results
Pias3 is selectively expressed in M cones
Section immunohistochemical analysis of the adult C57BL/6 mouse retina indicated that Trβ2, which is expressed in the nuclei of cone photoreceptor cells, was colocalized with Pias3 (Supplementary Fig. 1), as was previously observed for developing cones22. Using antibodies to M opsin and S opsin, we found that Pias3 was present in both the nucleus and inner segment of cone photoreceptors (Supplementary Fig. 1). Flat-mount immunohistochemistry revealed that Pias3 expression was elevated in M cones relative to S cones in dorsal retina (Fig. 1a). In ventral retina, which lacks M cones, but contains many cones that coexpress both M and S cone opsin, we observed higher levels of Pias3 in M opsin–expressing cones (Fig. 1a). Quantifying Pias3 immunoreactivity revealed that Pias3 expression correlated directly with levels of M opsin expression in cone photoreceptors and was independent of the dorso-ventral position of these cells (Fig. 1b). Z stack images of the flat mount images confirmed that Pias3 expression was greater in both the nucleus and inner segment of M opsin–expressing cones than in S opsin–expressing cones (Supplementary Fig. 1).
We further examined Pias3 expression in developing cone photoreceptors (Supplementary Fig. 2). Until postnatal day 4 (P4), when Pias3 is weakly expressed in cones, all cones express S cone opsins. After P6, the onset of cone expression of Pias3 coincides with repression of S opsin expression and the activation of M opsin expression. To determine whether enriched expression of Pias3 in long-wavelength cones is evolutionarily conserved, we identified two potential Pias3 homologs in zebrafish (Supplementary Fig. 3). We found that these genes were coexpressed in an identical subset of developing cone photoreceptors at both 5 d and 4 months post-fertilization (Supplementary Fig. 3 and data not shown). Two-color in situ hybridization using cone opsin probes at 4 months post-fertilization, indicated that both Pias3 homologs were expressed in LWS (long wavelength-sensitive) cones and rhodopsin 2 cone subtypes, along with ultraviolet-sensitive short wavelength–sensitive type 1 (SWS1) cones, but were not detectable in the blue-sensitive short wavelength–sensitive type 2 (SWS2) cone subtypes (Supplementary Fig. 3). This pattern of Pias3 expression resembles that of the mouse, in that Pias3 is expressed in cones that express the LWS opsin, the ortholog of mouse M cone opsin, and is excluded from the SWS2 cone opsins. It differs in that Pias3 is expressed in SWS1-postive cones, which is the ortholog of the mouse S cone opsin.
Pias3-dependent SUMOylation regulates M/S opsin expression
We used in vivo electroporation to determine whether Pias3-dependent SUMOylation controls cone opsin subtype expression22. For these experiments, we only examined electroporated cells in the central retina, where the vast majority of cone photoreceptors coexpress M and S cone opsins (Fig. 2a). When we overexpressed Pias3 using the ubiquitous CAG promoter, green fluorescent protein (GFP)-positive cones upregulated M opsin and downregulated S opsin, with many cones in the central retina shifting from coexpressing M and S cone opsins to only expressing M opsin (Fig. 2b,c). Small hairpin RNA (shRNA)-mediated knockdown of Pias3, however, resulted in an increase in GFP-positive cones that exclusively expressed S opsin and inhibited M opsin expression (Fig. 2d,e). Although Pias3 knockdown also induces S opsin expression in electroporated rods22, these cells expressed S opsin at much lower levels than cones and could easily be excluded from analysis (data not shown).
To further determine whether the E3 SUMO ligase activity of Pias3 was required for these effects, we overexpressed a SUMOylase-deficient Pias3 mutant (Pias3ΔSUMO)23, which was previously shown to inhibit Pias3-depedent SUMOylation of Nr2e3 (ref. 22); the resulting phenotype was similar to that seen following shRNA-mediated Pias3 knockdown (Fig. 2b,c). Finally, to determine whether a global inhibition of SUMOylation would phenocopy these effects, we overexpressed the adenoviral protein Gam1, which selectively targets the sole cellular E1 SUMO ligase for proteolytic destruction24; the inhibition phenocopied the effects of Pias3 loss of function (Fig. 2b,c).
Trβ2 and Rxrγ directly activate Pias3 expression
As Trβ2 and Rxrγ are both required for cone subtype specification, but are maximally expressed nearly 1 week before the onset of any differential expression of cone opsins16,17, we next investigated whether these transcription factors might act by directly regulating expression of Pias3. We first examined the proximal promoter of Pias3 and found a thyroid hormone response element (TRE) that was evolutionarily conserved among mammals (Fig. 3a). Because thyroid hormone receptors often bind TREs as heterodimers with Rxr family members25, we next determined whether Trβ2 and Rxrγ could cooperate to transactivate expression of Pias3. Using HEK293 cell–based luciferase reporters, we observed that transfection of both Trβ2 and Rxrγ led to a dose-dependent activation of transcription from the Pias3 proximal promoter (Fig. 3b).
In parallel, we tested the effect of ligands of these nuclear hormone receptors. 9-cis retinoic acid (9CRA), which is a physiological ligand for heterodimeric Rxrγ26, is enriched in the dorsal neonatal mouse retina27, whereas only low levels of T3 are detected. We found that 9CRA enhanced activation of the Pias3 promoter in the presence of both Trβ2 and Rxrγ. Neither 9CRA with Rxrγ alone nor T3 enhanced activation of Pias3 (Fig. 3b) and all-trans retinoic acid did not enhance Pias3 activation (data not shown). Finally, we observed that the dose-dependent transcriptional activation induced by 9CRA in cells expressing both Trβ2 and Rxrγ was blocked by the simultaneous addition of T3 (Fig. 3b). These data suggest that T3 might act to antagonize the effects of 9CRA on Pias3 expression. This finding may explain the seemingly paradoxical effects of treatment with high concentrations of T3 during the first postnatal week, which led to a repression of M opsin expression, rather than the activation of M opsin expression that is seen at later stages (A.O., unpublished observations), and implies that 9CRA can only efficiently upregulate Pias3 expression when T3 levels are low.
Genetic disruption of Trβ2 preserves retinal expression of the Trβ1 splice form of the Thrb gene, which differ in their 5′ UTR and N-terminal coding domains. Trβ1 is prominently expressed in the postnatal retina16 and we sought to determine whether it was expressed in a similar cellular pattern to Trβ2 and could functionally substitute for Trβ2 in regulating Pias3 transcription. In situ hybridization analysis with probes specific to the first exons of Trβ1 and Trβ2 indicated that both Trβ1 and Trβ2 mRNAs were enriched in a subset of cells in the outer portion of the outer nuclear layer, where cone photoreceptor cell bodies are located. This pattern of Trβ1 expression was preserved in Trβ2−/− mice (Supplementary Fig. 4). When we transfected both Trβ1 and Rxrγ, into 293T cells, Pias3 reporter expression was modestly increased (Supplementary Fig. 4). However, 9CRA did not produce a significant increase (P > 0.05) in reporter expression. We therefore conclude that Trβ2, in conjunction with Rxrγ and 9CRA, directly activates transcription of Pias3 in developing retina.
To confirm that Trβ2 and Rxrγ directly regulate Pias3 expression in vivo, we carried out chromatin immunoprecipitation (ChIP) analysis of the Pias3 promoter and found that Trβ2 and Rxrγ were bound to this region in developing retina (Fig. 3c,d). We next investigated whether loss of function of Trβ2 led to a loss of differential expression of Pias3 in developing cones. Examining retinas of Trβ2−/− mice, along with the loss of the great majority of M opsin expression, we found that all of the cone photoreceptors expressed the uniform and relatively low level of Pias3 that is characteristic of S cones (Fig. 3e,f).
Pias3 modulates Trβ2 and T3-regulated cone opsin expression
As thyroid hormone itself did not directly regulate the Pias3 promoter–driven luciferase construct, we asked whether Pias3 might influence T3-dependent regulation of cone opsin subtype expression. We observed, as have others18,20, that injecting T3 into mice in the second postnatal week both enhanced M opsin expression and repressed S opsin expression (Fig. 4a,b). We found that this effect was blocked by shRNA-mediated reduction of Pias3 expression (Fig. 4a,b).
Flat-mount immunohistochemistry indicated that some cone photoreceptors in the dorsal retina still expressed M opsin in Trβ2−/− mice, although no M opsin expression was detected in mice lacking both the β1 and β2 splice forms of the receptor (Fig. 5a). The expression patterns of Trβ1 and Trβ2 mRNA in cones of wild-type mice were very similar, and Trβ1 expression was preserved in Trβ2−/− retina (Supplementary Fig. 4). We found that, unlike Trβ2, Trβ1 did not efficiently cooperate with 9CRA-bound Rxrγ to induce expression of the Pias3 promoter–driven luciferase construct (Fig. 3b and Supplementary Fig. 4), suggesting that Trβ1 might not compensate for the loss of Trβ2 in regulating Pias3 expression. In addition, Trβ1 mRNA is upregulated beginning at P10 (refs. 16,17), when M opsin mRNA expression is first observed. We therefore hypothesized that Trβ1 might contribute to regulation of cone opsin expression following the developmental onset of expression of Pias3 and that the indispensable function of Trβ2 in regulating cone photoreceptor development might be mediated through transcriptional control of Pias3.
To determine whether Pias3 overexpression could regulate cone opsin expression in the absence of Trβ2, we used in vivo electroporation to overexpress Pias3 in neonatal Trβ2−/− retina. Overexpression of Pias3 robustly induced M opsin expression and repressed S opsin expression in Trβ2−/− retina at P14 (Fig. 5b,c). Notably, Trβ2−/− retinas electroporated with CAG-Pias3 also occasionally expressed M opsin in cells that were not GFP positive (Fig. 5c). The mechanism underlying this effect is unclear.
On the basis of these findings, we hypothesized that Pias3 might directly regulate cone opsin expression by binding and SUMOylating nuclear hormone receptors such as Trβ1 that are bound at these promoters. Using ChIP analysis, we found that Trβ1 and Trβ2, along with Rxrγ, bound to the promoters of many photoreceptor-specific genes, including both Opn1mw and Opn1sw, but were generally absent from the promoters of genes selectively expressed in either nonphotoreceptor cells of the retina or nonretinal tissues (Supplementary Fig. 5).
Pias3 directly regulates cone opsin expression
As Trβ2 is specifically expressed in cone photoreceptors in retina15,16, we carried out sequential ChIP to determine whether Pias3 is present at cone opsin promoters in retinal cones and whether these promoters are bound by hyperSUMOylated proteins, as has been previously reported for photoreceptor-specific promoters in retinal rods22. We found that Pias3 was present at both the Opn1sw and Opn1mw promoters in vivo, along with Trβ2 (Fig. 6a and Supplementary Fig. 5), and that proteins bound to these promoter sequences were hyperSUMOylated (Fig. 6b and Supplementary Fig. 5), suggesting that Pias3 may directly regulate cone opsin gene expression by SUMOylation of target proteins at these promoters. Furthermore, we also found that the promoters of the rod-specific genes rhodopsin and Gnat1 did not bind Pias3 or Sumo1-conjugated proteins when also bound by Trβ2, unlike the Opn1sw and Opn1mw promoters (Fig. 6a,b). Similarly, the rhodopsin and Gnat1 promoter sequences were not bound by Pias3 or by Sumo1-conjugated proteins in Nrl−/− mice, which lack rod photoreceptors altogether (Supplementary Fig. 6).
In addition to Trβ1, Trβ2 and Rxrγ, another strong candidate substrate for Pias3-dependent SUMOylation in cone photoreceptors is the orphan nuclear hormone receptor Rorα. This protein, which is expressed in cones after P3, binds to the promoters of cone-specific genes in mouse retina and can activate expression of Opn1sw promoter–driven luciferase constructs28. Furthermore, Rorα is a known substrate for Pias3-dependent SUMOylation29. Using a cell-based luciferase assay, we tested whether Pias3-dependent SUMOylation could directly account for activation of Opn1mw transcription and repression of Opn1sw transcription in the presence of these factors.
We first confirmed that both Trβ1 and Trβ2 activated expression of an Opn1mw promoter–driven luciferase construct in the presence of Crx. Pias3 enhanced Trβ-dependent transcription in a dose-dependent manner. Trβ1-dependent transcription was further enhanced by the addition of T3 and this effect was similarly enhanced by Pias3. There were no dose-dependent increases in reporter expression in SUMOylation-deficient Pias3 mutants in either the absence or presence of T3 (Fig. 6c,d). We conclude that Pias3-dependent SUMOylation activates M opsin expression by enhancing T3-dependent transcriptional activation mediated by either Trβ1 or Trβ2.
We also found that Crx, Trβ2 and Rxrγ cooperated to activate S opsin expression (Fig. 6e). Rorα, in combination with Crx, activated Opn1sw transcription, as was found previously28. However, when Rorα was tested in combination with Crx, Trβ2 and Rxrγ, this resulted in a significant inhibition (P < 0.05) of Opn1sw promoter–driven luciferase expression relative to that observed in the absence of Rorα. This inhibition was enhanced by Pias3-dependent SUMOylation, as E3 SUMO ligase–deficient Pias3 mutants had a significantly reduced (P < 0.05) ability to repress the Opn1sw promoter–driven luciferase construct relative to wild-type Pias3. To determine whether SUMOylation of Rorα by Pias3 was directly responsible for the observed repression of S-cone opsin, we tested whether repression was deficient in a K185R SUMOylation site Rorα mutant29. This mutant showed substantially reduced inhibition of S cone opsin reporter expression and adding Pias3 did not result in any enhanced transcriptional repression (Fig. 6f). Taken together, these findings suggest that Pias3-dependent SUMOylation of Rorα mediates repression of S cone opsin expression.
We also found that Rorα expression was upregulated in the cone photoreceptors of Trβ2−/− mice (Supplementary Fig. 7). This observation suggests that upregulated Rorα in Trβ2−/− cones, which express lower levels of Pias3, enhances S opsin expression in combination with Crx. Furthermore, overexpression of Pias3 in Trβ2−/− cones may result in higher levels of SUMOylated Rorα, accounting for the enhanced Pias3-dependent repression of S opsin that we found in this mutant background (Fig. 5b,c).
Discussion
These findings have a number of implications. First, they identify Pias3-dependent SUMOylation as being critical for both initial patterning of cone photoreceptor subtypes in mouse retina and in maintenance of cone opsin expression. This may resolve a number of mechanistic questions regarding cone opsin patterning in mice. Although both Trβ2 and Rxrγ are necessary for regulating cone opsin patterning, maximal expression of these genes in developing cones occurs just before birth, days before differential expression of S and M opsin is observed30. Furthermore, both genes are uniformly expressed in all cone subtypes15,16,17,18. Although a dorsoventral gradient of T3 has been detected in P10 retina, no such gradient is found in neonatal retina18. The reason for this early peak in expression of Trβ2 and Rxrγ has been unclear, along with the manner in which activity of these factors is regulated in early postnatal retina.
Our data suggest that the initial expression of Pias3 in dorsal M cone photoreceptors is directly mediated by Trβ2 and 9CRA-bound Rxrγ. 9CRA can be synthesized directly from 9-cis retinal and by spontaneous non-enzymatic isomerization of all-trans retinoic acid. In the developing mouse retina, two retinaldehyde dehydrogenases, Aldh1a1 and Aldh1a3, are expressed in the dorsal and ventral regions, respectively31,32. Aldh1a1 synthesizes 9CRA more effectively than Aldh1a3 (refs. 33,34). Furthermore, all-trans retinoic acid is enriched in dorsal neonatal mouse retina27. Our data suggest that this difference in 9CRA distribution leads to higher levels of Pias3 expression in cone photoreceptors in the dorsal retina. Late in the first postnatal week, Pias3-dependent SUMOylation modulates the activity of Rorα, which, in conjunction with unliganded Trβ1 and/or Trβ2 and Rxrγ, downregulates expression of S cone opsin. Simultaneously, Pias3 enhances T3- and Trβ-dependent activation of M-cone opsin expression, with the higher levels of M opsin expression in dorsal retina reflecting the dorsoventral gradient of T3 observed after the first postnatal week (Supplementary Fig. 8). Pias3 therefore acts as a dual-function transcriptional co-regulator to simultaneously coordinate expression of the Opn1mw and Opn1sw genes.
Second, these data reveal an unexpected common molecular pathway linking rod and cone photoreceptor specification. Genetic data have indicated that both developing photoreceptor subtypes must repress S cone–specific genes before activating rod- and M cone–specific genes. However, the transcription factors that mediate this process in rods, Nrl and Nr2e3, are not expressed in cones, whereas Trβ2 and Rxrγ are not expressed in rods. Thus, it has been unclear whether there is any common molecular pathway mediating this dual-specificity transcriptional regulatory activity. Our results suggest that Pias3 fulfills this function in both developing rods and cones, but does so by acting on different sets of nuclear hormone receptors in the two cell types. Furthermore, in cone photoreceptors, only cone-specific promoters have these modifications, unlike in retinal rods, where the promoters of both rod- and cone-specific genes are bound by both by Pias3 and by hyperSUMOylated proteins22. This may reflect the fact that although rod photoreceptors must actively repress expression of S cone–specific genes in mice, there is no evidence that rod-specific gene expression is actively repressed in mammalian cone photoreceptors.
Third, this conserved function of Pias3 in photoreceptor subtype specification implies that the dual-specificity regulation of nuclear hormone function by Pias3-dependent SUMOylation has been employed repeatedly over the course of vertebrate evolution as a mechanism to direct development of photoreceptor subtypes away from a default short-wavelength cone fate. Phylogenetic analysis indicates that LWS opsins were the first visual opsins to diverge from nonvisual ciliary opsins, with short wavelength–sensitive opsins diverging from the LWS opsins soon thereafter35,36,37. Thus, from very early stages of vertebrate retinal evolution, developing retinal photoreceptors would have needed to make a binary choice between expressing a long- and a short-wavelength cone opsin38,39. We hypothesize that the decision to keep SWS expression as a default developmental state was fixed at this stage. In mice, which possess only the SWS1 and LWS cone opsins and rhodopsin, SWS1 expression is a default developmental state for both rods and LWS-positive cones. As the process of Pias3-dependent regulation of photoreceptor subtype specification is conserved between rods and cones, whereas the targets of Pias3 are not, our findings suggest that the differential Pias3-dependent SUMOylation of nuclear hormone receptors in an ancestral cone-like photoreceptor may have been a triggering event in the initial generation of photoreceptor subtype diversity in the vertebrate retina.
Analysis of the role of Pias3 homologs in the specification of photoreceptor subtype in tetrachromatic vertebrates, such as zebrafish, may allow analysis of whether Pias3 acts more generally to promote differentiation of long-wavelength cones at the expense of short-wavelength cones. In zebrafish, genetic data suggest that SWS1 is not a default developmental fate for retinal photoreceptors40 and Pias3 homologs were expressed in all cone subtypes except for SWS2 (Supplementary Fig. 2), indicating that this short-wavelength cone subtype may instead serve as a default developmental fate in this species. Further investigation will be required to address this possibility.
It is now clear that neuronal subtype specification is often regulated by transcription factors that have opposing effects on expression of cell subtype–specific genes. There are many other examples in both vertebrates and invertebrate development41. Our finding that SUMOylation-dependent regulation of transcription factor activity coordinates the development of multiple different photoreceptor subtypes suggests that such a mechanism may be used more generally in the regulation of neuronal cell fate specification.
Methods
Animals.
Timed pregnant CD1 and C57BL/6 mice were purchased from Charles River Breeding Laboratory and Jackson Laboratory, respectively. Trβ2−/− and Trβ1−/−; Trβ2−/− mice were provided by D. Forrest (National Institute of Health). All experimental procedures were pre-approved by Johns Hopkins Medical Institutions animal care and the Institutional Animal Care and Use Committee of Washington University School of Medicine and conformed to the guidelines of the Association for Research in Vision and Ophthalmology for the use of live animals in vision research.
DNA constructs.
Most of cDNA constructs used in this study have been described previously22. The cDNA of mouse Trβ2 and Sop-600 luciferase vectors were provided by D. Forrest. A 600-bp fragment of mouse Pias3 promoter region (chromosome 3, base pairs 96,500,554–96,501,097) was generated by PCR and cloned into the pGL3 luciferase vector (Promega). cDNAs of human Rxrγ, Trβ1 and Rorα were shuttled from the entry clones of the Ultimate Human ORF Collection (Invitrogen) into pcDNA3.1/nV5-DEST (Invitrogen). The K185R mutant of Rorα was made using the QuikChange Site-Directed Mutagenesis Kit (Stratagene). All of the DNA constructs are listed in Supplementary Table 1.
In vivo electroporation.
In vivo electroporations were performed at P0 as described previously42. We injected ∼0.3 μl of DNA solution (5 μg μl−1) into the subretinal space of P0 mouse pups and applied square electric pulses (100 V, 5 50-ms pulses with 950-ms intervals) with tweezer-type electrodes (BTX, model 522). The CAG-GFP vector was electroporated (1 μg μl−1) with both shRNA constructs (U6-control and U6-Pias3) to allow for visualization of transfected cells. The electroporated retinas were harvested at P14 for immunohistochemistry. For T3 treatment experiments, electroporated pups were injected subcutaneously with 1.5 μg of T3 (Sigma) or saline every 24 h from P7 to P12. When quantitative data was obtained, a minimum of 100 electroporated cone photoreceptors from each of a minimum of three different retinas were scored.
Immunohistochemistry.
Whole retinas were fixed with 4% paraformaldehyde (vol/vol) in phosphate-buffered saline for ∼60 min at 25 °C. After 1 h blocking with 10% horse serum (vol/vol) in phosphate-buffered saline and 0.1% Triton X-100, the eyecups were immunostained for 2 d with antibodies to Pias3 (1:2,000, P0117, Sigma), M opsin (1:5,000, JH492, a gift from J. Nathans) and S opsin (1:500, sc-14363, Santa Cruz), respectively. After staining with secondary antibodies, the retinas were flattened on slide glasses. Images were taken on an Apotome confocal-style three-dimensional imaging microscope (Zeiss). The intensities of Pias3 signals in cone photoreceptor nuclei were measured by profile mode on AxioVision (Zeiss) software. Section immunohistochemistry was performed as previously described22. Goat polyclonal antibody to RORα (sc-6062, Santa Cruz) was used at a 1:1,000 dilution. When quantitative data was obtained, a minimum of 100 cone photoreceptors from each of a minimum of three different retinas were scored.
Luciferase assays.
Luciferase analysis was performed essentially as described22. We treated cells with 5 μM or 10 μM 9CRA (Sigma) and/or 1 nM or 5 nM T3 24 h after transfection. The transfected cells were harvested 48 h after transfection and luciferase activity was measured. As 293T cells express low levels of endogenous Pias3 (data not shown), 10 ng of an shRNA construct selectively targeting human, but not mouse, PIAS3 was included in each transfection to eliminate confounding effects of endogenous Pias3.
In situ hybridization.
Single-color in situ hybridization was performed as previously described43. Two-color in situ hybridization was performed as described previously with minor modifications44. The following regions were used to generate probes for probes for in situ hybridization in zebrafish retinal sections: Pias3-1 chr18, 14,151,257–14,151,760; Pias3-2 chr7, 29,557,068–29,557,473 (July 2007 assembly, UCSC Genome Browser); LWS, NM_131175, 111–1184; SWS1, NM_131319, 62–1072; SWS2, NM_131192, 128–1192; Rh2, NM_131253, 68–1117. The divergent 5′ regions of Trβ1 (NM_001113417.1, 0–469 bp) and Trβ2 (NM_009380.3, 0–554 bp) were used to generate probes for detection of mouse Trβ1 and Trβ2 transcripts.
ChIP.
ChIP was performed as described45. The results of ChIP assays were analyzed using candidate gene–based PCR with primers spanning the promoter region of each gene45. Results shown are representative of at least three separate experiments. Controls include the use of normal rabbit/mouse IgG (Santa Cruz) in immunoprecipitation reactions (negative controls) and input (without immunoprecipitate) as positive controls in PCR reactions. Double ChIP assays were performed as described previously22,46 with antibodies to Trβ1, Trβ2 and Rxrγ included in the assay. Antibodies to Pias3, Sumo1 and mouse IgG have been previously described22. We also used antibodies to Trβ1 (Millipore, 06-539), Trβ2 (gift of D. Forrest) and Rxrγ (Abgent, AT3749a). Primers used for PCR amplification of genomic DNA for Rho, Mop, Sop, Grm6, Thy1 and Alb have been previously described22. Other primer sets used are listed on Supplementary Table 2.
Statistical analysis.
The values in this study are represented as mean ± s.d. or s.e.m. Statistical comparisons were done using a two-tailed Student's t test and P < 0.05 was considered to be statistically significant.
|
Annnotations
- Denotations: 202
- Blocks: 0
- Relations: 0