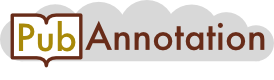
FA10@AikoHIRAKI:FA1056
The Jmjd3-Irf4 axis regulates M2 macrophage polarization and host responses against helminth infection
Abstract
Polarization of macrophages to M1 or M2 cells is important for mounting responses against bacterial and helminth infections, respectively. Jumonji domain containing-3 (Jmjd3), a histone 3 Lys27 (H3K27) demethylase, has been implicated in the activation of macrophages. Here we show that Jmjd3 is essential for M2 macrophage polarization in response to helminth infection and chitin, though Jmjd3 is dispensable for M1 responses. Furthermore, Jmjd3 (also known as Kdm6b) is essential for proper bone marrow macrophage differentiation, and this function depends on demethylase activity of Jmjd3. Jmjd3 deficiency affected trimethylation of H3K27 in only a limited number of genes. Among them, we identified Irf4 as encoding a key transcription factor that controls M2 macrophage polarization. Collectively, these results show that Jmjd3-mediated H3K27 demethylation is crucial for regulating M2 macrophage development leading to anti-helminth host responses.
Main
Innate immune cells such as macrophages sense the presence of microbial infection through pattern-recognition receptors and mount anti-microbial responses1,2,3. The Toll-like receptor (TLR) family is one of the best-characterized PRR families recognizing various pathogens such as bacteria, viruses, protozoa and fungi. TLRs are crucial in evoking innate immune responses to infection by various pathogens, leading to production of inflammatory mediators, including proinflammatory cytokines, chemokines and interferons.
Macrophages are functionally polarized into M1 and M2 cells in response to infection with microorganisms and host mediators4,5. M1 macrophages produce large amounts of nitric oxide by expressing inducible nitric oxide synthase (iNOS) and tumor necrosis factor (TNF), and are essential for clearing bacterial, viral and fungal infections6. Other macrophages, called alternatively activated macrophages or M2 macrophages, have an important role in responses to parasite infection, tissue remodeling, angiogenesis and tumor progression. M2 macrophages are characterized by their high expression of arginase-1 (Arg1), chitinase-like Ym1 (Chi3l3), found in inflammatory zone-1 (Fizz1, also called Retnla) mannose receptor (Mrc1 encoding MR, also known as CD206), and chemokines such as CCL17 and CCL24 (refs. 7, 8, 9, 10, 11). The PRR system responsible for the recognition of helminth infection and M2 polarization has yet to be identified.
Cytokines and growth factors have been implicated in the reprogramming of M1 and M2 macrophages. Whereas interferon-γ (IFN-γ), produced by activated T cells and TLR ligands, induces M1 macrophage generation, stimulation of macrophages with interleukin-4 (IL-4) or IL-13 induces M2-type macrophages4,5. In addition, immune complexes, IL-10 and glucocorticoid or secosteroid hormones are also known to generate M2 macrophages. Among growth factors, treatment of bone marrow cells with granulocyte-macrophage colony-stimulating factor (GM-CSF) and macrophage colony-stimulating factor (M-CSF) leads to generation of M1 and M2 cells, respectively12,13,14,15.
TLRs trigger intracellular signaling pathways, inducing activation of a set of transcription factors, such as NF-κB, AP-1, C/EBPβ, PU.1 and interferon-regulatory factors (IRFs)1,16. These transcription factors cooperatively upregulate the expression of multiple genes such as proinflammatory cytokines, leading to M1 macrophage polarization. Proteins induced by TLR signaling are known to modulate inflammatory responses. IκBζ, an IκB family member, positively regulates certain genes such as II6 by interacting with NF-κBp50 or inducing histone modification17,18, whereas ATF3 negatively regulates expression19. In contrast, transcription factors such as STAT6 and peroxisome proliferator-activated receptor-γ (PPARγ) are involved in polarization of M2 macrophages5,20.
In addition to regulation by transcription factors, epigenetic regulation is essential for controlling proper gene expression16. Histone modifications, particularly at the N-terminal tails, and dynamic chromatin remodeling have been shown to be important for controlling sets of genes. In the case of histone modification, trimethylation of H3K4 is associated with active gene transcription, whereas trimethylation of H3K9, H3K27 and H4K20 is linked to silencing of gene expression16,21,22. The methylation of H3K27 is mediated by the Polycomb repressive complex-2 (PRC2), composed of Ezh2, Suz12 and Eed (ref. 23). Proteins harboring a Jumonji-C (JmjC) domain, such as Jmjd3, Utx and Uty, are known to act as H3K27 demethylases, catalyzing the conversion of H3K27me3 (trimethylated) to H3K27me1 (monomethylated)24,25,26.
It has been reported that the expression of Jmjd3 is induced in macrophages by TLR stimuli in an NF-κB–dependent fashion26. Jmjd3 has also been identified as an early TLR-inducible gene in mouse macrophages by microarray analysis27. H3K27 trimethylation is implicated in the silencing of gene expression, and it has been shown that Jmjd3 is recruited to transcription start sites (TSSs) that have abundant RNA polymerase II and H3K4me3 (ref. 28). Jmjd3 is reported to fine-tune macrophage activation by controlling Bmp2 and Hox expression26. Furthermore, Jmjd3 has been linked to the control of development through the regulation of Hox, and to oncogenesis through promotion of the expression of Ink4a29,30. Here we report the role of Jmjd3 in vivo in controlling M2 macrophage polarization and identify Irf4 as a Jmjd3 target gene crucial for the regulation of macrophages.
Results
Generation of Jmjd3−/− mice
To investigate the functional roles of Jmjd3 in immune responses in vivo, we generated Jmjd3−/− mice (Supplementary Fig. 1a,b). Reverse-transcription PCR analysis showed that the expression of Jmjd3 was abrogated in Jmjd3−/− mouse embryonic fibroblasts (Supplementary Fig. 1c). Jmjd3−/− mice died perinatally, and adult Jmjd3−/− mice were not obtained (Supplementary Fig. 2a). Histological examination revealed that alveolar cell walls were thickened with tissues, and almost no air space was observed in lungs of Jmjd3−/− neonates (Supplementary Fig. 2b), suggesting that the postnatal lethal phenotype of Jmjd3−/− mice is due to premature development of lung tissues. To analyze the role of Jmjd3 in hematopoietic cells, we obtained fetal liver cells from Jmjd3−/− E15.5 embryos and established bone marrow–chimeric mice. Flow cytometry revealed that populations of T cells, B cells, conventional dendritic cells, plasmacytoid dendritic cells, natural killer cells, neutrophils, F4/80+CD11b+ macrophages and Ly6ChiCD11b+ inflammatory monocytes in the spleen were similar between wild-type and Jmjd3−/− chimeras (Supplementary Fig. 3). Proliferative responses of splenic T and B cells to mitogens and antigen receptor stimuli were also not altered (Supplementary Fig. 4). Furthermore, Jmjd3−/− splenic T cells differentiated into either type 1 or type 2 helper T cells (TH1 and TH2) produced normal amounts of IFN-γ or IL-4, respectively (Supplementary Fig. 5). These results indicate that T cells lacking Jmjd3 are capable of differentiating into TH1 and TH2 cells in response to cytokines.
Jmjd3 is dispensable for M1 macrophages
As Jmjd3 is a TLR-inducible gene in macrophages, we first examined cytokine production of peritoneally resident and thioglycollate-elicited peritoneal exudate cells (PECs) in response to TLR ligands including lipopeptide (Pam3CSK4, a TLR2 ligand), lipopolysaccharide (LPS, a TLR4 ligand), imidazoquinoline analog (R-848, a TLR7 ligand) and oligonucleotide with a CpG motif (CpG-DNA, a TLR9 ligand). Production of TNF and IL-6 in response to TLR ligands did not differ between wild-type and Jmjd3−/− tissue-resident macrophages and thioglycollate-elicited PECs (Fig. 1a–d). PECs elicited by peptone treatment of wild-type or Jmjd3−/− chimeras also produced similar amounts of TNF and IL-6 in response to TLR ligand stimulation (Supplementary Fig. 6a). Flow cytometry revealed that the proportions of F4/80+CD11b+Gr1− cells among resident and thioglycollate-elicited PECs did not differ between wild-type and Jmjd3−/− chimeras (Fig. 1e and Supplementary Fig. 7). The total number of peritoneally resident macrophages, thioglycollate-elicited macrophages and peptone-elicited macrophages was not changed in Jmjd3−/− chimeras (Fig. 1f and Supplementary Fig. 6b). We then examined the role of Jmjd3 in M1 macrophage polarization in response to Listeria monocytogenes infection. When L. monocytogenes was inoculated intraperitoneally, production of proinflammatory cytokines in the sera was similar in wild-type and Jmjd3−/− chimeras (Fig. 1g). F4/80+CD11b+Gr1+ macrophages, F4/80intCD11b+Gr1hi neutrophils, and F4/80−CD11bintB220+ and F4/80−CD11b−B220+ B cells were examined in PECs prepared from L. monocytogenes–infected mice (Supplementary Fig. 8). The number of macrophages recruited to the peritoneal cavity was similar in wild-type and Jmjd3−/− chimeras (Fig. 1e,f). Furthermore, the expression of genes encoding TNF, IL-6, IL-12p40 and iNOS in PECs were similarly upregulated (Fig. 1h). Collectively, these data suggest that Jmjd3 is not involved in the generation and recruitment of M1 macrophages in response to inflammatory reagents and bacterial infection in vivo.
Jmjd3 is needed for M2 polarization to chitin
Chitin is a polymerized sugar and a structural component of helminths, arthropods and fungi31. It has been shown that chitin administration recruits macrophages with the M2 phenotype to the site of administration, which are important for subsequent recruitment of eosinophils32,33. Indeed, we found that intraperitoneal administration of chitin recruited F4/80+CD11b+ macrophages (Fig. 2a), Siglec-F+CCR3+CD4− eosinophils and CD11bintB220+ B cells to the peritoneal cavity after 48 h in wild-type mice (Fig. 2b and Supplementary Fig. 9). Whereas the number of chitin-elicited F4/80+CD11b+ macrophages was similar between wild-type and Jmjd3−/− chimeras (Fig. 2c), the recruitment of eosinophils was severely impaired in Jmjd3−/− chimeras (Fig. 2b–e). Furthermore, the expression of MR on chitin-elicited macrophages was severely impaired in Jmjd3−/− chimeras (Fig. 2f). Chitin-elicited macrophages, but not eosinophils or B cells, expressed high levels of mRNA encoding Arg1, Ym1, Fizz1 and MR, the hallmarks of M2 macrophages (Supplementary Fig. 10). The expression of genes encoding Arg1, Ym1, Fizz1 and MR was considerably lower in chitin-induced PECs obtained from Jmjd3−/− chimeras compared with wild-type controls, whereas expression of the gene encoding iNOS, associated with M1 macrophages, was not altered (Fig. 2g). Of note, the numbers of eosinophils circulating in the blood were similar in wild-type and Jmjd3−/− chimeric mice, suggesting that eosinophil development was not impaired by Jmjd3 deficiency (data not shown). Together, these results suggest Jmjd3 is necessary for M2 macrophage polarization in response to chitin administration.
Role of Jmjd3 in helminth infection
Next we investigated whether Jmjd3 contributes to the responses against helminth infection in vivo. We used the Nippostrongylus brasiliensis infection model, which induces a strong type 2 immune response in the lung. Bronchoalveolar lavage (BAL) staining 5 and 13 d after infection revealed that macrophages were similarly recruited to the lung in wild-type and Jmjd3−/− bone marrow chimeras (Fig. 3a,b). However, the recruitment of eosinophils was severely impaired in Jmjd3−/− chimeric mice (Fig. 3b). To investigate the characteristics of recruited macrophages, we extracted RNA from lung tissue 5 d after N. brasiliensis infection. M2 markers such as Arg1, Ym1, Fizz1 and MR were barely expressed in Jmjd3−/− chimeras (Fig. 3c). Furthermore, induction of genes encoding the eosinophil-recruiting chemokine eotaxin-2 and the TH2-inducing cytokines IL-4 and IL-13 was severely impaired in Jmjd3−/− chimeric mice (Fig. 3c). Thus, we investigated the activation of T cells in the pulmonary lymph nodes 9 d after N. brasiliensis infection. Whereas CD4+ T cells prepared from wild-type pulmonary lymph nodes expressed IL-4, but not IFN-γ, the frequency of IL-4–producing CD4+ T cells was severely impaired in pulmonary T cells prepared from Jmjd3−/− chimeric mice, suggesting that Jmjd3-mediated M2 macrophage activation is crucial for N. brasiliensis to induce TH2 responses in the lung (Fig. 3d,e). However, histological changes in the intestine were not severely impaired in Jmjd3−/− chimeric mice (data not shown). Collectively, our results demonstrate that Jmjd3 is essential for mounting immune responses to helminth infection, directing M2 macrophage polarization in the lung but not in the small intestine.
Role of Jmjd3 in M2 macrophage generation in response to M-CSF
Numerous studies have shown that GM-CSF induces M1 macrophages from bone marrow cells and M-CSF induces M2 macrophages from bone marrow cells12,13,14,15. When we used GM-CSF to generate macrophages, similar amounts of TNF and IL-6 were produced in wild-type and Jmjd3−/− chimeras in response to TLR ligands from adherent CD11b+ macrophages (termed GM-BMMs, for GM-CSF–induced bone marrow–derived macrophages; Fig. 4a,b). In contrast, production of IL-6, but not TNF, in response to TLR ligand stimulation was partially impaired in Jmjd3−/− bone marrow cultured in the presence of M-CSF (M-BMMs; Fig. 4c,d). Furthermore, the expression of genes encoding Arg1, Ym1, Fizz1, MR and IL-13 was severely impaired in M-BMMs from Jmjd3−/− chimeras (Fig. 4e), which indicates that Jmjd3 is crucial for expression of hallmark M2 genes in M-BMMs. Jmjd3 is involved in the response of macrophages to IL-4 stimulation34; nevertheless, Arg1 and Fizz1 gene expression were similar after IL-4 stimulation in wild-type and Jmjd3−/− M-BMMs (Fig. 4f), suggesting that the responses to IL-4 were not impaired in Jmjd3−/− M-BMMs. We then used microarray analysis to examine the gene expression profiles in wild-type and Jmjd3−/− M-BMMs with or without LPS stimulation. The expression of 1,371 genes was more than 50% lower in unstimulated Jmjd3−/− M-BMMs compared with wild-type (Supplementary Table 1). In addition to Arg1, Chi3l3 and Fizz1, the expression of cytokine genes such as Il2, Il3, Il4, Il5 and Il13, as well as that of chemokine genes such as Ccl1, Ccl17, Ccl22 and Ccl24, was severely impaired in Jmjd3−/− M-BMMs (Supplementary Table 1). The expression of 2,188 genes was more than twofold higher in wild-type M-BMMs in response to LPS stimulation, and that of 436 genes was reduced by over 50% in LPS-stimulated Jmjd3−/− M-BMMs (Supplementary Table 2). For example, the expression of Il6 and Il12b was partially impaired in Jmjd3−/− M-BMMs, consistent with a previous report (Supplementary Table 2). Therefore, Jmjd3 is important for inducing expression of a large set of genes, and some of these are related to M2 macrophage polarization in M-BMMs.
In addition to its JmjC domain, Jmjd3 contains a putative tetratricopeptide repeat domain in the N terminus. We therefore examined whether the demethylase activity of Jmjd3 is needed for the defect in M2 macrophage marker expression. We retrovirally expressed the C-terminal part of Jmjd3, containing the JmjC domain (amino acid residues 1141–1641), or its iron binding–deficient mutant (A1388H) in Jmjd3−/− bone marrow cells and then induced M-BMMs (Fig. 4g). The A1388H mutation has been shown to abrogate the H3K27 demethylase activity of Jmjd3 (ref. 26). Expression of the C-terminal part of Jmjd3 was sufficient to upregulate M2 marker genes such as Arg1, Chi3l3 and Mrc1 (Fig. 4h). In contrast, the expression of Jmjd3 (A1388H) did not increase the expression of M2 marker genes, which indicates that the H3K27me3 demethylase activity of Jmjd3 is necessary and sufficient for expression of M2 marker genes in M-BMMs.
M-BMMs require Jmjd3 for cell cycle progression
In addition to the impaired M2 marker expression, the total number of M-BMM cells in Jmjd3−/− chimeras was considerably lower than in the wild type at days 5 and 7 of culture with M-CSF (Fig. 5a), although the number of GM-BMM cells (M1) was not altered. We found 5-bromodeoxyuridine (BrdU) incorporation, a measure of cell division, was severely impaired in Jmjd3−/− M-BMMs compared with wild-type cells (Fig. 5b), whereas wild-type and Jmjd3−/− GM-BMMs incorporated BrdU similarly. These results indicate that Jmjd3 controls cell-cycle progression in response to M-CSF stimulation. Expression of cell cycle–regulatory proteins (c-Myc, c-Myb, cyclin D1 and cyclin D2) was impaired in Jmjd3−/− M-BMMs at day 5 of culture (Fig. 5c); however, the surface expression of M-CSF receptor (CSF-1R) was normal in Jmjd3−/− M-BMMs (Fig. 5d). Furthermore, Jmjd3 deficiency did not affect activation of the intracellular signaling molecules Erk, p38 and Akt, as indicated by their phosphorylation in M-BMMs (Fig. 5e), implying that the cell proliferation defects in Jmjd3−/− M-BMMs are not due to less activation of MAP kinases or Akt. The expression of Jmjd3 in M-BMMs was much higher than that in GM-BMMs (Fig. 5f), suggesting that differential expression of Jmjd3 in M-BMMs and GM-BMMs determines the contribution of Jmjd3 to their proliferation. Together, these data indicate that Jmjd3 performs a key step in the generation of M-BMMs, but not GM-BMMs, by controlling cell proliferation downstream of CSF-1R signaling.
Genome-wide analysis of H3K27me3 controlled by Jmjd3
Next we analyzed the genome-wide distribution of H3K27 trimethylation in wild-type and Jmjd3−/− M-BMMs by chromatin immunoprecipitation–sequencing (ChIP-Seq) analysis. We obtained an overall picture of the H3K27me3 distribution in transcribed regions (based on the genome-wide RefSeq mouse gene annotations in the University of California, Santa Cruz database) and in regions 30 kb upstream and 30 kb downstream. High levels of H3K27me3 tags were detected surrounding TSSs in M-BMMs from wild-type and Jmjd3−/− chimeras (Fig. 6a). In contrast, H3K27me3 levels were low in transcribed loci compared with upstream and downstream regions (Fig. 6a). Notably, H3K27me3 signals at the promoter and downstream regions were higher in Jmjd3−/− M-BMMs compared with wild-type cells.
We then compared the gene expression data obtained by microarray experiments with H3K27 methylation status. Overall, H3K27me3 levels in regions close to the TSS (−5 to +1 kb) correlated negatively with gene expression in M-BMMs (correlation coefficient −0.441; Fig. 6b). Next, we sorted genes by their ratio of expression in wild-type and Jmjd3−/− M-BMMs and examined H3K27me3 levels. However, we did not detect a correlation between H3K27me3 status and the difference in gene expression in wild-type compared with Jmjd3−/− M-BMMs (Fig. 6c). These data suggest that only small numbers of genes were affected by the absence of Jmjd3, and most loci are regulated by Utx or by both Jmjd3 and Utx.
Given the higher concentration of H3K27me3 tags in the region near the TSS, and the lack of overall correlation between expression changes and tag numbers, we focused on the promoter regions of individual genes that showed H3K27me3 peaks. We looked for peaks in wild-type and Jmjd3−/− M-BMM samples and divided the genome-wide set of genes into three different classes depending on H3K27me3 status (Fig. 6d). Class 1 genes harbored an H3K27me3 peak in wild-type M-BMMs. Class 2 genes did not have an H3K27me3 peak in either wild-type or Jmjd3−/− M-BMMs. Class 3 genes such as Irf4 and Tm7sf4 had an H3K27me3 peak in Jmjd3−/− but not in wild-type M-BMMs. We generated a table of 500 genes differentially expressed in wild-type and Jmjd3−/− M-BMMs, comparing H3K27 methylation status and gene expression from the microarray data (Supplementary Table 3).
Although Hox genes, such as Hoxa7, Hoxa9 and Tlx1 (also called Hox11), and Bmp2 have been reported to be regulated by Jmjd3 (ref. 26), the H3K27 of their loci were highly trimethylated in M-BMMs both in the presence and absence of Jmjd3, and they therefore were assigned to class 1 (Fig. 6d and Supplementary Table 3). Furthermore, Hox and Bmp2 gene expression was not lower in Jmjd3−/− M-BMMs (Supplementary Table 3), which indicates that these genes are not crucially regulated by Jmjd3 in M-BMMs. Furthermore, M2 marker genes, such as Arg1, Chi3l3, Rentla and Mrc1, were all in class 2, which indicates that their expression is not directly regulated by Jmjd3 (Fig. 6d and Supplementary Table 3). Thus, we proposed that transcription factors directly regulated by Jmjd3-mediated demethylation are responsible for the polarization of macrophages. When we searched genes categorized in class 3, we found Irf4 and Cebpb. In particular, the promoter region close to the TSS of Irf4 had a high H3K27me3 signal in Jmjd3−/− but not in wild-type M-BMMs (Fig. 6d).
Identification of Irf4 as a Jmjd3 target gene
We confirmed by ChIP analysis that H3K27 at the promoter region of Irf4 is differentially methylated in wild-type and Jmjd3−/− macrophages (Fig. 7a). Furthermore, when we retrovirally expressed the C-terminal region of Jmjd3 or its mutant in Jmjd3−/− macrophages, we found the expression of Irf4 was demethylase activity dependent (Fig. 7b). These results demonstrate that Irf4 is one of the Jmjd3 target genes in M-BMMs. Therefore, we examined the contribution of Irf4 to expression of mRNAs encoding Arg1, Ym1, Fizz1 and MR by using Irf4−/− mice. Induction of M2-related genes was severely impaired in Irf4−/− M-BMMs; in contrast, the expression of Jmjd3 was similar between wild-type and Irf4−/− M-BMMs (Fig. 7c). Notably, the number of M-BMM cells was not lower in Irf4−/− mice (Fig. 7d). When chitin was administered peritoneally, recruitment of eosinophils, but not macrophages, was severely impaired in Irf4−/− mice (Fig. 7e,f). MR expression in chitin-elicited peritoneal macrophages was greatly impaired in Irf4−/− mice (Fig. 7g). In addition, the mRNA expression of the M2 macrophage markers encoding Arg1, Ym1, Fizz1 and MR was severely impaired in chitin-induced macrophages from Irf4−/− mice (Fig. 7h). These results demonstrate that Irf4 is crucial for the polarization of macrophages to M2 in M-BMMs and in vivo in response to chitin administration.
We then retrovirally expressed Irf4 in Jmjd3−/− M-BMMs and examined the expression of M2 marker genes (Fig. 7i). The expression of Irf4 upregulated mRNAs encoding Arg1, Ym1, Fizz1 and MR in Jmjd3−/− M-BMMs, though the expression of Jmjd3 was unaltered (Fig. 7i). These results suggest that Irf4 contributes to the expression of M2 marker genes downstream of Jmjd3.
Discussion
Here we focused on the role of Jmjd3 in macrophages mounting anti-bacterial and anti-parasitic responses. Whereas Jmjd3 was dispensable for M1 macrophage polarization, mice lacking Jmjd3 did not mount proper M2 responses against helminth infection or chitin administration. Furthermore, bone marrow macrophages induced by M-CSF showed demethylase activity–dependent defects in expressing various genes, including M2 macrophage markers. Nevertheless, only a subset of genes had H3K27me3 levels differentially regulated by the presence or absence of Jmjd3. Among these genes, we found Irf4 to be one of the direct targets of Jmjd3-mediated demethylation. Finally, we found that Irf4 is a transcription factor crucial for the induction of M2 macrophage responses.
Although Jmjd3 is a TLR-inducible gene, Jmjd3−/− mice showed vigorous M1 macrophage activation in response to Listeria inoculation. These results suggest that Jmjd3 is not essential for generating and recruiting M1 macrophages to bacterial infections. Our data are consistent with a previous report showing that gene expression in response to LPS stimulation is only modestly changed in macrophages lacking Jmjd3 and that Jmjd3 in this case fine-tunes the transcriptional output28. TLR signaling upregulates genes involved not only in the promotion of inflammation, but also in termination or tissue remodeling. For instance, ATF3 and Zc3h12a are rapidly induced in response to TLR stimulation and inhibit inflammatory cytokine production19,27. It has been shown that M2 macrophages promote tissue remodeling as well as TH2 responses. Thus, it is possible that Jmjd3 induction functions as part of a feedback mechanism acting to repair inflammatory damage caused by TLR stimulation.
Chitin is an abundant structural component of helminths, crustaceans and fungi, and administration of chitin strongly induces M2 macrophage activation. Intraperitoneal administration of chitin recruited M2 macrophages and eosinophils in a Jmjd3- and Irf4-dependent fashion. These results indicate that the Jmjd3-Irf4 axis is essential for M2 macrophage polarization to helminth infection.
However, addition of chitin to the macrophage culture did not stimulate the cells to upregulate M2 marker gene expression in our experiments (data not shown). Although TLR2 has been reported to mediate acute inflammation in response to chitin, another study has shown that chitin-mediated M2 macrophage activation is independent of MyD88, an adaptor molecule used by all TLRs (refs. 32,35). Currently, the mechanism by which chitin activates macrophages is not well understood. Moreover, it is still not clear what unique role Jmjd3 carries out in the generation of M2 macrophages in response to chitin and helminth infection. The identification of the chitin receptor(s) in the future will be vital for clarifying mechanisms of innate immune activation in response to helminth infection.
Jmjd3−/− mice also showed severe defects in recruiting M2 macrophages in response to N. brasiliensis infection. Although it is unknown which components of N. brasiliensis activate innate immune cells, Jmjd3-mediated H3K27me3 demethylation seems to be essential for macrophage responses to this parasite. Further studies are needed to identify the role of Jmjd3 in controlling infection with other helminths pathogenic to humans. M2 macrophages are known to be important for tumor cell survival and tissue remodeling in response to inflammation, in addition to the response against helminth infection36. Thus, it would be interesting to use this mouse model to explore how epigenetic regulation in macrophages promotes cancer progression or wound healing.
A previous report has found that Jmjd3 expression is upregulated in response to IL-4 and that H3K27me3 levels decrease in response to IL-4 stimulation34. We observed that although M-BMMs and chitin-induced peritoneal macrophages showed severe defects in M2 macrophage marker expression in the absence of Jmjd3, Jmjd3−/− M-BMMs were capable of upregulating expression of genes representative of M2 macrophages in response to IL-4 stimulation. These findings suggest that IL-4 acts independently of Jmjd3-mediated H3K27 demethylation to promote M2 polarization. The same report34 showed that H3K27me3 levels of various M2 marker genes were directly controlled by Jmjd3 to activate transcription. In contrast, our ChIP-Seq data demonstrate that H3K27me3 levels of most M2 marker genes, such as Arg1, are not changed in the absence of Jmjd3. Furthermore, deficiency in Irf4, one of the Jmjd3 target genes, resulted in defective M2 responses to chitin administration or M-CSF culture. Thus, it is more likely that Jmjd3 secondarily regulates M2 macrophage polarization by controlling expression of a set of transcription factors.
In addition to M2 marker gene expression, M-BMMs lacking Jmjd3 showed proliferation defects in response to M-CSF stimulation. This is not due to impaired M-CSF receptor expression or defective activation of initial signaling molecules. Although the expression of genes involved in cell-cycle progression, such as those encoding c-Myc, cyclin D1 and cyclin D2, was impaired in Jmjd3−/− M-BMMs, H3K27me3 levels of these genes did not differ between wild-type and Jmjd3−/− M-BMMs. Furthermore, Irf4−/− M-BMMs did not show a defect in cell cycling (data not shown). Thus, it is possible that other Jmjd3 target genes are responsible for controlling the proliferation of M-BMMs.
ChIP-Seq analysis revealed that, in general, differences between wild-type and Jmjd3−/− M-BMM H3K27me3 levels at gene promoter regions were subtle. Nevertheless, gene expression profiles examined by microarray analysis were substantially different in wild-type and Jmjd3−/− M-BMMs, and the responses to chitin or helminth infection in vivo were severely impaired in Jmjd3−/− mice. Although it has been shown that Hoxa and Bmp2 genes are potential targets of Jmjd3 (ref. 26), the expression of these genes was not lower in Jmjd3−/− cells, and the H3K27me3 levels were similar between wild-type and Jmjd3−/− M-BMMs. These results suggest that other H3K27 demethylases such as Utx and Uty compensate for the lack of Jmjd3 in macrophages.
However, we identified Irf4 as one direct Jmjd3-specific target transcription factor. Irf4 has been shown to be involved in TH2 cell polarization as well as in plasma cell differentiation and class-switch recombination in B cells37,38. It has also been reported that Irf4 functions in regulatory T cells to regulate TH2 responses39. Indeed, Irf4−/− mice have been found to show defective TH2 responses to N. brasiliensis infection40. Given that Irf4−/− mice did not induce M2 macrophages in response to chitin administration in our experiments, it is likely that the defects of macrophages in Irf4−/− mice also contribute to their abnormal responses to N. brasiliensis infection. In macrophages, Irf4 functions as a negative regulator of TLR signaling by associating with MyD88 (refs. 41,42).
Jmjd3−/− mice showed neonatal death due to a developmental defect in lung tissue. Although Jmjd3 directly regulated the expression of Irf4 in macrophages, Irf4−/− mice did not show a developmental defect. Thus, Jmjd3 controls genes other than Irf4 in the lung tissues for proper tissue development, and we focused solely on the role of this molecule in macrophages.
It is tempting to speculate that the change in epigenetic status is crucial for determining macrophage polarization. Future development of procedures to specifically regulate Jmjd3 demethylase activity might be useful for manipulating macrophages to mount anti-helminth host defenses and tissue repair.
Methods
Generation of Jmjd3−/− mice.
The Jmjd3 gene was isolated from genomic DNA extracted from embryonic stem cells (GSI-I) by PCR. The targeting vector was constructed by replacing a 4-kb fragment encoding the Jmjd3 open reading frame (exons 14–21, including exons encoding the JmjC domain) with a neomycin-resistance gene cassette (neo), and herpes simplex virus thymidine kinase was inserted into the genomic fragment for negative selection. After the targeting vector was transfected into embryonic stem cells, G418 and gancyclovir doubly-resistant colonies were selected and screened by PCR; recombination was further confirmed by Southern blotting. These homologous-recombinant clones were microinjected into blastocysts derived from C57BL/6 mice and were transferred to pseudopregnant females. Matings of chimeric male mice to C57BL/6 female mice resulted in transmission of the mutant allele to the germline. Resulting Jmjd3+/− mice were intercrossed to generate Jmjd3−/− mice. All animal experiments were done with the approval of the Animal Research Committee of the Research Institute for Microbial Diseases, Osaka University.
Mice, cells and reagents.
Irf4−/− mice were prepared as described41. Bone marrow–derived macrophages were generated in RPMI-1640 medium containing 10% (vol/vol) FCS, 50 μM 2-mercaptoethanol and 10 ng/ml GM-CSF (PeproTech) or 10 ng/ml M-CSF (PeproTech). Pam3CSK4 and R-848 were prepared as described27. LPS (Salmonella minnesota Re595) was from Sigma.
Generation of bone marrow–chimeric mice.
Fetal liver cells were prepared from wild-type and Jmjd3−/− embryos (embryonic day 15.5). The cell suspensions were intravenously injected into lethally irradiated CD45.1 C57BL/6 mice. The chimeric mice were given neomycin and ampicillin in their drinking water for 4 weeks. The mice were analyzed at least 8 weeks after reconstitution. More than 90% of splenocytes from chimeric mice were CD45.2 positive.
Quantitative PCR analysis.
Total RNA was isolated with TRIzol (Invitrogen), and reverse transcription was performed with ReverTra Ace (Toyobo) according to the manufacturer's instructions. For quantitative PCR, cDNA fragments were amplified by Realtime PCR Master Mix (Toyobo); fluorescence from the TaqMan probe for each cytokine was detected by a 7500 real-time PCR system (Applied Biosystems). To determine the relative induction of cytokine mRNA in response to various stimuli, the mRNA expression level of each gene was normalized to the expression level of 18S rRNA. The experiments were repeated at least twice.
Immunoblot analysis.
M-BMMs were cultured for 4 h in medium without M-CSF (PeproTech) and then were collected and replated. M-BMMs were stimulated with M-CSF for times indicated in Figure 5e and were lysed with lysis buffer (20 mM Tris-HCl, pH 7.5, 150 mM NaCl, 1 mM EDTA and 1% (vol/vol) Nonidet P-40) containing complete mini protease inhibitor cocktail (Roche). Cell lysates were separated by standard SDS-PAGE and analyzed by immunoblot. Antibodies to the following proteins were used: phosphorylated Erk (Cell Signaling no. 9101), phosphorylated Akt (Cell Signaling 9271), phosphorylated p38 (Cell Signaling 9211), Akt (Cell Signaling 9272), p38 (Santa Cruz C-20), Erk (Santa Cruz K-23) and β-actin (Santa Cruz C-11).
Flow cytometry.
Antibodies for flow cytometry were purchased from BD Biosciences and eBioscience. Cells were washed in ice-cold flow-cytometry buffer (2% (vol/vol) FCS and 2 mM EDTA in PBS, pH 7.5), then incubated with each antibody for 15 min and washed twice with flow-cytometry buffer. Intracellular cytokines were stained with Cytofix/Cytoperm Plus Fixation/Permeabilization Kit (BD Biosciences) according to the manufacturer's instructions. Data were acquired on a FACSCalibur flow cytometer (BD Biosciences) and analyzed with FlowJo (Tree Star).
Construction of Jmjd3 expression plasmids.
Jmjd3 cDNA (corresponding to amino acid residues 1141–1641) was obtained by PCR from a mouse cDNA library, and a point mutation resulting in the A1388H substitution in the JmjC domain was introduced by site-directed mutagenesis (Stratagene). The full or mutated Jmjd3 cDNAs were cloned into the pMRX-ires-puro vector for retrovirus production43.
Retroviral transduction.
Bone marrow cells were isolated from Jmjd3−/− mice that had been injected intraperitoneally 4 d earlier with 5 mg of 5-fluorouracil (Nacalai Tesque). Cells were cultured in stem cell medium (RPMI supplemented with 15% (vol/vol) FCS, 10 mM sodium pyruvate, 2 μM L-glutamine, 50 μM β-mercaptoethanol, 100 U/ml penicillin, 100 g/ml streptomycin, 100 ng/ml stem cell factor, 10 ng/ml IL-6 and 10 ng/ml IL-3). Then, 48 h later, these cells were transduced with retroviral supernatant (supplemented with stem cell factor, IL-6, IL-3 and 10 ng/ml of polybrene) on two successive days. Virus was produced by PlatE packaging cells transfected with various plasmids. After the second transduction, cells were washed and resuspended in macrophage growth medium (RPMI-1640 medium supplemented with 10% (vol/vol) FCS, 50 μM β-mercaptoethanol, 100 U/ml penicillin, 100 μg/ml streptomycin and 20 ng/ml M-CSF). After 3.5 d in culture, cells were washed once and macrophage growth medium with 2.5 μg/ml puromycin (InvivoGen) was added. The cells were cultivated for 2 d after changing of the medium and then were analyzed.
Chitin administration.
Chitin (Sigma) was washed three times in PBS and then sonicated with a UR-20P device (Tomy) for 30 min on ice. After filtration with 100 μM cell strainer, chitin was diluted in 50 ml PBS. About 800 ng chitin was intraperitoneally injected, and PECs were collected 2 d after administration.
Responses to N. brasiliensis infection.
Wild-type and Jmjd3−/− fetal liver–chimeric mice were subcutaneously inoculated with 300 third-stage larvae of N. brasiliensis 8 weeks after fetal liver transfer. On day 5 after infection, N. brasiliensis–inoculated mice were killed and perfused with PBS, and total RNAs from lungs were extracted. RNA was subjected to quantitative PCR for the analysis of expression of various genes. Nine days after infection, hilar lymph nodes were harvested, a single-cell suspension was prepared and cell numbers were counted. The lymph node cells were stimulated with anti-CD3 and anti-CD28. They were stained with CD4 and treated with cytofix (BD Biosciences), then stained with anti–IL-4 and anti–IFN-γ. Next, the cells were examined by flow cytometry. BAL was performed at 5 and 13 d after N. brasiliensis infection, and macrophages and eosinophils were enumerated on cytospin smears stained with Diff-Quick (Baxter Healthcare).
Microarray and chromatin immunoprecipitation–sequencing analysis.
Microarray and ChIP-Seq protocols and data analysis are described in Supplementary Methods.
Statistics.
Statistical significance was calculated with the two-tailed Student's t-test.
Accession codes.
GEO: microarray data, GSE23180; ChIP-Seq data, GSE23297.
Accession codes
Accessions
Gene Expression Omnibus
GSE23180
GSE23297
|
Annnotations
- Denotations: 482
- Blocks: 0
- Relations: 0