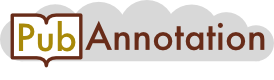
CORD-19:e4c4f6a93633b2ac17771d3386131521bfabf7a6 8 Projects
ANTIVIRAL COMPOUNDS IN THE PIPELINE TO TACKLE H1N1 INFLUENZA INFECTION
Abstract
The recent pandemic of H1N1 has demonstrated the potential vulnerability of the human population to novel influenza viruses. While there is recent increased interest and effort in developing effective anti-influenza agents, few new products have entered clinical studies. This review will highlight the limited armamentarium of licensed influenza agents, and discuss novel compounds and strategies that have entered clinical studies and may therefore be imminently available to the treating clinician.
In March 2009, a novel strain of influenza (A/H1N1 2009) emerged, causing an increase in reports of influenza-like illness in North America. The initial reported cases occurred in Mexico City, which was seen as the epicenter of the influenza pandemic, the world's first in 40 years. Initial reports suggested a high mortality rate (6-7%), although subsequent analysis suggested that these early reports likely inaccurately attributed deaths to novel H1N1, while substantially underestimating the total number of individuals infected with mild cases of the disease. As of May 7, 2010, more than 214 countries and overseas territories or communities worldwide have reported laboratory-confirmed cases of pandemic influenza A/H1N1 2009, including at least 18,001 deaths (1) . In contrast to seasonal influenza, influenza A/H1N1 2009 affects a younger, healthier population. Viral glycoproteins (hemagglutinin [HA] and neuraminidase [NA] ) are the main targets of the immune response to influenza. Vaccines elicit an immune response to these glycoproteins, but as these vary continuously as a result of antigenic drift and shift, and given the time to produce an antigenically appropriate immunogenic product, antiviral drugs are the principal countermeasure to emerging influenza viruses (2, 3) . The currently licensed anti-influenza medications consist of four drugs: two oral adamantanes, amantadine and rimantadine, and two NA inhibitors (NAIs), the oral drug oseltamivir (Tamiflu®) and the inhaled medication zanamivir (Relenza®). Ribavirin, while not licensed for influenza, is available in many countries and does have anti-influenza activity.
The resistance of influenza A viruses to amantadine or rimantadine can occur spontaneously or emerge rapidly during treatment. Most (> 95%) seasonal H3N2 isolates are resistant to these compounds (4), although they still maintain sensitivity to oseltamivir and zanamivir. Most (> 98%) seasonal H1N1 isolates prior to 2009 were resistant to oseltamivir (5) , although they remained sensitive to amantadine, rimantadine and zanamivir. The influenza A/H1N1 2009 isolates are resistant to amantadine and rimantadine (6) , and some isolates have additional resistance to oseltamivir and peramivir (7) .
The limited armamentarium of effective anti-influenza agents and increasing resistance highlight the potential vulnerability of the human population to novel influenza viruses. This has not been overlooked in recent years, as there have been over 2,800 patent publications for aspects of therapeutic anti-influenza agents (8) . The development of anti-influenza agents has occurred in the following areas:
• Adamantane-like compounds (muscarinic acetylcholine M 2 receptor antagonists)
• Antisense, siRNA and miRNA Most compounds and strategies involving these approaches are still in preclinical studies, and only a few have entered clinical studies. Figure 1 shows the mechanism of action of the major compounds/classes in clinical studies. This review will focus on compounds and strategies that have entered clinical evaluation and may therefore be imminently available to the treating clinician. Furthermore, as history suggests that influenza pandemics recur several times a century, strategies that can be applied to both H1N1 and other emerging influenza strains will be reviewed.
The antiviral strategies that are most easily implemented for the novel H1N1 influenza virus are those that utilize existing licensed antivirals.
The trials supporting the use of oseltamivir in the treatment of adults with human influenza who presented within 36 h of developing fever, respiratory symptoms and constitutional symptoms randomized patients to placebo, oseltamivir 75 mg b.i.d. or oseltamivir 150 mg b.i.d. (9, 10) . While the primary endpoint was clinical resolution of symptoms, in both studies oseltamivir treatment was suggestive of decreasing nasal viral titers compared to placebo (although in neither study did this reach statistical significance). In one study this was suggested to be dose-dependent (10) .
Gorvorkova et al. evaluated oseltamivir and other NAIs of avian influenza viruses in a mouse model, and demonstrated a dose-related reduction in virus titers in the lungs for H5N1 and other avian influenza viruses (11) . The maximum suppression was seen with the highest dose tested of 10 mg/kg/day (only tested in avian H9N2 influenza). Other murine studies indicate that higher oseltamivir doses (10 mg/kg/day instead of 1 mg/kg/day) and more prolonged administration (8 days instead of 5 days) are necessary to achieve antiviral and survival effects against a 2004 H5N1 avian influenza virus (12) .
In early development studies in humans, oseltamivir dosage regimens of up to 500 mg twice daily were shown to be well tolerated (13) . In an effort to determine if a higher dose of oseltamivir conveyed clinical benefit, a multicenter study was initiated in Asia and the U.S. in June 2007 to compare standard-vs. high-dose regimens (defined as 150 mg twice daily for adults with normal renal function) of oseltamivir for 5 days for severe seasonal and avian influenza. This study has recently completed enrollment, although results are not yet available (ClinicalTrials.gov Identifier NCT00298233). A separate study comparing 75 mg vs. 225 mg twice daily for 10 days in subjects with influenza in the intensive care unit was initiated in November 2009 (ClinicalTrials.gov Identifier NCT01010087). Pending the results of these studies, definitive recommendations on higher oseltamivir doses for the treatment of H1N1 influenza or other novel strains cannot be supported by clinical data.
The paradigm of combination antivirals to more effectively control viral replication was explored in influenza long before it became an established tenant in the treatment of HIV (14) . In vitro studies of dual antivirals have evaluated amantadine/oseltamivir (15) (16) (17) , amantadine/ribavirin (16, 18) , rimantadine/NAIs (zanamivir, oseltamivir or peramivir) (19) , ribavirin/adamantanes (amantadine or rimantadine) (16) (17) (18) , ribavirin/NAIs (oseltamivir or peramivir) (16, 17, 20) , dual NAIs (17) and combinations involving novel agents such as favipiravir/oseltamivir (21) . Dual antiviral combinations have been evaluated in animal studies, including amantadine/oseltamivir (16, 22) , amantadine/ribavirin (16) , rimantadine/ oseltamivir (23, 24) and ribavirin/NAIs (oseltamivir and peramivir) (15, 16, 25, 26) . In vivo, most dual combinations were synergistic (although some were simply additive). There is no synergy for the oseltamivir/amantadine combination for amantadine-resistant influenza virus (16) . Dual NAIs have shown mixed results, with synergy shown at certain doses, while other studies demonstrated potential antagonism (17) . Combination of amantadine and oseltamivir reduced the emergence of both oseltamivir-and amantadine-resistant viruses in vivo (27) .
Triple antiviral combinations have been shown to increase the antiviral activity (reduction in the EC 50 ) of each drug compared to its activity in double combinations or as single agents (28) . Triple antiviral combinations for viruses resistant to one component (such as amantadine resistance in 2009 H1N1) were shown to have a dose effect of that component, suggesting that incorporation of the resistant compound still contributes to antiviral efficacy (17) .
Oral rimantadine plus either nebulized zanamivir or saline placebo was evaluated in the treatment of adults hospitalized with influenza. Subjects randomized to rimantadine/ zanamivir were more likely to have either no cough or only a mild cough by the third day of treatment, although there was no difference in viral shedding, duration of hospitalization, oxygen requirements or other clinical outcomes (29) .
A phase II trial (ClinicalTrials.gov Identifier NCT00799760) is under way in France to compare the efficacy and safety of combination oseltamivir/zanamivir vs. monotherapy with each agent and will help determine if there is added benefit from combination NAIs. Another study comparing oseltamivir/zanamivir and oseltamivir/amantadine vs. oseltamivir monotherapy should help determine if there is clinical benefit to the synergy seen in vivo (ClinicalTrials.gov Identifier NCT00830323).
Two studies are evaluating triple antiviral therapy. The first is an international multicenter study comparing the antiviral effects of a triple-drug regimen of ADS-890 (a fixed-dose combination of amantadine and ribavirin) and oseltamivir vs. oseltamivir alone (ClinicalTrials.gov Identifier NCT00979251). A second study is evaluating amantadine/ ribavirin/oseltamivir compared to NAI (oseltamivir or zanamivir) monotherapy in immunocompromised patients (ClinicalTrials.gov Identifier NCT00867139). Additional clinical studies of combination therapy are needed. Until results of these studies are available, definitive recommendations on combinations of antivirals cannot be supported by clinical data.
The use of currently licensed medications for the treatment of H1N1 would significantly shorten the development time of these new treatments. Agents in this category would likely have an established safety profile, and potentially would be off patent, which may make the medications inexpensive and therefore more widely available. Only nitazoxanide currently fits this category.
Nitazoxanide is a licensed antiprotozoal agent. The antiprotozoal activity of nitazoxanide is believed to be due to inhibition of pyruvic-ferredoxin oxidoreductase (POR), an enzyme essential for anaerobic energy metabolism (30) . Nitazoxanide is currently licensed for the treatment of diarrhea caused by Giardia lamblia or Cryptosporidium parvum. Nitazoxanide and its metabolite tizoxanide caused concentration-dependent inhibition of influenza virus replication, with EC 50 values of 1 μg/mL for all strains tested (mean C max for licensed dose of nitazoxanide is 10.6 μg/mL) (31) . This class of agents was shown to act at the posttranslational level by selectively blocking the maturation of the viral HA by impairing HA intracellular trafficking and insertion of this protein into the host plasma membrane. Based on these data, a phase II study was initiated in early 2010 for the treatment of uncomplicated influenza (ClinicalTrials.gov Identifier NCT01056380).
The development of new formulations of currently licensed antivirals may shorten development times. There would be safety data on the primary compound, although different doses and C max may portend different toxicities. Additionally, clinicians may be familiar with the compound, increasing acceptability.
It was first reported in 1974 that derivatives of 2-deoxy-2,3-dehydro-N-acetylneuraminic acid (DANA), a sialic acid analogue, were able to inhibit NA activity of influenza A and B viruses (32) . Using a then novel computer-assisted drug design, DANA was optimized for enzyme active-site binding of the influenza NA, with the result being 4-guanidino-2-deoxy-2,3-dehydro-N-acetylneuraminic acid (zanamivir) (33) . Subsequent clinical studies showed an oral bioavailability of zanamivir of only 2%. Although parenteral administration of zanamivir provided a C max 20 times higher than the inhaled formulation (1275 and 54 μg/ L, respectively) (34), the inhaled powder formulation proved sufficient for the treatment of outpatient influenza (35, 36) .
A mouse model of influenza H5N1 suggested that viral shedding and mortality were decreased with increasing doses of zanamivir (37) . Data obtained from subjects with avian influenza suggest that severe disease is associated with viremia (38) . Only 17% of inhaled dry powder zanamivir is systemically absorbed (34) . It is therefore unknown if inhaled zanamivir is sufficient for severe disease. Although initially developed as an inhaled powder, previous clinical studies have evaluated the efficacy of zanamivir in other formulations, including nebulized and i.v. formulations (29, 39) . The recent H1N1 pandemic has reinvigorated interest in a parenteral NAI. A single-arm open-label trial is evaluating the safety data on i.v. zanamivir (ClinicalTrials.gov Identifier NCT01014988). Additionally, i.v. zanamivir is available from GlaxoSmithKline in a compassionate-use expanded-access program.
Serum or plasma treatment of infectious diseases is a special case. Serum therapy was the first effective antimicrobial therapy, preceding the use of sulfonamides or penicillin. The safety of plasma containing influenza antibodies is reasonably well established, as current plasma supplies (for clinical indications) contain these antibodies from seasonal vaccinations and infections. Immune plasma may be collected faster than new small molecules can be developed for therapy, and plasma may therefore be considered as a therapeutic for emerging diseases (40) .
The therapeutic efficacy of F(ab′) 2 fragments was demonstrated in a BALB/c mouse model given a lethal dose of H5N1 virus (41) . In this model, the mice received an i.p. injection of 50, 100 or 200 μg F(ab′) 2 fragments/mouse with normal horse antibody as a control 24 h after infection. Anti-H5N1 F(ab′) 2 at 100 or 200 μg gave 100% protection against death as compared to the antibody-negative control (200 μg of nonimmune equine antibody), with 0% survival. Eleven-week-old mice injected i.p. with 350 μL of mouse immune serum and subsequently challenged with 10 MLD 50 of A/Vietnam/1203/04 demonstrated 100% survival as compared to 8% in the saline controls (42) .
Multiple small clinical trials conducted in the Soviet Union in the 1960s-1980s reported that convalescent plasma and IVIG were effective in the treatment of influenza (43) (44) (45) (46) (47) (48) (49) . These studies were often small and not randomized, but consistent beneficial effects suggested that antibody-based therapies may alter the course of disease enough to afford clinical benefit. A meta-analysis of the use of plasma therapy in 1918 influenza compiled data from 8 studies involving 1,703 patients administered convalescent plasma (50) . The typical volume of plasma or serum administered was 125-250 mL. While these studies were not randomized or blinded, and the intervention was not standardized for volume or HA antibody titer, the overall crude case-fatality rate was 16% (54 of 336) among treated patients and 37% (452 of 1,219) among controls. Efforts to collect plasma with high-titer H1N1 antibodies are currently under way, and a treatment study is scheduled to be open for enrollment by mid-2010 (ClinicalTrials.gov Identifiers NCT00984451 and NCT01052480).
Plasma-based therapies, as they are collected from human donors, are relatively expensive and improved manufacturing costs are not achieved by increasing the economies of scale. Monoclonal antibodies for influenza have the potential for improved costs through economies of scale, although monoclonal antibodies are still in pre-clinical development and have not yet started clinical trials.
Peramivir (also known as BCX-1812 or RWJ-270201) is a highly selective inhibitor of influenza A and B virus NA. Unlike oseltamivir or zanamivir, peramivir has a cyclopentane structure and was designed for improved binding to the active site on the NA enzyme (51) . While preclinical models predicted good bioavailability and once-daily dosing (52, 53) , clinical studies revealed an oral bioavailability of roughly 3% (54) . Large doses were able to overcome this poor bioavailability, with phase II studies showing significantly reduced viral shedding at doses of 400 mg daily (54) .
Given the need for a parenteral NAI agent, clinical studies of i.v. and i.m. peramivir began in 2006. Four i.v. and two i.m. studies have been completed. While none have been published to date, they are summarized in the FDA Fact Sheet for Health Care Professionals. In uncomplicated influenza, a single i.v. dose of peramivir was better than placebo for time to alleviation of symptoms (although this was not true for a single i.m. dose) (55) . In comparison to oseltamivir, peramivir did not demonstrate superiority for the primary endpoint of alleviation of symptoms. Although peramivir is still being evaluated in licensing studies, due to the pandemic with H1N1, the U.S. FDA made i.v. peramivir available through a mechanism called an Emergency Use Authorization (EUA) (56) . This is the first EUA that has been issued for an unapproved drug. As this is an unapproved drug, the use of peramivir is restricted to confirmed 2009 H1N1 infections, especially in subjects who are at high risk or may not tolerate standard antivirals. The development of oseltamivir resistance due to H275Y also confers clinically significant peramivir resistance (7) .
Like other NAIs, laninamivir (previously known as R-125489) is a sialic acid analogue that has activity against a variety of influenza A and B viruses. Laninamivir has a very similar structure to zanamivir, with a methyl substitution for hydrogen in one side-chain. The IC 50 for laninamivir is generally 50-100% higher than that for zanamivir. However, when laninamivir is acylated with a carbon chain at position 3 (forming the prodrug CS-8958), the half-life of the active drug (laninamivir) significantly increases. In a BALB/c mouse model, treatment with zanamivir, laninamivir or CS-8958 4 h before infection with influenza A/PR/ 8/34 showed 0%, 20% and 100% survival, respectively (57) .
CS-8958 is currently being developed as an inhaled formulation. In a dose-escalating phase I study, a single inhaled dose of CS-8958 had a serum half-life of 1.7-29 h but gave a half-life of up to 80 h for the active compound laninamivir (at 80 mg) (58) . Mouse studies suggest that CS-8958 is rapidly metabolized to laninamivir in the respiratory tract, with a lung halflife of 0.8 h (59) . Laninamivir was present in the lung for several days, likely being gradually absorbed, accounting for its long half-life (40 h in mice in this study). Comparatively, laninamivir given i.v. to mice has a half-life of 0.5 h.
Daiichi Sankyo reported results from a phase III study in August 2009, although to date this study has not been published (60) . There were approximately 1,000 adult patients with confirmed influenza A or B who were randomized to receive either 20 or 40 mg of laninamivir as a single inhaled dose or 75 mg of oseltamivir twice daily for 5 days. The primary endpoint of the trial was time to symptom resolution, and both doses of laninamivir were as effective as oseltamivir. A separate phase II/III study was performed in 180 children, again randomized to 20 or 40 mg of laninamivir or oseltamivir 2 mg/kg twice daily for 5 days. It was reported that there was a trend towards a faster time to alleviation of influenza symptoms in the laninamivir groups, but to date the data have not been published.
Cell-surface sialic acids (predominantly N-acetylneuraminic acid) are the host cell receptors for influenza A and B viruses. One potential mechanism to minimize the infectivity of influenza viruses is to remove this target from cell surfaces. DAS-181 is a bacterial sialidase cloned from Actinomyces viscosus coupled with the heparin-binding sequence derived from the human protein amphiregulin to serve as a respiratory epithelium-anchoring domain (61) . DAS-181 has demonstrated activity against 2009 H1N1 and H5N1 isolates, and retained activity against oseltamivir-and zanamivir-resistant viruses (62) (63) (64) . In mouse models with a control group mortality of 57%, DAS-181 given 48 h after infection was 100% effective at preventing death (61) . It was slightly less effective when given at 72 h after infection. Additionally, in mouse models of coinfection with influenza and Streptococcus pneumoniae, treatment with DAS-181 was associated with lower bacterial colony counts (65) . Whether this represents unique properties of a sialidase or simply that lower bacterial counts are associated with lower viral titers is not known. Phase I studies have been completed although not yet reported, and phase II studies are currently under way (ClinicalTrials.gov Identifier NCT01037205).
Favipiravir (previously known as T-705) is a pyrazine analogue (6-fluoro-3-hydroxy-2pyrazinecarboxamide) that inhibits influenza virus RNA polymerase activity in a concentration-dependent, GTP-competitive manner (66, 67) . In a mouse model, oral administration of favipiravir prevented death to a degree comparable with oseltamivir (68). Additionally, favipiravir has shown in vivo efficacy against a range of RNA viruses, including poliovirus, rhinovirus, respiratory syncytial virus, arenaviral hemorrhagic fever, Western equine encephalitis virus and West Nile virus (67, (69) (70) (71) . A phase I clinical trial was initiated in healthy adult volunteers in January 2007 in Japan. A phase II study in infected patients was initiated in October 2009 (clinicaltrials.jp Identifier JapicCTI-090934), and a phase II study in an older population with uncomplicated influenza is set to begin in early 2010 (ClinicalTrials.gov Identifier NCT01068912).
Peri-exposure, nonspecific activation of innate immunity through the use of therapeutic viral mimetics or pathogen-associated molecular patterns (PAMPs), such as poly-ICLC, may provide immediate protection against a broad variety of pathogens, including influenza, while at the same time activating key elements of adaptive immunity and bridging the gap between early nonspecific and longer-term specific host defense systems. Poly-ICLC is a clinically active dsRNA consisting of two homopolymers of poly I and poly C, which is then stabilized with poly-L-lysine. Stabilization increases resistance to endogenous ribonuclease hydrolysis severalfold. Poly-ICLC may act by the induction of dsRNA-dependent 2′-5′oligoadenylate synthase and RNA helicase p68 enzyme systems, or through Toll-like receptor TLR3 (72, 73) . Early downstream effects include induction of interferon beta (IFNβ), interleukins IL-6 and IL-12α, and TNF-α (74) . In a mouse model, two doses of poly-ICLC (1 mg/kg per dose) administered intranasally within 12 days prior to infection with 10 LD 50 of influenza A/PR/8 virus fully (100%) protected the mice against death (75) . Poly-ICLC did not prevent infection, but virus titers by hemagglutination and plaque assays showed more than a 2 log 10 decrease in lung homogenates of pretreated mice compared with those in the lungs of the non-pretreated group. This efficacy was demonstrated up to 14 days prior to infection.
Additionally, animal studies have demonstrated efficacy against a variety of viral pathogens, including SARS, smallpox and viral agents (76, 77) . As the mechanism of action is not dependent on the virus (or even knowing the causative virus in an outbreak), topical poly-ICLC may be an effective first-response medication. This agent can be combined with specific vaccination, antiviral segregation or other intervention programs. Due to this potential, intranasal poly-ICLC has entered clinical studies. A phase I dose-finding and safety study in healthy volunteers has recently completed enrollment (ClinicalTrials.gov Identifier NCT00646152).
In the last 5-8 years, the limited armamentarium of effective anti-influenza agents has become evident, with multiple new strategies in development attempting to improve the treatment of influenza. Most influenza infections are self-limited, although current antivirals fail to mitigate all morbidity and a significant socioeconomic impact is still associated with this disease. As new drugs and strategies come to fruition, it will be critical to define the population where these are best utilized. The influenza A virus replication cycle. Sites of novel antiviral targets are noted by the dark boxes.
|
Annnotations
- Denotations: 60
- Blocks: 0
- Relations: 0