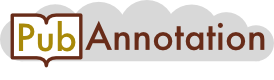
CORD-19:0cd9ee946fc48b03fc5272dc194f4e9fe52ab957 9 Projects
Chapter Outline Introduction and Basic Anatomy of Lymphoid Tissues in the Respiratory Tract 43 Lymphoid Architecture of NALT 44 NALT Development 45 Antigen Acquisition in NALT 46 Immune Responses in NALT 46 NALT and Nasal Tolerance 48 Lymphoid Architecture of BALT 49 BALT Development 50 Antigen Acquisition in BALT 51 Immune Responses in BALT 52 BALT and Asthma 53 BALT and Chronic Obstructive Pulmonary Disease 54 BALT and Autoimmunity 54 BALT and Pulmonary Malignancy 54 References 55 Structure, Organization, and Development of the Mucosal Immune System of the Respiratory Tract INTRODUCTION AND BASIC ANATOMY OF LYMPHOID TISSUES IN THE RESPIRATORY TRACT
Abstract
The respiratory tract is continuously exposed to environmental triggers, including pathogens, nonpathogenic organisms, allergens, particulates, chemicals, and toxins, all of which have to be dealt with in some way. Most of these triggers do not stimulate an immune response and are simply cleared from the respiratory tract without eliciting inflammation or immune recognition. However, immune responses are required to eliminate respiratory pathogens and the respiratory tract has a variety of lymphoid tissues at different sites in the upper and lower respiratory tract that facilitate local antigen uptake and presentation and promote local immune responses that are tailored to provide local immunity.
In the upper respiratory tract of humans, Waldeyer's ring is found at the intersection of the nasal and oral cavities and consists of the adenoid and the paired pharyngeal tonsils at the back of the nasal passages, the paired palatine tonsils to each side of the throat, and the lingual tonsil at the back of the tongue. These tissues are formed during development in the absence of antigen or inflammation, but often become enlarged, often painfully so, in response to local infection. Humans also have nasal-associated lymphoid tissue (NALT), which exists as small clusters of lymphoid follicles underneath the nasal epithelium in various locations on the middle concha of the nasal passages (Debertin et al., 2003) . The number and placement of NALT are not fixed in humans and NALT is not found in every individual (Debertin et al., 2003) , suggesting that this tissue is likely an ectopic lymphoid tissue that forms following antigenic or inflammatory stimulation. Humans may also have nasal polyps, which are formed in response to ongoing inflammatory immune responses. Although nasal polyps exhibit some aspects of lymphoid organ structure (Gevaert et al., 2005) , they primarily contain inflammatory cells and plasma cells and are unlikely to be a primary immune inductive site.
Rodents, the most widely studied animal model of respiratory immunity, lack a structure that is precisely equivalent to Waldeyer's ring. Instead, they have paired subepithelial lymphoid tissues in the nasal passages that are also termed NALT (Asanuma et al., 1997) . However, unlike the NALT of humans, the NALT of rodents is constitutively present, even in the absence of inflammation or infection, and always occurs in the same numbers and location in the nasal passages. The upper respiratory tract of both humans and rodents is also drained by multiple lymph nodes, including the superficial and deep cervical and posterior cervical lymph nodes.
The lower respiratory tract in both humans and mice lacks developmentally formed submucosal lymphoid tissues. However, both humans and mice develop bronchus-associated lymphoid tissue (BALT) in response to inflammation or infection (Moyron-Quiroz et al., 2004; Randall, 2010; Tschernig and Pabst, 2000) . Thus, rodents maintained in sterile laboratory environments typically lack visible BALT, as do most healthy adult humans (Pabst and Gehrke, 1990; Tschernig and Pabst, 2000) . However, both mice and humans develop BALT (otherwise known as inducible BALT, or iBALT) as an ectopic lymphoid tissue that forms in variable numbers and locations, particularly along major airways and blood vessels. The lower respiratory tract of humans is also drained by regional lymph nodes, including the pulmonary nodes, the bronchopulmonary (hilar) nodes, the tracheobronchial nodes, and the tracheal nodes, whereas in rodents, the lower respiratory tract is drained by the mediastinal lymph node. The rest of this chapter will discuss the architecture, development, and specialized functions of NALT and BALT and how these tissues provide immunity to inhaled antigens and pathogens.
In mice, the NALT can be seen in cross-section as paired lymphoid organs on either side of the nasal septum at the base of the nasal passages, directly dorsal to the soft palette of the oral cavity (Asanuma et al., 1997; Harmsen et al., 2002) (Figure 1 ). Each NALT cross-section is triangular, with the nasal epithelium forming one edge of the triangle. In cross-section, a single B cell follicle dominates the center of NALT, whereas a series of B cell follicles can be seen in longitudinal sections. In cross-sections, T cells are found surrounding the follicles in the corners of the triangular tissue; and in longitudinal sections, T cells are found between the follicles, similar to their placement in the interfollicular areas of Peyer's patches. Dendritic cells (DCs) are found in the T cell areas of NALT and are also located just beneath the epithelium, where they are poised to encounter antigens being transported from the lumen of the nasal passages (Rangel-Moreno et al., 2005) . The epithelium overlaying NALT has specialized epithelial cells known as microfold or "M" cells that have the ability to transport antigens and even pathogens from the lumen of the nasal passages to underlying B cells and DCs (Fujimura, 2000; Kraehenbuhl and Neutra, 2000) . The epithelial layer that contains M cells and lies over the B cell follicle is known as the dome epithelium or the follicle-associated epithelium (FAE) .
The lymphoid architecture of NALT is orchestrated by chemokines that are expressed by specialized stromal cell subsets and by the dome epithelial cells of NALT ( Fukuyama et al., 2006; Rangel-Moreno et al., 2005) . Follicular dendritic cells (FDCs) are fibroblastic reticular cells that form a network in the center of the B cell follicle. These cells express and display the chemokine CXCL13 ( Fukuyama et al., 2006; Rangel-Moreno et al., 2005) , which attracts cells, including B cells and T follicular helper cells that express the chemokine receptor CXCR5. The chemokines CCL19 and CCL21 are expressed by stromal cells in the T cell areas surrounding the follicles and attract cells, such as T cells and activated DCs, that express the receptor CCR7. Cells in the dome epithelium also express chemokines, including CCL20 (Rangel-Moreno et al., 2005) , which attracts CCR6-expressing lymphocytes and DCs.
Like all secondary lymphoid organs, NALT has high endothelial venules (HEVs) and lymphatic vessels that allow cells to be recruited from the blood and that allow cells to leave the NALT (Rangel-Moreno et al., 2005) . HEVs are typically found in the T cell areas or at the T cell-B cell FIGURE 1 Cross-section of murine NALT. NALT is seen in cross-section as a single B cell follicle, but consists of a series of B cell follicles that run lengthwise along the nasal passages. The B cell follicle (blue) is situated underneath a dome epithelium (green) that is underlined with a thin layer of DCs (yellow) that are poised to receive antigens transported across the epithelium. The T cell area (red) surrounds the B cell follicle and may also be between B cell follicles. HEVs (gray) are found in the T cell zone and at the border of the T and B cell areas. (See color plate section.) border of NALT. The specialized vascular endothelium of NALT HEVs expresses peripheral lymph node addressin (PNAd) (Csencsits et al., 1999; Ohmichi et al., 2011) , which is normally found on HEVs of peripheral lymph nodes and facilitates lymphocyte rolling adhesion prior to lymphocyte entry to the lymph node. Unlike the HEVs in mucosal lymphoid tissues in the gut, including Peyer's patches and mesenteric lymph nodes, the HEVs in NALT do not express mucosal addressin cell adhesion molecule (MAd-CAM) (Csencsits et al., 1999) . In addition to PNAd, HEVs in NALT also express the chemokine CCL21 (Rangel-Moreno et al., 2005) , the same chemokine expressed in the T cell areas. Together, PNAd and CCL21 efficiently recruit naïve B and T cells from blood. Following rolling adhesion on PNAd-expressing HEVs, the engagement of CCL21 by its receptor, CCR7, on lymphocytes triggers integrin activation, which promotes tight adhesion and arrest on the HEVs. Once arrested, lymphocytes follow a chemokine gradient into NALT and home to their appropriate locations in the B or T cell zones. Efferent lymphatics are located around the outside of the T cell area in NALT and likely collect activated lymphocytes and other cells and direct them to the cervical lymph nodes. NALT does not have afferent lymphatics, since antigen is collected directly from the nasal lumen via the M cells.
The development of lymphoid organs, including Peyer's patches, lymph nodes, and the spleen, has received considerable attention since the early 1990s , particularly following the observation that mice lacking the TNF-related cytokine lymphotoxin (LT) fail to develop lymph nodes and Peyer's patches and have a disrupted splenic architecture (De Togni et al., 1994) . In fact, TNF, LTα, and LTβ are all involved in lymphoid development and organization via their ability to bind the LTβR and the TNFR1 (Futterer et al., 1998; Koni et al., 1997; Pasparakis et al., 1996; Rennert et al., 1996) . Signaling through these receptors promotes (among many things) the expression of the homeostatic chemokines CXCL13, CCL19, and CCL21 by lymphoid stromal cells (Ngo et al., 1999) , and facilitates the organization of lymphoid tissues, including NALT. However, these chemokines, particularly CXCL13, are also expressed during embryonic development at sites of future lymphoid tissue formation (van de Pavert et al., 2009) . Although mature T and B cells that express receptors for these chemokines are not present during embryonic development, a specialized population of innate lymphoid cells, known as lymphoid tissue inducer (LTi) cells (Cupedo et al., 2002) , develop from precursors in the fetal liver via pathways that require the transcription factors RORγt and Id2 (Sun et al., 2000; Yokota et al., 1999) . LTi cells express CXCR5 and CCR7 and home to sites of lymphoid organ development, where they express LTα and LTαβ heterotrimers that engage the TNFR1 and LTβR on local mesenchymal stem cells, causing them to differentiate into lymphoid stromal cells that form the scaffolding of the developing lymphoid organ (Cupedo et al., 2002) . Thus, when any of these pathways are disrupted (for example, in mice that lack CXCL13, CXCR5, LTα, LTβ, LTβR, RORγt, or Id2), the interactions between LTi cells and mesenchymal stem cells are interrupted and lymphoid organs fail to develop .
Not surprisingly, many of these same pathways are involved in the development of NALT (Fukuyama et al., 2002; Harmsen et al., 2002; Ying et al., 2005) . However, NALT formation occurs during a slightly different developmental window relative to lymph nodes and Peyer's patches (Fukuyama et al., 2006) , which seems to allow the development of NALT independently of some of these pathways. For example, NALT development still occurs in the absence of LTα, TNF, TNFR1, and LTβR, even though the lymphoid architecture of NALT is disrupted due to the poor expression of CXCL13, CCL21, CCL19, and CCL20; the failure to form FDC networks; and the lack of PNAd-and CCL21-expressing HEVs. Similarly, the loss of CXCL13 or the combined loss of CCL21 and CCL19 do not abrogate NALT development (Fukuyama et al., 2006; Rangel-Moreno et al., 2005) , but did lead to impaired lymphoid organization. Surprisingly, RORγt-deficient mice, which fail to form LTi cells, generate apparently normal NALT (Harmsen et al., 2002) , whereas Id2 −/− mice, which also fail to form LTi cells, do not generate NALT at all (Fukuyama et al., 2002) . Thus, it is possible that LTi cells capable of inducing NALT development are dependent on Id2, but not RORγt (Kiyono and Fukuyama, 2004) . Alternatively, because NALT development occurs after birth, it is possible that other lymphocytes, like B cells, T cells, or NK cells, have the ability to replace LTi cell function. Intriguingly, Id2-deficient mice, but not RORγt-deficient mice, fail to develop NK cells (Yokota et al., 1999) , suggesting that this population may replace LTi cells in NALT development. Thus, NALT uses pathways of development that are similar, but not identical, to conventional lymphoid organs.
Given that NALT development is initiated after birth, it is possible that exposure to environmental stimuli, although not obligate, may play an important role in the development or at least the maturation of NALT. For example, although NALT is poorly developed in CXCR5-deficient and LTβR-deficient mice, postnatal exposures can promote the formation of PNAd-expressing HEVs and B cell follicle formation-events that are partly dependent on CD40 signaling, indicating that components of the adaptive immune response can facilitate NALT development (Krege et al., 2009) . In fact, even in developmentally normal mice, exposure to nasal antigens, adjuvants, or pathogens dramatically alter the architecture of NALT-germinal centers are induced, HEVs and lymphatic vessels become more numerous, and there are changes to the number and phenotype of B cells, T cells, and DCs in NALT. Thus, NALT requires preprogrammed developmental signals as well as environmental stimuli for its complete maturation.
Unlike lymph nodes, NALT lacks afferent lymphatics and instead acquires antigens by transport across the dome epithelium via specialized M cells (Hsieh et al., 2010) . M cells have evolved mechanisms to transport macromolecules and particles, including viruses and bacteria, across the epithelium, where they can be taken up by underlying DCs and macrophages and recognized by B and T cells (Kraehenbuhl and Neutra, 2000) . Unlike the columnar epithelial cells, M cells lack cilia on the mucosal surface and instead are covered in microfold ruffles-a defining characteristic that allows their unequivocal identification by electron microscopy and provides them with the name "M" cells. The basolateral surface of M cells contains deep pockets in which B cells and other antigen-presenting cells, such as macrophages or DCs, reside and await antigens that have been transcytosed from the nasal passages. Interactions with lymphocytes are an important part of M cell maturation, as the morphology of M cells and the basolateral pocket is lost when lymphocytes are depleted (Ermak et al., 1989) . Although interactions with B cells are not required for M cell development, it appears that they interact with M cells via the engagement of CD137 (a member of the TNFR family) on M cells by its ligand on B cells (Hsieh et al., 2010; Wang et al., 2011) . Although M cells are formed in the NALT of CD137-deficient mice, they lack B cells in their basolateral pockets and fail to transcytose microparticles.
Interestingly, CD137 is inducibly expressed on epithelial cell lines by TNF or LT signaling (Hsieh et al., 2010) , suggesting that interactions with LT-or TNF-expressing cells, such as B cells, also maintain CD137 expression on M cells. This type of back-and-forth interaction between cell types is reminiscent of the interactions between lymphocytes and stromal cells that reinforce homeostatic chemokine expression and maintain lymphoid architecture (Ansel et al., 2000; Ngo et al., 1999) . In fact, LT signaling is also required for the expression of CCL20 by dome epithelial cells (Rangel-Moreno et al., 2005) , possibly M cells, in NALT. Thus, one can envision a scenario in which B cells and antigen-presenting cells in the basolateral pocket of M cells express LT and CD137 ligand and engage their receptors on M cells. In turn, M cells likely express CCL20 and other chemokines to recruit CCR6-expressing B cells and antigen-presenting cells to the basolateral pocket, where they can be supplied with antigen.
Environmental triggers are also thought to play a role in M cell differentiation and maturation. For example, the number of M cells is lower in specific pathogen-free rats than in conventional rats (Jeong et al., 2000) . In addition, exposure to mycobacteria increases the numbers of M cells in the airways (Teitelbaum et al., 1999) , as does exposure to cholera toxin (Wang et al., 2011) . Interestingly, cholera toxin increases the numbers of M cells in the airways without cell division, suggesting that M cells directly transdifferentiate from some type of mature epithelial cell (Wang et al., 2011) .
Given the ability of M cells to acquire antigen and deliver it to underlying antigen-presenting cells and lymphocytes, many investigators have tried targeting antigens directly to NALT M cells in order to generate effective nasal vaccines. For example, influenza hemagglutinin targeted to NALT M cells via a claudin 4-targeting peptide elicits higher nasal IgA responses (Lo et al., 2012) . There is also interest in lectin binding patterns of NALT epithelium and M cells with the hope that specific lectins, such as Griffonia simplicifolia I isolectin B4 (GSI-B4), Dolichos biflorus agglutinin (DBA), and Ulex europaeus agglutinin (UEA) (Giannasca et al., 1997; Takata et al., 2000) , can be used to specifically target antigens to M cells. Despite the potential utility of targeting antigens to M cells via lectins or other proteins, most of these reagents are not truly M cell specific and often bind to other cells like goblet cells. In addition, targeting antigens to M cells is only half the battle in immune induction and investigators are also pairing their favorite vaccine candidates with mucosal adjuvants like cholera toxin (Gizurarson et al., 1991; Porgador et al., 1998; Tamura et al., 1989 Tamura et al., , 1994 , heat-labile toxin IIa-B5 (Lee et al., 2011) , and cryotoxin Cry1Ac (Rodriguez-Monroy and Moreno-Fierros, 2010) to activate M cells and DCs, resulting in enhanced nasal immune responses.
M cells transcytose soluble antigens as well as particles, including viruses and bacteria. In many cases, the organisms transported by M cells are commensal organisms that are part of the normal nasal microbiota and generally do not cause infection. In this case, active transport and immune recognition are probably helpful to elicit noninflammatory IgA responses, which help to constrain commensal organisms to the luminal side of the epithelial barrier. However, some pathogens have taken advantage of this property to hitch a ride across the epithelium. For example, mouse mammary tumor virus (MMTV) (Velin et al., 1997) , Group A streptococcus (GAS) (Park et al., 2003) , and Burkholderia pseudomallei (Owen et al., 2009) all target NALT as a portal of entry.
By analogy to canonical mucosal lymphoid organs like Peyer's patch, one might expect that NALT would promote the differentiation of TGFβ and IL-10-producing T cells and IgA-producing B cells. However, like many aspects of NALT biology, the function of NALT cannot be completely modeled on any other type of lymphoid organ. Although NALT clearly primes IgA-secreting B cells following nasal immunization with a mucosal adjuvant, it also primes IgGsecreting B cells as well as IFNγ-producing CD4 and CD8 T cells in response to some types of intranasal infection or vaccination. Thus, the type of immune response initiated by NALT is often dictated by the type of antigen/adjuvant rather than by an intrinsic property of the tissue.
Nasal immunization with a variety of protein antigens using cholera toxin as a mucosal adjuvant typically elicits IgA-secreting B cells as well as T cells that have an apparently Th2-biased phenotype and express IL-4 and IL-5 Matsuo et al., 2000; Wu et al., , 1996 . Immunization in NALT also promotes longterm IgA responses in the nasal passages (Liang et al., 2001) , as well as in salivary glands and the intestinal lamina propria (Wu et al., 1996; , consistent with the idea of a common mucosal immune system in which immune responses in one mucosal site will lead to a diaspora of IgA-secreting cells to all mucosal sites ( Moldoveanu et al., 1995) .
Intranasal immunization is particularly beneficial in eliciting immunity in the female genital tract (Czerkinsky and Holmgren, 2012; Mestecky and Fultz, 1999) . For example, intranasal immunization against a variety of sexually transmitted diseases, including human immunodeficiency virus-1 (HIV-1) (Peacock et al., 2004) , herpes simplex virus-2 (HSV-2) (Milligan et al., 2004) , human papillomavirus (HPV) (Dupuy et al., 1999; Kawana et al., 2001) , and Chlamydia pneumoniae (Manam et al., 2013) , elicits both B and T cell responses that protect the vaginal tract. Interestingly, although both nasal and vaginal immunization lead to protective immune responses against HSV-2, the protection elicited by nasal immunization is much more dependent on B cells than that elicited by vaginal immunization (Milligan et al., 2004) , suggesting that there are some aspects of local immunization, most likely the generation of resident memory T cells, that cannot be mimicked by intranasal immunization. Moreover, intranasal immunization of males and females may lead to different types of immune responses, as intranasal immunization with HIV-1 peptides followed by a systemic boost using recombinant modified vaccinia virus Ankara elicits optimal protective mucosal responses in the genitals, colon, and lungs of female, but not male, mice (Peacock et al., 2004) . Thus, there are a variety of factors to consider when developing an intranasal vaccine.
Although immunization with cholera toxin typically leads to IgA responses in the NALT, other types of immunizations, such as with protein-containing microparticles, elicit primarily IgG responses (Heritage et al., 1998) . IgG responses in NALT seemed to be generated locally, as they appear in the NALT prior to their appearance in cervical lymph nodes (Heritage et al., 1998) . Later studies with influenza virus (Tamura et al., 1989 and reovirus (Zuercher et al., 2002) show that NALT supports mixed B cell responses consisting of both Ig-and IgA-secreting cells. Although NALT is not committed to exclusively generating IgA-switched B cells, experiments with influenza infection demonstrate that B cells more often switched to IgA in NALT than they do in mediastinal lymph nodes, lung, or spleen (Boyden et al., 2012) . The ability of NALT to promote IgA responses is strikingly documented in a comparison of influenza vaccination with the conventional trivalent inactivated virus (TIV; shot in the arm) vaccine and vaccination with the live attenuated influenza virus (LAIV; intranasal) vaccine. Whereas both vaccines are effective at preventing influenza infection, TIV primarily elicits IgGsecreting cells in spleen and bone marrow, whereas LAIV elicits both IgG-and IgA-secreting cells in the nasal passages and lung (Petukhova et al., 2009; Sealy et al., 2013) . Thus, the ability of NALT to promote IgA-class switching has real consequences in terms of vaccination.
Intriguingly, the bias toward IgA responses in NALT is not simply a matter of directing isotype-switching via the production of particular cytokines like IL-6 or TGFβ (Hiroi et al., 1998; Matsuo et al., 2000) . For example, although nasal immunization with (4-hydroxy-3-nitrophenyl) acetylconjugated chicken gamma globulin with cholera toxin promotes both IgG and IgA germinal center and memory B cell responses in NALT, IgA memory B cells accumulate more somatic mutations characteristic of high-affinity antigen receptors than do IgG memory cells (Shimoda et al., 2001) . Thus, high-affinity, IgA-expressing B cells are somehow preferentially selected into the memory compartment (Shimoda et al., 2001) .
Despite an early focus on B cell responses, NALT is quite capable of generating robust CD8 T cell responses. For example, nasal immunization with a class I-restricted peptide epitope and cholera toxin elicits CTL responses in NALT (Porgador et al., 1998) . Interestingly, NALT DCs are only able to stimulate peptide-specific CD8 T cells when the peptide is administered in the context of cholera toxin (Porgador et al., 1998) , suggesting either that peptide does not enter NALT on its own or that it fails to stimulate DCs such that they can prime CD8 T cells. Various viral infections of the nasal passage, including influenza (Harmsen et al., 2002; Tamura et al., 1998; Wiley et al., 2005) , reovirus (Zuercher et al., 2002) , and Sendai virus , also promote CD8 T cell responses. Moreover, virus-specific CD8 T cells persist in nasal passages longterm following influenza infection (Wiley et al., 2001) . Intriguingly, CD8 responses to intranasal infection with Sendai virus lead to the development of clonally related CD8 T cells in the NALT and lung , suggesting that CD8 T cells primed in NALT may also traffic to the lung. Given that CD8 T cell responses are critical for killing virally infected respiratory epithelial cells and promoting viral clearance in the respiratory tract, it is not surprising that NALT has the ability to prime these T cells and direct their homing to the nasal passages and more distal parts of the respiratory tract.
NALT also promotes immune responses to intranasal bacteria, including GAS (Dileepan et al., 2011; Park et al., 2003) . GAS commonly infects the upper respiratory tract of humans and recent studies in mice show that it preferentially colonizes NALT following intranasal infection (Park et al., 2003) . Nasal infection with GAS elicits CD4 T cell responses that consist of Th1 cells (Costalonga et al., 2009) and Th17 cells (Dileepan et al., 2011) . The differentiation of Th17 cells in response to GAS is dependent on the intranasal route of infection as well as the presence of TGFβ and IL-6 (Dileepan et al., 2011; Wang et al., 2010) , both of which are known to be involved in the differentiation of Th17 cells. Surprisingly, despite the preferential ability of NALT to acquire GAS via M cell-mediated uptake, the CCR6 + CD11c + DCs that reside just beneath the dome epithelium are not involved in the presentation of antigens derived from GAS (Costalonga et al., 2009) .
Although NALT has been shown many times to be an immune inductive organ that recruits naïve B and T cells from the blood and primes them to antigens collected from the nasal passages, it is difficult to distinguish between cells primed locally in NALT and those primed in the cervical lymph nodes that have recirculated back to the nasal passages. However, some experiments have overcome this difficulty. For example, although the architecture of NALT is disrupted in LTα-deficient mice, it can be completely restored by reconstitution of LTα-deficient recipients with LTα-sufficient bone marrow-even though none of the lymph nodes and Peyer's patches reappear following reconstitution (Harmsen et al., 2002) . When intranasally infected with influenza, these NALT-sufficient, lymph node-deficient mice generate normal B and T cell responses to influenza in the nasal passages (Harmsen et al., 2002) . Thus, NALT is sufficient to generate primary immune responses to some types of antigens without help from conventional lymphoid organs.
Although NALT can mediate immune responses to nasal antigens, it is not obligate for immune responses to those antigens. For example, investigators have surgically removed the NALT and showed that nasal immune responses to influenza are essentially intact and that virus is cleared from the nasal passages with normal kinetics (Wiley et al., 2005) . Similarly, the surgical removal of NALT from young mice followed by intranasal vaccination with pneumococcal polysaccharide conjugate does not alter their ability to produce nasal or serum antibody responses or to protect against pneumococcal colonization following nasal challenge (Sabirov and Metzger, 2008) . In contrast, removal of the cervical lymph node does impair antibody production and protection against pneumococcal challenge (Sabirov and Metzger, 2008) . Thus, NALT is sufficient, but not necessary, for nasal immune responses, whereas cervical lymph nodes are important to achieve nasal immune responses of a normal magnitude. One of the most interesting observations to come out of these studies is the idea that some types of antigens, like soluble peptides, can be taken directly to the cervical lymph nodes and bypass the NALT (Porgador et al., 1998) . In fact, this idea is not that surprising, since the epithelial surface of the nasal passages is vastly larger than the epithelium that covers the NALT. Thus, unless antigen is targeted to the NALT by adjuvants, lectins or pathogen-expressed proteins, it may entirely escape detection by the NALT.
Although NALT can clearly act as an immune inductive organ to antigens accompanied by adjuvants or to bacterial and viral infections, the majority of the antigens that enter the nasal passages are likely to be inert. Immune responses to these types of antigens would likely lead to inappropriate inflammatory responses, such as allergic rhinitis (Kim et al., 2012) . Thus, one might envision that NALT would be involved in responding to these antigens in a way that would lead to nasal tolerance. In fact nasal tolerance to inert antigens does occur and involves clonal deletion, clonal anergy, and active immune suppression of responding lymphocytes (Hoyne et al., 1993; Tsitoura et al., 1999) . Moreover, an understanding of how nasal tolerance is established may lead to the therapeutic use of nasal tolerance to restore tolerance to autoantigens and reverse allergy and hypersensitivity to specific antigens.
For example, investigators have used nasal immunization to elicit tolerance to insulin (Fourlanos et al., 2011) , glutamic acid decarboxylase (Wang et al., 2009) , myelin basic protein (Melo et al., 2004) , and cartilage proteoglycan (Broere et al., 2008) , among others (Sun et al., 2010) . There is a general consensus that nasal tolerance elicited by antigen and cholera toxin B (CTB) leads to antigen presentation by B cells, which promote the development of FOXP3-expressing regulatory T cells (Tregs) (Sun et al., 2010) . Once generated, these Tregs may home to sites of inflammation, such as the joints of arthritic mice (Broere et al., 2008) , where they suppress ongoing immune responses. Consistent with the studies in mice, intranasal immunization of humans with a neoantigen leads to systemic tolerance (Mestecky et al., 1996) , but only in a prophylactic setting. Once a systemic immune response is initiated, then therapeutic immunization via the nasal route does not promote tolerance (Mestecky et al., 2005) . Picking the right antigen is also important, as the exposure of early-stage diabetic humans to intranasal insulin prevents the accumulation of anti-insulin antibodies (Fourlanos et al., 2011) , but does not prevent the progression to diabetes. However, the intranasal immunization of recent-onset diabetic mice with proinsulin in combination with systemic anti-CD3 can reverse the course of diabetes (Bresson et al., 2006) . Thus, any tolerance-inducing strategy must take into account the nature of the antigen, the timing of antigen exposure, and the route of administration.
Although NALT may be involved in nasal tolerance, one study shows that the cervical lymph nodes are essential for nasal tolerance (Wolvers et al., 1999) . In fact, the surgical removal of the cervical nodes prior to nasal sensitization prevents nasal tolerance. Moreover, nasal tolerance induction specifically requires the cervical nodes, as the replacement of the cervical nodes with another peripheral node results in immunity rather than tolerance (Wolvers et al., 1999) . Thus, NALT is insufficient to induce tolerance in the absence of cervical lymph nodes.
The early literature describes BALT as a mucosal secondary lymphoid tissue that is constitutively present in the walls of the large airways (Sminia et al., 1989) . However, we now know that BALT formation is not constitutively present in most species and its formation is triggered by infection or inflammation rather than through a preprogrammed developmental process (Gould and Isaacson, 1993; Pabst, 1992; Pabst and Gehrke, 1990; Tschernig and Pabst, 2000) . In addition, the number, size, and architectural complexity of BALT increases in response to microbial or inflammatory stimulation (Delventhal et al., 1992; Sarradell et al., 2003; Tschernig and Pabst, 2000) . In humans, pulmonary bacterial exposure commonly leads to BALT formation, particularly in fetuses, in which BALT is observed 20-40 times more frequently in cases of chorioamnionitis and intrauterine pneumonia than in fetuses with no signs of pulmonary infection (Ersch et al., 2005; Gould and Isaacson, 1993) . Noninfectious exposures to inert inflammatory stimuli, including bacterial lipopolysaccharide (LPS) (Charavaryamath et al., 2005; Vernooy et al., 2002) , diesel exhaust particles (Hiramatsu et al., 2003) , fungi (Seal et al., 1968; Suda et al., 1999) , albumin (Escolar et al., 1994) , and cigarette smoke (van der Strate et al., 2006) , can all lead to BALT formation, although it is not currently understood if the same pathways are involved in each of these circumstances.
As originally described (Sminia et al., 1989) , BALT consists of large follicular structures beneath a classic dome epithelium along major airways (Sminia et al., 1989; van der Brugge-Gamelkoorn et al., 1986a,b) , similar to the placement of Peyer's patches in the small intestine or the placement of NALT in the nasal passages (Kiyono and Fukuyama, 2004) . However, later data suggest that BALT rarely has such a defined structure or precise placement in the lung. Instead, it is located throughout the lung, may not have an identifiable dome epithelium, and may even be located around small pulmonary arteries rather than airways (Moyron-Quiroz et al., 2006 , 2004 (Figure 2 ). In fact, BALT often occupies the capillary-rich perivascular space Tschernig et al., 2002) . Since pulmonary arteries are typically parallel to bronchial airways, the formation of BALT in the perivascular space also tends to place BALT next to airways. Thus, there is a wide diversity of BALT structures that range from fully differentiated lymphoid tissues to isolated B cell follicles without adjacent T cell areas to small clusters of B cells with little apparent organization. Thus, the term BALT is often used to encompass a wide range of tissues in various locations throughout the lung.
B cell follicles in BALT are composed of mature resting B cells (Kocks et al., 2007) , often surrounding germinal centers of activated, proliferating B cells (Chvatchko et al., 1996; Moyron-Quiroz et al., 2004; Rangel-Moreno et al., 2006) . T follicular helper cells are also found scattered throughout the germinal centers , where they provide help to B cells. DCs and macrophages are also found within the follicle, where they may present antigen or facilitate the clearance of apoptotic germinal center B cells. Plasma cells are located around the edge of the follicle or in the T cell zone (GeurtsvanKessel et al., 2009; Rangel-Moreno et al., 2006) . As they do in other lymphoid tissues, the B cell follicles of BALT contain FDCs (Moyron-Quiroz et al., 2004) , which maintain the FIGURE 2 Structure of BALT. Although the structure of BALT varies widely, it is often observed along the major airways in the lung and typically fills the perivascular space surrounding small pulmonary arteries. B cell follicles (blue) are observed underneath the bronchial epithelium, but also away from the epithelium as well. T cell areas (red) surround the B cell follicles and may fill in the space between follicles in larger areas of BALT. HEVs (gray) are observed surrounding the B cell follicles in the T cell zone. (See color plate section.) organization of the follicle and facilitate affinity maturation of B cells responding in the germinal centers. In fact, the presence of FDCs is one way to distinguish non-BALT clusters of B cells in the lungs from true areas of BALT.
T cell zones in BALT are typically found surrounding B cell follicles or between B cell follicles . In some cases, the T cell zone is minimally populated with T cells and those present are widely scattered . In these cases, the structure of BALT consists almost entirely of the B cell follicle. However, the T cell zone is often densely packed with T cells and DCs and appears similar to the intrafollicular T cell zone in Peyer's patches . Plasma cells (GeurtsvanKessel et al., 2009) , macrophages (Hiramatsu et al., 2003) , eosinophils (Lee et al., 1997) , and neutrophils can also be located in or around the outside of the T cell zone. HEVs and lymphatics are found in the T cell zone or sometimes at the boundary between the B cell follicle and the T cell area , 2007b .
As they do in other lymphoid organs, including NALT, homeostatic chemokines control the organization of BALT. CXCL13 is expressed in the center of the B cell follicle on FDCs, where it attracts CXCR5-expressing B cells and T follicular helper cells. Mice lacking CXCL13 still develop B cell areas, but lack a well-organized follicle with FDCs (Rangel-Moreno et al., 2007b). The T cell-attracting chemokines CCL21 and CCL19 are also expressed in the lung (Moyron-Quiroz et al., 2004; Rangel-Moreno et al., 2007b) , and the mRNA expression of CCL19 in particular is increased following infection or inflammation (Khader et al., 2009; Moyron-Quiroz et al., 2004) . However, it has been difficult to obtain histological evidence for the stromal cell expression of CCL19 in BALT, whereas CCL21 seems to be primarily expressed on HEVs and lymphatics ( Rangel-Moreno et al., 2007b) . Nevertheless, the T cell areas of BALT are significantly reduced in plt/plt mice, which lack CCL19 and CCL21 (Rangel-Moreno et al., 2007b) . However, BALT is still formed and still contains some B and T cells, which are separated into distinct zones. In contrast, CCR7-deficient mice not only develop BALT, but have more extensive areas of BALT than do normal mice (Kahnert et al., 2007; Kocks et al., 2007) , possibly due to the failure of regulatory mechanisms (Kocks et al., 2007) . Nevertheless, B cells and T cells are separated into distinct regions in CCR7-deficient mice, suggesting that additional chemokines, such as CXCL12 or others, help to define B and T cell zones in BALT.
HEVs are found around and between the B cell follicles in the T cell areas of BALT, and likely recruit recirculating lymphocytes from the blood. Like HEVs in NALT, HEVs in BALT express PNAd and CCL21, but lack MAdCAM (Moyron-Quiroz et al., 2004; Xu et al., 2003) . Interestingly, HEVs in BALT areas of mice express much higher levels of VCAM-1 than HEVs in other secondary lymphoid organs (Xu et al., 2003) . Consistent with their expression patterns, L-selectin, PNAd, and LFA-1 are important for B and T cell homing to BALT, whereas α4β7 integrin and MAdCAM are not involved (Xu et al., 2003) .
Unlike the development of Peyer's patches or NALT, which occurs independently of infection or inflammation during embryogenesis or immediately after birth, BALT development occurs only following infection or inflammation and is not restricted to a particular developmental window. Nevertheless, some of the pathways for the development of conventional lymphoid organs are also used during BALT development. For example, the homeostatic chemokines CXCL13, CCL19, and CCL21 are all involved in the development and organization of BALT and mice simultaneously lacking CXCL13, CCL19, and CCL21 fail to develop BALT (Rangel-Moreno et al., 2007a) . Interestingly, however, although most lymph nodes fail to develop in CXCL13-deficient mice (Ansel et al., 2000) , BALT forms nearly normally in these mice following influenza infection and has separated B and T cell areas, HEVs, and lymphatics (Rangel-Moreno et al., 2007a) . However, the B cell areas in these mice contain only loose collections of B cells and fail to develop FDCs. In contrast, mice lacking CCL19 and CCL21 (plt/plt mice) form BALT with large B cell follicles but much smaller T cell zones (Rangel-Moreno et al., 2007a) . CCL21-expressing vessels are also smaller in these animals, possibly due to the lack of CCL21 and reduced traffic through the endothelium (Rangel-Moreno et al., 2007a) . Thus, both B cell-attracting and T cell-attracting chemokines are important for BALT formation and play different roles in its architecture.
One striking difference between the development of BALT and other lymphoid organs is that BALT development occurs normally in the apparent absence of LTi cells , as shown using RORγtdeficient and Id2-deficient mice, which lack LTi cells and fail to develop most lymphoid organs (Sun et al., 2000; Yokota et al., 1999) . Despite the lack of LTi cells in these mice, the repeated pulmonary administration of LPS leads to the formation of BALT areas with separated B and T cell zones, FDCs, HEVs, and lymphatics . During the development of conventional lymphoid organs, LTi cells serve as the initial source of LT (Cupedo et al., 2002) , which promotes stromal cell maturation and the expression of homeostatic chemokines like CXCL13, CCL21, and CCL19. However, despite the lack of LTi involvement in BALT development, LT signaling is still required. Although lymphocytes accumulate in the lungs of LTα-deficient and LTβR-deficient mice-often in areas where BALT is normally observed (Futterer et al., 1998 )-B and T cells fail to segregate into separate areas and neither FDCs nor HEVs are found. LT signaling is also essential for the maintenance of BALT, as the blockade of LT in mice with preexisting BALT promotes BALT dissolution (GeurtsvanKessel et al., 2009) .
Although the constitutive expression of CXCL13, CCL19, and CCL21 in conventional secondary lymphoid organs is dependent on LT signaling (Ngo et al., 1999) , these same chemokines are inducibly expressed in the lung independently of LT (Moyron-Quiroz et al., 2004) . In fact, the lungs of naïve mice lack detectable expression of CXCL13 and CCL19. However, upon infection with influenza (Moyron-Quiroz et al., 2004) or Mycobacterium tuberculosis (MTB) (Kahnert et al., 2007; Khader et al., 2009) , or exposure to other inflammatory stimuli like LPS , CXCL13 and CCL19 are inducibly expressed and CCL21 is expressed to much higher levels. Thus, whereas homeostatic LT signaling controls constitutive expression of these chemokines in conventional lymphoid organs, some other inflammation-driven pathway controls the inducible expression of these chemokines in the lung.
In fact, it seems that the IL-17 pathway of inflammation is involved in the inducible expression of CXCL13 and CCL19 and also controls BALT formation in response to inflammatory stimuli. Mice lacking IL-17, IL-22, and IL-23 exhibit impaired BALT formation and reduced expression of both inflammatory and homeostatic chemokines in response to both LPS and MTB . Moreover, IL-17 can directly induce the expression of CXCL13 and CCL19 from cultured pulmonary fibroblasts , the probable precursors to stromal cells and FDCs in BALT. Although IL-17 and IL-22 are both made by LTi cells, particularly in response to IL-23, LTi cells are not required for BALT formation. Instead, γδT cells and αβT cells cooperate in the development of BALT . The most likely scenario is that pulmonary inflammation triggers IL-23 production, which promotes the expression of IL-17 and IL-22 from γδT cells and triggers the expression of CXCL13 by pulmonary fibroblasts. Once CXCL13 is expressed, CXCR5-expressing T follicular helper cells and B cells are recruited to the site of BALT formation and interact with each other and with the local stromal cells. Both B cells and T follicular helper cells express LT and probably contribute to the initial differentiation as well as the maintenance of stromal cells in BALT. Importantly, the requirement for IL-17 in BALT development is limited to the acute inflammatory phase. Once BALT is formed, IL-17 is no longer needed and BALT is maintained by reciprocal interactions between LT-expressing lymphocytes, DC, and chemokine-expressing stromal cells.
In addition to T cells and stromal cells, DCs are also implicated in the maintenance of BALT ( GeurtsvanKessel et al., 2009; Halle et al., 2009) . In particular, the accumulation of CD11b hi MHCII hi DCs following infection correlates with the formation of BALT (GeurtsvanKessel et al., 2009) . Conversely, the depletion of pulmonary DCs in mice that have already formed BALT triggers the dissolution of BALT (GeurtsvanKessel et al., 2009; Halle et al., 2009) . Given that the depletion of pulmonary DCs only modestly reduces LTβ expression and has no effect on either CXCL13 or CCL21 expression (GeurtsvanKessel et al., 2009) , it seems that DCs are performing some other function, such as the production of IL-23, which could facilitate the maintenance of BALT by expressing IL-23 and promoting IL-17 production by T cells. However, IL-17 does not appear to be important for BALT formation in the same model in which DCs are important. Thus, the actual role of DCs in the maintenance of BALT remains uncertain.
Most mucosal lymphoid tissues are located directly beneath an M cell-containing dome epithelium that transports antigens and particles from the mucosal lumen to the underlying lymphoid tissue (Kraehenbuhl and Neutra, 2000) . Consistent with this idea, M cells can be observed in the epithelial layer over some BALT areas (Gregson et al., 1982; Sminia et al., 1989; Tango et al., 2000) . Pulmonary inflammation and antigen stimulation also lead to the differentiation of M cells in the epithelium overlaying BALT (van der Brugge-Gamelkoorn et al., 1986b) . Thus, M cells are likely one way in which BALT can acquire antigen from the airways. However, not all areas of BALT have a dome epithelium and some areas of BALT appear to be exclusively perivascular or are found in the lower airspaces that lack an association with either airways or arterioles. Thus, BALT must have additional mechanisms to acquire antigens.
One possible mechanism for antigen acquisition by BALT is via afferent lymphatics. Lymphatic vessels are observed in the T cell area of BALT and often surround the B cell follicle Rangel-Moreno et al., 2006) . However, it is difficult to assess histologically whether these are afferent lymphatics that bring antigens and cells from distal regions of the lung to BALT or whether they are efferent lymphatics that collect lymphocytes from BALT and return them to the circulation. The possibility that DCs acquire antigen in the airways and then migrate to BALT is supported by histological evidence of DC and macrophages filled with diesel exhaust particles (Hiramatsu et al., 2003) , silica particles (Lee and Kelly, 1993) , cigarette smoke particles (van der Strate et al., 2006) , and other antigens (Halle et al., 2009) , located in the T cell zone of BALT near lymphatics. Importantly, live imaging of ex vivo lung tissue shows that DCs can migrate from the lung airways into BALT (Halle et al., 2009) , suggesting either that DCs migrate directly across the epithelium or that they enter afferent lymphatics that lead to BALT (Halle et al., 2009 ).
In either case, these data demonstrate that pulmonary antigens can be acquired by BALT via other mechanisms that transfer across the epithelium by M cells.
BALT may not always have to acquire antigens externally. For example, BALT supports immune responses to autoantigens , which could be expressed directly in the follicle by DCs or other cell types. In addition, BALT forms at sites of infection and may surround cells infected with viruses or bacteria (Khader et al., , 2009 Moyron-Quiroz et al., 2004) . Similarly, some viruses, such as Epstein-Barr virus or γHV-68, directly infect and establish latency in B cells (Kim et al., 2003) , and if they are reactivated, may represent a source of foreign antigen in the B cell follicles of BALT (Kocks et al., 2007) . Conversely, the reactivity of BALT to environmental antigens may lead to viral reactivation, as is suggested for both Epstein-Barr virus and Kaposi's sarcoma herpesvirus in ectopic follicles in the central nervous system of patients with multiple sclerosis (Franciotta et al., 2008) .
Given that BALT forms following inflammation or infection, it is possible that it is merely a site that accumulates effector B and T cells that are initially primed in conventional lymphoid organs. However, there is now evidence that antigen-specific T and B cell responses can be initiated directly in BALT. For example, mice lacking spleen, lymph nodes, and Peyer's patches generate CD8 T cell responses and isotype-switched B cell responses to influenza with normal kinetics in the lungs (Moyron-Quiroz et al., 2007 , 2004 . Similar studies using splenectomized LTαdeficient mice, which also lack lymph nodes and Peyer's patches, show that immune responses to pulmonary infection with MTB (Day et al., 2010; Kashino et al., 2010) and Leishmania major antigens (Constant et al., 2002) are intact in the absence of conventional lymphoid organs and that antigen presentation occurs directly in the lung.
Later studies directly demonstrate that BALT can recruit and prime naïve T cells. For example, following the induction of BALT by infection with MTB, adoptively transferred ovalbumin (OVA)-specific T cells are primed in the lungs by pulmonary exposure to OVA (Day et al., 2010) . Importantly, T cell priming is intact in the lungs of MTB-infected mice that lack conventional secondary lymphoid organs, but does not occur if BALT is not previously induced (Day et al., 2010) . Similar studies show that after BALT is induced in response to modified vaccinnia virus, adoptively transferred OVA-specific T cells are recruited to BALT and proliferate locally in response to intratracheally administered OVA-pulsed DCs (Halle et al., 2009 ). This study also visualizes the dynamic interactions between T cells and antigen-bearing DCs in BALT explants (Halle et al., 2009) , consistent with the idea that T cells encounter antigen and are primed locally in BALT. Memory T cells are also maintained in BALT and can be locally reactivated (Moyron-Quiroz et al., 2006) , demonstrating that BALT supports both primary and secondary immune responses.
Based on the idea that BALT is a mucosal lymphoid tissue, one might expect that isotype switching in BALT would primarily result in IgA-producing B cells. However, IgGsecreting cells often predominate over IgA-secreting cells in BALT (Plesch et al., 1983; Sminia et al., 1989) , suggesting that BALT does not act like a typical mucosal lymphoid tissue. Nevertheless, IgA can be easily observed under a variety of conditions (Kolopp-Sarda et al., 1994) , including after influenza infection (GeurtsvanKessel et al., 2009 ). Interestingly, BALT seems to generate IgA-secreting plasma cells that home to the lung as well as IgG-secreting plasma cells that home to the bone marrow (GeurtsvanKessel et al., 2009) . Importantly, the isotype-switched plasma cells generated in BALT are long-lived and maintain virus-neutralizing antibodies in the serum and bronchial lavage fluid that can completely prevent infection following a secondary challenge (Moyron-Quiroz et al., 2006) . Isotype switching in BALT is not restricted to IgG and IgA, since IgE is often found in BALT areas of patients with allergies (Slavin et al., 1992) or in experimental animals that have been sensitized to antigens under Th2-inducing conditions (Chvatchko et al., 1996; Gajewska et al., 2001) . Thus, isotype switching in BALT is primarily directed by the type of immune response rather than by the mucosal nature of the tissue.
In addition to its ability to support primary B and T cell immune responses, BALT seems to modify those responses in ways that reduce pulmonary pathology. For example, preexisting BALT elicited by protein nanoparticles reduces morbidity and mortality in response to a number of viruses, including influenza, SARS coronavirus, and mouse pneumovirus (Wiley et al., 2009) . Similar results are found in mice that lack conventional lymphoid organs but have BALT (Moyron-Quiroz et al., 2004) , suggesting that on its own, BALT is able to initiate pulmonary immune responses that are faster and more protective than those initiated in systemic sites.
BALT is also important for immunity to MTB. Pulmonary infection with MTB leads to the development of granulomas, which consist of dense aggregates of activated lymphocytes and other cells surrounding infected macrophages in the lungs. Lymphoid clusters reminiscent of BALT, with well-defined B cell follicles, FDCs, and germinal centers, are formed around the granulomas in both humans (Ulrichs et al., 2004) and mice (Kahnert et al., 2007) . The importance of BALT for local immunity to MTB is demonstrated in two studies showing that mice lacking conventional lymphoid organs efficiently form granulomas, generate MTB-specific IFNγ-producing CD4 T cells in BALT following pulmonary challenge with MTB, and control infection (Day et al., 2010; Kashino et al., 2010) .
Interestingly, the pulmonary expression of both CCL19 and CXCL13 progressively increases following MTB infection and keeps pace with the formation of granulomas and BALT (Kahnert et al., 2007; Khader et al., 2009) , suggesting that these chemokines may play a role in immune responses to MTB. In fact, CXCL13deficient mice and plt/plt mice (lacking CCL19 and CCL21), as well as CXCL13-deficient x plt/plt mice, exhibit disrupted BALT architecture and delayed granuloma formation (Khader et al., 2009) . Interestingly, the architecture of granulomas and T cell placement is disrupted in CXCL13-deficient mice, whereas granuloma formation is apparently normal in plt/plt mice, but the differentiation of Th1 cells is delayed (Khader et al., 2009) . Importantly, mycobacterial titers are higher in the lungs of both CXCL13-deficient mice and plt/plt mice and are even higher in the lungs of CXCL13-deficient x plt/plt mice (Khader et al., 2009) .
Given that IL-17 is important for iBALT formation via the induction of CXCL13, it is not altogether surprising that IL-17 is also important in immunity to MTB following vaccination. In fact, pulmonary vaccination of mice with an MTB antigen in combination with a mucosal adjuvant, type II heat-labile enterotoxin, elicits MTB-specific Th17 cells . Upon challenge infection, memory Th17 cells promote the expression of CXCL13 in the lung, which allows the recruitment of CXCR5-expressing T cells to BALT areas Slight et al., 2013) . As a result, MTB-specific T cells are properly placed to promote early and efficient macrophage activation and control of infection.
Given that BALT can be formed in response to inflammation, it is also likely to be involved in some types of inflammatory diseases, such as asthma. However, studies comparing transbronchial biopsies from young adult asthmatics and controls found no correlation between the presence BALT and a history of respiratory allergy or asthma (Sue-Chu et al., 1998) . Similarly, the presence of BALT in infants does not correlate with lung function or atopy (Heier et al., 2008) . However, the reactivity of BALT does increase in asthma patients (Elliot et al., 2004) , with larger BALT areas found in subjects with asthma compared to either nonsmokers or smokers. In addition, exposure to allergens, such as Aspergillus fumigatus, can lead to an asthma-like disease that is accompanied by the development of BALT in which IgEexpressing germinal centers are prevalent (Slavin et al., 1992) . Similar results, including the formation of IgEexpressing germinal centers, are observed in mice following sensitization and pulmonary challenge with OVA (Chvatchko et al., 1996) .
The ability of the lung to locally prime Th2 responses in the absence of conventional lymphoid organs is addressed in two studies that come to opposite conclusions. Both studies expose splenectomized LTα-deficient mice, which lack lymph nodes, Peyer's patches, and spleen, to pulmonary antigens. In one study (Gajewska et al., 2001) , repeated aerosol administration of OVA in the context of pulmonary granulocyte-macrophage colony-stimulating factor overexpression produces Th2 inflammatory responses in the lungs of normal mice, but completely fails to elicit pulmonary Th2 responses in mice lacking conventional secondary lymphoid organs, suggesting that local priming in the lung is insufficient to elicit pulmonary Th2 responses. In the second study (Constant et al., 2002) , exposure of normal and splenectomized LTα-deficient mice to Leishmania major parasites followed by pulmonary challenge with Leishmania major elicits robust Th2 responses in both groups, including the production of IL-5 and IL-13 and the accumulation of eosinophils. Although BALT is not defined histologically in this study, large numbers of antigen-bearing DCs remain in the lungs following challenge. Thus, they conclude that Th2 responses can be initiated directly in the lung (Constant et al., 2002) . The difference between the conclusions of these studies is striking and is likely due to the differences in the antigens used to prime Th2 responses.
Transgenic mice expressing IL-5 in the lung exhibit pulmonary pathology that is reminiscent of asthma-even in the absence of allergen exposure. Pathologic features of these mice include a dramatic eosinophilia, goblet cell hyperplasia, and epithelial cell hypertrophy (Lee et al., 1997) . These mice also develop extensive areas of BALT. Interestingly, eosinophils are clustered around the B cell follicles of BALT, in the same location where T cells and DCs are normally located (Lee et al., 1997) . These mice also exhibit airway hyper-responsiveness, independently of any antigenic challenge, suggesting that the pathologic changes induced by IL-5 overexpression directly trigger this asthma-like condition.
Mice expressing an IL-6 transgene in the lung also develop BALT (Goya et al., 2003) , but without the accumulation of eosinophils. The BALT in IL-6 transgenic mice consists primarily of primary B cell follicles without germinal centers. T cells, particularly CD4 T cells, are present around and between B cell follicles, whereas plasma cells are observed around the circumference of BALT (Goya et al., 2003) . Although pulmonary function is not measured in this study, there are no visible changes in the overall lung architecture (other than the accumulation of BALT) that might be suggestive of an asthma phenotype. Thus, the development of BALT per se does not directly lead to asthma or even lung disease, but the presence of BALT may participate in ongoing Th2 responses and exacerbate preexisting asthma.
The exposure to cigarette smoke is an important risk factor for developing chronic obstructive pulmonary disease (COPD) (Pauwels and Rabe, 2004) . Interestingly, the frequency of BALT in the lungs of smokers is higher than that in nonsmokers. Similarly, smokers with significant airway obstruction show an increased frequency of BALT areas relative to smokers without airway obstruction (Bosken et al., 1992) . The frequency of BALT areas with reactive germinal centers is also higher in smokers than in nonsmokers (Elliot et al., 2004) , and exposure to cigarette smoke increases BALT area and contact with epithelium in rats by nearly fourfold (Escolar Castellon et al., 1992) . In fact, the development of BALT is most strikingly observed in patients classified with GOLD stage 3 and stage 4 disease (Hogg et al., 2004) . Importantly, changes in the forced expiratory volume in 1 s (FEV 1 ) correlate strongly with the percentage of airways with lymphoid follicles, the percentage of airways with B cells, and the accumulated volume of B cells in the lungs (Hogg et al., 2004) , suggesting the possibility that BALT plays an important role in COPD.
Consistent with the idea that cigarette smoke is directly linked to BALT formation, cells filled with black pigmentpresumed to be from smoke exposure-are found clustered around the edge of the T cell areas of BALT (van der Strate et al., 2006) . These cells likely correspond to macrophages or DCs that have phagocytosed cigarette smoke particles in the airways and then migrated to the BALT areas. Importantly, B cell follicles in the BALT of COPD patients often contain germinal centers, many of which have evidence of clonal B cell populations and ongoing somatic hypermutation (van der Strate et al., 2006) , suggesting that the B cells in BALT are responding to antigen and may contribute to pathology. In opposition to the idea that B cells and BALT contribute to the pathology of COPD, other studies show that exposure to cigarette smoke leads to the development of pulmonary emphysema to the same extent in normal and SCID mice, even though SCID mice completely lack B cells and BALT (D'Hulst A et al., 2005) . Thus, although exposure to cigarette smoke promotes the formation of BALT in experimental model systems and BALT is associated with severe COPD in human patients, the causal link between BALT and COPD remains nebulous and it is unclear whether BALT is beneficial, harmful, or neutral to the progression of COPD (Brusselle et al., 2009 ).
Rheumatoid arthritis (RA) and Sjogren syndrome (SS) are two autoimmune diseases that are associated with the development of ectopic lymphoid follicles in affected target tissues such as the joints (Takemura et al., 2001a,b) , and salivary glands (Gatumu et al., 2009) . These follicles are thought to be involved in autoimmunity by generating highaffinity autoreactive B cells and by maintaining autoreactive effector T cells. In addition, a subset of RA and SS patients develop pulmonary symptoms prior to systemic manifestations and often have more severe disease and a worse longterm prognosis (Turesson et al., 2002) . These patients also develop BALT areas with extensive germinal centers and FDC networks (Atkins et al., 2006; Rangel-Moreno et al., 2006; Turesson et al., 2005) . Additional studies show that some areas of BALT in RA patients have a prominent dome epithelium and IgA-expressing B cells (Hayakawa et al., 1998; Sato et al., 1996) . However, BALT areas in many RA patients are not associated with a dome epithelium and in some cases seem to be encapsulated by lymphatic endothelium.
The B cell follicles of RA and SS patients seem to contribute to local disease, since they contain plasma cells producing IgG specific for citrullinated proteins and rheumatoid factor . Interestingly, there is a strong association between RA and smoking (Klareskog et al., 2006) , which may be explained by the induction of the citrullination process by cigarette smoke. Moreover, citrullinated proteins are observed in lymphoid areas of the lungs of RA patients (Bongartz et al., 2007) , suggesting that autoantigens are located inside BALT areas. Interestingly, a 2013 study shows that BALT forms in arthritis-prone autoimmune K/BxN mice, which have glucose-6-phosphate isomerase-specific B cells and T cells (Shilling et al., 2013) . Surprisingly, however, when challenged with bleomycin to induce pulmonary fibrosis, the K/BxN mice failed to elicit a significant fibrotic response (Shilling et al., 2013) , suggesting that the presence of BALT actually reduced pulmonary pathology. Thus, it is not clear whether the BALT found in patients with RA is exacerbating pulmonary pathology or may actually be preventing more severe disease.
Given the role of BALT in local immune responses to pulmonary pathogens and antigens, one might predict that the development of BALT adjacent to pulmonary malignancies would promote antitumor immune responses. In fact, BALT sporadically forms in the vicinity of lung tumors , including non-small cell lung carcinoma (Dieu-Nosjean et al., 2008) . In these patients, the development of BALT is associated with a better long-term prognosis (Dieu-Nosjean et al., 2008) . Interestingly, the density of DCs in BALT was the only feature to significantly correlate with long-term survival, perhaps suggesting that DCs promote CD8 T cell priming to tumor antigens.
Given the role of LT in the development of ectopic lymphoid areas, it is not surprising that LT:antibody fusion proteins can be used to simultaneously engage tumor antigens and the TNFR1 and promote tertiary lymphoid tissue neogenesis (Schrama et al., 2001) . The formation of tumor-associated lymphoid tissue is accompanied by clonal expansions of tumor-specific T cells (Schrama et al., 2001) . Targeting LT to the tumor site is equally effective in mice that completely lack conventional lymph nodes, suggesting that local immune responses are sufficient for tumor eradication.
Although BALT may trigger antitumor immune responses and promote tumor regression, other studies show that BALT may also contribute to tumor metastasis in the lung. For example, intravenously injected prostate tumor cells metastasize to BALT regions in rats (Geldof and Rao, 1989) , suggesting that specialized vasculature and specific homing molecules may recruit tumor cells to BALT and lead to metastatic lesions. These data are consistent with other results showing that inflammatory stimuli, such as cigarette smoke, can increase the efficiency of pulmonary metastases (Lu et al., 2007) .
Although BALT may initiate antitumor immunity or promote tumor metastasis, mucosa-associated lymphoid tissue (MALT) lymphomas may also masquerade as BALT. MALT lymphomas are clonal B cell malignancies and, when found in the lung, are referred to as BALT lymphomas (Isaacson and Wright, 1983) . These tumors present diagnostic problems for pathologists due to their heterogeneous appearance and similarity to conventional BALT (Koss, 1995) . Most BALT lymphomas are of low histologic grade and have a relatively benign clinical behavior, typically leading to good clinical prognosis. Morphologically, BALT lymphomas often appear as dense B cell follicles (Kurtin et al., 2001) , mixed with stromal-type cells that are likely equivalent to FDCs (Koss, 2004) . Some BALT lymphomas even contain histologically defined germinal centers (Koss, 2004; Kurtin et al., 2001) . T cells are often found around the outside of BALT lymphoma follicles (Koss, 2004) , similar to their placement in conventional BALT. Most BALT lymphomas interact with the bronchial epithelial cells and form a structure similar to the dome epithelium of BALT ( Kurtin et al., 2001) . Thus, many features of BALT lymphoma lesions correspond to the architecture of normal BALT (Harris and Isaacson, 1999) .
The most common MALT lymphoma, gastric lymphoma (Isaacson, 1999) , is associated with Helicobacter pylori infection (Go and Smoot, 2000) , and lymphomagenesis is directly associated with infection, as antibiotics can often induce tumor regression. Thus, chronic inflammation, as well as antigen stimulation and T cell help, seem to cooperate to trigger tumorigenesis (Isaacson, 1999) . Similar events are likely to occur in BALT lymphoma; however, the etiology of the disease is not as clear-cut (Koss, 2004) . For example, some BALT lymphomas are associated with underlying autoimmune diseases, such as SS (Ingegnoli et al., 2008) , systemic lupus erythematosus (Gayed et al., 2009) , and RA (Bende et al., 2005) . In contrast, the development of some BALT lymphomas is associated with chronic mycobacterial infections, including infection with Mycobacterium avium and M. tuberculosis (Gaur et al., 2004; Inadome et al., 2001) . This observation may not be altogether surprising, given the association of pulmonary mycobacterial infection with the development of BALT in both mice and humans (Kahnert et al., 2007; Ulrichs et al., 2004) . Interestingly, a microbiome analysis of BALT lymphomas revealed that bacteria of the Alcaligenaceae family is associated with 8 of 9 samples (Adam et al., 2008) . Although further studies are required to establish a connection between pulmonary exposure to bacteria of the Alcaligenaceae family and lymphomagenesis, there is likely to be some connection between chronic inflammation or infection and the development of BALT lymphoma.
|
Annnotations
- Denotations: 163
- Blocks: 0
- Relations: 0