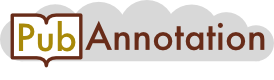
CORD-19:05082393ba4c7ec530190dd887d99c74fd72f6d6 8 Projects
Self-assembly of the RZZ complex into filaments drives kinetochore expansion in the absence of microtubule attachment
Abstract
To examine the role of the kinetochore dynein module (RZZ-Spindly-dynein-dynactin) in kinetochore expansion, we incubated HeLa cells with nocodazole to depolymerize microtubules and used immunostaining for the outer kinetochore proteins CENP-E and CENP-F to assess crescent formation (Fig. 1A) . In cells treated with control siRNA, CENP-E and CENP-F expanded into crescents that partially encircled the compact inner kinetochore, marked by CENP-C, as expected (Fig. 1B) . Depletion of the RZZ subunit ROD by RNAi, which eliminated Spindly localization to kinetochores, supported CENP-E and CENP-F recruitment, but kinetochores no longer expanded into crescents (Fig. 1B) . Measurements of kinetochore fluorescence confirmed that ROD depletion reduced both the volume occupied by CENP-E and CENP-F and their overall levels ( Fig. 1C -F) . Depletion of Spindly also reduced kinetochore expansion, albeit not to the same degree as depletion of ROD ( Fig. 1B -F) . By contrast, depletion of the dynactin subunit DCTN1, which prevents kinetochore recruitment of both dynactin and dynein, did not affect kinetochore expansion, as judged by immunostaining for Spindly ( Fig. 1G -I) . We conclude that kinetochore expansion requires RZZ and Spindly but is independent of dynein-dynactin.
Human Spindly directly interacts with the ROD b-propeller [16] , and the b-propeller is essential for Spindly recruitment to kinetochores [15] . The atomic-resolution structure of the RZZ subunit ZWILCH, which forms a tight complex with the ROD b-propeller, features a conserved surface-exposed patch of unknown function in domain 2 [29] (Fig. 1J ). In C. elegans, two acidic residues in this patch are required for an interaction between ZWL-1 Zwilch and SPDL-1 Spindly [15] .
We used the Flp-In system in HeLa cells and RNAi-based molecular replacement to assess the consequences of introducing analogous mutations (E422A/D426A) into human ZWILCH (Fig. 1J ).
Expression of transgene-encoded GFP::ZWILCH, harboring silent mutations that confer RNAi resistance, supported recruitment of Spindly to kinetochores and facilitated kinetochore expansion in nocodazole, as judged by immunostaining for GFP and Spindly (Fig. 1K -M) . By contrast, GFP::ZWILCH(E422A/D426A), while supporting Spindly recruitment to kinetochores, inhibited kinetochore expansion to a similar degree as depletion of ROD ( Fig. 1K -M) . We conclude that kinetochore expansion requires two conserved acidic ZWLICH residues that in C. elegans ZWL-1 Zwilch are implicated in an interaction with SPDL-1 Spindly .
The expanded outer kinetochore is a distinct multi-protein domain that can be dissociated from the KMN network
We found that, following a 4-h incubation in nocodazole, a 30-min treatment of mitotic cells with the CDK1 inhibitor RO-3306 resulted in complete detachment of the expanded outer domain from the centromere ( Fig. 2A) . Immunostaining showed that crescent-like assemblies in the cytoplasm, separated by several µm from the closest sister kinetochore pair, marked by CENP-C or human anti-centromere antibodies (ACA), contained ZW10, ZWILCH, Spindly, the dynactin subunit DCTN1, CENP-E, MAD1, and MAD2 (Fig. 2B, D) . By contrast, we could not detect KNL1, BUB1, BUBR1, BUB3, CENP-F, HEC1, DSN1, or CENP-C on detached crescents ( Fig. 2D; Fig. S1 ). Consistently, detached crescents were never observed after depletion of ROD or Spindly.
We conclude that kinetochore expansion involves the assembly of a distinct multi-protein domain with defined composition that is separable from the inner kinetochore and the KMN network.
The robust presence of MAD1-MAD2 on detached kinetochore crescents in the absence of detectable KNL1 or BUB1 implied that RZZ is directly involved in MAD1-MAD2 recruitment.
Interestingly, when we generated detached crescents in cells expressing transgene-encoded GFP::ROD on top of endogenous ROD, CENP-F, BUBR1, and BUB3 were consistently detected on detached crescents (Fig. 2C, D) . Thus, over-expression of GFP::ROD recruited outer kinetochore components that were normally not present at detached crescents. Of note, prior work showed that CENP-F has affinity for recombinant GFP::RZZ [16] , suggesting that RZZ may promote CENP-F recruitment though a direct interaction. Regarding the SAC, these results imply that RZZ is capable of recruiting MCC components to the expanded outer kinetochore independently of KNL1-BUB1.
To test whether over-expression of GFP::ROD promotes kinetochore expansion per se, we expressed GFP::ROD under conditions that usually compromise expansion. Consistent with Strikingly, expression of GFP::ROD in KNL1-depleted cells produced robustly expanded kinetochores (Fig. 3B) . Indeed, GFP::ROD-containing crescents in KNL1-depleted cells were often larger than GFP::ROD crescents in cells treated with control siRNA and occasionally connected kinetochores of adjacent chromosomes (Fig. 3C) . GFP::ROD-containing crescents and rings that were partially or even fully detached from sister kinetochore pairs were also prevalent in KNL1-depleted cells but were never observed in cells treated with control siRNA (Fig. 3B , C). The protein composition of detached GFP::ROD crescents was identical to the detached crescents generated by acute CDK1 inhibition ( Fig. 3C and data not shown). As expected, codepletion of KNL1 and Spindly suppressed kinetochore expansion in cells expressing GFP::ROD ( Fig. 3D) . We also examined the effect of a GFP::ROD mutant missing its N-terminal b-propeller domain (D1-375), which we previously showed localizes to kinetochores but prevents recruitment of ZWILCH and Spindly [15] . GFP::ROD(D1-375) did not support expansion, as we pointed out previously [15] , and depletion of KNL1 greatly reduced the kinetochore signal of the ROD mutant compared to cells treated with control siRNA (Fig. S2 ).
Taken together, these experiments suggest that increased ROD levels facilitate robust kinetochore expansion even when kinetochores have a reduced capacity for RZZ recruitment, and that this depends on Spindly and the ROD b-propeller. This is consistent with an expansion mechanism based on self-assembly of RZZ, for which we present direct evidence below. The GFP::ROD(D1-375) signal likely represents the RZZ pool directly recruited by KNL1-BUB1 without expansion, while the GFP::ROD signal also reflects higher-order assemblies of RZZ. These experiments also show that KNL1-BUB1 are required to tether the expanded outer domain to the core kinetochore.
Rod proteins share a common architecture with membrane coat precursors, which selfassemble into higher-order structures [16] . Self-assembly of ROD into filaments could underlie the central role of ROD in kinetochore expansion that we describe above, but there is currently no evidence that Rod proteins can form oligomeric assemblies. In our search for such evidence, we turned to the nematode C. elegans. In mature oocytes and meiosis I embryos, small filamentous assemblies, referred to as 'linear elements', are present in the cytoplasm and The copyright holder for this preprint (which was not peer-reviewed) is the author/funder. It . https://doi.org/10.1101/282707 doi: bioRxiv preprint S3). Depletion of ROD-1 prevented the formation of linear elements (Fig. S3A ), suggesting that they may represent oligomeric assemblies of ROD-1. Intriguingly, we found that after tagging endogenous ROD-1 with GFP using CRISPR/Cas9-mediated genome editing, GFP::ROD-1 filaments were not only present in meiosis but also appeared in the cytoplasm of early multicellular embryos (Fig. 4A) . Live imaging revealed that µm-scale GFP::ROD-1 filaments formed with highly reproducible kinetics during mitosis at the 8-cell stage, but not in earlier divisions ( Fig. 4A ; Movie S1). In addition to forming filaments, GFP::ROD-1 localized transiently to holocentric kinetochores and was enriched in nuclei in early embryos at all stages ( Fig. 4A ). At the 8-cell stage, filaments formed rapidly (within ~40 s) in nuclei about 3 min prior to NEBD, at about the same time when the GFP::ROD-1 signal first appeared on kinetochores ( Fig. 4B ; Movie S2). GFP::ROD-1 filaments remained distinct from mitotic chromosomes and segregated to daughter cells by clustering at spindle poles ( Fig. 4B; Fig. S4A ; Movie S2). At the end of mitosis, the filaments largely disassembled before forming again in nuclei prior to NEBD of the subsequent division ( Fig. S4B ; Movie S2). Interestingly, depletion of SPDL-1 Spindly had no effect on the formation of GFP::ROD-1 filaments but prevented clustering at spindle poles (Fig. S4B) , suggesting that the filaments become tethered to spindle poles via dynein.
We sought to investigate why GFP::ROD-1 filaments formed at this specific time in development. Quantification of the GFP::ROD-1 signal revealed that nuclear levels of GFP::ROD-1 gradually increased with every embryonic division, as well as during the course of each cell cycle, reaching a peak before NEBD (Fig. 4C -E) . Consequently, nuclear GFP::ROD-1 levels before NEBD in 8-cell embryos were significantly higher than nuclear levels in all previous divisions ( Fig. 4C -E ). This suggested a straightforward explanation for the invariant timing of filament formation, namely that GFP::ROD-1 must become sufficiently concentrated before oligomerization is triggered. To directly test this idea, we used a mild RNAi regime to slightly reduce total GFP::ROD-1 levels in the embryo (Fig. 4E , F). In these RNAi conditions, nuclear GFP::ROD-1 levels in 8-cell embryos were comparable to those in control embryos at the 2-cell stage (Fig. 4E) . Strikingly, GFP::ROD-1 no longer formed filaments in 8-cell embryos or at later developmental stages, despite the still prominent enrichment in nuclei and normal localization to mitotic kinetochores (Fig. 4E, F) . These results demonstrate that ROD-1 has a propensity to oligomerize into filaments and suggest that oligomerization can be triggered locally by concentrating ROD-1 above a critical threshold.
. CC-BY-NC-ND 4.0 International license is made available under a The copyright holder for this preprint (which was not peer-reviewed) is the author/funder. It . https://doi.org/10.1101/282707 doi: bioRxiv preprint
The ROD-1 b-propeller suppresses ubiquitous and complete oligomerization of ROD-1 into filaments In human cells, deletion of the ROD b-propeller prevents kinetochore expansion, which may at least in part be a consequence of Spindly's inability to bind RZZ. Because oligomerization of C. elegans ROD-1 into filaments was independent of SPDL-1 Spindly , we wanted to address the role of the ROD-1 b-propeller. We therefore generated animals expressing mCherry::ROD-1 without b-propeller (D1-372) from an RNAi-resistant transgene integrated in single copy at a defined chromosomal locus (Fig. 5A ). We found that mCherry::ROD-1(D1-372), just like full-length mCherry::ROD-1, localized to early embryonic nuclei and mitotic kinetochores when endogenous ROD-1 was present (Fig. 5B ). By contrast, when we depleted endogenous ROD-1 by RNAi, mCherry::ROD-1(D1-372), but not full-length mCherry::ROD-1, oligomerized into filaments measuring up to 15 µm in length that were ubiquitously present throughout the cytoplasm of the oocyte-producing gonad and early embryo, irrespective of developmental and cell cycle stage ( Fig. 5B, D; Fig. S5A ). Oligomerization of mCherry::ROD-1(D1-372) into filaments appeared to be essentially complete judging by the lack of diffuse cytoplasmic signal (Fig. 5B) . Consequently, after depletion of endogenous ROD-1, mCherry::ROD-1(D1-372) was no longer detectable at mitotic kinetochores, and, as predicted by RZZ's essential role at kinetochores, dividing embryos exhibited chromosome bridges in anaphase and were inviable (Fig. 5B, C) . We conclude that ROD-1's propensity to oligomerize into filaments is antagonized by its N-terminal b-propeller. The behavior of C. elegans ROD-1(D1-372) was unexpected given that human ROD(D1-375) prevented kinetochore expansion. While the molecular basis for this difference is currently unclear, the experiments in human cells and C. elegans identify the Rod b-propeller as a key regulator of RZZ self-assembly. The ROD-1(D1-372) mutant also illustrates that cells must have tight regulatory mechanisms in place to control Rod's tendency to oligomerize.
Immunostaining confirmed that endogenously tagged 3xFLAG::CZW-1 Zw10 co-localized with mCherry::ROD-1(D1-372) on filaments (Fig. 5E ), while ZWL-1 Zwilch and SPDL-1 Spindly were not detectable ( Fig. S5B and data not shown). This agrees with prior work showing that CZW-1 Zw10 binds to ROD-1's Sec39 domain, which is present in ROD-1(D1-372), and that the ROD-1 bpropeller binds to ZWL-1 Zwilch and SPDL-1 Spindly [15, 32] . Unlike the GFP::ROD-1 filaments ( Fig. 4B ; Fig. S4 ), mCherry::ROD-1(D1-372) filaments never clustered at mitotic spindle poles and showed no evidence of directed movement (Fig. 5F ), consistent with the lack of SPDL-1 Spindly on these filaments. In the -1 oocyte and meiosis I embryo, where small ROD-1-dependent filaments . CC-BY-NC-ND 4.0 International license is made available under a The copyright holder for this preprint (which was not peer-reviewed) is the author/funder. It . https://doi.org/10.1101/282707 doi: bioRxiv preprint are naturally present, the significantly larger filaments of mCherry::ROD-1(D1-372) were prominently decorated with KNL-1 and BUB-1 ( Fig. 5G; Fig. S5C ). KNL-3, NDC-80, and CENP-C also localized to the filaments, but to a lesser extent (data not shown). The association of kinetochore proteins with mCherry::ROD-1(D1-372) filaments was strictly limited to the -1 oocyte and meiosis I embryo (Fig. S5C ), implying that their recruitment is regulated by posttranslational modifications and/or as-yet-unknown meiosis-specific factors. These results demonstrate that ROD-1(D1-372) and CZW-1 Zw10 retain the ability to interact with other kinetochore proteins after assembly into higher-order oligomers.
A complex of ROD-1(D1-372) and CZW-1 Zw10 self-assembles efficiently into higher-order oligomers in vitro.
We next sought to exploit the unique behavior of the ROD-1(D1-372) mutant to dissect the molecular requirements for ROD-1 oligomerization. Depletion of CZW-1 Zw10 by RNAi completely suppressed filament formation of mCherry::ROD-1(D1-372) (Fig. 5H ), likely because CZW-1 Zw10 is essential for ROD-1 stability [15] . By contrast, mCherry::ROD-1(D1-372) filaments were not affected by depletion of ZWL-1 Zwilch or SPDL-1 Spindly , consistent with the observation that ZWL-1 Zwilch and SPDL-1 Spindly did not localize to filaments. Furthermore, mCherry::ROD-1(D1-372) filaments readily formed after depletion of KNL-1, BUB-1, the Mis12 complex subunit KNL-3, and NDC-80, as well as after depletion of the mitotic regulators ICP-1 INCENP , CDK-1, and PLK-1 (data not shown). This indicated that a complex of ROD-1(D1-372) and CZW-1 Zw10 may be both necessary and sufficient for efficient oligomerization into filaments. To directly test this idea, we expressed the full-length RZZ complex and the ROD-1(D1-372)-CZW-1 Zw10 complex in insect Sf21 cells using baculovirus (Fig. 6A ). For purification and visualization, ROD-1 was N-terminally tagged with 6xHis followed by GFP, and CZW-1 Zw10 contained an N-terminal StrepTagII. After a first affinity purification step with nickel resin, size exclusion chromatography (SEC) showed that GFP::RZZ fractionated as a complex with equal stoichiometry of its three subunits and without other obvious protein contaminants (Fig. 6A ). After SEC, we examined the peak fractions by fluorescence microscopy but found no evidence that GFP::ROD-1 was present in filaments ( Fig. 6B ). We then applied the same purification procedure to the GFP::ROD-1(D1-372)-CZW-1 Zw10 complex, which we obtained in similar amounts and purity as GFP::RZZ (Fig. 6A) . In contrast to GFP::RZZ, GFP::ROD-1(D1-372)-CZW-1 Zw10 fractionated in a broader peak in SEC. Subsequent examination of peak fractions using fluorescence and transmission electron microscopy revealed . CC-BY-NC-ND 4.0 International license is made available under a The copyright holder for this preprint (which was not peer-reviewed) is the author/funder. It . https://doi.org/10.1101/282707 doi: bioRxiv preprint that GFP::ROD-1(D1-372) was present in filaments that were typically several µm in length (up to ~15 µm) and had an invariant width of ~50 nm (Fig. 6B -G) . Filaments frequently associated laterally with each other to form loose bundles (Fig. 6C , F, G). Staining for the StrepTagII confirmed that CZW-1 Zw10 co-localized with GFP::ROD-1(D1-372) on filaments (Fig. 6D) . We also readily obtained filaments with a ROD-1(D1-372)-CZW-1 Zw10 complex, confirming that the GFP tag was dispensable for ROD-1 oligomerization (Fig. S6 ). Once formed, filaments remained static and were stable at 4 ºC for several weeks, but did not tolerate freezing or high salt (500 mM NaCl), suggesting oligomerization involves electrostatic interactions. If the SEC step was omitted and partially purified GFP::ROD-1(D1-372)-CZW-1 Zw10 was examined directly after nickel affinity chromatography, filament formation could occasionally be followed by live imaging, which revealed that filaments grow from both ends (Fig. 6E) . We conclude that a purified ROD-1(D1-372)-CZW-1 Zw10 complex oligomerizes efficiently into µm-scale filaments in vitro, and that these filaments resemble those observed with this ROD-1 mutant in vivo. Thus, ROD-1(D1-372)-CZW-1 Zw10 represents a minimal RZZ construct sufficient for self-assembly into higher-order oligomers.
. CC-BY-NC-ND 4.0 International license is made available under a The copyright holder for this preprint (which was not peer-reviewed) is the author/funder. It . https://doi.org/10.1101/282707 doi: bioRxiv preprint
The RZZ complex is well-established as an outer kinetochore component required for the recruitment of Mad1-Mad2 and dynein [11, [33] [34] [35] [36] [37] . Here, we present evidence that RZZ additionally functions as a structural precursor for the assembly of the dynamic outermost kinetochore domain, which expands in early prometaphase to accelerate spindle assembly [22] . Recent work has revealed that the RZZ subunit Rod is evolutionarily related to coat proteins that form higher order assemblies on membranes [16, 29] . We demonstrate in C. elegans that Rod proteins are also capable of selfassembly. Although additional components may be required in vivo, we show in vitro that a complex consisting of ROD-1 without its b-propeller and CZW-1 Zw10 is sufficient to form µm-scale filaments, which have an invariant diameter of ~50 nm. Based on the human RZZ structure [16] , the precursor of these filaments likely corresponds to a ROD-1(D1-372)-CZW-1 Zw10 dimer. Similarly, the precursor of the GFP::RZZ filaments we observe in vivo is likely a dimer of a trimer, containing two copies each of ROD-1, CZW-1 Zw10 , and ZWL-1 Zwilch . Deletion of the b-propeller greatly favors oligomerization of ROD-1 in vitro and in vivo. The ROD-1 b-propeller forms a tight complex with ZWL-1 Zwilch [15, 32] , raising the possibility that the presence of ZWL-1 Zwilch in RZZ has an inhibitory effect on self-assembly.
However, depletion of ZWL-1 Zwilch in animals expressing mCherry::ROD-1 does not result in the ubiquitous formation of filaments we observe with mCherry::ROD-1(D1-372) [15] .
This argues that the inhibitory effect of the ROD-1 b-propeller on RZZ self-assembly is direct. The extent to which CZW-1 Zw10 contributes to self-assembly is difficult to assess, because CZW-1 Zw10 is essential for the stability of ROD-1 [15] . Future work may reveal the spatial arrangement of precursors within ROD-1(D1-372)-CZW-1 Zw10 filaments and provide insight into the self-assembly mechanism.
Based on our analysis of C. elegans RZZ, we suggest that human ROD's central role in kinetochore expansion is directly related to its capacity for oligomerization. We envision that filamentous RZZ assemblies form the structural scaffold of the expanded kinetochore domain and function as dynamic recruitment platforms for corona proteins, including SAC components, the motors dynein and CENP-E, and CENP-F. In contrast to the KMN network, protein-protein interactions among components in the expanded outer domain are low-affinity and therefore challenging to dissect with biochemical approaches.
Our finding that the expanded domain can be fully detached from the underlying . CC-BY-NC-ND 4.0 International license is made available under a The copyright holder for this preprint (which was not peer-reviewed) is the author/funder. It . https://doi.org/10.1101/282707 doi: bioRxiv preprint kinetochore, either by acute CDK1 inhibition or by combining GFP::ROD over-expression with Knl1 depletion, suggests that RZZ-dependent expansion results in a distinct multiprotein assembly that is at least transiently stable without the KMN network. Consistent with recent studies [13, 17] , our findings imply that kinetochores contain two distinct pools of Mad1-Mad2: one pool recruited by the KMN network through a direct interaction with Bub1 [7] [8] [9] , and another pool localized to the expanded outer domain, most likely recruited through a direct interaction with RZZ. The presence of BUBR1 and BUB3 on detached crescents may indicate that the expanded domain is able to generate MCC independently of BUB1.
Given RZZ's propensity to form oligomeric assemblies, an important question is how this behavior is spatially restricted to kinetochores. Our observations in C. elegans embryos expressing endogenous GFP::RZZ show that local precursor concentration is a critical parameter governing oligomerization behavior. Specifically, analysis of GFP::RZZ filament formation suggests that the complex is refractory to oligomerization until a threshold density of precursors is achieved. This may in part explain why we failed to observe filaments with GFP::RZZ in vitro, as the concentrations we obtained in the preparations were relatively low. In the context of kinetochores, recruitment of RZZ by Knl-1-Bub1 could bring about the spatial proximity of RZZ precursors necessary to trigger oligomerization, which then proceeds autonomously. An important implication of the mechanism we propose is that self-recruitment contributes significantly to overall RZZ levels at unattached kinetochores. Thus, even low levels of Knl1-Bub1 may support kinetochore expansion, so long as a minimal number of RZZ precursors can be recruited to start filament assembly. This idea is consistent with the observation that kinetochores are able to expand in GFP::ROD-expressing cells depleted of KNL1. While Knl1-Bub1 appear to be dispensable for expansion per se, our results in GFP::ROD-expressing cells show that Knl1-Bub1 are required for the physical linkage between the expanded domain and the underlying kinetochore.
We also show that RZZ's direct binding partner Spindly makes an important contribution to kinetochore expansion. This function of Spindly is separable from its function as the kinetochore adaptor for dynein-dynactin [15] , because DCTN1 depletion shows that dynein-dynactin are dispensable for kinetochore expansion. Previous work indicates that Spindly is recruited to kinetochores through a direct interaction with the ROD b-propeller [15, 16] , and, consistently, deletion of the ROD b-propeller suppresses . CC-BY-NC-ND 4.0 International license is made available under a The copyright holder for this preprint (which was not peer-reviewed) is the author/funder. It . https://doi.org/10.1101/282707 doi: bioRxiv preprint kinetochore expansion [15] . However, the ZWILCH(E422A/D426A) mutant shows that Spindly recruitment by RZZ per se is not sufficient for kinetochore expansion. Work in C. elegans suggested that SPDL-1 Spindly , in addition to binding the ROD-1 b-propeller, also makes contact with ZWL-1 Zwilch [15] . The interaction between SPDL-1 Spindly and ZWL-1 Zwilch involves the conserved acidic residues we mutated in human ZWILCH, raising the possibility that an interaction between Spindly and ZWILCH may play role in RZZ selfassembly. Mitotic kinases have been implicated in the regulation of kinetochore expansion in Xenopus egg extracts [21] . It is therefore likely that kinetochore-localized kinase activity acts on RZZ and/or Spindly to promote self-assembly of RZZ. Mps1 is a particularly attractive candidate given that a recent study identified multiple Mps1-dependent phospho-sites on both Spindly and the ROD b-propeller [38] .
In summary, we propose that recruitment of RZZ to kinetochores by Knl1-Bub1 locally concentrates Rod to favor self-assembly. Aided by Spindly and possibly kinase activity, this generates RZZ-based filaments that engage weakly-associating corona proteins to form the supramolecular assemblies visible as crescents and fibrous coronas by light and electron microscopy, respectively. Disassembly of the expanded domain after end-on microtubule attachment is likely achieved by dynein-dependent poleward transport of corona proteins, a process that is well documented [11, [39] [40] [41] [42] . Indeed, failure to completely disassemble the corona may contribute to the partial retention of MAD1 at microtubule-attached kinetochores that is observed in human Spindly mutants deficient in dynein recruitment [43, 44] . Thus, RZZ not only helps build the expanded kinetochore but also recruits the motor that brings about its eventual compaction.
. CC-BY-NC-ND 4.0 International license is made available under a The copyright holder for this preprint (which was not peer-reviewed) is the author/funder. It . https://doi.org/10.1101/282707 doi: bioRxiv preprint
cDNA coding for residues 1-591 of wild-type ZWILCH and the ZWILCH mutant E422A/D426A were cloned into a pcDNA5/FRT/TO-based vector (Invitrogen) modified to contain N-terminal Myc-GFP-TEV-S-peptide. Point mutations were subsequently introduced to make the transgene resistant to siRNA. Stable isogenic HeLa Flp-In T-REx cell lines expressing ZWILCH were generated by FRT/Flp-mediated recombination, as described previously [15] .
Worm strains (Table S1 ) were maintained at 20 ºC on standard NGM plates seeded with OP50 bacteria. To generate a strain stably expressing mCherry::ROD-1(373-2177) from chromosome II (locus ttTi5605), we used the same Mos1 transposon-based approach described previously for the strain expressing mCherry::ROD-1(1-2177) [15] .
Integration of the transgene was verified by PCR and sequencing. Endogenous GFP tagging of the rod-1 locus was done using CRISPR/Cas9-mediated genome editing, as described [45] . The repair template for gfp::rod-1 included gfp(S65C) with introns, inserted upstream of the rod-1 start codon via a GGRAGS linker, and the homology arms (1046 bp left; 1036 bp right). The PAM site for the guide RNA (5'-CCACAGCTTTTGCTTCGCCT-3') was mutated from AGG to AGA in the repair template. To modify the rod-1 locus using Cas9-triggered homologous recombination, the repair template (50 ng/µL) was co-injected with two separate plasmids, one expressing guide RNA under the U6 promoter (50 ng/µL) and the other expressing Cas9 under the eft-3 promoter (30 ng/µL) into N2 worms [46] .
The injection mix also contained three plasmids encoding fluorescent markers [pCFJ90 (Pmyo-2::mCherry, 2.5 ng/µL), pCFJ104 (Pmyo-3::mCherry, 5 ng/µL) and pGH8 (Prab-3::mCherry, 10 ng/µL) that allowed selection for F1 transgenic animals. Progeny of F1 transgenic animals were screened for integration of gfp by PCR using a primer within gfp and a primer outside the left homology arm. Strains were outcrossed 6x with the wild-type N2 strain and other fluorescent markers were subsequently introduced by mating. The copyright holder for this preprint (which was not peer-reviewed) is the author/funder. It . https://doi.org/10.1101/282707 doi: bioRxiv preprint
HeLa cells were maintained at 37 °C in a 5 % CO 2 atmosphere in Dulbecco's modified Eagle's medium (Gibco) supplemented with 10 % fetal bovine serum (Gibco), 100 units/mL penicillin, 100 units/mL streptomycin, and 2 mM GlutaMAX (Gibco). For immunofluorescence, cells were seeded on 12-mm poly-L-lysine-coated coverslips in 12well plates 24 h prior to transfection with siRNAs. Cells were transfected with siRNAs (Dharmacon On-Target plus; Table S2 ) targeting ROD/KNTC1, DCTN1, KNL1/CASC5, ZWILCH, and SPDL1/CCDC99, as described previously [43] . An siRNA against luciferase was used as a control. Transgene expression (Fig. 1K -M; Fig. 2C; Fig. 3B -D; Fig. S2) was induced with 0.2 µg/mL tetracycline (Sigma-Aldrich) 24 h post-transfection and cells were fixed 20 -24 h later. To depolymerize microtubules prior to immunofluorescence, cells were treated with 1 µM nocodazole (Sigma-Aldrich) for 4 h. To detach kinetochore crescents from centromeres ( Fig. 2; Fig. S1 ), cells were incubated for 4 h with 1 µM nocodazole followed by a 30-min incubation with nocodazole and 10 µM CDK1 inhibitor RO-3306 (Sigma-Aldrich).
For production of double stranded RNA (dsRNA ; Table S3) The copyright holder for this preprint (which was not peer-reviewed) is the author/funder. It . https://doi.org/10.1101/282707 doi: bioRxiv preprint Immunofluorescence in C. elegans embryos was carried out as described [47] , using the following primary antibodies: goat anti-GFP, 1:15000; rabbit anti-mCherry OD78, 1 µg/mL; mouse anti-a-Tubulin DM1a, 1:1000 (Sigma-Aldrich); mouse anti-FLAG M2, 1:1000 (Sigma-Aldrich); rabbit anti-KNL-1 OD33, 1 µg/mL; rabbit anti-BUB-1 OD31, 1 µg/mL; rabbit anti-ZWL-1 OD85, 1 µg/mL; rabbit anti-SPDL-1 OD164, 1:7000. Antibodies OD31, OD33, OD78, OD85, and OD164 were a gift from Arshad Desai.
Images were recorded on a Zeiss Axio Observer microscope controlled by ZEN The copyright holder for this preprint (which was not peer-reviewed) is the author/funder. It . https://doi.org/10.1101/282707 doi: bioRxiv preprint
Protein fractions were allowed to settle on 300 mesh Formvar-carbon-coated nickel grids and were negatively stained with a 2 % aqueous solution of uranyl acetate.
Grids were examined with a JEM1400 transmission electron microscope (JEOL) operating at 120 kV. Images were acquired using a post-column high-resolution (11 megapixels) high-speed camera (SC1000 Orius, Gatan).
Image analysis was performed using Fiji software (Image J version 2.0.0-rc-56/1.51h).
Volume and intensity of kinetochore fluorescence for CENP-E, CENP-F, Spindly, and GFP were measured using the 3D Objects Counter tool. To each image stack (0.2µm z-steps) encompassing one cell, the Subtract Background function was applied (rolling ball radius of 5 pixels), and a mask was generated based on an empirically determined threshold that maximized the number of detected kinetochores, while minimizing the detection of false objects. The mask was re-directed to the original, unprocessed image stack to determine the number of voxels and the integrated intensity for each object, which could consist of one or several closely apposed kinetochores. Object values were then summed to give the total number of voxels and the total integrated intensity for kinetochores in the image stack. To determine background intensity, the number of voxels and integrated intensity were measured for five separate regions in the image stack that did not contain kinetochores. Values for the five regions were averaged, normalized to the total number of kinetochore voxels, and subtracted from the total integrated kinetochore intensity. The total number of kinetochores in the image stack was determined separately using the CENP-C signal after maximum intensity projection of the image stack, followed by thresholding, automatic particle counting, and verification by visual inspection. Finally, the total number of kinetochore voxels and the total integrated kinetochore intensity were divided by the total number of kinetochores to yield the average number of voxels and integrated intensity per kinetochore per cell. Average kinetochore volumes and integrated intensities were determined for 20 cells in two independent experiments. The copyright holder for this preprint (which was not peer-reviewed) is the author/funder. It . https://doi.org/10.1101/282707 doi: bioRxiv preprint
Measurements of nuclear and cytoplasmic GFP::ROD-1 signal (Fig. 4D, E) were performed after maximum intensity projection of 12 x 1 µm z-stacks, captured every 20 s in embryos developing from the 2-cell to the 8-cell stage. The mean intensity in the nucleus or in the cytoplasm of the same cell was determined, and the mean intensity of a region outside the embryos (camera background) was subtracted.
Values in figures are reported as mean ± 95 % confidence interval. Statistical analysis was performed with GraphPad Prism 7.0 software. The type of statistical analysis (two-tailed t-test or one-way ANOVA/Bonferroni's multiple comparison test) is indicated in the figure legends. Differences were considered significant at P values below 0.05.
The cDNAs coding for ZWL-1, CZW-1, and ROD-1 (residues 1-2177 and 373-2177) were cloned into the pACEbac1 expression vector. ROD-1 constructs were tagged N-terminally with 6xHis followed by GFP. CZW-1 contained an N-terminal StrepTagII.
Bacmid recombination and virus production were carried out as described previously [48] . The copyright holder for this preprint (which was not peer-reviewed) is the author/funder. It . https://doi.org/10.1101/282707 doi: bioRxiv preprint The copyright holder for this preprint (which was not peer-reviewed) is the author/funder. It . https://doi.org/10.1101/282707 doi: bioRxiv preprint mitosis at the 8-cell stage, but not earlier (see also Movie S1). Dashed lines mark cell boundaries.
Scale bar, 5 µm. Blow-up, 2 µm. The copyright holder for this preprint (which was not peer-reviewed) is the author/funder. It . https://doi.org/10.1101/282707 doi: bioRxiv preprint (F) Selected images from 8-cell embryos whose AB lineage cells are going through mitosis. Mild depletion of GFP::ROD-1 slightly lowers enrichment in nuclei, which suppresses filament formation but does not prevent GFP::ROD-1 localization to kinetochores. Scale bar, 5 µm. The copyright holder for this preprint (which was not peer-reviewed) is the author/funder. It . https://doi.org/10.1101/282707 doi: bioRxiv preprint The copyright holder for this preprint (which was not peer-reviewed) is the author/funder. It . https://doi.org/10.1101/282707 doi: bioRxiv preprint
|
Annnotations
- Denotations: 0
- Blocks: 0
- Relations: 0