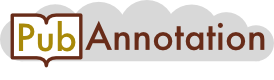
CORD-19:cf085fe7f16317b15dddc417b76252025fc80f61 8 Projects
ARTICLE Neutralization and clearance of GM-CSF by autoantibodies in pulmonary alveolar proteinosis
Abstract
A utoantibodies against cytokines have been frequently reported both in healthy individuals and in patients with autoimmune or infectious diseases 1 . In several instances, a pathogenic role for cytokine autoantibodies has not been formally demonstrated, as it is the case for autoantibodies to interleukin (IL)-17 in patients with mucocutaneous candidiasis or autoantibodies to interferon (IFN)-g in patients with mycobacterial infections [1] [2] [3] [4] . In other instances, autoantibodies have been shown to cause severe pathology by neutralizing the biological activity of the target cytokine, as it is the case for autoantibodies to the granulocyte-macrophage colony-stimulating factor (GM-CSF) in autoimmune pulmonary alveolar proteinosis (PAP) and autoantibodies to erythropoietin in pure red-cell aplasia 5, 6 . While in some cases autoantibody production has been linked to the administration of recombinant cytokines, such as erythropoietin, GM-CSF or , in most cases the stimuli that elicit the production of cytokine autoantibodies remain unknown.
The reason why cytokine autoantibodies may or may not cause pathology is not entirely clear. The prevailing view is that, when of enough affinity and present above a certain threshold of concentration, an autoantibody can neutralize the biological activity of the cytokine by simply binding and preventing its interaction with the cognate cellular receptor, a mechanism that can be recapitulated in vitro using cell proliferation bioassays with cytokine-dependent cell lines. Interestingly, however, several studies with toxins [9] [10] [11] and cytokines 12 demonstrated a synergy between different antibodies binding to the same molecule, suggesting that in some cases neutralization may be dependent on the production of antibodies targeting multiple antigenic sites, thus leading to the formation of immune complexes with the cytokine that can be efficiently cleared in vivo. In addition, it has been reported that monoclonal antibodies to IL-2, that neutralize the cytokine activity in vitro, can paradoxically enhance and extend IL-2 activity when injected in vivo 13 .
Autoimmune PAP is a rare and severe disease caused by the production of autoantibodies that neutralize the biological activity of GM-CSF. GM-CSF is essential for the terminal differentiation and function of alveolar macrophages that are responsible for the catabolism of surfactant lipids and proteins in the lungs 14 . The dysfunction of alveolar macrophages as a consequence of GM-CSF neutralization leads to the accumulation of lipoproteinaceous material within the alveoli, causing respiratory insufficiency and increased risk of infections 5 . In addition, GM-CSF deficiency results in impaired antimicrobial activity of neutrophils 15 . The pathogenic role of GM-CSF autoantibodies has been demonstrated by adoptive transfer of GM-CSF autoantibodies purified from PAP patients into non-human primates 16 . The sera of PAP patients contain levels of autoantibodies that exceed the pathogenic threshold of 10 mg ml À 1 , and that bind and neutralize GM-CSF at high titres 17 . A recent study reported the isolation of several monoclonal autoantibodies from PAP patients that showed different binding affinity and suggested that single human monoclonal antibodies neutralize the cytokine activity by blocking binding to the GM-CSF receptor 18 .
In this study we isolate a panel of monoclonal autoantibodies from PAP patients and show that single antibodies can only partially neutralize GM-CSF activity in vitro, depending on the experimental conditions of the bioassay, whereas three non-crosscompeting antibodies completely neutralize GM-CSF activity. In addition, we show that, in vivo, single antibodies enhance the levels of bioavailable GM-CSF, while three non-cross-competing antibodies induce a rapid Fc-dependent clearance of the cytokine. Finally, we show that in most cases somatic mutations are critical for binding to GM-CSF, suggesting that clones that give rise to autoantibodies derive from memory rather than naive B cells.
Characterization of GM-CSF autoantibodies from PAP patients. Peripheral blood mononuclear cells (PBMCs) and sera were collected from five chronic PAP patients of age ranging from 32 to 69 years. All patients had high serum titres of GM-CSF autoantibodies, ranging from 46 to 805 mg ml À 1 (Supplementary Table 1 ). Memory B cells were isolated and immortalized with Epstein-Barr virus and CpG, as described 19 . The supernatants were screened for the presence of GM-CSF-specific IgG using enzyme-linked immunosorbent assay (ELISA) and 21 clones that produced GM-CSF autoantibodies (monoclonal antibodies, mAbs) were identified. The antibody V genes were sequenced and analysed using the IMGT (international ImMunoGeneTics information system) database 20 . Most antibodies were IgG1 (20/21) and used the k light chain (19/21) , with no clear preference for V gene usage. The load of somatic mutations was comparable to that characteristic of T-cell-dependent responses against non-self-antigens, ranging from 3.8 to 17.4% in the VH gene segment and from 0 to 14.5% in the VL gene segment. All antibodies were produced recombinantly as IgG1 and were tested for binding to GM-CSF ( Table 1 ). The EC50 values determined using ELISA ranged from 16 to 836 ng ml À 1 and the K D values, as determined by surface plasmon resonance (SPR), ranged from 0.2 to 5.1 nM, consistent with high-affinity binding. Of notice, the kinetics values were heterogeneous. For instance, antibodies GCA7 and GCE402, which had comparable EC50 and K D values, showed different kinetics, being GCA7 characterized by a low on-/low off-rate and GCE402 by a high on-/high off-rate. The properties of the monoclonal antibodies isolated are consistent with a polyclonal response of somatically mutated B cells.
Binding to GM-CSF is dependent on somatic mutations. The role of somatic mutations in the development of GM-CSF autoantibodies was investigated by testing versions of the autoantibodies in which the somatic mutations of the whole V(D)J gene were removed. We refer to these antibody variants as the unmutated common ancestors (UCA). We also produced shuffled variants in which only the VH or the VL were reverted to the UCA sequence. When analysed using SPR and ELISA, five out of the seven UCA antibodies tested did not bind to GM-CSF, while the remaining two showed a reduced, but still detectable, binding (Fig. 1 and Supplementary Table 2 ). Shuffling experiments showed that somatic mutations in the heavy chain played a major role in high-affinity binding. Since a precise identification of somatic mutations in the HCDR3 is not possible due to the difficulty of unequivocally assign the D segment and determine the junctional rearrangement, we also tested antibody variants in which only the V gene segment was reverted to the germline sequence, while the HCDR3 was left in the wild-type form. Interestingly, in four out of seven cases removal of mutations in the V segment was sufficient to abolish binding (Supplementary Table 2 ), providing formal evidence for the role of somatic mutations in determining autoantibody specificity.
GM-CSF autoantibodies form high-molecular-weight complexes. Using SPR cross-competition experiments we identified multiple antigenic sites on GM-CSF and a complete antigenic map based on a matrix of cross-competition experiments was generated (Fig. 2a) . Sites I, II, III and IV are defined by four non-cross-competing autoantibodies GCA21, GCA7, GCB59 and GCC9. Interestingly, using SPR we could show that three non-cross-competing autoantibodies can bind simultaneously to a single molecule of GM-CSF (Fig. 2b) . Furthermore, when GM-CSF was incubated with an excess of three antibodies, formation of high-molecularweight immune complexes could be detected using size-exclusion chromatography-high-performance liquid chromatography (SEC-HPLC; Fig. 2c ).
Complete in vitro neutralization of GM-CSF by three antibodies. The neutralizing activity of the autoantibodies was assessed by measuring their ability to inhibit the proliferation of TF-1 cells in response to recombinant GM-CSF. Polyclonal IgG and autoantibodies purified from the sera of PAP patients showed potent and complete neutralizing activity, with IC90 values ranging from 0.53 to 36 mg ml À 1 and from 0.018 to 0.181 mg ml À 1 , respectively (Fig. 3a ). From these values it was estimated that GM-CSF autoantibodies account for 0.1 up to 5.6% of total IgG in the serum of PAP patients (that is, 7.6 to 1,300 mg ml À 1 ). These findings are consistent with previous reports 17, 21 and indicate that PAP patients have very high levels of GM-CSF autoantibodies capable of neutralizing the biologic activity of the cytokine.
Surprisingly, in the same bioassay, most monoclonal autoantibodies failed to neutralize GM-CSF (Fig. 3b) . The only exception was GCE536 that neutralized GM-CSF activity with an IC90 value of 2.43 mg ml À 1 , while the therapeutic antibodies Namilumab and MOR103 (refs 22,23) showed IC90 values of 0.80 and 0.16, respectively. Interestingly, when combined together, two non-cross-competing antibodies showed enhanced neutralizing activity both in terms of dose-response and percent inhibition, the combination of GCA21 (site I) and GCB59 (site IV) being the most effective (Fig. 3b) . Furthermore, a combination of three non-cross-competing antibodies (GCA21, GCA7 and GCB59, specific for sites I, II and IV) led to a complete inhibition of proliferation with an IC90 value of 0.08 mg ml À 1 (expressed as the total concentration of the three mAbs), which was lower than that of the therapeutic antibodies MOR103 and Namilumab. A strong synergy between three non-crosscompeting antibodies was observed for most combinations tested, with IC90 values comparable to those of affinity-purified antibodies from PAP sera ( Supplementary Fig. 1 ).
Considering the law of mass action, we hypothesized that in the presence of a single antibody a small fraction of GM-CSF may continuously dissociate from the antibody and become available to trigger the high-affinity GM-CSF receptor. In contrast, in the presence of three antibodies, GM-CSF may be sequestered irreversibly in stable immune complexes. As expected from the law of mass action, we found that by varying the cell number and the GM-CSF concentration the sensitivity of the assay was dramatically affected. In particular, lowering the number of TF-1 cells and the concentration of GM-CSF led to a more sensitive test that showed increased neutralization by single and multiple antibodies (Fig. 3c ). In contrast, when high number of TF-1 cells and high doses of GM-CSF were used, even the most potent neutralizing antibodies MOR103 and Namilumab, failed to neutralize GM-CSF, even when present in a 400-fold molar excess. Strikingly, in all conditions, a combination of three non-cross-competing antibodies was capable of completely neutralizing GM-CSF.
Having established that GM-CSF can form complexes with three antibodies resulting in efficient in vitro neutralization of the cytokine biological activity, we were interested in understanding the effect of single versus multiple autoantibodies in vivo. Mice were injected with a total of 100 mg of single or multiple monoclonal antibodies or with 2 mg of the IgG fraction isolated from the serum of a PAP patient, followed by injection of 2 mg of human GM-CSF. At different time points serum was collected and the amount of GM-CSF present was measured by a sandwich ELISA using an antibody specific for site II for capture and site I for detection. The assay was performed on serum either untreated or after alkaline treatment to dissociate the immune complexes ( Supplementary Fig. 2 ). In the absence of antibodies, the injected GM-CSF disappeared rapidly from the serum and was undetectable after 24 h (Fig. 4a) . In contrast, when single antibodies (GCA21 or MOR103) were used, high levels of GM-CSF were recovered from serum on day 1 and were still present on day 5. Of note, GM-CSF detection required alkaline dissociation in the case of MOR103 but not for GCA21, consistent with the different dissociation rates of the two antibodies (Table 1 ). In striking contrast, when mice received three non-cross-competing antibodies (GCA21, GCA7 and GCB59) or PAP IgG, GM-CSF was rapidly cleared since only low or undetectable amounts of the cytokine could be detected in the day-1 and day-5 sera, respectively, after alkaline dissociation.
To address the possible role of Fc receptors in the clearance of GM-CSF, we tested the same antibodies in a variant form, called LALA, which does not bind to C1q or to Fcg receptors. Similarly to the wild-type antibodies, single LALA antibodies led to an increase in GM-CSF levels in the serum. However, in contrast to what observed for three wild-type antibodies, three LALA antibodies failed to clear GM-CSF, which was quantitatively recovered in the sera following alkaline dissociation even on day 5 (Fig. 4a) .
To ask whether the antibody-bound GM-CSF would be bioavailable, we tested the sera of mice for their ability to support TF-1 proliferation (Fig. 4b) . Sera of mice receiving GCA21 or MOR103 led to a robust proliferation of TF-1 cells, consistent with a GM-CSF dissociation rate sufficient to engage the cytokine receptor. In contrast, sera of mice receiving three wild-type antibodies or PAP IgG were not able to stimulate proliferation, consistent with clearance of the immune complexes in vivo. In addition, although containing high level of GM-CSF, sera of mice receiving three LALA antibodies were not stimulatory, a finding consistent with irreversible sequestration of GM-CSF in stable immune complexes.
To further address the role of Fcg receptors, we tested immune complexes formed between GM-CSF and wild-type or LALA antibodies for their capacity to bind to TZM-bl cells expressing different Fcg receptors. Strong binding was observed only on FcgRIIa-and FcgRIIb-expressing cells and when immune complexes were formed by three wild-type, but not LALA, antibodies ( Fig. 4c and Supplementary Fig. 3 ). Taken together, the above results indicate that single antibodies, even when potently neutralizing in vitro, increase the half-life of GM-CSF and build up a circulating pool of bioavailable cytokine. In contrast, three or more antibodies lead to the formation of immune complexes that are efficiently cleared through an Fc-dependent mechanism.
Low levels of GM-CSF autoantibodies in healthy donors. To address whether low levels of autoantibodies to GM-CSF may be found in the serum of healthy donors, we took advantage of the synergy between autoantibodies in GM-CSF neutralization. When tested for their capacity to neutralize GM-CSF bioactivity on TF-1 cells, the IgG fraction isolated from serum of healthy donors did not show detectable neutralizing activity. However, when serum IgG was supplemented with a single non-neutralizing monoclonal antibody, a clear neutralizing activity was measured, which varied depending on the serum donor and on the site specificity of the antibodies used. In one case we could observe complete neutralization using the serum IgG from donor 6 (D6) combined with GCB9 and GCA21 antibodies (Fig. 5) . These results suggest that low levels of anti-GM-CSF antibodies devoid of neutralizing activity are present in the sera of healthy donors and can be detected using a sensitive complementation assay.
Our study identifies two mechanisms by which polyclonal anti-GM-CSF antibodies produced by PAP patients inhibit the biological activity of GM-CSF. The first is the irreversible sequestration of GM-CSF in high-molecular-weight immune complexes that can be readily detected in vitro using cell proliferation bioassays. The second is the in vivo Fc-dependent degradation of immune complexes formed between GM-CSF and multiple autoantibodies.
The autoantibodies isolated show affinities in the nanomolar range and distinct kinetics of binding, differing in particular in their off-rates. Overall, they were comparable to those reported in a recent study 18 . Interestingly, while this study claimed that all the antibodies were capable of neutralizing GM-CSF in the TF-1 bioassay, we show that neutralization by single antibodies is strictly dependent on the concentration of the cytokine and the number of receptors in the system, a finding that can be explained by the reversibility of antibody-antigen interaction, according to the law of mass action, and the affinity of GM-CSF receptor for its ligand, which is higher than that of antibodies 24, 25 . It was therefore interesting to discover that, when added together, three antibodies that bind to nonoverlapping sites can completely ARTICLE neutralize GM-CSF bioactivity, irrespective of the concentration of cytokine or receptor. When tested under stringent conditions, a cocktail of three antibodies was, on a weight basis, more potent than two anti-GM-CSF antibodies that are currently developed for therapy of autoimmune and inflammatory diseases 22, 23, 26 . We envisage that, when complexed with three antibodies, GM-CSF becomes completely sequestered and no longer available for interaction with the receptor.
A key observation in this study was that, when injected in mice, single antibodies led to the accumulation of a large pool of longlived GM-CSF that was still able to dissociate and trigger the receptor, as shown by the capacity of the sera to stimulate proliferation of TF-1 cells. The capacity of a single antibody to build up a large cytokine reservoir, as we observed for GM-CSF, could be the basis of the enhancing activity of monoclonal antibodies to common gamma-chain cytokines in vivo 13 . In striking contrast, we showed that three antibodies formed immune complexes that were rapidly degraded in vivo in an Fc-dependent manner. The degradation of GM-CSF induced by polyclonal antibodies is reminiscent of a previous study where three antibodies were shown to cause degradation of IL-6 in vivo 12 . Our result extend this concept to autoantibodies and suggest that degradation is the main mechanism by which polyclonal autoantibodies, such as those found in the serum of PAP patients, can lead to complete clearance of the cytokine, thus explaining the severe phenotype characteristic of these patients. In summary, our results suggest a two-step mechanism by which polyclonal antibodies lead to a severe GM-CSF deficiency: first, they irreversibly sequester GM-CSF thus completely preventing its interaction with the receptor and, second, by inducing its degradation in an Fc-dependent manner. Our findings also help explain why PAP has not been reported following administration of an anti-GM-CSF monoclonal antibody in humans 26 . We suggest that the main effect of the therapeutic anti-GM-CSF antibody would be to redistribute the cytokine into the circulation, thus decreasing its concentration in inflammatory sites, while leaving enough free GM-CSF for the development of alveolar macrophages. In addition, it is worth considering that systemically administered IgG accumulates preferentially in inflamed tissues as compared with the lung 27, 28 .
An interesting observation of this study is related to the role of somatic mutations and the presence of low levels of GM-CSF antibodies in healthy individuals. The finding that the UCA of the autoantibodies did not bind, or showed minimal binding to GM-CSF, is consistent with previous studies on the role of somatic mutations in determining the specificity of autoantibodies to desmogleins or DNA in patients with pemphigus or lupus [29] [30] [31] . In contrast, we observed that the UCA versions of antiviral antibodies showed only a slightly reduced binding to the specific antigen [32] [33] [34] . These findings support the notion that autoantibodies are preferentially generated from activated memory B cells that have been triggered and have undergone somatic mutation in response to foreign antigens. It is tempting to speculate that the preferential origin of autoantibodies from memory B cells is because of the fact that these cells have a lower activation threshold or may be more difficult to anergize as compared with naive B cells. In addition, it has been suggested that memory B cells may be able to efficiently prime naive T cells 35 . GM-CSF autoantibodies have been described not only in PAP patients but also in the serum of healthy donors or in patients with inflammatory bowel disease with normal pulmonary function 21, 36 . Building our findings, we developed a sensitive complementation assay that detects in the serum of healthy donors non-neutralizing GM-CSF autoantibodies based on their capacity to synergize with non-neutralizing monoclonal autoantibodies. These findings suggest that healthy donors may have anti-GM-CSF antibodies in serum at low concentrations and/or in combinations that cannot promote GM-CSF sequestration and degradation. Conversely, PAP patients developed high levels of GM-CSF autoantibodies to multiple sites that form immune complexes that mediate sequestration and degradation of the cytokine.
Isolation and production of monoclonal antibodies from PAP patients. Peripheral blood samples were obtained from five PAP patients with high levels of GM-CSF autoantibodies. Determination of the serum level of GM-CSF autoantibodies was performed in the Laboratory of the Rare Lung Disease Consortium at the Cincinnati Children's Hospital Medical Center, as described 17, 37 . Briefly, ELISA plates (MaxiSorp, Nunc) were coated with 1 mg ml À 1 of recombinant human GM-CSF (Miltenyi Biotec), blocked with blocking solution Stabilcoat (Surmodics, Eden Prairie, MN, USA) and incubated with titrated sera (1/101, 1/3,001, 1/6,001 and 1/12,001), followed by incubation with horseradish peroxidase-conjugated anti-human IgG F(ab 0 )2 fragment. Plates were then washed, substrate (tetramethylbenzidine; T4444, Sigma-Aldrich) was added followed by 1 N H 2 SO 4 and the plates were read at 450 nm. Serum concentration of GM-CSF autoantibodies was determined from a GM-CSF-autoantibody polyclonal reference standard by quadratic regression analysis using Microsoft Excel (Microsoft Corp, Seattle, WA, USA). The Disease Severity Score, a combination of presence and ARTICLE absence of symptoms and degree of PaO2, was determined according to the classification previously described 38 . Memory B cells were isolated from cryopreserved or fresh PBMCs using anti-fluorescein isothiocyanate (FITC) microbeads (Miltenyi Biotec) following staining of PBMCs with CD22-FITC (BD Phamingen), and were immortalized with Epstein-Barr virus and CpG in multiple wells as described previously 19 . Culture supernatants were tested for binding to recombinant human GM-CSF (Gentaur, 04-RHUGM-CSF) using ELISA. cDNA was synthesized from positive cultures and both heavy chain and light chain variable regions were sequenced. All monoclonal antibodies were produced recombinantly as IgG1 by transient transfection of HEK 293 Freestyle Cells (Invitrogen) using polyethylenimine, and tested for binding to GM-CSF using ELISA. The study was conducted in accordance with the Declaration of Helsinki guidelines and was approved by the Ethics Committee of IRCCS San Matteo Hospital Foundation of Pavia, Italy. All patients gave informed consent.
Sequence analysis of antibodies and reversion to germline. The usage of VH and VL genes and the amount of somatic mutations were determined by analysing the homology of VH and VL sequences of mAbs to known human V, D and J genes by the IMGT database 20 . Sequences of UCA were determined by reverting mutations to the germline sequence while retaining the original CDR3 junctions and terminal deoxy-nucleotidyl transferase N nucleotides. UCA sequences in which putative mutations of the HCDR3 were not removed were also determined. VH UCA, VH UCA (HCDR3 WT) and VL UCA nucleotide sequences were synthesized by Genscript, and their accuracies were confirmed by sequencing. Four different versions of seven selected mAbs were produced recombinantly: UCA, UCA-HCDR3 WT, and shuffled mAbs either with VH UCA þ wild-type VL or with wild-type VH þ VL UCA.
Antibody purification and ELISA assays. Human mAbs and total IgG from PAP sera were purified by protein A or protein G chromatography (GE Healthcare). Total IgG from healthy donors were purified using the HiTrap Protein A HP columns (GE Healthcare) and concentrated using Amicon Ultra filter units (100 K, Millipore). Total GM-CSF antibodies were affinity-purified from PAP sera using magnetic beads (Invitrogen) conjugated with human GM-CSF. Total IgGs were quantified using ELISA plates coated with anti-human IgG (SouthernBiotech) using Certified Reference Material 470 (ERMs-DA470, Sigma-Aldrich) as standard. Binding to GM-CSF was tested by ELISA using 384-well SpectraPlates (PerkinElmer) for primary screenings or 96-well MaxiSorp plates (Nunc) for any following test. Briefly, ELISA plates were coated with 1 mg ml À 1 of recombinant human GM-CSF (Gentaur), blocked with 1% BSA and incubated with titrated antibodies, followed by AP-conjugated anti-human IgG secondary antibodies (SouthernBiotech). Plates were then washed, substrate (p-NPP, Sigma) was added and plates were read at 405 nm. EC50 (ng ml À 1 ) was calculated for every sample by nonlinear regression analysis using the GraphPad Prism 5 software. Size-exclusion HPLC. Three non-cross-competing mAbs were diluted in PBS singularly or as a three-antibody-combination (10 mg of total antibody amount) and were mixed with GM-CSF (1:1 or 10:1 molar ratios) for 1 h at room temperature (RT). Samples were analysed with the Agilent 1100 HPLC machine using TSK-GEL G3000SW columns (Tosoh, bed volume: 13 ml, void volume: 4.6 ml) with PBS as the mobile phase (flow rate: 1 ml min À 1 ). A universal solvent 2-mm filter (Agilent) was put between injector and column. Detection was performed using a Variable Wavelength Detector (Agilent) with ultraviolet absorption at 220 nm.
TF-1 proliferation bioassays. TF-1 cells (Cell Lines Service) were maintained in RPMI 1640 medium supplemented with 10% fetal bovine serum (Hyclone), 1% GlutaMAX, 1% Penicillin/Streptavidin, 1% non-essential amino acids, 1% sodium pyruvate, 1% 2-mercaptoethanol (all from GIBCO), 5 ng ml À 1 human GM-CSF (Gentaur) and 10 ng ml À 1 human IL-3 (ImmunoTools). Cells were grown at 37°C in a humidified incubator with 5% CO 2 . A GM-CSF neutralization assay was performed by serially diluting mAbs (or a combination of mAbs, total IgG or affinity-purified antibodies) in growth medium with neither GM-CSF nor IL-3, adding GM-CSF at a concentration of 100 pg ml À 1 , and preincubating in 96-well flat-bottom cell culture plates (Costar) at 37°C for 1 h. TF-1 cells were washed five times, diluted in the growth medium with neither GM-CSF nor IL-3, and 10,000 cells per well were seeded (final GM-CSF concentration equal to 50 pg ml À 1 ). In other tests, GM-CSF was used at the final concentration of 500 and 5,000 pg ml À 1 , and 1,000 cells per well were seeded. Cells with or without GM-CSF in absence of antibodies were used as control to determine maximum and minimum levels of cell proliferation. Plates were incubated at 37°C in a humidified incubator with 5% CO 2 for 72 h, and cell proliferation was measured after 6-h incubation with 0.2 mCi per well of [3H]-thymidine (PerkinElmer). GM-CSF neutralization was calculated as percentage of inhibition of TF-1 growth with the following formula: [1 À (CCPM of a single well À average CCPM of control cells grown without GM-CSF) Â (average CCPM of control cells grown with GM-CSF À average CCPM of control cells grown without GM-CSF) À 1 ] Â 100 (CCPM ¼ corrected counts per minute). IC90 (mg ml À 1 ) was calculated for every sample by a nonlinear regression analysis using the GraphPad Prism 5 software. In some experiments mouse sera were titrated in TF-1 growth medium and preincubated at 37°C for 30 min. TF-1 cells were washed and seeded (1,000 cells per well). A titration of GM-CSF (60,000-0.3 ng ml À 1 ) was added as growth control. CCPM of each single well were plotted against the serum titration.
In vivo clearance of GM-CSF immune complexes. BALB/C mice were supplied by The Jackson Laboratory, USA; Harlan, Italy; Charles River, Germany, Italy. Animal experiments were approved by the Cantonal Autorithies of Cantone Ticino. Groups of 6-to 8-week-old female BALB/c mice were injected intravenously with 100 mg of purified mAbs or 2 mg of total IgG purified from PA96 patient. After 16 h, 2 mg of human GM-CSF were injected. Serum samples were collected on day 1 and day 5. GM-CSF was quantified with a sandwich ELISA. Briefly, 10 mg ml À 1 of an antibody that bound to site II of GM-CSF was used to coat 96-well Maxisorp plates (Nunc), which were then blocked with PBS þ 10% FBS (Gibco). All sera and GM-CSF, which was used as standard (range 3.4-600,000 pg ml À 1 ), were titrated and tested in parallel under different conditions: in one plate all samples were supplemented with 25% (vol/vol) of an alkaline dissociation buffer (2.5% Triton X-100, 2 M ethanolamine, 0.15 M NaCl, pH 11.6), in the other plate all samples were supplemented with 25% (vol/vol) of PBS þ 10% FBS. Plates were left overnight at RT. Detection of captured GM-CSF was made with 1 mg ml À 1 of a biotinylated antibody that bound to site I of GM-CSF for 1 h at RT, followed by binding of 0.5 mg ml À 1 streptavidin-AP (Jackson ImmunoResearch) for 1 h at RT. Plates were then washed, substrate (p-NPP, Sigma) was added and plates were read at 405 nm.
Binding of GM-CSF immune complexes to FccR. Four TZM-bl cell lines (NIH AIDS Research & Reference Reagent Program) each transfected with a specific Fcg receptor (FcgRI, FcgRIIa, FcgRIIb or FcgRIIIa) were maintained in DMEM medium supplemented with 10% fetal bovine serum (Hyclone), 0.025 M Hepes, 10 mg ml À 1 Gentamicin and 20 mg ml À 1 Blasticidin. Untransfected TZM-bl cells were used as negative control and were maintained in DMEM medium supplemented with 10% fetal bovine serum (Hyclone) and 2% Penicillin/Streptavidin. Cells were grown at 37°C in a humidified incubator with 5% CO 2 . Expression of specific FcgRs was assessed by staining TZM-bl cells with FITC-conjugated anti-CD64 (anti-FcgRI), anti-CD32 (anti-FcgRIIa and anti-FcgRIIb) and anti-CD16 (anti-FcgRIIIa) antibodies (all from BD Pharmingen). Untransfected and transfected TZM-bl cells were washed with staining buffer (PBS with 10% fetal bovine serum and 2 mM EDTA) and seeded in 96-well plates at a density of 50,000 cells per well. A single anti-GM-CSF mAb (GCA21) or a combination of three noncross-competing mAbs (GCA21, GCA7 and GCB59) at a final concentration of 2.5 mg ml À 1 were mixed with 0.05 mg ml À 1 GM-CSF, or staining buffer (PBS with 10% fetal bovine serum and 2 mM EDTA). The LALA versions of all antibodies and a mAb with a different specificity were included as controls. Samples were incubated at 37°C for 30 min to allow the formation of immune complexes and then cooled down to 4°C before adding them to TZM-bl cells for 30 min. Cells were washed twice and stained with anti-human IgG Fcg fragment specific F(ab 0 ) 2 fragment (Jackson ImmunoResearch). Samples were analysed on BD FACSCanto (BD Biosciences) and the median intensity fluorescence was analysed and compared between samples.
|
Annnotations
- Denotations: 0
- Blocks: 0
- Relations: 0