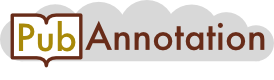
PMC:7366549 / 34-58 JSONTXT
Detection of coronaviruses in Pteropus & Rousettus species of bats from different States of India
Abstract
Background & objectives:
Bats are considered to be the natural reservoir for many viruses, of which some are potential human pathogens. In India, an association of Pteropus medius bats with the Nipah virus was reported in the past. It is suspected that the recently emerged severe acute respiratory syndrome coronavirus 2 (SARS-CoV-2) also has its association with bats. To assess the presence of CoVs in bats, we performed identification and characterization of bat CoV (BtCoV) in P. medius and Rousettus species from representative States in India, collected during 2018 and 2019.
Methods:
Representative rectal swab (RS) and throat swab specimens of Pteropus and Rousettus spp. bats were screened for CoVs using a pan-CoV reverse transcription-polymerase chain reaction (RT-PCR) targeting the RNA-dependent RNA polymerase (RdRp) gene. A single-step RT-PCR was performed on the RNA extracted from the bat specimens. Next-generation sequencing (NGS) was performed on a few representative bat specimens that were tested positive. Phylogenetic analysis was carried out on the partial sequences of RdRp gene sequences retrieved from both the bat species and complete viral genomes recovered from Rousettus spp.
Results:
Bat samples from the seven States were screened, and the RS specimens of eight Rousettus spp. and 21 Pteropus spp. were found positive for CoV RdRp gene. Among these, by Sanger sequencing, partial RdRp sequences could be retrieved from three Rousettus and eight Pteropus bat specimens. Phylogenetic analysis of the partial RdRp region demonstrated distinct subclustering of the BtCoV sequences retrieved from these Rousettus and Pteropus spp. bats. NGS led to the recovery of four sequences covering approximately 94.3 per cent of the whole genome of the BtCoVs from Rousettus bats. Three BtCoV sequences had 93.69 per cent identity to CoV BtRt-BetaCoV/GX2018. The fourth BtCoV sequence was 96.8 per cent identical to BtCoV HKU9-1.
Interpretation & conclusions:
This study was a step towards understanding the CoV circulation in Indian bats. Detection of potentially pathogenic CoVs in Indian bats stresses the need for enhanced screening for novel viruses in them. One Health approach with collaborative activities by the animal health and human health sectors in these surveillance activities shall be of use to public health. This would help in the development of diagnostic assays for novel viruses with outbreak potential and be useful in disease interventions. Proactive surveillance remains crucial for identifying the emerging novel viruses with epidemic potential and measures for risk mitigation.
A large number of emerging infectious diseases are known to be zoonotic in origin. In the last two decades, many viruses have been identified from bat species1. Bats have been recognized as the natural reservoirs of a variety of pathogenic viruses such as Rabies, Hendra, Marburg, Nipah and Ebola virus2. Bats are known to harbour coronaviruses (CoVs) and serve as their reservoirs. Alpha-CoV (α-CoV) and beta-CoV (β-CoV) have been detected in bats in Asia, Europe, Africa, North and South America and Australasia3. In the last two decades, bat CoVs (BtCoVs) garnered considerable attention as potential human pathogens45. Severe acute respiratory syndrome (SARS)-CoV-2 causing the current pandemic [CoV disease 2019 (COVID-19)] is also a member of the same genus and found to be similar to bat-derived CoV strain RATG136. SARS-CoV-2 is reported to be 96 per cent identical to BtCoV at the whole genome level, and related viruses were identified in the previously sampled bat population in China7.
CoVs are enveloped, single-stranded, positive-sense RNA viruses with a comparatively large genome size of 26 to 32 kb, classified under the family Coronaviridae in the order Nidovirales8. According to the International Committee on Taxonomy of Viruses (ICTV), they are classified into four genera, namely, α-CoV, β-CoV, γ-CoV and δ-CoV)9. β-CoVs are further classified into four different lineages [lineage A (L_A), lineage B (L_B), lineage C (L_C) and lineage D (L_D)]10. Most of the human CoVs are either zoonotic in origin or circulate in animals11. CoVs can cause a wide range of infections, including respiratory tract infections, gastroenteritis, hepatitis and encephalomyelitis in their respective hosts. It is believed that many of the currently circulating α-CoVs and β-CoVs of mammals have evolutionary links to CoVs from bats1.
India has a diverse population of bats; around 117 species of bats have been recorded, with around 100 subspecies coming under 39 genera belonging to eight families (Pteropodidae, Rhinolophidae, Hipposideridae, Megadermatidae, Rhinopomatidae, Emballonuridae, Molossidae and Vespertilionidae)12. The Indian Council of Medical Research-National Institute of Virology (ICMR-NIV) at Pune, India, has detected several viruses in bats, including the Nipah virus in Pteropus medius, Malsoor virus, Tioman virus and a novel adenovirus in Rousettus leschenaultii131415. Nipah viral RNA antibodies could be detected in Pteropus bats from many States of India, and the possible link of transmission from bats could be established during the Nipah outbreak which occurred in Kerala in 2018 and 20191617. The use of conventional polymerase chain reaction/reverse transcription-polymerase chain reaction (PCR/RT-PCR), as well as metagenomics and next-generation sequencing (NGS) technologies, has led to the discovery of many novel viruses in bats. The identification of new CoVs in bats in several neighbouring Asian countries such as China3, Sri Lanka18 and Singapore1920 and the growing threats of novel CoV diseases such as COVID-19 led us to investigate Pteropus and Rousettus bats commonly found in India, for identification and characterization of BtCoVs.
Material & Methods
This study was approved by the Institutional Animal Ethics Committee (IAEC) of ICMR-NIV, Pune (IAEC/2019/MEZ/04). Permissions were also obtained from the Principal Chief Conservators of Forests (PCCF)/wildlife wardens of different States/Union Territories (UT) (Kerala, Karnataka, Tamil Nadu, Himachal Pradesh, Punjab, Gujarat, Odisha, Telangana, Chandigarh and Puducherry).
Study sites and sample collection: Upon obtaining permission from the respective State authorities, bat-roosting sites in each State/UT were identified with the help of the forest officials. Bats were trapped using mist nets and were chemically restrained using isoflurane anaesthesia. Throat swabs (TS) and rectal swabs (RSs) were collected in virus transport medium (VTM) and were transported to ICMR-NIV, Pune, on dry ice. The specimens were collected from Pteropus spp. bats from Kerala, Karnataka, Chandigarh, Gujarat, Himachal Pradesh, Odisha, Puducherry, Punjab, Tamil Nadu and Telangana and Rousettus spp. bats from Kerala, Karnataka, Chandigarh, Gujarat, Odisha, Punjab and Telangana States during 2018-2019. These bats were monitored and released after recovery. Twelve bats that died during the trapping process were transported to ICMR-NIV on dry ice. Necropsy of these bats was carried out in the Biosafety Level 4 (BSL-4) containment facility, and tissue specimens (intestine and kidney) collected were tested.
Detection of bat coronavirus using RT-PCR: RNA was extracted from the bat specimens using the MagMAX pathogen RNA/DNA isolation kit (Invitrogen, USA). RT-PCR was performed using Superscript III one-step RT-PCR (Invitrogen, USA) with Platinum High-Fidelity Taq polymerase (Invitrogen, USA) using the published BtCoV-specific primers targeting the conserved region of RNA-dependent RNA polymerase (RdRp) gene21. The amplicon of 440 bp was separated on 1.5 per cent agarose gel and visualized under VersaDoc MP 4000 ultraviolet transilluminator (Bio-Rad, USA).
Sequencing of the positive coronavirus specimens
Sanger sequencing of bat coronavirus: The RT-PCR products were separated on 1.5 per cent agarose gel, and 440 bp bands were excised. The excised gels were extracted and purified using a QIAQuick gel extraction kit (Qiagen, Hilden, Germany). The purified products were quantified, and chain-terminated PCR reactions were performed using pathogen-specific forward and reverse primers21 with the BigDye Terminator 3.1 sequencing kit (Applied Biosystems, USA). BigDye reactions were purified using the DyeEx 2.0 spin kit (Qiagen, Germany). The purified chain-terminated reactions were sequenced using the ABI PRISM® 3100 Automated DNA Sequencer (Thermo Fisher Scientific, USA). The sequence data generated were assembled using the Sequencer 5.1 software (Accelrys Inc., USA).
Next-generation sequencing (NGS) of bat coronavirus: Selected bat specimens were used for RNA extraction2223. RNA libraries were prepared and quantified by Qubit® 2.0 Fluorometer (Invitrogen, USA). NEB NextrRNA depletion kit (New England Biolabs, USA) was used to remove host ribosomal RNA and re-quantified using Qubit® 2.0 Fluorometer (Invitrogen, USA). In brief, the RNA library preparation involved fragmentation, adenylation, adapter ligation and amplification. The amplified libraries were quantified using KAPA Library Quantification Kit (KapaBiosystems, Roche, Switzerland) as per the manufacturer's protocol and further loaded onto the Illumina Miniseq NGS platform (Illumina, USA)2223.
The FASTQ files generated after the completion of the run were analyzed using CLC Genomics Workbench software version 11 (CLC, Qiagen, Germany). De novo assembly programme was used to assemble contiguous sequences (contigs). The contigs generated were analyzed using BLAST (https://blast.ncbi.nlm.nih.gov/Blast.cgi) to identify matching sequences. The closest matching sequence from GenBank (https://www.ncbi.nlm.nih.gov/genbank/) was used for reference mapping.
Phylogenetic analysis of partial and complete genome sequences of bat coronavirus: The CoV sequences retrieved from RS specimens of Rousettus spp. bats (n=4) were aligned with whole-genome sequences from GenBank using the create alignment function of the CLC genomics workbench (https://digitalinsights.qiagen.com). Partial RdRp gene sequences (~419 bp) retrieved by Sanger sequencing, for both the bat species specimens (genomic location: 14,701-15,120) were used to construct a phylogenetic tree along with the available RdRp sequences in GenBank. Phylogenetic analysis was carried out using the neighbour-joining method available in MEGA v7 software24 using the Kimura 2-parameter nucleotide (nt) substitution model with 1000 bootstrap replicates. The nt divergence for the open reading frame (ORF) 1a polyprotein (ORF 1a), ORF 1b polyprotein (ORF 1b), spike protein (S), nucleocapsid phospoprotein (N), envelope protein (E) and membrane glycoprotein (M) genes was estimated using the Kimura 2-parameter model as implemented in the MEGA software. The sequences retrieved in the current study, along with those downloaded from GenBank, were grouped into the genus.
The viruses from the β-CoV genus were further grouped into lineages, L_A, L_B, L_C and L_D, to estimate the evolutionary divergence over the respective gene sequence pairs between groups using the MEGA software24. The distance was estimated using a Kimura 2-parameter model with uniform rates among the sites. The bootstrap of 500 replicates was used to estimate the variation in the model.
Results
The TS and RS specimens for 78 Rousettus spp. bats were collected in VTM from seven States (Kerala, Karnataka, Chandigarh, Gujarat, Odisha, Punjab and Telangana). The TS and RS specimens of 508 Pteropus spp. bats were also collected in VTM from 10 States/UTs in India (Kerala, Karnataka, Chandigarh, Gujarat, Himachal Pradesh, Odisha, Puducherry, Punjab, Tamil Nadu and Telangana). During the trapping process, 12 (8 Rousettus and 4 Pteropus spp.) bats died. Organ specimens (intestine and kidney) were collected from these bats (TS and RS specimens of these 12 bats were included in the total number of samples).
Detection of bat coronavirus using RdRp gene RT-PCR: Four of the 78 RS of Rousettus spp. bats screened for the BtCoV were found positive. All the positive RS samples belonged to Kerala State. Intestinal specimens of two bats were also found to be positive for the BtCoV. One bat (MCL-19-Bat-606), from Kerala, was tested positive in both the intestinal specimen and the RS. The second bat (MCL-20-Bat-76), from Karnataka, was tested positive only in the intestinal specimen. Altogether, five Rousettus spp. bats were positive for the BtCoV. All TS specimens from Rousettus spp. were found negative for BtCoV (Table I).
Table I Bat coronavirus positivity in bat specimens screened using RNA-dependent RNA polymerase (RdRp) gene-specific reverse transcription-polymerase chain reaction (RT-PCR) in different States
Place of collection Number of positive/number tested (%) for different bat species for BtCoV RdRp gene-specific RT-PCR
Pteropus bats (%) Rousettus bats (%)
Rectal swabs Throat swabs Rectal swabs Throat swabs
Kerala 12/217 (5.53) 0/21 (0.00) 4/42 (9.52) 0/4 (0.00)
Karnataka 0/78 (0.00) NT 0/4 (0.00) 0/4 (0.00)
Chandigarh 0/27 (0.00) NT 0/6 (0.00) 0/6 (0.00)
Gujarat 0/30 (0.00) NT 0/18 (0.00) 0/18 (0.00)
Odisha 0/30 (0.00) NT 0/2 (0.00) 0/2 (0.00)
Punjab 0/14 (0.00) NT 0/2 (0.00) 0/2 (0.00)
Telangana 0/30 (0.00) NT 0/4 (0.00) 0/4 (0.00)
Himachal Pradesh 2/29 (6.89) 0/6 (0.00) NA NA
Puducherry 6/23 (26.09) 0/10 (0.00) NA NA
Tamil Nadu 1/30 (3.33) 0/5 (0.00) NA NA
21/508 (4.13) 0/42 (0.00) 4/78 (5.13) 0/40 (0.00)
NT, not tested; NA, not available; BtCoV, bat coronavirus Twenty one of the 508 RSs from Pteropus spp. bats screened were tested positive for the BtCoV (Table I). These positive bats belonged to Kerala (n=12), Himachal Pradesh (n=2), Puducherry (n=6) and Tamil Nadu (n=1). The TS specimens of the same bats were tested negative for BtCoV. The TS specimens of RS-negative (n=42) bats were also screened and found to be negative (Table I). A total of 25 bats from both the species were found positive.
Sequencing of the positive coronavirus specimens
Sanger sequencing of bat coronavirus: Using the Sanger sequencing protocol, partial RdRp sequences of BtCoV were retrieved from two (out of 4 amplicons) specimens of Rousettus spp. One of the sequences (MCL-19-bat-588/2) showed close identity to BtCoV HKU9-5-2 (AN): HM211099.1; sequence identity (SI): 99.2 per cent, whereas the second RdRp sequence (MCL-20-bat-76/10) had an SI of 98.8 per cent with BtCoV HKU9-1 (AN: EF065513.1), both from China.
Sanger's sequencing protocol led to retrieval of eight partial RdRp sequences which belonged to Pteropus spp. These bats were collected from Kerala (n=5) and Tamil Nadu (n=3) States. One of the three partial RdRp sequences from Tamil Nadu had 97.93 per cent SI with BtCoV/B55951/Pte_lyl/CB2-THA (AN: MG256459.1, Thailand). The other two sequences had a minimum of 99.48 per cent SI with the CoV PREDICT_CoV-17/PB072 (AN: KX284942.1, Nepal). One of the five partial RdRp sequences from Kerala had 98.88 per cent SI with BtCoV/B55951/Pte_lyl/CB2-THA (AN: MG256459.1, Thailand). The remaining four partial RdRp sequences had >97 per cent SI with CoV PREDICT_CoV-17/PB072 (AN: KX284942.1, Nepal).
Next-generation sequencing of bat coronavirus: NGS was performed on 10 specimens [4 RS, 2 kidney and 4 intestinal tissue) of the five Rousettus bats to retrieve the complete genome of the BtCoV. Kidney and intestine tissues of the bats from Karnataka State (MCL-20-Bat-76) and RS along with intestine tissue of bats from Kerala State (MCL-19-Bat-606) were used for sequencing and analysis.
Two different viruses were retrieved based on the BLAST analysis of the sequences from the kidney and intestine tissues of the bats from Karnataka. Kidney specimen of MCL-20-Bat-76 had an SI of 94 per cent and query coverage (QC) of 94 per cent with CoV BtRt-BetaCoV/GX2018 (AN: MK211379.1), whereas the intestine tissue of the MCL-20-Bat-76 had an SI of 96.8 and 95 per cent QC with the BtCoV HKU9-1 (AN: EF065513.1). The sequences from RS and intestine tissue of the MCL-19-Bat-606 from Kerala, had 93.69 and 93.99 per cent SI to CoV BtRt-BetaCoV/GX2018 (AN: MK211379.1), respectively, with 100 per cent QC. Further, 99.8 per cent of the CoV BtRt-BetaCoV/GX2018 sequences were retrieved from the intestine specimen of the MCL-19-Bat-606. The details of the genome recovered reads mapped and the per cent of reads mapped are summarized in Table II.
Table II Details of the genome recovered reads mapped and the per cent of reads mapped from the Rousettus bat samples
Sample details Sample type Virus retrieved Relevant reads Per cent of reads Per cent of genome recovered
MCL-20-Bat-76 Kidney Coronavirus BtRt-BetaCoV/GX2018 1632 0.015 94.39
Intestine BtCoV HKU9-1 4499 0.056 95.75
MCL-19-Bat-606 Rectal swab Coronavirus BtRt-BetaCoV/GX2018 13,973 0.114 99.53
Intestine Coronavirus BtRt-BetaCoV/GX2018 10,214,492 93.476 99.87 Phylogenetic analysis of partial and complete genome sequences of bat coronavirus: A neighbour-joining tree was generated using the partial RdRp region sequences derived from Pteropus and Rousettus spp. bat specimens. It was observed that all the BtCoV sequences were clustered within the L_D sequences of beta CoVs. A distinct subclustering of the sequences retrieved from Pteropus and Rousettus spp. bats is shown in Figure 1. The sequences in the light pink colour are retrieved from the Pteropus spp., whereas those in the dark pink region belong to Rousettus spp. The sequence divergence of 0.35 was observed between Pteropus spp. and Rousettus spp., which was obtained by averaging over all the sequence pairs between the two species, determining those to be distinct sequences to each species.
Fig. 1 Neighbour-joining tree for the RNA-dependent RNA polymerase (RdRp) partial sequence (genomic location: 14,701-15,120) generated from Sanger sequencing. The tree was constructed using the RdRp sequence available in the GenBank sequences. Kimura 2-parameter model was used as the substitution model to generate the tree. A bootstrap replication of 1000 cycles was performed to generate the tree to assess the statistical robustness. The complete genome sequences of four BtCoV obtained from Rousettus spp. specimens were used for generating a neighbour-joining tree (Fig. 2). These sequences were also clustered within L_D of β-CoVs as observed for partial RdRp sequence tree. These complete genome sequences were grouped into gene pairs to identify the gene with higher and lower divergence. The complete genomes of the Indian BtCoV sequences were grouped under L_D. The evolutionary divergence of ORF 1b was <0.54 between the different β-CoV lineages with a maximum score of 0.7 between different BtCoV sequences used in this study (Table III). E gene sequences had larger divergence within the β-CoV genus ranging from 2.18 to 0.94. Lineages L_A and L_C had the maximum divergence of 2.18, whereas the L_B and L_C were the least (0.94). N gene has an overall higher divergence among different lineages (ranging: 2.08-0.75). Overall, evolutionary divergence for the sequences of each gene pair demonstrated that S, N, E and M genes from the α- and δ-CoV highly diverged across the different genus. In contrast, the ORF 1b was less divergent across the genera (Table III).
Fig. 2 Phylogenetic tree for the complete genome tree: A neighbour-joining tree was generated using the representative complete genome sequence available in the GenBank sequences. Kimura 2-parameter model was used as the substitution model to generate the tree. A bootstrap replication of 1000 cycles was performed to generate the tree to assess the statistical robustness.
Table III Evolutionary divergence for ORF 1b, S, N and M genes for the retrieved sequences with other reference sequences. The lower right-check hand matrix of the table depicts the divergence and the upper left-check matrix of the matrix (blue colour) depicts the variation observed in the bootstrap replication
N gene Alpha Delta Gamma L_A L_B L_C L_D M gene Alpha Delta Gamma L_A L_B L_C L_D
Alpha 0.15 0.09 0.10 0.08 0.08 0.09 Alpha 0.11 0.12 0.05 0.06 0.05 0.06
Delta 2.08 0.11 0.16 0.09 0.11 0.10 Delta 1.50 0.26 0.08 0.16 0.10 0.11
Gamma 1.57 1.49 0.08 0.08 0.09 0.08 Gamma 1.53 1.84 0.10 0.12 0.11 0.09
L_A 1.84 1.73 1.37 0.05 0.05 0.06 L_A 0.92 1.24 1.30 0.06 0.05 0.05
L_B 1.48 1.37 1.32 1.09 0.03 0.04 L_B 1.05 1.51 1.37 0.92 0.05 0.05
L_C 1.57 1.52 1.42 1.07 0.75 0.04 L_C 0.99 1.35 1.27 0.80 0.82 0.05
L_D 1.64 1.46 1.36 1.27 0.90 0.97 L_D 0.99 1.42 1.23 0.84 0.79 0.82
ORF 1b Alpha Delta Gamma L_A L_B L_C L_D ORF 1a Alpha Delta Gamma L_A L_B L_C L_D
Alpha 0.01 0.01 0.01 0.01 0.01 0.01 Alpha 0.02 0.02 0.01 0.02 0.02 0.03
Delta 0.70 0.01 0.02 0.01 0.01 0.01 Delta 1.32 0.03 0.02 0.03 0.03 0.04
Gamma 0.62 0.67 0.01 0.01 0.01 0.01 Gamma 1.14 1.33 0.02 0.03 0.02 0.04
L_A 0.61 0.69 0.60 0.01 0.01 0.01 L_A 1.22 1.01 1.30 0.02 0.02 0.04
L_B 0.60 0.70 0.65 0.54 0.01 0.01 L_B 1.26 1.42 1.41 1.19 0.01 0.02
L_C 0.58 0.69 0.62 0.53 0.50 0.01 L_C 1.35 1.41 1.44 1.19 0.97 0.03
L_D 0.60 0.67 0.61 0.53 0.50 0.52 L_D 1.26 1.27 1.39 1.09 0.90 1.03
S gene Alpha Delta Gamma L_A L_B L_C L_D E gene Alpha Delta Gamma L_A L_B L_C L_D
Alpha 0.02 0.02 0.03 0.03 0.03 0.02 Alpha 0.12 0.18 0.09 0.15 0.15 0.12
Delta 0.86 0.03 0.04 0.04 0.06 0.03 Delta 1.14 0.47 0.22 0.41 0.28 0.17
Gamma 1.14 0.96 0.04 0.05 0.06 0.04 Gamma 1.59 1.64 0.22 0.24 0.32 0.19
L_A 1.36 1.28 1.43 0.03 0.03 0.02 L_A 1.03 1.58 1.57 0.23 0.21 0.25
L_B 1.33 1.23 1.34 1.19 0.04 0.02 L_B 1.24 1.75 1.40 1.83 0.11 0.14
L_C 1.42 1.32 1.46 1.17 1.03 0.03 L_C 1.37 1.64 1.83 2.18 0.94 0.17
L_D 1.34 1.24 1.41 1.16 1.00 1.11 L_D 1.25 1.42 1.52 1.95 1.16 1.37
ORF 1a, open reading frame 1a polyprotein; ORF 1b, ORF 1b polyprotein; S, spike glycoprotein; N, nuclocapsid phospoptotein; M, membrane glycoprotein; E, envelope protein
Discussion
As per the available information, the BtCoV causing human infection belongs to α- and β-CoV genera of the Coronaviridae family. β-CoV genus has five strains known to infect humans25. The two human-infecting strains (NL63 and 229E) from α-CoV genus which cause mild-to-moderate respiratory infections are believed to have originated in bats25. Two members of the β-CoV genus (HCoV-OC43 and HCoV-HKU1) are known to cause the common cold and lower respiratory tract infections26. The other three are now shown to be pathogenic to humans (SARS-CoV-1, MERS-CoV and SARS-CoV-2). The SARS-CoV-1 and SARS-CoV-2 belong to L_B and MERS CoV belongs to L_C of β-CoV genus27.
The phylogenetic analysis for the partial RdRp region revealed the presence of distinct BtCoVs in both the bats. The genomic sequences retrieved from the Indian sequences form a distinct cluster. The three CoV_BtRtBetaCoV/GX2018 sequences retrieved from the Indian Rousettus bats were 5.8-6.7 per cent different from the reference sequence, which was retrieved from Rhinolophus affinis. The two CoV_BtRtBetaCoV/GX2018 sequences retrieved from different bats were 1.2 per cent different from each other. The effect of host influence on the nt usage of the virus cannot be denied; however, it needs to be explored further in detail.
Bats are reservoirs for viruses with human pathogenic potential2829, and are known to harbour a broad range of CoVs1. The global distribution of bats, along with the different types of cell receptors present within them, favours virus replication, and is a possible link to their intraspecies transmission. The interspecies spill-over of a BtCoV to humans is thought to occur through an intermediate host, in which the virus replicates through yet completely unidentified routes. In India, regions of the Western Ghats, particularly in Kerala, are reported to have habitat for diverse bat populations. The reports of pathogenic human viruses from bat specimens demand enhanced methods to monitor human exposure to various bat species. Investigations in unexplored regions/States should be focused on gaining further insights into CoV diversity within Indian bat populations.
Earlier, we had reported the presence of pathogenic viruses such as the Nipah virus in Pteropus bats in India16. In the present scenario of changing demography and ecological manipulations, it is challenging to have checks on the encounters of bats with other animals and humans. Therefore, active and continuous surveillance remains crucial for outbreak alerts for bat-associated viral agents with epidemic potential, which would be helpful in timely interventions.
Although CoVs in the subfamily Coronavirinae do not usually produce clinical symptoms in their natural hosts (bats), accidental transmission of these viruses to humans and other animals may result in respiratory, enteric, hepatic or neurologic diseases of variable severity. It is still not understood as to why only certain CoVs can infect people.
There is a need of proactive surveillance of zoonotic infections in bats. Detection and identifications of such aetiological agents will provide leads for the development of diagnostic along with preparedness and readiness to deal with such emergent viruses thereby quickly containing them. The detection and identification of such viruses from bats also recommends cross-sectional antibody surveys (human and domestic animals) in localities where the viruses have been detected. Similarly, if epidemiological situation demands, evidence-based surveillance should also be conducted. There is a need of developing strong mechanisms for working jointly with various stakeholders such as wildlife, poultry, animal husbandry and human health departments.
In conclusion, our study showed detection of pathogenic CoVs in two species of Indian bats. Continuous active surveillance is required to identify the emerging novel viruses with epidemic potential.
Acknowledgment
Authors acknowledge the encouragement and support extended by Prof. (Dr) Balram Bhargava, Secretary, Department of Health Research, Ministry of Health & Family Welfare, Director-General, Indian Council of Medical Research (ICMR), New Delhi, and Dr Raman Gangakhedkar, Division of Epidemiology & Communicable Diseases, ICMR, New Delhi. Authors acknowledge the Principal Conservators of Forests of Kerala, Karnataka, Tamil Nadu, Himachal Pradesh, Punjab, Gujarat, Odisha, Telangana, Chandigarh and Puducherry and other concerned officials for their permission to capture bats. Authors are also thankful to Servshri Rajen Lakra, Prasad Sarkale, Pranita Gawande, Kaumudi Kalele, Shrimati Ashwini Waghmare, Nikita Bankar and Shri Sanjay Thorat for providing technical support. Authors acknowledge the contribution of the field team for the hard work performed by Servshri Sanjay Gopale, Annasaheb Suryawanshi, Manjunath Holeppanavar, Ganesh Chopade, Madhav Acharya, D.K. Singh and Sachin Daigude for technical assistance during the study. Financial support & sponsorship: This work was supported by intramural funding by the Indian Council of Medical Research, New Delhi
Conflicts of Interest: None.
|
Document structure show
article-title | Detection of coronaviruses in Pteropus & Rousettus species of bats from different States of India |
abstract | Background & objectives: Bats are considered to be the natural reservoir for many viruses, of which some are potential human pathogens. In India, an association of Pteropus medius bats with the Nipah virus was reported in the past. It is suspected that the recently emerged severe acute respiratory syndrome coronavirus 2 (SARS-CoV-2) also has its association with bats. To assess the presence of CoVs in bats, we performed identification and characterization of bat CoV (BtCoV) in P. medius and Rousettus species from representative States in India, collected during 2018 and 2019. Methods: Representative rectal swab (RS) and throat swab specimens of Pteropus and Rousettus spp. bats were screened for CoVs using a pan-CoV reverse transcription-polymerase chain reaction (RT-PCR) targeting the RNA-dependent RNA polymerase (RdRp) gene. A single-step RT-PCR was performed on the RNA extracted from the bat specimens. Next-generation sequencing (NGS) was performed on a few representative bat specimens that were tested positive. Phylogenetic analysis was carried out on the partial sequences of RdRp gene sequences retrieved from both the bat species and complete viral genomes recovered from Rousettus spp. Results: Bat samples from the seven States were screened, and the RS specimens of eight Rousettus spp. and 21 Pteropus spp. were found positive for CoV RdRp gene. Among these, by Sanger sequencing, partial RdRp sequences could be retrieved from three Rousettus and eight Pteropus bat specimens. Phylogenetic analysis of the partial RdRp region demonstrated distinct subclustering of the BtCoV sequences retrieved from these Rousettus and Pteropus spp. bats. NGS led to the recovery of four sequences covering approximately 94.3 per cent of the whole genome of the BtCoVs from Rousettus bats. Three BtCoV sequences had 93.69 per cent identity to CoV BtRt-BetaCoV/GX2018. The fourth BtCoV sequence was 96.8 per cent identical to BtCoV HKU9-1. Interpretation & conclusions: This study was a step towards understanding the CoV circulation in Indian bats. Detection of potentially pathogenic CoVs in Indian bats stresses the need for enhanced screening for novel viruses in them. One Health approach with collaborative activities by the animal health and human health sectors in these surveillance activities shall be of use to public health. This would help in the development of diagnostic assays for novel viruses with outbreak potential and be useful in disease interventions. Proactive surveillance remains crucial for identifying the emerging novel viruses with epidemic potential and measures for risk mitigation. |
sec | Background & objectives: Bats are considered to be the natural reservoir for many viruses, of which some are potential human pathogens. In India, an association of Pteropus medius bats with the Nipah virus was reported in the past. It is suspected that the recently emerged severe acute respiratory syndrome coronavirus 2 (SARS-CoV-2) also has its association with bats. To assess the presence of CoVs in bats, we performed identification and characterization of bat CoV (BtCoV) in P. medius and Rousettus species from representative States in India, collected during 2018 and 2019. |
title | Background & objectives: |
p | Bats are considered to be the natural reservoir for many viruses, of which some are potential human pathogens. In India, an association of Pteropus medius bats with the Nipah virus was reported in the past. It is suspected that the recently emerged severe acute respiratory syndrome coronavirus 2 (SARS-CoV-2) also has its association with bats. To assess the presence of CoVs in bats, we performed identification and characterization of bat CoV (BtCoV) in P. medius and Rousettus species from representative States in India, collected during 2018 and 2019. |
sec | Methods: Representative rectal swab (RS) and throat swab specimens of Pteropus and Rousettus spp. bats were screened for CoVs using a pan-CoV reverse transcription-polymerase chain reaction (RT-PCR) targeting the RNA-dependent RNA polymerase (RdRp) gene. A single-step RT-PCR was performed on the RNA extracted from the bat specimens. Next-generation sequencing (NGS) was performed on a few representative bat specimens that were tested positive. Phylogenetic analysis was carried out on the partial sequences of RdRp gene sequences retrieved from both the bat species and complete viral genomes recovered from Rousettus spp. |
title | Methods: |
p | Representative rectal swab (RS) and throat swab specimens of Pteropus and Rousettus spp. bats were screened for CoVs using a pan-CoV reverse transcription-polymerase chain reaction (RT-PCR) targeting the RNA-dependent RNA polymerase (RdRp) gene. A single-step RT-PCR was performed on the RNA extracted from the bat specimens. Next-generation sequencing (NGS) was performed on a few representative bat specimens that were tested positive. Phylogenetic analysis was carried out on the partial sequences of RdRp gene sequences retrieved from both the bat species and complete viral genomes recovered from Rousettus spp. |
sec | Results: Bat samples from the seven States were screened, and the RS specimens of eight Rousettus spp. and 21 Pteropus spp. were found positive for CoV RdRp gene. Among these, by Sanger sequencing, partial RdRp sequences could be retrieved from three Rousettus and eight Pteropus bat specimens. Phylogenetic analysis of the partial RdRp region demonstrated distinct subclustering of the BtCoV sequences retrieved from these Rousettus and Pteropus spp. bats. NGS led to the recovery of four sequences covering approximately 94.3 per cent of the whole genome of the BtCoVs from Rousettus bats. Three BtCoV sequences had 93.69 per cent identity to CoV BtRt-BetaCoV/GX2018. The fourth BtCoV sequence was 96.8 per cent identical to BtCoV HKU9-1. |
title | Results: |
p | Bat samples from the seven States were screened, and the RS specimens of eight Rousettus spp. and 21 Pteropus spp. were found positive for CoV RdRp gene. Among these, by Sanger sequencing, partial RdRp sequences could be retrieved from three Rousettus and eight Pteropus bat specimens. Phylogenetic analysis of the partial RdRp region demonstrated distinct subclustering of the BtCoV sequences retrieved from these Rousettus and Pteropus spp. bats. NGS led to the recovery of four sequences covering approximately 94.3 per cent of the whole genome of the BtCoVs from Rousettus bats. Three BtCoV sequences had 93.69 per cent identity to CoV BtRt-BetaCoV/GX2018. The fourth BtCoV sequence was 96.8 per cent identical to BtCoV HKU9-1. |
sec | Interpretation & conclusions: This study was a step towards understanding the CoV circulation in Indian bats. Detection of potentially pathogenic CoVs in Indian bats stresses the need for enhanced screening for novel viruses in them. One Health approach with collaborative activities by the animal health and human health sectors in these surveillance activities shall be of use to public health. This would help in the development of diagnostic assays for novel viruses with outbreak potential and be useful in disease interventions. Proactive surveillance remains crucial for identifying the emerging novel viruses with epidemic potential and measures for risk mitigation. |
title | Interpretation & conclusions: |
p | This study was a step towards understanding the CoV circulation in Indian bats. Detection of potentially pathogenic CoVs in Indian bats stresses the need for enhanced screening for novel viruses in them. One Health approach with collaborative activities by the animal health and human health sectors in these surveillance activities shall be of use to public health. This would help in the development of diagnostic assays for novel viruses with outbreak potential and be useful in disease interventions. Proactive surveillance remains crucial for identifying the emerging novel viruses with epidemic potential and measures for risk mitigation. |
body | A large number of emerging infectious diseases are known to be zoonotic in origin. In the last two decades, many viruses have been identified from bat species1. Bats have been recognized as the natural reservoirs of a variety of pathogenic viruses such as Rabies, Hendra, Marburg, Nipah and Ebola virus2. Bats are known to harbour coronaviruses (CoVs) and serve as their reservoirs. Alpha-CoV (α-CoV) and beta-CoV (β-CoV) have been detected in bats in Asia, Europe, Africa, North and South America and Australasia3. In the last two decades, bat CoVs (BtCoVs) garnered considerable attention as potential human pathogens45. Severe acute respiratory syndrome (SARS)-CoV-2 causing the current pandemic [CoV disease 2019 (COVID-19)] is also a member of the same genus and found to be similar to bat-derived CoV strain RATG136. SARS-CoV-2 is reported to be 96 per cent identical to BtCoV at the whole genome level, and related viruses were identified in the previously sampled bat population in China7. CoVs are enveloped, single-stranded, positive-sense RNA viruses with a comparatively large genome size of 26 to 32 kb, classified under the family Coronaviridae in the order Nidovirales8. According to the International Committee on Taxonomy of Viruses (ICTV), they are classified into four genera, namely, α-CoV, β-CoV, γ-CoV and δ-CoV)9. β-CoVs are further classified into four different lineages [lineage A (L_A), lineage B (L_B), lineage C (L_C) and lineage D (L_D)]10. Most of the human CoVs are either zoonotic in origin or circulate in animals11. CoVs can cause a wide range of infections, including respiratory tract infections, gastroenteritis, hepatitis and encephalomyelitis in their respective hosts. It is believed that many of the currently circulating α-CoVs and β-CoVs of mammals have evolutionary links to CoVs from bats1. India has a diverse population of bats; around 117 species of bats have been recorded, with around 100 subspecies coming under 39 genera belonging to eight families (Pteropodidae, Rhinolophidae, Hipposideridae, Megadermatidae, Rhinopomatidae, Emballonuridae, Molossidae and Vespertilionidae)12. The Indian Council of Medical Research-National Institute of Virology (ICMR-NIV) at Pune, India, has detected several viruses in bats, including the Nipah virus in Pteropus medius, Malsoor virus, Tioman virus and a novel adenovirus in Rousettus leschenaultii131415. Nipah viral RNA antibodies could be detected in Pteropus bats from many States of India, and the possible link of transmission from bats could be established during the Nipah outbreak which occurred in Kerala in 2018 and 20191617. The use of conventional polymerase chain reaction/reverse transcription-polymerase chain reaction (PCR/RT-PCR), as well as metagenomics and next-generation sequencing (NGS) technologies, has led to the discovery of many novel viruses in bats. The identification of new CoVs in bats in several neighbouring Asian countries such as China3, Sri Lanka18 and Singapore1920 and the growing threats of novel CoV diseases such as COVID-19 led us to investigate Pteropus and Rousettus bats commonly found in India, for identification and characterization of BtCoVs. Material & Methods This study was approved by the Institutional Animal Ethics Committee (IAEC) of ICMR-NIV, Pune (IAEC/2019/MEZ/04). Permissions were also obtained from the Principal Chief Conservators of Forests (PCCF)/wildlife wardens of different States/Union Territories (UT) (Kerala, Karnataka, Tamil Nadu, Himachal Pradesh, Punjab, Gujarat, Odisha, Telangana, Chandigarh and Puducherry). Study sites and sample collection: Upon obtaining permission from the respective State authorities, bat-roosting sites in each State/UT were identified with the help of the forest officials. Bats were trapped using mist nets and were chemically restrained using isoflurane anaesthesia. Throat swabs (TS) and rectal swabs (RSs) were collected in virus transport medium (VTM) and were transported to ICMR-NIV, Pune, on dry ice. The specimens were collected from Pteropus spp. bats from Kerala, Karnataka, Chandigarh, Gujarat, Himachal Pradesh, Odisha, Puducherry, Punjab, Tamil Nadu and Telangana and Rousettus spp. bats from Kerala, Karnataka, Chandigarh, Gujarat, Odisha, Punjab and Telangana States during 2018-2019. These bats were monitored and released after recovery. Twelve bats that died during the trapping process were transported to ICMR-NIV on dry ice. Necropsy of these bats was carried out in the Biosafety Level 4 (BSL-4) containment facility, and tissue specimens (intestine and kidney) collected were tested. Detection of bat coronavirus using RT-PCR: RNA was extracted from the bat specimens using the MagMAX pathogen RNA/DNA isolation kit (Invitrogen, USA). RT-PCR was performed using Superscript III one-step RT-PCR (Invitrogen, USA) with Platinum High-Fidelity Taq polymerase (Invitrogen, USA) using the published BtCoV-specific primers targeting the conserved region of RNA-dependent RNA polymerase (RdRp) gene21. The amplicon of 440 bp was separated on 1.5 per cent agarose gel and visualized under VersaDoc MP 4000 ultraviolet transilluminator (Bio-Rad, USA). Sequencing of the positive coronavirus specimens Sanger sequencing of bat coronavirus: The RT-PCR products were separated on 1.5 per cent agarose gel, and 440 bp bands were excised. The excised gels were extracted and purified using a QIAQuick gel extraction kit (Qiagen, Hilden, Germany). The purified products were quantified, and chain-terminated PCR reactions were performed using pathogen-specific forward and reverse primers21 with the BigDye Terminator 3.1 sequencing kit (Applied Biosystems, USA). BigDye reactions were purified using the DyeEx 2.0 spin kit (Qiagen, Germany). The purified chain-terminated reactions were sequenced using the ABI PRISM® 3100 Automated DNA Sequencer (Thermo Fisher Scientific, USA). The sequence data generated were assembled using the Sequencer 5.1 software (Accelrys Inc., USA). Next-generation sequencing (NGS) of bat coronavirus: Selected bat specimens were used for RNA extraction2223. RNA libraries were prepared and quantified by Qubit® 2.0 Fluorometer (Invitrogen, USA). NEB NextrRNA depletion kit (New England Biolabs, USA) was used to remove host ribosomal RNA and re-quantified using Qubit® 2.0 Fluorometer (Invitrogen, USA). In brief, the RNA library preparation involved fragmentation, adenylation, adapter ligation and amplification. The amplified libraries were quantified using KAPA Library Quantification Kit (KapaBiosystems, Roche, Switzerland) as per the manufacturer's protocol and further loaded onto the Illumina Miniseq NGS platform (Illumina, USA)2223. The FASTQ files generated after the completion of the run were analyzed using CLC Genomics Workbench software version 11 (CLC, Qiagen, Germany). De novo assembly programme was used to assemble contiguous sequences (contigs). The contigs generated were analyzed using BLAST (https://blast.ncbi.nlm.nih.gov/Blast.cgi) to identify matching sequences. The closest matching sequence from GenBank (https://www.ncbi.nlm.nih.gov/genbank/) was used for reference mapping. Phylogenetic analysis of partial and complete genome sequences of bat coronavirus: The CoV sequences retrieved from RS specimens of Rousettus spp. bats (n=4) were aligned with whole-genome sequences from GenBank using the create alignment function of the CLC genomics workbench (https://digitalinsights.qiagen.com). Partial RdRp gene sequences (~419 bp) retrieved by Sanger sequencing, for both the bat species specimens (genomic location: 14,701-15,120) were used to construct a phylogenetic tree along with the available RdRp sequences in GenBank. Phylogenetic analysis was carried out using the neighbour-joining method available in MEGA v7 software24 using the Kimura 2-parameter nucleotide (nt) substitution model with 1000 bootstrap replicates. The nt divergence for the open reading frame (ORF) 1a polyprotein (ORF 1a), ORF 1b polyprotein (ORF 1b), spike protein (S), nucleocapsid phospoprotein (N), envelope protein (E) and membrane glycoprotein (M) genes was estimated using the Kimura 2-parameter model as implemented in the MEGA software. The sequences retrieved in the current study, along with those downloaded from GenBank, were grouped into the genus. The viruses from the β-CoV genus were further grouped into lineages, L_A, L_B, L_C and L_D, to estimate the evolutionary divergence over the respective gene sequence pairs between groups using the MEGA software24. The distance was estimated using a Kimura 2-parameter model with uniform rates among the sites. The bootstrap of 500 replicates was used to estimate the variation in the model. Results The TS and RS specimens for 78 Rousettus spp. bats were collected in VTM from seven States (Kerala, Karnataka, Chandigarh, Gujarat, Odisha, Punjab and Telangana). The TS and RS specimens of 508 Pteropus spp. bats were also collected in VTM from 10 States/UTs in India (Kerala, Karnataka, Chandigarh, Gujarat, Himachal Pradesh, Odisha, Puducherry, Punjab, Tamil Nadu and Telangana). During the trapping process, 12 (8 Rousettus and 4 Pteropus spp.) bats died. Organ specimens (intestine and kidney) were collected from these bats (TS and RS specimens of these 12 bats were included in the total number of samples). Detection of bat coronavirus using RdRp gene RT-PCR: Four of the 78 RS of Rousettus spp. bats screened for the BtCoV were found positive. All the positive RS samples belonged to Kerala State. Intestinal specimens of two bats were also found to be positive for the BtCoV. One bat (MCL-19-Bat-606), from Kerala, was tested positive in both the intestinal specimen and the RS. The second bat (MCL-20-Bat-76), from Karnataka, was tested positive only in the intestinal specimen. Altogether, five Rousettus spp. bats were positive for the BtCoV. All TS specimens from Rousettus spp. were found negative for BtCoV (Table I). Table I Bat coronavirus positivity in bat specimens screened using RNA-dependent RNA polymerase (RdRp) gene-specific reverse transcription-polymerase chain reaction (RT-PCR) in different States Place of collection Number of positive/number tested (%) for different bat species for BtCoV RdRp gene-specific RT-PCR Pteropus bats (%) Rousettus bats (%) Rectal swabs Throat swabs Rectal swabs Throat swabs Kerala 12/217 (5.53) 0/21 (0.00) 4/42 (9.52) 0/4 (0.00) Karnataka 0/78 (0.00) NT 0/4 (0.00) 0/4 (0.00) Chandigarh 0/27 (0.00) NT 0/6 (0.00) 0/6 (0.00) Gujarat 0/30 (0.00) NT 0/18 (0.00) 0/18 (0.00) Odisha 0/30 (0.00) NT 0/2 (0.00) 0/2 (0.00) Punjab 0/14 (0.00) NT 0/2 (0.00) 0/2 (0.00) Telangana 0/30 (0.00) NT 0/4 (0.00) 0/4 (0.00) Himachal Pradesh 2/29 (6.89) 0/6 (0.00) NA NA Puducherry 6/23 (26.09) 0/10 (0.00) NA NA Tamil Nadu 1/30 (3.33) 0/5 (0.00) NA NA 21/508 (4.13) 0/42 (0.00) 4/78 (5.13) 0/40 (0.00) NT, not tested; NA, not available; BtCoV, bat coronavirus Twenty one of the 508 RSs from Pteropus spp. bats screened were tested positive for the BtCoV (Table I). These positive bats belonged to Kerala (n=12), Himachal Pradesh (n=2), Puducherry (n=6) and Tamil Nadu (n=1). The TS specimens of the same bats were tested negative for BtCoV. The TS specimens of RS-negative (n=42) bats were also screened and found to be negative (Table I). A total of 25 bats from both the species were found positive. Sequencing of the positive coronavirus specimens Sanger sequencing of bat coronavirus: Using the Sanger sequencing protocol, partial RdRp sequences of BtCoV were retrieved from two (out of 4 amplicons) specimens of Rousettus spp. One of the sequences (MCL-19-bat-588/2) showed close identity to BtCoV HKU9-5-2 (AN): HM211099.1; sequence identity (SI): 99.2 per cent, whereas the second RdRp sequence (MCL-20-bat-76/10) had an SI of 98.8 per cent with BtCoV HKU9-1 (AN: EF065513.1), both from China. Sanger's sequencing protocol led to retrieval of eight partial RdRp sequences which belonged to Pteropus spp. These bats were collected from Kerala (n=5) and Tamil Nadu (n=3) States. One of the three partial RdRp sequences from Tamil Nadu had 97.93 per cent SI with BtCoV/B55951/Pte_lyl/CB2-THA (AN: MG256459.1, Thailand). The other two sequences had a minimum of 99.48 per cent SI with the CoV PREDICT_CoV-17/PB072 (AN: KX284942.1, Nepal). One of the five partial RdRp sequences from Kerala had 98.88 per cent SI with BtCoV/B55951/Pte_lyl/CB2-THA (AN: MG256459.1, Thailand). The remaining four partial RdRp sequences had >97 per cent SI with CoV PREDICT_CoV-17/PB072 (AN: KX284942.1, Nepal). Next-generation sequencing of bat coronavirus: NGS was performed on 10 specimens [4 RS, 2 kidney and 4 intestinal tissue) of the five Rousettus bats to retrieve the complete genome of the BtCoV. Kidney and intestine tissues of the bats from Karnataka State (MCL-20-Bat-76) and RS along with intestine tissue of bats from Kerala State (MCL-19-Bat-606) were used for sequencing and analysis. Two different viruses were retrieved based on the BLAST analysis of the sequences from the kidney and intestine tissues of the bats from Karnataka. Kidney specimen of MCL-20-Bat-76 had an SI of 94 per cent and query coverage (QC) of 94 per cent with CoV BtRt-BetaCoV/GX2018 (AN: MK211379.1), whereas the intestine tissue of the MCL-20-Bat-76 had an SI of 96.8 and 95 per cent QC with the BtCoV HKU9-1 (AN: EF065513.1). The sequences from RS and intestine tissue of the MCL-19-Bat-606 from Kerala, had 93.69 and 93.99 per cent SI to CoV BtRt-BetaCoV/GX2018 (AN: MK211379.1), respectively, with 100 per cent QC. Further, 99.8 per cent of the CoV BtRt-BetaCoV/GX2018 sequences were retrieved from the intestine specimen of the MCL-19-Bat-606. The details of the genome recovered reads mapped and the per cent of reads mapped are summarized in Table II. Table II Details of the genome recovered reads mapped and the per cent of reads mapped from the Rousettus bat samples Sample details Sample type Virus retrieved Relevant reads Per cent of reads Per cent of genome recovered MCL-20-Bat-76 Kidney Coronavirus BtRt-BetaCoV/GX2018 1632 0.015 94.39 Intestine BtCoV HKU9-1 4499 0.056 95.75 MCL-19-Bat-606 Rectal swab Coronavirus BtRt-BetaCoV/GX2018 13,973 0.114 99.53 Intestine Coronavirus BtRt-BetaCoV/GX2018 10,214,492 93.476 99.87 Phylogenetic analysis of partial and complete genome sequences of bat coronavirus: A neighbour-joining tree was generated using the partial RdRp region sequences derived from Pteropus and Rousettus spp. bat specimens. It was observed that all the BtCoV sequences were clustered within the L_D sequences of beta CoVs. A distinct subclustering of the sequences retrieved from Pteropus and Rousettus spp. bats is shown in Figure 1. The sequences in the light pink colour are retrieved from the Pteropus spp., whereas those in the dark pink region belong to Rousettus spp. The sequence divergence of 0.35 was observed between Pteropus spp. and Rousettus spp., which was obtained by averaging over all the sequence pairs between the two species, determining those to be distinct sequences to each species. Fig. 1 Neighbour-joining tree for the RNA-dependent RNA polymerase (RdRp) partial sequence (genomic location: 14,701-15,120) generated from Sanger sequencing. The tree was constructed using the RdRp sequence available in the GenBank sequences. Kimura 2-parameter model was used as the substitution model to generate the tree. A bootstrap replication of 1000 cycles was performed to generate the tree to assess the statistical robustness. The complete genome sequences of four BtCoV obtained from Rousettus spp. specimens were used for generating a neighbour-joining tree (Fig. 2). These sequences were also clustered within L_D of β-CoVs as observed for partial RdRp sequence tree. These complete genome sequences were grouped into gene pairs to identify the gene with higher and lower divergence. The complete genomes of the Indian BtCoV sequences were grouped under L_D. The evolutionary divergence of ORF 1b was <0.54 between the different β-CoV lineages with a maximum score of 0.7 between different BtCoV sequences used in this study (Table III). E gene sequences had larger divergence within the β-CoV genus ranging from 2.18 to 0.94. Lineages L_A and L_C had the maximum divergence of 2.18, whereas the L_B and L_C were the least (0.94). N gene has an overall higher divergence among different lineages (ranging: 2.08-0.75). Overall, evolutionary divergence for the sequences of each gene pair demonstrated that S, N, E and M genes from the α- and δ-CoV highly diverged across the different genus. In contrast, the ORF 1b was less divergent across the genera (Table III). Fig. 2 Phylogenetic tree for the complete genome tree: A neighbour-joining tree was generated using the representative complete genome sequence available in the GenBank sequences. Kimura 2-parameter model was used as the substitution model to generate the tree. A bootstrap replication of 1000 cycles was performed to generate the tree to assess the statistical robustness. Table III Evolutionary divergence for ORF 1b, S, N and M genes for the retrieved sequences with other reference sequences. The lower right-check hand matrix of the table depicts the divergence and the upper left-check matrix of the matrix (blue colour) depicts the variation observed in the bootstrap replication N gene Alpha Delta Gamma L_A L_B L_C L_D M gene Alpha Delta Gamma L_A L_B L_C L_D Alpha 0.15 0.09 0.10 0.08 0.08 0.09 Alpha 0.11 0.12 0.05 0.06 0.05 0.06 Delta 2.08 0.11 0.16 0.09 0.11 0.10 Delta 1.50 0.26 0.08 0.16 0.10 0.11 Gamma 1.57 1.49 0.08 0.08 0.09 0.08 Gamma 1.53 1.84 0.10 0.12 0.11 0.09 L_A 1.84 1.73 1.37 0.05 0.05 0.06 L_A 0.92 1.24 1.30 0.06 0.05 0.05 L_B 1.48 1.37 1.32 1.09 0.03 0.04 L_B 1.05 1.51 1.37 0.92 0.05 0.05 L_C 1.57 1.52 1.42 1.07 0.75 0.04 L_C 0.99 1.35 1.27 0.80 0.82 0.05 L_D 1.64 1.46 1.36 1.27 0.90 0.97 L_D 0.99 1.42 1.23 0.84 0.79 0.82 ORF 1b Alpha Delta Gamma L_A L_B L_C L_D ORF 1a Alpha Delta Gamma L_A L_B L_C L_D Alpha 0.01 0.01 0.01 0.01 0.01 0.01 Alpha 0.02 0.02 0.01 0.02 0.02 0.03 Delta 0.70 0.01 0.02 0.01 0.01 0.01 Delta 1.32 0.03 0.02 0.03 0.03 0.04 Gamma 0.62 0.67 0.01 0.01 0.01 0.01 Gamma 1.14 1.33 0.02 0.03 0.02 0.04 L_A 0.61 0.69 0.60 0.01 0.01 0.01 L_A 1.22 1.01 1.30 0.02 0.02 0.04 L_B 0.60 0.70 0.65 0.54 0.01 0.01 L_B 1.26 1.42 1.41 1.19 0.01 0.02 L_C 0.58 0.69 0.62 0.53 0.50 0.01 L_C 1.35 1.41 1.44 1.19 0.97 0.03 L_D 0.60 0.67 0.61 0.53 0.50 0.52 L_D 1.26 1.27 1.39 1.09 0.90 1.03 S gene Alpha Delta Gamma L_A L_B L_C L_D E gene Alpha Delta Gamma L_A L_B L_C L_D Alpha 0.02 0.02 0.03 0.03 0.03 0.02 Alpha 0.12 0.18 0.09 0.15 0.15 0.12 Delta 0.86 0.03 0.04 0.04 0.06 0.03 Delta 1.14 0.47 0.22 0.41 0.28 0.17 Gamma 1.14 0.96 0.04 0.05 0.06 0.04 Gamma 1.59 1.64 0.22 0.24 0.32 0.19 L_A 1.36 1.28 1.43 0.03 0.03 0.02 L_A 1.03 1.58 1.57 0.23 0.21 0.25 L_B 1.33 1.23 1.34 1.19 0.04 0.02 L_B 1.24 1.75 1.40 1.83 0.11 0.14 L_C 1.42 1.32 1.46 1.17 1.03 0.03 L_C 1.37 1.64 1.83 2.18 0.94 0.17 L_D 1.34 1.24 1.41 1.16 1.00 1.11 L_D 1.25 1.42 1.52 1.95 1.16 1.37 ORF 1a, open reading frame 1a polyprotein; ORF 1b, ORF 1b polyprotein; S, spike glycoprotein; N, nuclocapsid phospoptotein; M, membrane glycoprotein; E, envelope protein Discussion As per the available information, the BtCoV causing human infection belongs to α- and β-CoV genera of the Coronaviridae family. β-CoV genus has five strains known to infect humans25. The two human-infecting strains (NL63 and 229E) from α-CoV genus which cause mild-to-moderate respiratory infections are believed to have originated in bats25. Two members of the β-CoV genus (HCoV-OC43 and HCoV-HKU1) are known to cause the common cold and lower respiratory tract infections26. The other three are now shown to be pathogenic to humans (SARS-CoV-1, MERS-CoV and SARS-CoV-2). The SARS-CoV-1 and SARS-CoV-2 belong to L_B and MERS CoV belongs to L_C of β-CoV genus27. The phylogenetic analysis for the partial RdRp region revealed the presence of distinct BtCoVs in both the bats. The genomic sequences retrieved from the Indian sequences form a distinct cluster. The three CoV_BtRtBetaCoV/GX2018 sequences retrieved from the Indian Rousettus bats were 5.8-6.7 per cent different from the reference sequence, which was retrieved from Rhinolophus affinis. The two CoV_BtRtBetaCoV/GX2018 sequences retrieved from different bats were 1.2 per cent different from each other. The effect of host influence on the nt usage of the virus cannot be denied; however, it needs to be explored further in detail. Bats are reservoirs for viruses with human pathogenic potential2829, and are known to harbour a broad range of CoVs1. The global distribution of bats, along with the different types of cell receptors present within them, favours virus replication, and is a possible link to their intraspecies transmission. The interspecies spill-over of a BtCoV to humans is thought to occur through an intermediate host, in which the virus replicates through yet completely unidentified routes. In India, regions of the Western Ghats, particularly in Kerala, are reported to have habitat for diverse bat populations. The reports of pathogenic human viruses from bat specimens demand enhanced methods to monitor human exposure to various bat species. Investigations in unexplored regions/States should be focused on gaining further insights into CoV diversity within Indian bat populations. Earlier, we had reported the presence of pathogenic viruses such as the Nipah virus in Pteropus bats in India16. In the present scenario of changing demography and ecological manipulations, it is challenging to have checks on the encounters of bats with other animals and humans. Therefore, active and continuous surveillance remains crucial for outbreak alerts for bat-associated viral agents with epidemic potential, which would be helpful in timely interventions. Although CoVs in the subfamily Coronavirinae do not usually produce clinical symptoms in their natural hosts (bats), accidental transmission of these viruses to humans and other animals may result in respiratory, enteric, hepatic or neurologic diseases of variable severity. It is still not understood as to why only certain CoVs can infect people. There is a need of proactive surveillance of zoonotic infections in bats. Detection and identifications of such aetiological agents will provide leads for the development of diagnostic along with preparedness and readiness to deal with such emergent viruses thereby quickly containing them. The detection and identification of such viruses from bats also recommends cross-sectional antibody surveys (human and domestic animals) in localities where the viruses have been detected. Similarly, if epidemiological situation demands, evidence-based surveillance should also be conducted. There is a need of developing strong mechanisms for working jointly with various stakeholders such as wildlife, poultry, animal husbandry and human health departments. In conclusion, our study showed detection of pathogenic CoVs in two species of Indian bats. Continuous active surveillance is required to identify the emerging novel viruses with epidemic potential. |
p | A large number of emerging infectious diseases are known to be zoonotic in origin. In the last two decades, many viruses have been identified from bat species1. Bats have been recognized as the natural reservoirs of a variety of pathogenic viruses such as Rabies, Hendra, Marburg, Nipah and Ebola virus2. Bats are known to harbour coronaviruses (CoVs) and serve as their reservoirs. Alpha-CoV (α-CoV) and beta-CoV (β-CoV) have been detected in bats in Asia, Europe, Africa, North and South America and Australasia3. In the last two decades, bat CoVs (BtCoVs) garnered considerable attention as potential human pathogens45. Severe acute respiratory syndrome (SARS)-CoV-2 causing the current pandemic [CoV disease 2019 (COVID-19)] is also a member of the same genus and found to be similar to bat-derived CoV strain RATG136. SARS-CoV-2 is reported to be 96 per cent identical to BtCoV at the whole genome level, and related viruses were identified in the previously sampled bat population in China7. |
p | CoVs are enveloped, single-stranded, positive-sense RNA viruses with a comparatively large genome size of 26 to 32 kb, classified under the family Coronaviridae in the order Nidovirales8. According to the International Committee on Taxonomy of Viruses (ICTV), they are classified into four genera, namely, α-CoV, β-CoV, γ-CoV and δ-CoV)9. β-CoVs are further classified into four different lineages [lineage A (L_A), lineage B (L_B), lineage C (L_C) and lineage D (L_D)]10. Most of the human CoVs are either zoonotic in origin or circulate in animals11. CoVs can cause a wide range of infections, including respiratory tract infections, gastroenteritis, hepatitis and encephalomyelitis in their respective hosts. It is believed that many of the currently circulating α-CoVs and β-CoVs of mammals have evolutionary links to CoVs from bats1. |
p | India has a diverse population of bats; around 117 species of bats have been recorded, with around 100 subspecies coming under 39 genera belonging to eight families (Pteropodidae, Rhinolophidae, Hipposideridae, Megadermatidae, Rhinopomatidae, Emballonuridae, Molossidae and Vespertilionidae)12. The Indian Council of Medical Research-National Institute of Virology (ICMR-NIV) at Pune, India, has detected several viruses in bats, including the Nipah virus in Pteropus medius, Malsoor virus, Tioman virus and a novel adenovirus in Rousettus leschenaultii131415. Nipah viral RNA antibodies could be detected in Pteropus bats from many States of India, and the possible link of transmission from bats could be established during the Nipah outbreak which occurred in Kerala in 2018 and 20191617. The use of conventional polymerase chain reaction/reverse transcription-polymerase chain reaction (PCR/RT-PCR), as well as metagenomics and next-generation sequencing (NGS) technologies, has led to the discovery of many novel viruses in bats. The identification of new CoVs in bats in several neighbouring Asian countries such as China3, Sri Lanka18 and Singapore1920 and the growing threats of novel CoV diseases such as COVID-19 led us to investigate Pteropus and Rousettus bats commonly found in India, for identification and characterization of BtCoVs. |
sec | Material & Methods This study was approved by the Institutional Animal Ethics Committee (IAEC) of ICMR-NIV, Pune (IAEC/2019/MEZ/04). Permissions were also obtained from the Principal Chief Conservators of Forests (PCCF)/wildlife wardens of different States/Union Territories (UT) (Kerala, Karnataka, Tamil Nadu, Himachal Pradesh, Punjab, Gujarat, Odisha, Telangana, Chandigarh and Puducherry). Study sites and sample collection: Upon obtaining permission from the respective State authorities, bat-roosting sites in each State/UT were identified with the help of the forest officials. Bats were trapped using mist nets and were chemically restrained using isoflurane anaesthesia. Throat swabs (TS) and rectal swabs (RSs) were collected in virus transport medium (VTM) and were transported to ICMR-NIV, Pune, on dry ice. The specimens were collected from Pteropus spp. bats from Kerala, Karnataka, Chandigarh, Gujarat, Himachal Pradesh, Odisha, Puducherry, Punjab, Tamil Nadu and Telangana and Rousettus spp. bats from Kerala, Karnataka, Chandigarh, Gujarat, Odisha, Punjab and Telangana States during 2018-2019. These bats were monitored and released after recovery. Twelve bats that died during the trapping process were transported to ICMR-NIV on dry ice. Necropsy of these bats was carried out in the Biosafety Level 4 (BSL-4) containment facility, and tissue specimens (intestine and kidney) collected were tested. Detection of bat coronavirus using RT-PCR: RNA was extracted from the bat specimens using the MagMAX pathogen RNA/DNA isolation kit (Invitrogen, USA). RT-PCR was performed using Superscript III one-step RT-PCR (Invitrogen, USA) with Platinum High-Fidelity Taq polymerase (Invitrogen, USA) using the published BtCoV-specific primers targeting the conserved region of RNA-dependent RNA polymerase (RdRp) gene21. The amplicon of 440 bp was separated on 1.5 per cent agarose gel and visualized under VersaDoc MP 4000 ultraviolet transilluminator (Bio-Rad, USA). Sequencing of the positive coronavirus specimens Sanger sequencing of bat coronavirus: The RT-PCR products were separated on 1.5 per cent agarose gel, and 440 bp bands were excised. The excised gels were extracted and purified using a QIAQuick gel extraction kit (Qiagen, Hilden, Germany). The purified products were quantified, and chain-terminated PCR reactions were performed using pathogen-specific forward and reverse primers21 with the BigDye Terminator 3.1 sequencing kit (Applied Biosystems, USA). BigDye reactions were purified using the DyeEx 2.0 spin kit (Qiagen, Germany). The purified chain-terminated reactions were sequenced using the ABI PRISM® 3100 Automated DNA Sequencer (Thermo Fisher Scientific, USA). The sequence data generated were assembled using the Sequencer 5.1 software (Accelrys Inc., USA). Next-generation sequencing (NGS) of bat coronavirus: Selected bat specimens were used for RNA extraction2223. RNA libraries were prepared and quantified by Qubit® 2.0 Fluorometer (Invitrogen, USA). NEB NextrRNA depletion kit (New England Biolabs, USA) was used to remove host ribosomal RNA and re-quantified using Qubit® 2.0 Fluorometer (Invitrogen, USA). In brief, the RNA library preparation involved fragmentation, adenylation, adapter ligation and amplification. The amplified libraries were quantified using KAPA Library Quantification Kit (KapaBiosystems, Roche, Switzerland) as per the manufacturer's protocol and further loaded onto the Illumina Miniseq NGS platform (Illumina, USA)2223. The FASTQ files generated after the completion of the run were analyzed using CLC Genomics Workbench software version 11 (CLC, Qiagen, Germany). De novo assembly programme was used to assemble contiguous sequences (contigs). The contigs generated were analyzed using BLAST (https://blast.ncbi.nlm.nih.gov/Blast.cgi) to identify matching sequences. The closest matching sequence from GenBank (https://www.ncbi.nlm.nih.gov/genbank/) was used for reference mapping. Phylogenetic analysis of partial and complete genome sequences of bat coronavirus: The CoV sequences retrieved from RS specimens of Rousettus spp. bats (n=4) were aligned with whole-genome sequences from GenBank using the create alignment function of the CLC genomics workbench (https://digitalinsights.qiagen.com). Partial RdRp gene sequences (~419 bp) retrieved by Sanger sequencing, for both the bat species specimens (genomic location: 14,701-15,120) were used to construct a phylogenetic tree along with the available RdRp sequences in GenBank. Phylogenetic analysis was carried out using the neighbour-joining method available in MEGA v7 software24 using the Kimura 2-parameter nucleotide (nt) substitution model with 1000 bootstrap replicates. The nt divergence for the open reading frame (ORF) 1a polyprotein (ORF 1a), ORF 1b polyprotein (ORF 1b), spike protein (S), nucleocapsid phospoprotein (N), envelope protein (E) and membrane glycoprotein (M) genes was estimated using the Kimura 2-parameter model as implemented in the MEGA software. The sequences retrieved in the current study, along with those downloaded from GenBank, were grouped into the genus. The viruses from the β-CoV genus were further grouped into lineages, L_A, L_B, L_C and L_D, to estimate the evolutionary divergence over the respective gene sequence pairs between groups using the MEGA software24. The distance was estimated using a Kimura 2-parameter model with uniform rates among the sites. The bootstrap of 500 replicates was used to estimate the variation in the model. |
title | Material & Methods |
p | This study was approved by the Institutional Animal Ethics Committee (IAEC) of ICMR-NIV, Pune (IAEC/2019/MEZ/04). Permissions were also obtained from the Principal Chief Conservators of Forests (PCCF)/wildlife wardens of different States/Union Territories (UT) (Kerala, Karnataka, Tamil Nadu, Himachal Pradesh, Punjab, Gujarat, Odisha, Telangana, Chandigarh and Puducherry). |
p | Study sites and sample collection: Upon obtaining permission from the respective State authorities, bat-roosting sites in each State/UT were identified with the help of the forest officials. Bats were trapped using mist nets and were chemically restrained using isoflurane anaesthesia. Throat swabs (TS) and rectal swabs (RSs) were collected in virus transport medium (VTM) and were transported to ICMR-NIV, Pune, on dry ice. The specimens were collected from Pteropus spp. bats from Kerala, Karnataka, Chandigarh, Gujarat, Himachal Pradesh, Odisha, Puducherry, Punjab, Tamil Nadu and Telangana and Rousettus spp. bats from Kerala, Karnataka, Chandigarh, Gujarat, Odisha, Punjab and Telangana States during 2018-2019. These bats were monitored and released after recovery. Twelve bats that died during the trapping process were transported to ICMR-NIV on dry ice. Necropsy of these bats was carried out in the Biosafety Level 4 (BSL-4) containment facility, and tissue specimens (intestine and kidney) collected were tested. |
p | Detection of bat coronavirus using RT-PCR: RNA was extracted from the bat specimens using the MagMAX pathogen RNA/DNA isolation kit (Invitrogen, USA). RT-PCR was performed using Superscript III one-step RT-PCR (Invitrogen, USA) with Platinum High-Fidelity Taq polymerase (Invitrogen, USA) using the published BtCoV-specific primers targeting the conserved region of RNA-dependent RNA polymerase (RdRp) gene21. The amplicon of 440 bp was separated on 1.5 per cent agarose gel and visualized under VersaDoc MP 4000 ultraviolet transilluminator (Bio-Rad, USA). |
sec | Sequencing of the positive coronavirus specimens Sanger sequencing of bat coronavirus: The RT-PCR products were separated on 1.5 per cent agarose gel, and 440 bp bands were excised. The excised gels were extracted and purified using a QIAQuick gel extraction kit (Qiagen, Hilden, Germany). The purified products were quantified, and chain-terminated PCR reactions were performed using pathogen-specific forward and reverse primers21 with the BigDye Terminator 3.1 sequencing kit (Applied Biosystems, USA). BigDye reactions were purified using the DyeEx 2.0 spin kit (Qiagen, Germany). The purified chain-terminated reactions were sequenced using the ABI PRISM® 3100 Automated DNA Sequencer (Thermo Fisher Scientific, USA). The sequence data generated were assembled using the Sequencer 5.1 software (Accelrys Inc., USA). Next-generation sequencing (NGS) of bat coronavirus: Selected bat specimens were used for RNA extraction2223. RNA libraries were prepared and quantified by Qubit® 2.0 Fluorometer (Invitrogen, USA). NEB NextrRNA depletion kit (New England Biolabs, USA) was used to remove host ribosomal RNA and re-quantified using Qubit® 2.0 Fluorometer (Invitrogen, USA). In brief, the RNA library preparation involved fragmentation, adenylation, adapter ligation and amplification. The amplified libraries were quantified using KAPA Library Quantification Kit (KapaBiosystems, Roche, Switzerland) as per the manufacturer's protocol and further loaded onto the Illumina Miniseq NGS platform (Illumina, USA)2223. The FASTQ files generated after the completion of the run were analyzed using CLC Genomics Workbench software version 11 (CLC, Qiagen, Germany). De novo assembly programme was used to assemble contiguous sequences (contigs). The contigs generated were analyzed using BLAST (https://blast.ncbi.nlm.nih.gov/Blast.cgi) to identify matching sequences. The closest matching sequence from GenBank (https://www.ncbi.nlm.nih.gov/genbank/) was used for reference mapping. Phylogenetic analysis of partial and complete genome sequences of bat coronavirus: The CoV sequences retrieved from RS specimens of Rousettus spp. bats (n=4) were aligned with whole-genome sequences from GenBank using the create alignment function of the CLC genomics workbench (https://digitalinsights.qiagen.com). Partial RdRp gene sequences (~419 bp) retrieved by Sanger sequencing, for both the bat species specimens (genomic location: 14,701-15,120) were used to construct a phylogenetic tree along with the available RdRp sequences in GenBank. Phylogenetic analysis was carried out using the neighbour-joining method available in MEGA v7 software24 using the Kimura 2-parameter nucleotide (nt) substitution model with 1000 bootstrap replicates. The nt divergence for the open reading frame (ORF) 1a polyprotein (ORF 1a), ORF 1b polyprotein (ORF 1b), spike protein (S), nucleocapsid phospoprotein (N), envelope protein (E) and membrane glycoprotein (M) genes was estimated using the Kimura 2-parameter model as implemented in the MEGA software. The sequences retrieved in the current study, along with those downloaded from GenBank, were grouped into the genus. The viruses from the β-CoV genus were further grouped into lineages, L_A, L_B, L_C and L_D, to estimate the evolutionary divergence over the respective gene sequence pairs between groups using the MEGA software24. The distance was estimated using a Kimura 2-parameter model with uniform rates among the sites. The bootstrap of 500 replicates was used to estimate the variation in the model. |
sec | Sequencing of the positive coronavirus specimens Sanger sequencing of bat coronavirus: The RT-PCR products were separated on 1.5 per cent agarose gel, and 440 bp bands were excised. The excised gels were extracted and purified using a QIAQuick gel extraction kit (Qiagen, Hilden, Germany). The purified products were quantified, and chain-terminated PCR reactions were performed using pathogen-specific forward and reverse primers21 with the BigDye Terminator 3.1 sequencing kit (Applied Biosystems, USA). BigDye reactions were purified using the DyeEx 2.0 spin kit (Qiagen, Germany). The purified chain-terminated reactions were sequenced using the ABI PRISM® 3100 Automated DNA Sequencer (Thermo Fisher Scientific, USA). The sequence data generated were assembled using the Sequencer 5.1 software (Accelrys Inc., USA). Next-generation sequencing (NGS) of bat coronavirus: Selected bat specimens were used for RNA extraction2223. RNA libraries were prepared and quantified by Qubit® 2.0 Fluorometer (Invitrogen, USA). NEB NextrRNA depletion kit (New England Biolabs, USA) was used to remove host ribosomal RNA and re-quantified using Qubit® 2.0 Fluorometer (Invitrogen, USA). In brief, the RNA library preparation involved fragmentation, adenylation, adapter ligation and amplification. The amplified libraries were quantified using KAPA Library Quantification Kit (KapaBiosystems, Roche, Switzerland) as per the manufacturer's protocol and further loaded onto the Illumina Miniseq NGS platform (Illumina, USA)2223. The FASTQ files generated after the completion of the run were analyzed using CLC Genomics Workbench software version 11 (CLC, Qiagen, Germany). De novo assembly programme was used to assemble contiguous sequences (contigs). The contigs generated were analyzed using BLAST (https://blast.ncbi.nlm.nih.gov/Blast.cgi) to identify matching sequences. The closest matching sequence from GenBank (https://www.ncbi.nlm.nih.gov/genbank/) was used for reference mapping. Phylogenetic analysis of partial and complete genome sequences of bat coronavirus: The CoV sequences retrieved from RS specimens of Rousettus spp. bats (n=4) were aligned with whole-genome sequences from GenBank using the create alignment function of the CLC genomics workbench (https://digitalinsights.qiagen.com). Partial RdRp gene sequences (~419 bp) retrieved by Sanger sequencing, for both the bat species specimens (genomic location: 14,701-15,120) were used to construct a phylogenetic tree along with the available RdRp sequences in GenBank. Phylogenetic analysis was carried out using the neighbour-joining method available in MEGA v7 software24 using the Kimura 2-parameter nucleotide (nt) substitution model with 1000 bootstrap replicates. The nt divergence for the open reading frame (ORF) 1a polyprotein (ORF 1a), ORF 1b polyprotein (ORF 1b), spike protein (S), nucleocapsid phospoprotein (N), envelope protein (E) and membrane glycoprotein (M) genes was estimated using the Kimura 2-parameter model as implemented in the MEGA software. The sequences retrieved in the current study, along with those downloaded from GenBank, were grouped into the genus. The viruses from the β-CoV genus were further grouped into lineages, L_A, L_B, L_C and L_D, to estimate the evolutionary divergence over the respective gene sequence pairs between groups using the MEGA software24. The distance was estimated using a Kimura 2-parameter model with uniform rates among the sites. The bootstrap of 500 replicates was used to estimate the variation in the model. |
title | Sequencing of the positive coronavirus specimens |
p | Sanger sequencing of bat coronavirus: The RT-PCR products were separated on 1.5 per cent agarose gel, and 440 bp bands were excised. The excised gels were extracted and purified using a QIAQuick gel extraction kit (Qiagen, Hilden, Germany). The purified products were quantified, and chain-terminated PCR reactions were performed using pathogen-specific forward and reverse primers21 with the BigDye Terminator 3.1 sequencing kit (Applied Biosystems, USA). BigDye reactions were purified using the DyeEx 2.0 spin kit (Qiagen, Germany). The purified chain-terminated reactions were sequenced using the ABI PRISM® 3100 Automated DNA Sequencer (Thermo Fisher Scientific, USA). The sequence data generated were assembled using the Sequencer 5.1 software (Accelrys Inc., USA). |
p | Next-generation sequencing (NGS) of bat coronavirus: Selected bat specimens were used for RNA extraction2223. RNA libraries were prepared and quantified by Qubit® 2.0 Fluorometer (Invitrogen, USA). NEB NextrRNA depletion kit (New England Biolabs, USA) was used to remove host ribosomal RNA and re-quantified using Qubit® 2.0 Fluorometer (Invitrogen, USA). In brief, the RNA library preparation involved fragmentation, adenylation, adapter ligation and amplification. The amplified libraries were quantified using KAPA Library Quantification Kit (KapaBiosystems, Roche, Switzerland) as per the manufacturer's protocol and further loaded onto the Illumina Miniseq NGS platform (Illumina, USA)2223. |
p | The FASTQ files generated after the completion of the run were analyzed using CLC Genomics Workbench software version 11 (CLC, Qiagen, Germany). De novo assembly programme was used to assemble contiguous sequences (contigs). The contigs generated were analyzed using BLAST (https://blast.ncbi.nlm.nih.gov/Blast.cgi) to identify matching sequences. The closest matching sequence from GenBank (https://www.ncbi.nlm.nih.gov/genbank/) was used for reference mapping. |
p | Phylogenetic analysis of partial and complete genome sequences of bat coronavirus: The CoV sequences retrieved from RS specimens of Rousettus spp. bats (n=4) were aligned with whole-genome sequences from GenBank using the create alignment function of the CLC genomics workbench (https://digitalinsights.qiagen.com). Partial RdRp gene sequences (~419 bp) retrieved by Sanger sequencing, for both the bat species specimens (genomic location: 14,701-15,120) were used to construct a phylogenetic tree along with the available RdRp sequences in GenBank. Phylogenetic analysis was carried out using the neighbour-joining method available in MEGA v7 software24 using the Kimura 2-parameter nucleotide (nt) substitution model with 1000 bootstrap replicates. The nt divergence for the open reading frame (ORF) 1a polyprotein (ORF 1a), ORF 1b polyprotein (ORF 1b), spike protein (S), nucleocapsid phospoprotein (N), envelope protein (E) and membrane glycoprotein (M) genes was estimated using the Kimura 2-parameter model as implemented in the MEGA software. The sequences retrieved in the current study, along with those downloaded from GenBank, were grouped into the genus. |
p | The viruses from the β-CoV genus were further grouped into lineages, L_A, L_B, L_C and L_D, to estimate the evolutionary divergence over the respective gene sequence pairs between groups using the MEGA software24. The distance was estimated using a Kimura 2-parameter model with uniform rates among the sites. The bootstrap of 500 replicates was used to estimate the variation in the model. |
sec | Results The TS and RS specimens for 78 Rousettus spp. bats were collected in VTM from seven States (Kerala, Karnataka, Chandigarh, Gujarat, Odisha, Punjab and Telangana). The TS and RS specimens of 508 Pteropus spp. bats were also collected in VTM from 10 States/UTs in India (Kerala, Karnataka, Chandigarh, Gujarat, Himachal Pradesh, Odisha, Puducherry, Punjab, Tamil Nadu and Telangana). During the trapping process, 12 (8 Rousettus and 4 Pteropus spp.) bats died. Organ specimens (intestine and kidney) were collected from these bats (TS and RS specimens of these 12 bats were included in the total number of samples). Detection of bat coronavirus using RdRp gene RT-PCR: Four of the 78 RS of Rousettus spp. bats screened for the BtCoV were found positive. All the positive RS samples belonged to Kerala State. Intestinal specimens of two bats were also found to be positive for the BtCoV. One bat (MCL-19-Bat-606), from Kerala, was tested positive in both the intestinal specimen and the RS. The second bat (MCL-20-Bat-76), from Karnataka, was tested positive only in the intestinal specimen. Altogether, five Rousettus spp. bats were positive for the BtCoV. All TS specimens from Rousettus spp. were found negative for BtCoV (Table I). Table I Bat coronavirus positivity in bat specimens screened using RNA-dependent RNA polymerase (RdRp) gene-specific reverse transcription-polymerase chain reaction (RT-PCR) in different States Place of collection Number of positive/number tested (%) for different bat species for BtCoV RdRp gene-specific RT-PCR Pteropus bats (%) Rousettus bats (%) Rectal swabs Throat swabs Rectal swabs Throat swabs Kerala 12/217 (5.53) 0/21 (0.00) 4/42 (9.52) 0/4 (0.00) Karnataka 0/78 (0.00) NT 0/4 (0.00) 0/4 (0.00) Chandigarh 0/27 (0.00) NT 0/6 (0.00) 0/6 (0.00) Gujarat 0/30 (0.00) NT 0/18 (0.00) 0/18 (0.00) Odisha 0/30 (0.00) NT 0/2 (0.00) 0/2 (0.00) Punjab 0/14 (0.00) NT 0/2 (0.00) 0/2 (0.00) Telangana 0/30 (0.00) NT 0/4 (0.00) 0/4 (0.00) Himachal Pradesh 2/29 (6.89) 0/6 (0.00) NA NA Puducherry 6/23 (26.09) 0/10 (0.00) NA NA Tamil Nadu 1/30 (3.33) 0/5 (0.00) NA NA 21/508 (4.13) 0/42 (0.00) 4/78 (5.13) 0/40 (0.00) NT, not tested; NA, not available; BtCoV, bat coronavirus Twenty one of the 508 RSs from Pteropus spp. bats screened were tested positive for the BtCoV (Table I). These positive bats belonged to Kerala (n=12), Himachal Pradesh (n=2), Puducherry (n=6) and Tamil Nadu (n=1). The TS specimens of the same bats were tested negative for BtCoV. The TS specimens of RS-negative (n=42) bats were also screened and found to be negative (Table I). A total of 25 bats from both the species were found positive. Sequencing of the positive coronavirus specimens Sanger sequencing of bat coronavirus: Using the Sanger sequencing protocol, partial RdRp sequences of BtCoV were retrieved from two (out of 4 amplicons) specimens of Rousettus spp. One of the sequences (MCL-19-bat-588/2) showed close identity to BtCoV HKU9-5-2 (AN): HM211099.1; sequence identity (SI): 99.2 per cent, whereas the second RdRp sequence (MCL-20-bat-76/10) had an SI of 98.8 per cent with BtCoV HKU9-1 (AN: EF065513.1), both from China. Sanger's sequencing protocol led to retrieval of eight partial RdRp sequences which belonged to Pteropus spp. These bats were collected from Kerala (n=5) and Tamil Nadu (n=3) States. One of the three partial RdRp sequences from Tamil Nadu had 97.93 per cent SI with BtCoV/B55951/Pte_lyl/CB2-THA (AN: MG256459.1, Thailand). The other two sequences had a minimum of 99.48 per cent SI with the CoV PREDICT_CoV-17/PB072 (AN: KX284942.1, Nepal). One of the five partial RdRp sequences from Kerala had 98.88 per cent SI with BtCoV/B55951/Pte_lyl/CB2-THA (AN: MG256459.1, Thailand). The remaining four partial RdRp sequences had >97 per cent SI with CoV PREDICT_CoV-17/PB072 (AN: KX284942.1, Nepal). Next-generation sequencing of bat coronavirus: NGS was performed on 10 specimens [4 RS, 2 kidney and 4 intestinal tissue) of the five Rousettus bats to retrieve the complete genome of the BtCoV. Kidney and intestine tissues of the bats from Karnataka State (MCL-20-Bat-76) and RS along with intestine tissue of bats from Kerala State (MCL-19-Bat-606) were used for sequencing and analysis. Two different viruses were retrieved based on the BLAST analysis of the sequences from the kidney and intestine tissues of the bats from Karnataka. Kidney specimen of MCL-20-Bat-76 had an SI of 94 per cent and query coverage (QC) of 94 per cent with CoV BtRt-BetaCoV/GX2018 (AN: MK211379.1), whereas the intestine tissue of the MCL-20-Bat-76 had an SI of 96.8 and 95 per cent QC with the BtCoV HKU9-1 (AN: EF065513.1). The sequences from RS and intestine tissue of the MCL-19-Bat-606 from Kerala, had 93.69 and 93.99 per cent SI to CoV BtRt-BetaCoV/GX2018 (AN: MK211379.1), respectively, with 100 per cent QC. Further, 99.8 per cent of the CoV BtRt-BetaCoV/GX2018 sequences were retrieved from the intestine specimen of the MCL-19-Bat-606. The details of the genome recovered reads mapped and the per cent of reads mapped are summarized in Table II. Table II Details of the genome recovered reads mapped and the per cent of reads mapped from the Rousettus bat samples Sample details Sample type Virus retrieved Relevant reads Per cent of reads Per cent of genome recovered MCL-20-Bat-76 Kidney Coronavirus BtRt-BetaCoV/GX2018 1632 0.015 94.39 Intestine BtCoV HKU9-1 4499 0.056 95.75 MCL-19-Bat-606 Rectal swab Coronavirus BtRt-BetaCoV/GX2018 13,973 0.114 99.53 Intestine Coronavirus BtRt-BetaCoV/GX2018 10,214,492 93.476 99.87 Phylogenetic analysis of partial and complete genome sequences of bat coronavirus: A neighbour-joining tree was generated using the partial RdRp region sequences derived from Pteropus and Rousettus spp. bat specimens. It was observed that all the BtCoV sequences were clustered within the L_D sequences of beta CoVs. A distinct subclustering of the sequences retrieved from Pteropus and Rousettus spp. bats is shown in Figure 1. The sequences in the light pink colour are retrieved from the Pteropus spp., whereas those in the dark pink region belong to Rousettus spp. The sequence divergence of 0.35 was observed between Pteropus spp. and Rousettus spp., which was obtained by averaging over all the sequence pairs between the two species, determining those to be distinct sequences to each species. Fig. 1 Neighbour-joining tree for the RNA-dependent RNA polymerase (RdRp) partial sequence (genomic location: 14,701-15,120) generated from Sanger sequencing. The tree was constructed using the RdRp sequence available in the GenBank sequences. Kimura 2-parameter model was used as the substitution model to generate the tree. A bootstrap replication of 1000 cycles was performed to generate the tree to assess the statistical robustness. The complete genome sequences of four BtCoV obtained from Rousettus spp. specimens were used for generating a neighbour-joining tree (Fig. 2). These sequences were also clustered within L_D of β-CoVs as observed for partial RdRp sequence tree. These complete genome sequences were grouped into gene pairs to identify the gene with higher and lower divergence. The complete genomes of the Indian BtCoV sequences were grouped under L_D. The evolutionary divergence of ORF 1b was <0.54 between the different β-CoV lineages with a maximum score of 0.7 between different BtCoV sequences used in this study (Table III). E gene sequences had larger divergence within the β-CoV genus ranging from 2.18 to 0.94. Lineages L_A and L_C had the maximum divergence of 2.18, whereas the L_B and L_C were the least (0.94). N gene has an overall higher divergence among different lineages (ranging: 2.08-0.75). Overall, evolutionary divergence for the sequences of each gene pair demonstrated that S, N, E and M genes from the α- and δ-CoV highly diverged across the different genus. In contrast, the ORF 1b was less divergent across the genera (Table III). Fig. 2 Phylogenetic tree for the complete genome tree: A neighbour-joining tree was generated using the representative complete genome sequence available in the GenBank sequences. Kimura 2-parameter model was used as the substitution model to generate the tree. A bootstrap replication of 1000 cycles was performed to generate the tree to assess the statistical robustness. Table III Evolutionary divergence for ORF 1b, S, N and M genes for the retrieved sequences with other reference sequences. The lower right-check hand matrix of the table depicts the divergence and the upper left-check matrix of the matrix (blue colour) depicts the variation observed in the bootstrap replication N gene Alpha Delta Gamma L_A L_B L_C L_D M gene Alpha Delta Gamma L_A L_B L_C L_D Alpha 0.15 0.09 0.10 0.08 0.08 0.09 Alpha 0.11 0.12 0.05 0.06 0.05 0.06 Delta 2.08 0.11 0.16 0.09 0.11 0.10 Delta 1.50 0.26 0.08 0.16 0.10 0.11 Gamma 1.57 1.49 0.08 0.08 0.09 0.08 Gamma 1.53 1.84 0.10 0.12 0.11 0.09 L_A 1.84 1.73 1.37 0.05 0.05 0.06 L_A 0.92 1.24 1.30 0.06 0.05 0.05 L_B 1.48 1.37 1.32 1.09 0.03 0.04 L_B 1.05 1.51 1.37 0.92 0.05 0.05 L_C 1.57 1.52 1.42 1.07 0.75 0.04 L_C 0.99 1.35 1.27 0.80 0.82 0.05 L_D 1.64 1.46 1.36 1.27 0.90 0.97 L_D 0.99 1.42 1.23 0.84 0.79 0.82 ORF 1b Alpha Delta Gamma L_A L_B L_C L_D ORF 1a Alpha Delta Gamma L_A L_B L_C L_D Alpha 0.01 0.01 0.01 0.01 0.01 0.01 Alpha 0.02 0.02 0.01 0.02 0.02 0.03 Delta 0.70 0.01 0.02 0.01 0.01 0.01 Delta 1.32 0.03 0.02 0.03 0.03 0.04 Gamma 0.62 0.67 0.01 0.01 0.01 0.01 Gamma 1.14 1.33 0.02 0.03 0.02 0.04 L_A 0.61 0.69 0.60 0.01 0.01 0.01 L_A 1.22 1.01 1.30 0.02 0.02 0.04 L_B 0.60 0.70 0.65 0.54 0.01 0.01 L_B 1.26 1.42 1.41 1.19 0.01 0.02 L_C 0.58 0.69 0.62 0.53 0.50 0.01 L_C 1.35 1.41 1.44 1.19 0.97 0.03 L_D 0.60 0.67 0.61 0.53 0.50 0.52 L_D 1.26 1.27 1.39 1.09 0.90 1.03 S gene Alpha Delta Gamma L_A L_B L_C L_D E gene Alpha Delta Gamma L_A L_B L_C L_D Alpha 0.02 0.02 0.03 0.03 0.03 0.02 Alpha 0.12 0.18 0.09 0.15 0.15 0.12 Delta 0.86 0.03 0.04 0.04 0.06 0.03 Delta 1.14 0.47 0.22 0.41 0.28 0.17 Gamma 1.14 0.96 0.04 0.05 0.06 0.04 Gamma 1.59 1.64 0.22 0.24 0.32 0.19 L_A 1.36 1.28 1.43 0.03 0.03 0.02 L_A 1.03 1.58 1.57 0.23 0.21 0.25 L_B 1.33 1.23 1.34 1.19 0.04 0.02 L_B 1.24 1.75 1.40 1.83 0.11 0.14 L_C 1.42 1.32 1.46 1.17 1.03 0.03 L_C 1.37 1.64 1.83 2.18 0.94 0.17 L_D 1.34 1.24 1.41 1.16 1.00 1.11 L_D 1.25 1.42 1.52 1.95 1.16 1.37 ORF 1a, open reading frame 1a polyprotein; ORF 1b, ORF 1b polyprotein; S, spike glycoprotein; N, nuclocapsid phospoptotein; M, membrane glycoprotein; E, envelope protein |
title | Results |
p | The TS and RS specimens for 78 Rousettus spp. bats were collected in VTM from seven States (Kerala, Karnataka, Chandigarh, Gujarat, Odisha, Punjab and Telangana). The TS and RS specimens of 508 Pteropus spp. bats were also collected in VTM from 10 States/UTs in India (Kerala, Karnataka, Chandigarh, Gujarat, Himachal Pradesh, Odisha, Puducherry, Punjab, Tamil Nadu and Telangana). During the trapping process, 12 (8 Rousettus and 4 Pteropus spp.) bats died. Organ specimens (intestine and kidney) were collected from these bats (TS and RS specimens of these 12 bats were included in the total number of samples). |
p | Detection of bat coronavirus using RdRp gene RT-PCR: Four of the 78 RS of Rousettus spp. bats screened for the BtCoV were found positive. All the positive RS samples belonged to Kerala State. Intestinal specimens of two bats were also found to be positive for the BtCoV. One bat (MCL-19-Bat-606), from Kerala, was tested positive in both the intestinal specimen and the RS. The second bat (MCL-20-Bat-76), from Karnataka, was tested positive only in the intestinal specimen. Altogether, five Rousettus spp. bats were positive for the BtCoV. All TS specimens from Rousettus spp. were found negative for BtCoV (Table I). |
table-wrap | Table I Bat coronavirus positivity in bat specimens screened using RNA-dependent RNA polymerase (RdRp) gene-specific reverse transcription-polymerase chain reaction (RT-PCR) in different States Place of collection Number of positive/number tested (%) for different bat species for BtCoV RdRp gene-specific RT-PCR Pteropus bats (%) Rousettus bats (%) Rectal swabs Throat swabs Rectal swabs Throat swabs Kerala 12/217 (5.53) 0/21 (0.00) 4/42 (9.52) 0/4 (0.00) Karnataka 0/78 (0.00) NT 0/4 (0.00) 0/4 (0.00) Chandigarh 0/27 (0.00) NT 0/6 (0.00) 0/6 (0.00) Gujarat 0/30 (0.00) NT 0/18 (0.00) 0/18 (0.00) Odisha 0/30 (0.00) NT 0/2 (0.00) 0/2 (0.00) Punjab 0/14 (0.00) NT 0/2 (0.00) 0/2 (0.00) Telangana 0/30 (0.00) NT 0/4 (0.00) 0/4 (0.00) Himachal Pradesh 2/29 (6.89) 0/6 (0.00) NA NA Puducherry 6/23 (26.09) 0/10 (0.00) NA NA Tamil Nadu 1/30 (3.33) 0/5 (0.00) NA NA 21/508 (4.13) 0/42 (0.00) 4/78 (5.13) 0/40 (0.00) NT, not tested; NA, not available; BtCoV, bat coronavirus |
label | Table I |
caption | Bat coronavirus positivity in bat specimens screened using RNA-dependent RNA polymerase (RdRp) gene-specific reverse transcription-polymerase chain reaction (RT-PCR) in different States |
p | Bat coronavirus positivity in bat specimens screened using RNA-dependent RNA polymerase (RdRp) gene-specific reverse transcription-polymerase chain reaction (RT-PCR) in different States |
table | Place of collection Number of positive/number tested (%) for different bat species for BtCoV RdRp gene-specific RT-PCR Pteropus bats (%) Rousettus bats (%) Rectal swabs Throat swabs Rectal swabs Throat swabs Kerala 12/217 (5.53) 0/21 (0.00) 4/42 (9.52) 0/4 (0.00) Karnataka 0/78 (0.00) NT 0/4 (0.00) 0/4 (0.00) Chandigarh 0/27 (0.00) NT 0/6 (0.00) 0/6 (0.00) Gujarat 0/30 (0.00) NT 0/18 (0.00) 0/18 (0.00) Odisha 0/30 (0.00) NT 0/2 (0.00) 0/2 (0.00) Punjab 0/14 (0.00) NT 0/2 (0.00) 0/2 (0.00) Telangana 0/30 (0.00) NT 0/4 (0.00) 0/4 (0.00) Himachal Pradesh 2/29 (6.89) 0/6 (0.00) NA NA Puducherry 6/23 (26.09) 0/10 (0.00) NA NA Tamil Nadu 1/30 (3.33) 0/5 (0.00) NA NA 21/508 (4.13) 0/42 (0.00) 4/78 (5.13) 0/40 (0.00) |
tr | Place of collection Number of positive/number tested (%) for different bat species for BtCoV RdRp gene-specific RT-PCR |
th | Place of collection |
th | Number of positive/number tested (%) for different bat species for BtCoV RdRp gene-specific RT-PCR |
tr | Pteropus bats (%) Rousettus bats (%) |
th | Pteropus bats (%) |
th | Rousettus bats (%) |
tr | Rectal swabs Throat swabs Rectal swabs Throat swabs |
th | Rectal swabs |
th | Throat swabs |
th | Rectal swabs |
th | Throat swabs |
tr | Kerala 12/217 (5.53) 0/21 (0.00) 4/42 (9.52) 0/4 (0.00) |
td | Kerala |
td | 12/217 (5.53) |
td | 0/21 (0.00) |
td | 4/42 (9.52) |
td | 0/4 (0.00) |
tr | Karnataka 0/78 (0.00) NT 0/4 (0.00) 0/4 (0.00) |
td | Karnataka |
td | 0/78 (0.00) |
td | NT |
td | 0/4 (0.00) |
td | 0/4 (0.00) |
tr | Chandigarh 0/27 (0.00) NT 0/6 (0.00) 0/6 (0.00) |
td | Chandigarh |
td | 0/27 (0.00) |
td | NT |
td | 0/6 (0.00) |
td | 0/6 (0.00) |
tr | Gujarat 0/30 (0.00) NT 0/18 (0.00) 0/18 (0.00) |
td | Gujarat |
td | 0/30 (0.00) |
td | NT |
td | 0/18 (0.00) |
td | 0/18 (0.00) |
tr | Odisha 0/30 (0.00) NT 0/2 (0.00) 0/2 (0.00) |
td | Odisha |
td | 0/30 (0.00) |
td | NT |
td | 0/2 (0.00) |
td | 0/2 (0.00) |
tr | Punjab 0/14 (0.00) NT 0/2 (0.00) 0/2 (0.00) |
td | Punjab |
td | 0/14 (0.00) |
td | NT |
td | 0/2 (0.00) |
td | 0/2 (0.00) |
tr | Telangana 0/30 (0.00) NT 0/4 (0.00) 0/4 (0.00) |
td | Telangana |
td | 0/30 (0.00) |
td | NT |
td | 0/4 (0.00) |
td | 0/4 (0.00) |
tr | Himachal Pradesh 2/29 (6.89) 0/6 (0.00) NA NA |
td | Himachal Pradesh |
td | 2/29 (6.89) |
td | 0/6 (0.00) |
td | NA |
td | NA |
tr | Puducherry 6/23 (26.09) 0/10 (0.00) NA NA |
td | Puducherry |
td | 6/23 (26.09) |
td | 0/10 (0.00) |
td | NA |
td | NA |
tr | Tamil Nadu 1/30 (3.33) 0/5 (0.00) NA NA |
td | Tamil Nadu |
td | 1/30 (3.33) |
td | 0/5 (0.00) |
td | NA |
td | NA |
tr | 21/508 (4.13) 0/42 (0.00) 4/78 (5.13) 0/40 (0.00) |
td | 21/508 (4.13) |
td | 0/42 (0.00) |
td | 4/78 (5.13) |
td | 0/40 (0.00) |
table-wrap-foot | NT, not tested; NA, not available; BtCoV, bat coronavirus |
footnote | NT, not tested; NA, not available; BtCoV, bat coronavirus |
p | NT, not tested; NA, not available; BtCoV, bat coronavirus |
p | Twenty one of the 508 RSs from Pteropus spp. bats screened were tested positive for the BtCoV (Table I). These positive bats belonged to Kerala (n=12), Himachal Pradesh (n=2), Puducherry (n=6) and Tamil Nadu (n=1). The TS specimens of the same bats were tested negative for BtCoV. The TS specimens of RS-negative (n=42) bats were also screened and found to be negative (Table I). A total of 25 bats from both the species were found positive. |
sec | Sequencing of the positive coronavirus specimens Sanger sequencing of bat coronavirus: Using the Sanger sequencing protocol, partial RdRp sequences of BtCoV were retrieved from two (out of 4 amplicons) specimens of Rousettus spp. One of the sequences (MCL-19-bat-588/2) showed close identity to BtCoV HKU9-5-2 (AN): HM211099.1; sequence identity (SI): 99.2 per cent, whereas the second RdRp sequence (MCL-20-bat-76/10) had an SI of 98.8 per cent with BtCoV HKU9-1 (AN: EF065513.1), both from China. Sanger's sequencing protocol led to retrieval of eight partial RdRp sequences which belonged to Pteropus spp. These bats were collected from Kerala (n=5) and Tamil Nadu (n=3) States. One of the three partial RdRp sequences from Tamil Nadu had 97.93 per cent SI with BtCoV/B55951/Pte_lyl/CB2-THA (AN: MG256459.1, Thailand). The other two sequences had a minimum of 99.48 per cent SI with the CoV PREDICT_CoV-17/PB072 (AN: KX284942.1, Nepal). One of the five partial RdRp sequences from Kerala had 98.88 per cent SI with BtCoV/B55951/Pte_lyl/CB2-THA (AN: MG256459.1, Thailand). The remaining four partial RdRp sequences had >97 per cent SI with CoV PREDICT_CoV-17/PB072 (AN: KX284942.1, Nepal). Next-generation sequencing of bat coronavirus: NGS was performed on 10 specimens [4 RS, 2 kidney and 4 intestinal tissue) of the five Rousettus bats to retrieve the complete genome of the BtCoV. Kidney and intestine tissues of the bats from Karnataka State (MCL-20-Bat-76) and RS along with intestine tissue of bats from Kerala State (MCL-19-Bat-606) were used for sequencing and analysis. Two different viruses were retrieved based on the BLAST analysis of the sequences from the kidney and intestine tissues of the bats from Karnataka. Kidney specimen of MCL-20-Bat-76 had an SI of 94 per cent and query coverage (QC) of 94 per cent with CoV BtRt-BetaCoV/GX2018 (AN: MK211379.1), whereas the intestine tissue of the MCL-20-Bat-76 had an SI of 96.8 and 95 per cent QC with the BtCoV HKU9-1 (AN: EF065513.1). The sequences from RS and intestine tissue of the MCL-19-Bat-606 from Kerala, had 93.69 and 93.99 per cent SI to CoV BtRt-BetaCoV/GX2018 (AN: MK211379.1), respectively, with 100 per cent QC. Further, 99.8 per cent of the CoV BtRt-BetaCoV/GX2018 sequences were retrieved from the intestine specimen of the MCL-19-Bat-606. The details of the genome recovered reads mapped and the per cent of reads mapped are summarized in Table II. Table II Details of the genome recovered reads mapped and the per cent of reads mapped from the Rousettus bat samples Sample details Sample type Virus retrieved Relevant reads Per cent of reads Per cent of genome recovered MCL-20-Bat-76 Kidney Coronavirus BtRt-BetaCoV/GX2018 1632 0.015 94.39 Intestine BtCoV HKU9-1 4499 0.056 95.75 MCL-19-Bat-606 Rectal swab Coronavirus BtRt-BetaCoV/GX2018 13,973 0.114 99.53 Intestine Coronavirus BtRt-BetaCoV/GX2018 10,214,492 93.476 99.87 Phylogenetic analysis of partial and complete genome sequences of bat coronavirus: A neighbour-joining tree was generated using the partial RdRp region sequences derived from Pteropus and Rousettus spp. bat specimens. It was observed that all the BtCoV sequences were clustered within the L_D sequences of beta CoVs. A distinct subclustering of the sequences retrieved from Pteropus and Rousettus spp. bats is shown in Figure 1. The sequences in the light pink colour are retrieved from the Pteropus spp., whereas those in the dark pink region belong to Rousettus spp. The sequence divergence of 0.35 was observed between Pteropus spp. and Rousettus spp., which was obtained by averaging over all the sequence pairs between the two species, determining those to be distinct sequences to each species. Fig. 1 Neighbour-joining tree for the RNA-dependent RNA polymerase (RdRp) partial sequence (genomic location: 14,701-15,120) generated from Sanger sequencing. The tree was constructed using the RdRp sequence available in the GenBank sequences. Kimura 2-parameter model was used as the substitution model to generate the tree. A bootstrap replication of 1000 cycles was performed to generate the tree to assess the statistical robustness. The complete genome sequences of four BtCoV obtained from Rousettus spp. specimens were used for generating a neighbour-joining tree (Fig. 2). These sequences were also clustered within L_D of β-CoVs as observed for partial RdRp sequence tree. These complete genome sequences were grouped into gene pairs to identify the gene with higher and lower divergence. The complete genomes of the Indian BtCoV sequences were grouped under L_D. The evolutionary divergence of ORF 1b was <0.54 between the different β-CoV lineages with a maximum score of 0.7 between different BtCoV sequences used in this study (Table III). E gene sequences had larger divergence within the β-CoV genus ranging from 2.18 to 0.94. Lineages L_A and L_C had the maximum divergence of 2.18, whereas the L_B and L_C were the least (0.94). N gene has an overall higher divergence among different lineages (ranging: 2.08-0.75). Overall, evolutionary divergence for the sequences of each gene pair demonstrated that S, N, E and M genes from the α- and δ-CoV highly diverged across the different genus. In contrast, the ORF 1b was less divergent across the genera (Table III). Fig. 2 Phylogenetic tree for the complete genome tree: A neighbour-joining tree was generated using the representative complete genome sequence available in the GenBank sequences. Kimura 2-parameter model was used as the substitution model to generate the tree. A bootstrap replication of 1000 cycles was performed to generate the tree to assess the statistical robustness. Table III Evolutionary divergence for ORF 1b, S, N and M genes for the retrieved sequences with other reference sequences. The lower right-check hand matrix of the table depicts the divergence and the upper left-check matrix of the matrix (blue colour) depicts the variation observed in the bootstrap replication N gene Alpha Delta Gamma L_A L_B L_C L_D M gene Alpha Delta Gamma L_A L_B L_C L_D Alpha 0.15 0.09 0.10 0.08 0.08 0.09 Alpha 0.11 0.12 0.05 0.06 0.05 0.06 Delta 2.08 0.11 0.16 0.09 0.11 0.10 Delta 1.50 0.26 0.08 0.16 0.10 0.11 Gamma 1.57 1.49 0.08 0.08 0.09 0.08 Gamma 1.53 1.84 0.10 0.12 0.11 0.09 L_A 1.84 1.73 1.37 0.05 0.05 0.06 L_A 0.92 1.24 1.30 0.06 0.05 0.05 L_B 1.48 1.37 1.32 1.09 0.03 0.04 L_B 1.05 1.51 1.37 0.92 0.05 0.05 L_C 1.57 1.52 1.42 1.07 0.75 0.04 L_C 0.99 1.35 1.27 0.80 0.82 0.05 L_D 1.64 1.46 1.36 1.27 0.90 0.97 L_D 0.99 1.42 1.23 0.84 0.79 0.82 ORF 1b Alpha Delta Gamma L_A L_B L_C L_D ORF 1a Alpha Delta Gamma L_A L_B L_C L_D Alpha 0.01 0.01 0.01 0.01 0.01 0.01 Alpha 0.02 0.02 0.01 0.02 0.02 0.03 Delta 0.70 0.01 0.02 0.01 0.01 0.01 Delta 1.32 0.03 0.02 0.03 0.03 0.04 Gamma 0.62 0.67 0.01 0.01 0.01 0.01 Gamma 1.14 1.33 0.02 0.03 0.02 0.04 L_A 0.61 0.69 0.60 0.01 0.01 0.01 L_A 1.22 1.01 1.30 0.02 0.02 0.04 L_B 0.60 0.70 0.65 0.54 0.01 0.01 L_B 1.26 1.42 1.41 1.19 0.01 0.02 L_C 0.58 0.69 0.62 0.53 0.50 0.01 L_C 1.35 1.41 1.44 1.19 0.97 0.03 L_D 0.60 0.67 0.61 0.53 0.50 0.52 L_D 1.26 1.27 1.39 1.09 0.90 1.03 S gene Alpha Delta Gamma L_A L_B L_C L_D E gene Alpha Delta Gamma L_A L_B L_C L_D Alpha 0.02 0.02 0.03 0.03 0.03 0.02 Alpha 0.12 0.18 0.09 0.15 0.15 0.12 Delta 0.86 0.03 0.04 0.04 0.06 0.03 Delta 1.14 0.47 0.22 0.41 0.28 0.17 Gamma 1.14 0.96 0.04 0.05 0.06 0.04 Gamma 1.59 1.64 0.22 0.24 0.32 0.19 L_A 1.36 1.28 1.43 0.03 0.03 0.02 L_A 1.03 1.58 1.57 0.23 0.21 0.25 L_B 1.33 1.23 1.34 1.19 0.04 0.02 L_B 1.24 1.75 1.40 1.83 0.11 0.14 L_C 1.42 1.32 1.46 1.17 1.03 0.03 L_C 1.37 1.64 1.83 2.18 0.94 0.17 L_D 1.34 1.24 1.41 1.16 1.00 1.11 L_D 1.25 1.42 1.52 1.95 1.16 1.37 ORF 1a, open reading frame 1a polyprotein; ORF 1b, ORF 1b polyprotein; S, spike glycoprotein; N, nuclocapsid phospoptotein; M, membrane glycoprotein; E, envelope protein |
sec | Sequencing of the positive coronavirus specimens Sanger sequencing of bat coronavirus: Using the Sanger sequencing protocol, partial RdRp sequences of BtCoV were retrieved from two (out of 4 amplicons) specimens of Rousettus spp. One of the sequences (MCL-19-bat-588/2) showed close identity to BtCoV HKU9-5-2 (AN): HM211099.1; sequence identity (SI): 99.2 per cent, whereas the second RdRp sequence (MCL-20-bat-76/10) had an SI of 98.8 per cent with BtCoV HKU9-1 (AN: EF065513.1), both from China. Sanger's sequencing protocol led to retrieval of eight partial RdRp sequences which belonged to Pteropus spp. These bats were collected from Kerala (n=5) and Tamil Nadu (n=3) States. One of the three partial RdRp sequences from Tamil Nadu had 97.93 per cent SI with BtCoV/B55951/Pte_lyl/CB2-THA (AN: MG256459.1, Thailand). The other two sequences had a minimum of 99.48 per cent SI with the CoV PREDICT_CoV-17/PB072 (AN: KX284942.1, Nepal). One of the five partial RdRp sequences from Kerala had 98.88 per cent SI with BtCoV/B55951/Pte_lyl/CB2-THA (AN: MG256459.1, Thailand). The remaining four partial RdRp sequences had >97 per cent SI with CoV PREDICT_CoV-17/PB072 (AN: KX284942.1, Nepal). Next-generation sequencing of bat coronavirus: NGS was performed on 10 specimens [4 RS, 2 kidney and 4 intestinal tissue) of the five Rousettus bats to retrieve the complete genome of the BtCoV. Kidney and intestine tissues of the bats from Karnataka State (MCL-20-Bat-76) and RS along with intestine tissue of bats from Kerala State (MCL-19-Bat-606) were used for sequencing and analysis. Two different viruses were retrieved based on the BLAST analysis of the sequences from the kidney and intestine tissues of the bats from Karnataka. Kidney specimen of MCL-20-Bat-76 had an SI of 94 per cent and query coverage (QC) of 94 per cent with CoV BtRt-BetaCoV/GX2018 (AN: MK211379.1), whereas the intestine tissue of the MCL-20-Bat-76 had an SI of 96.8 and 95 per cent QC with the BtCoV HKU9-1 (AN: EF065513.1). The sequences from RS and intestine tissue of the MCL-19-Bat-606 from Kerala, had 93.69 and 93.99 per cent SI to CoV BtRt-BetaCoV/GX2018 (AN: MK211379.1), respectively, with 100 per cent QC. Further, 99.8 per cent of the CoV BtRt-BetaCoV/GX2018 sequences were retrieved from the intestine specimen of the MCL-19-Bat-606. The details of the genome recovered reads mapped and the per cent of reads mapped are summarized in Table II. Table II Details of the genome recovered reads mapped and the per cent of reads mapped from the Rousettus bat samples Sample details Sample type Virus retrieved Relevant reads Per cent of reads Per cent of genome recovered MCL-20-Bat-76 Kidney Coronavirus BtRt-BetaCoV/GX2018 1632 0.015 94.39 Intestine BtCoV HKU9-1 4499 0.056 95.75 MCL-19-Bat-606 Rectal swab Coronavirus BtRt-BetaCoV/GX2018 13,973 0.114 99.53 Intestine Coronavirus BtRt-BetaCoV/GX2018 10,214,492 93.476 99.87 Phylogenetic analysis of partial and complete genome sequences of bat coronavirus: A neighbour-joining tree was generated using the partial RdRp region sequences derived from Pteropus and Rousettus spp. bat specimens. It was observed that all the BtCoV sequences were clustered within the L_D sequences of beta CoVs. A distinct subclustering of the sequences retrieved from Pteropus and Rousettus spp. bats is shown in Figure 1. The sequences in the light pink colour are retrieved from the Pteropus spp., whereas those in the dark pink region belong to Rousettus spp. The sequence divergence of 0.35 was observed between Pteropus spp. and Rousettus spp., which was obtained by averaging over all the sequence pairs between the two species, determining those to be distinct sequences to each species. Fig. 1 Neighbour-joining tree for the RNA-dependent RNA polymerase (RdRp) partial sequence (genomic location: 14,701-15,120) generated from Sanger sequencing. The tree was constructed using the RdRp sequence available in the GenBank sequences. Kimura 2-parameter model was used as the substitution model to generate the tree. A bootstrap replication of 1000 cycles was performed to generate the tree to assess the statistical robustness. The complete genome sequences of four BtCoV obtained from Rousettus spp. specimens were used for generating a neighbour-joining tree (Fig. 2). These sequences were also clustered within L_D of β-CoVs as observed for partial RdRp sequence tree. These complete genome sequences were grouped into gene pairs to identify the gene with higher and lower divergence. The complete genomes of the Indian BtCoV sequences were grouped under L_D. The evolutionary divergence of ORF 1b was <0.54 between the different β-CoV lineages with a maximum score of 0.7 between different BtCoV sequences used in this study (Table III). E gene sequences had larger divergence within the β-CoV genus ranging from 2.18 to 0.94. Lineages L_A and L_C had the maximum divergence of 2.18, whereas the L_B and L_C were the least (0.94). N gene has an overall higher divergence among different lineages (ranging: 2.08-0.75). Overall, evolutionary divergence for the sequences of each gene pair demonstrated that S, N, E and M genes from the α- and δ-CoV highly diverged across the different genus. In contrast, the ORF 1b was less divergent across the genera (Table III). Fig. 2 Phylogenetic tree for the complete genome tree: A neighbour-joining tree was generated using the representative complete genome sequence available in the GenBank sequences. Kimura 2-parameter model was used as the substitution model to generate the tree. A bootstrap replication of 1000 cycles was performed to generate the tree to assess the statistical robustness. Table III Evolutionary divergence for ORF 1b, S, N and M genes for the retrieved sequences with other reference sequences. The lower right-check hand matrix of the table depicts the divergence and the upper left-check matrix of the matrix (blue colour) depicts the variation observed in the bootstrap replication N gene Alpha Delta Gamma L_A L_B L_C L_D M gene Alpha Delta Gamma L_A L_B L_C L_D Alpha 0.15 0.09 0.10 0.08 0.08 0.09 Alpha 0.11 0.12 0.05 0.06 0.05 0.06 Delta 2.08 0.11 0.16 0.09 0.11 0.10 Delta 1.50 0.26 0.08 0.16 0.10 0.11 Gamma 1.57 1.49 0.08 0.08 0.09 0.08 Gamma 1.53 1.84 0.10 0.12 0.11 0.09 L_A 1.84 1.73 1.37 0.05 0.05 0.06 L_A 0.92 1.24 1.30 0.06 0.05 0.05 L_B 1.48 1.37 1.32 1.09 0.03 0.04 L_B 1.05 1.51 1.37 0.92 0.05 0.05 L_C 1.57 1.52 1.42 1.07 0.75 0.04 L_C 0.99 1.35 1.27 0.80 0.82 0.05 L_D 1.64 1.46 1.36 1.27 0.90 0.97 L_D 0.99 1.42 1.23 0.84 0.79 0.82 ORF 1b Alpha Delta Gamma L_A L_B L_C L_D ORF 1a Alpha Delta Gamma L_A L_B L_C L_D Alpha 0.01 0.01 0.01 0.01 0.01 0.01 Alpha 0.02 0.02 0.01 0.02 0.02 0.03 Delta 0.70 0.01 0.02 0.01 0.01 0.01 Delta 1.32 0.03 0.02 0.03 0.03 0.04 Gamma 0.62 0.67 0.01 0.01 0.01 0.01 Gamma 1.14 1.33 0.02 0.03 0.02 0.04 L_A 0.61 0.69 0.60 0.01 0.01 0.01 L_A 1.22 1.01 1.30 0.02 0.02 0.04 L_B 0.60 0.70 0.65 0.54 0.01 0.01 L_B 1.26 1.42 1.41 1.19 0.01 0.02 L_C 0.58 0.69 0.62 0.53 0.50 0.01 L_C 1.35 1.41 1.44 1.19 0.97 0.03 L_D 0.60 0.67 0.61 0.53 0.50 0.52 L_D 1.26 1.27 1.39 1.09 0.90 1.03 S gene Alpha Delta Gamma L_A L_B L_C L_D E gene Alpha Delta Gamma L_A L_B L_C L_D Alpha 0.02 0.02 0.03 0.03 0.03 0.02 Alpha 0.12 0.18 0.09 0.15 0.15 0.12 Delta 0.86 0.03 0.04 0.04 0.06 0.03 Delta 1.14 0.47 0.22 0.41 0.28 0.17 Gamma 1.14 0.96 0.04 0.05 0.06 0.04 Gamma 1.59 1.64 0.22 0.24 0.32 0.19 L_A 1.36 1.28 1.43 0.03 0.03 0.02 L_A 1.03 1.58 1.57 0.23 0.21 0.25 L_B 1.33 1.23 1.34 1.19 0.04 0.02 L_B 1.24 1.75 1.40 1.83 0.11 0.14 L_C 1.42 1.32 1.46 1.17 1.03 0.03 L_C 1.37 1.64 1.83 2.18 0.94 0.17 L_D 1.34 1.24 1.41 1.16 1.00 1.11 L_D 1.25 1.42 1.52 1.95 1.16 1.37 ORF 1a, open reading frame 1a polyprotein; ORF 1b, ORF 1b polyprotein; S, spike glycoprotein; N, nuclocapsid phospoptotein; M, membrane glycoprotein; E, envelope protein |
title | Sequencing of the positive coronavirus specimens |
p | Sanger sequencing of bat coronavirus: Using the Sanger sequencing protocol, partial RdRp sequences of BtCoV were retrieved from two (out of 4 amplicons) specimens of Rousettus spp. One of the sequences (MCL-19-bat-588/2) showed close identity to BtCoV HKU9-5-2 (AN): HM211099.1; sequence identity (SI): 99.2 per cent, whereas the second RdRp sequence (MCL-20-bat-76/10) had an SI of 98.8 per cent with BtCoV HKU9-1 (AN: EF065513.1), both from China. |
p | Sanger's sequencing protocol led to retrieval of eight partial RdRp sequences which belonged to Pteropus spp. These bats were collected from Kerala (n=5) and Tamil Nadu (n=3) States. One of the three partial RdRp sequences from Tamil Nadu had 97.93 per cent SI with BtCoV/B55951/Pte_lyl/CB2-THA (AN: MG256459.1, Thailand). The other two sequences had a minimum of 99.48 per cent SI with the CoV PREDICT_CoV-17/PB072 (AN: KX284942.1, Nepal). One of the five partial RdRp sequences from Kerala had 98.88 per cent SI with BtCoV/B55951/Pte_lyl/CB2-THA (AN: MG256459.1, Thailand). The remaining four partial RdRp sequences had >97 per cent SI with CoV PREDICT_CoV-17/PB072 (AN: KX284942.1, Nepal). |
p | Next-generation sequencing of bat coronavirus: NGS was performed on 10 specimens [4 RS, 2 kidney and 4 intestinal tissue) of the five Rousettus bats to retrieve the complete genome of the BtCoV. Kidney and intestine tissues of the bats from Karnataka State (MCL-20-Bat-76) and RS along with intestine tissue of bats from Kerala State (MCL-19-Bat-606) were used for sequencing and analysis. |
p | Two different viruses were retrieved based on the BLAST analysis of the sequences from the kidney and intestine tissues of the bats from Karnataka. Kidney specimen of MCL-20-Bat-76 had an SI of 94 per cent and query coverage (QC) of 94 per cent with CoV BtRt-BetaCoV/GX2018 (AN: MK211379.1), whereas the intestine tissue of the MCL-20-Bat-76 had an SI of 96.8 and 95 per cent QC with the BtCoV HKU9-1 (AN: EF065513.1). The sequences from RS and intestine tissue of the MCL-19-Bat-606 from Kerala, had 93.69 and 93.99 per cent SI to CoV BtRt-BetaCoV/GX2018 (AN: MK211379.1), respectively, with 100 per cent QC. Further, 99.8 per cent of the CoV BtRt-BetaCoV/GX2018 sequences were retrieved from the intestine specimen of the MCL-19-Bat-606. The details of the genome recovered reads mapped and the per cent of reads mapped are summarized in Table II. |
table-wrap | Table II Details of the genome recovered reads mapped and the per cent of reads mapped from the Rousettus bat samples Sample details Sample type Virus retrieved Relevant reads Per cent of reads Per cent of genome recovered MCL-20-Bat-76 Kidney Coronavirus BtRt-BetaCoV/GX2018 1632 0.015 94.39 Intestine BtCoV HKU9-1 4499 0.056 95.75 MCL-19-Bat-606 Rectal swab Coronavirus BtRt-BetaCoV/GX2018 13,973 0.114 99.53 Intestine Coronavirus BtRt-BetaCoV/GX2018 10,214,492 93.476 99.87 |
label | Table II |
caption | Details of the genome recovered reads mapped and the per cent of reads mapped from the Rousettus bat samples |
p | Details of the genome recovered reads mapped and the per cent of reads mapped from the Rousettus bat samples |
table | Sample details Sample type Virus retrieved Relevant reads Per cent of reads Per cent of genome recovered MCL-20-Bat-76 Kidney Coronavirus BtRt-BetaCoV/GX2018 1632 0.015 94.39 Intestine BtCoV HKU9-1 4499 0.056 95.75 MCL-19-Bat-606 Rectal swab Coronavirus BtRt-BetaCoV/GX2018 13,973 0.114 99.53 Intestine Coronavirus BtRt-BetaCoV/GX2018 10,214,492 93.476 99.87 |
tr | Sample details Sample type Virus retrieved Relevant reads Per cent of reads Per cent of genome recovered |
th | Sample details |
th | Sample type |
th | Virus retrieved |
th | Relevant reads |
th | Per cent of reads |
th | Per cent of genome recovered |
tr | MCL-20-Bat-76 Kidney Coronavirus BtRt-BetaCoV/GX2018 1632 0.015 94.39 |
td | MCL-20-Bat-76 |
td | Kidney |
td | Coronavirus BtRt-BetaCoV/GX2018 |
td | 1632 |
td | 0.015 |
td | 94.39 |
tr | Intestine BtCoV HKU9-1 4499 0.056 95.75 |
td | Intestine |
td | BtCoV HKU9-1 |
td | 4499 |
td | 0.056 |
td | 95.75 |
tr | MCL-19-Bat-606 Rectal swab Coronavirus BtRt-BetaCoV/GX2018 13,973 0.114 99.53 |
td | MCL-19-Bat-606 |
td | Rectal swab |
td | Coronavirus BtRt-BetaCoV/GX2018 |
td | 13,973 |
td | 0.114 |
td | 99.53 |
tr | Intestine Coronavirus BtRt-BetaCoV/GX2018 10,214,492 93.476 99.87 |
td | Intestine |
td | Coronavirus BtRt-BetaCoV/GX2018 |
td | 10,214,492 |
td | 93.476 |
td | 99.87 |
p | Phylogenetic analysis of partial and complete genome sequences of bat coronavirus: A neighbour-joining tree was generated using the partial RdRp region sequences derived from Pteropus and Rousettus spp. bat specimens. It was observed that all the BtCoV sequences were clustered within the L_D sequences of beta CoVs. A distinct subclustering of the sequences retrieved from Pteropus and Rousettus spp. bats is shown in Figure 1. The sequences in the light pink colour are retrieved from the Pteropus spp., whereas those in the dark pink region belong to Rousettus spp. The sequence divergence of 0.35 was observed between Pteropus spp. and Rousettus spp., which was obtained by averaging over all the sequence pairs between the two species, determining those to be distinct sequences to each species. |
figure | Fig. 1 Neighbour-joining tree for the RNA-dependent RNA polymerase (RdRp) partial sequence (genomic location: 14,701-15,120) generated from Sanger sequencing. The tree was constructed using the RdRp sequence available in the GenBank sequences. Kimura 2-parameter model was used as the substitution model to generate the tree. A bootstrap replication of 1000 cycles was performed to generate the tree to assess the statistical robustness. |
label | Fig. 1 |
caption | Neighbour-joining tree for the RNA-dependent RNA polymerase (RdRp) partial sequence (genomic location: 14,701-15,120) generated from Sanger sequencing. The tree was constructed using the RdRp sequence available in the GenBank sequences. Kimura 2-parameter model was used as the substitution model to generate the tree. A bootstrap replication of 1000 cycles was performed to generate the tree to assess the statistical robustness. |
p | Neighbour-joining tree for the RNA-dependent RNA polymerase (RdRp) partial sequence (genomic location: 14,701-15,120) generated from Sanger sequencing. The tree was constructed using the RdRp sequence available in the GenBank sequences. Kimura 2-parameter model was used as the substitution model to generate the tree. A bootstrap replication of 1000 cycles was performed to generate the tree to assess the statistical robustness. |
p | The complete genome sequences of four BtCoV obtained from Rousettus spp. specimens were used for generating a neighbour-joining tree (Fig. 2). These sequences were also clustered within L_D of β-CoVs as observed for partial RdRp sequence tree. These complete genome sequences were grouped into gene pairs to identify the gene with higher and lower divergence. The complete genomes of the Indian BtCoV sequences were grouped under L_D. The evolutionary divergence of ORF 1b was <0.54 between the different β-CoV lineages with a maximum score of 0.7 between different BtCoV sequences used in this study (Table III). E gene sequences had larger divergence within the β-CoV genus ranging from 2.18 to 0.94. Lineages L_A and L_C had the maximum divergence of 2.18, whereas the L_B and L_C were the least (0.94). N gene has an overall higher divergence among different lineages (ranging: 2.08-0.75). Overall, evolutionary divergence for the sequences of each gene pair demonstrated that S, N, E and M genes from the α- and δ-CoV highly diverged across the different genus. In contrast, the ORF 1b was less divergent across the genera (Table III). |
figure | Fig. 2 Phylogenetic tree for the complete genome tree: A neighbour-joining tree was generated using the representative complete genome sequence available in the GenBank sequences. Kimura 2-parameter model was used as the substitution model to generate the tree. A bootstrap replication of 1000 cycles was performed to generate the tree to assess the statistical robustness. |
label | Fig. 2 |
caption | Phylogenetic tree for the complete genome tree: A neighbour-joining tree was generated using the representative complete genome sequence available in the GenBank sequences. Kimura 2-parameter model was used as the substitution model to generate the tree. A bootstrap replication of 1000 cycles was performed to generate the tree to assess the statistical robustness. |
p | Phylogenetic tree for the complete genome tree: A neighbour-joining tree was generated using the representative complete genome sequence available in the GenBank sequences. Kimura 2-parameter model was used as the substitution model to generate the tree. A bootstrap replication of 1000 cycles was performed to generate the tree to assess the statistical robustness. |
table-wrap | Table III Evolutionary divergence for ORF 1b, S, N and M genes for the retrieved sequences with other reference sequences. The lower right-check hand matrix of the table depicts the divergence and the upper left-check matrix of the matrix (blue colour) depicts the variation observed in the bootstrap replication N gene Alpha Delta Gamma L_A L_B L_C L_D M gene Alpha Delta Gamma L_A L_B L_C L_D Alpha 0.15 0.09 0.10 0.08 0.08 0.09 Alpha 0.11 0.12 0.05 0.06 0.05 0.06 Delta 2.08 0.11 0.16 0.09 0.11 0.10 Delta 1.50 0.26 0.08 0.16 0.10 0.11 Gamma 1.57 1.49 0.08 0.08 0.09 0.08 Gamma 1.53 1.84 0.10 0.12 0.11 0.09 L_A 1.84 1.73 1.37 0.05 0.05 0.06 L_A 0.92 1.24 1.30 0.06 0.05 0.05 L_B 1.48 1.37 1.32 1.09 0.03 0.04 L_B 1.05 1.51 1.37 0.92 0.05 0.05 L_C 1.57 1.52 1.42 1.07 0.75 0.04 L_C 0.99 1.35 1.27 0.80 0.82 0.05 L_D 1.64 1.46 1.36 1.27 0.90 0.97 L_D 0.99 1.42 1.23 0.84 0.79 0.82 ORF 1b Alpha Delta Gamma L_A L_B L_C L_D ORF 1a Alpha Delta Gamma L_A L_B L_C L_D Alpha 0.01 0.01 0.01 0.01 0.01 0.01 Alpha 0.02 0.02 0.01 0.02 0.02 0.03 Delta 0.70 0.01 0.02 0.01 0.01 0.01 Delta 1.32 0.03 0.02 0.03 0.03 0.04 Gamma 0.62 0.67 0.01 0.01 0.01 0.01 Gamma 1.14 1.33 0.02 0.03 0.02 0.04 L_A 0.61 0.69 0.60 0.01 0.01 0.01 L_A 1.22 1.01 1.30 0.02 0.02 0.04 L_B 0.60 0.70 0.65 0.54 0.01 0.01 L_B 1.26 1.42 1.41 1.19 0.01 0.02 L_C 0.58 0.69 0.62 0.53 0.50 0.01 L_C 1.35 1.41 1.44 1.19 0.97 0.03 L_D 0.60 0.67 0.61 0.53 0.50 0.52 L_D 1.26 1.27 1.39 1.09 0.90 1.03 S gene Alpha Delta Gamma L_A L_B L_C L_D E gene Alpha Delta Gamma L_A L_B L_C L_D Alpha 0.02 0.02 0.03 0.03 0.03 0.02 Alpha 0.12 0.18 0.09 0.15 0.15 0.12 Delta 0.86 0.03 0.04 0.04 0.06 0.03 Delta 1.14 0.47 0.22 0.41 0.28 0.17 Gamma 1.14 0.96 0.04 0.05 0.06 0.04 Gamma 1.59 1.64 0.22 0.24 0.32 0.19 L_A 1.36 1.28 1.43 0.03 0.03 0.02 L_A 1.03 1.58 1.57 0.23 0.21 0.25 L_B 1.33 1.23 1.34 1.19 0.04 0.02 L_B 1.24 1.75 1.40 1.83 0.11 0.14 L_C 1.42 1.32 1.46 1.17 1.03 0.03 L_C 1.37 1.64 1.83 2.18 0.94 0.17 L_D 1.34 1.24 1.41 1.16 1.00 1.11 L_D 1.25 1.42 1.52 1.95 1.16 1.37 ORF 1a, open reading frame 1a polyprotein; ORF 1b, ORF 1b polyprotein; S, spike glycoprotein; N, nuclocapsid phospoptotein; M, membrane glycoprotein; E, envelope protein |
label | Table III |
caption | Evolutionary divergence for ORF 1b, S, N and M genes for the retrieved sequences with other reference sequences. The lower right-check hand matrix of the table depicts the divergence and the upper left-check matrix of the matrix (blue colour) depicts the variation observed in the bootstrap replication |
p | Evolutionary divergence for ORF 1b, S, N and M genes for the retrieved sequences with other reference sequences. The lower right-check hand matrix of the table depicts the divergence and the upper left-check matrix of the matrix (blue colour) depicts the variation observed in the bootstrap replication |
table | N gene Alpha Delta Gamma L_A L_B L_C L_D M gene Alpha Delta Gamma L_A L_B L_C L_D Alpha 0.15 0.09 0.10 0.08 0.08 0.09 Alpha 0.11 0.12 0.05 0.06 0.05 0.06 Delta 2.08 0.11 0.16 0.09 0.11 0.10 Delta 1.50 0.26 0.08 0.16 0.10 0.11 Gamma 1.57 1.49 0.08 0.08 0.09 0.08 Gamma 1.53 1.84 0.10 0.12 0.11 0.09 L_A 1.84 1.73 1.37 0.05 0.05 0.06 L_A 0.92 1.24 1.30 0.06 0.05 0.05 L_B 1.48 1.37 1.32 1.09 0.03 0.04 L_B 1.05 1.51 1.37 0.92 0.05 0.05 L_C 1.57 1.52 1.42 1.07 0.75 0.04 L_C 0.99 1.35 1.27 0.80 0.82 0.05 L_D 1.64 1.46 1.36 1.27 0.90 0.97 L_D 0.99 1.42 1.23 0.84 0.79 0.82 ORF 1b Alpha Delta Gamma L_A L_B L_C L_D ORF 1a Alpha Delta Gamma L_A L_B L_C L_D Alpha 0.01 0.01 0.01 0.01 0.01 0.01 Alpha 0.02 0.02 0.01 0.02 0.02 0.03 Delta 0.70 0.01 0.02 0.01 0.01 0.01 Delta 1.32 0.03 0.02 0.03 0.03 0.04 Gamma 0.62 0.67 0.01 0.01 0.01 0.01 Gamma 1.14 1.33 0.02 0.03 0.02 0.04 L_A 0.61 0.69 0.60 0.01 0.01 0.01 L_A 1.22 1.01 1.30 0.02 0.02 0.04 L_B 0.60 0.70 0.65 0.54 0.01 0.01 L_B 1.26 1.42 1.41 1.19 0.01 0.02 L_C 0.58 0.69 0.62 0.53 0.50 0.01 L_C 1.35 1.41 1.44 1.19 0.97 0.03 L_D 0.60 0.67 0.61 0.53 0.50 0.52 L_D 1.26 1.27 1.39 1.09 0.90 1.03 S gene Alpha Delta Gamma L_A L_B L_C L_D E gene Alpha Delta Gamma L_A L_B L_C L_D Alpha 0.02 0.02 0.03 0.03 0.03 0.02 Alpha 0.12 0.18 0.09 0.15 0.15 0.12 Delta 0.86 0.03 0.04 0.04 0.06 0.03 Delta 1.14 0.47 0.22 0.41 0.28 0.17 Gamma 1.14 0.96 0.04 0.05 0.06 0.04 Gamma 1.59 1.64 0.22 0.24 0.32 0.19 L_A 1.36 1.28 1.43 0.03 0.03 0.02 L_A 1.03 1.58 1.57 0.23 0.21 0.25 L_B 1.33 1.23 1.34 1.19 0.04 0.02 L_B 1.24 1.75 1.40 1.83 0.11 0.14 L_C 1.42 1.32 1.46 1.17 1.03 0.03 L_C 1.37 1.64 1.83 2.18 0.94 0.17 L_D 1.34 1.24 1.41 1.16 1.00 1.11 L_D 1.25 1.42 1.52 1.95 1.16 1.37 |
tr | N gene Alpha Delta Gamma L_A L_B L_C L_D M gene Alpha Delta Gamma L_A L_B L_C L_D |
th | N gene |
th | Alpha |
th | Delta |
th | Gamma |
th | L_A |
th | L_B |
th | L_C |
th | L_D |
th | M gene |
th | Alpha |
th | Delta |
th | Gamma |
th | L_A |
th | L_B |
th | L_C |
th | L_D |
tr | Alpha 0.15 0.09 0.10 0.08 0.08 0.09 Alpha 0.11 0.12 0.05 0.06 0.05 0.06 |
td | Alpha |
td | 0.15 |
td | 0.09 |
td | 0.10 |
td | 0.08 |
td | 0.08 |
td | 0.09 |
td | Alpha |
td | 0.11 |
td | 0.12 |
td | 0.05 |
td | 0.06 |
td | 0.05 |
td | 0.06 |
tr | Delta 2.08 0.11 0.16 0.09 0.11 0.10 Delta 1.50 0.26 0.08 0.16 0.10 0.11 |
td | Delta |
td | 2.08 |
td | 0.11 |
td | 0.16 |
td | 0.09 |
td | 0.11 |
td | 0.10 |
td | Delta |
td | 1.50 |
td | 0.26 |
td | 0.08 |
td | 0.16 |
td | 0.10 |
td | 0.11 |
tr | Gamma 1.57 1.49 0.08 0.08 0.09 0.08 Gamma 1.53 1.84 0.10 0.12 0.11 0.09 |
td | Gamma |
td | 1.57 |
td | 1.49 |
td | 0.08 |
td | 0.08 |
td | 0.09 |
td | 0.08 |
td | Gamma |
td | 1.53 |
td | 1.84 |
td | 0.10 |
td | 0.12 |
td | 0.11 |
td | 0.09 |
tr | L_A 1.84 1.73 1.37 0.05 0.05 0.06 L_A 0.92 1.24 1.30 0.06 0.05 0.05 |
td | L_A |
td | 1.84 |
td | 1.73 |
td | 1.37 |
td | 0.05 |
td | 0.05 |
td | 0.06 |
td | L_A |
td | 0.92 |
td | 1.24 |
td | 1.30 |
td | 0.06 |
td | 0.05 |
td | 0.05 |
tr | L_B 1.48 1.37 1.32 1.09 0.03 0.04 L_B 1.05 1.51 1.37 0.92 0.05 0.05 |
td | L_B |
td | 1.48 |
td | 1.37 |
td | 1.32 |
td | 1.09 |
td | 0.03 |
td | 0.04 |
td | L_B |
td | 1.05 |
td | 1.51 |
td | 1.37 |
td | 0.92 |
td | 0.05 |
td | 0.05 |
tr | L_C 1.57 1.52 1.42 1.07 0.75 0.04 L_C 0.99 1.35 1.27 0.80 0.82 0.05 |
td | L_C |
td | 1.57 |
td | 1.52 |
td | 1.42 |
td | 1.07 |
td | 0.75 |
td | 0.04 |
td | L_C |
td | 0.99 |
td | 1.35 |
td | 1.27 |
td | 0.80 |
td | 0.82 |
td | 0.05 |
tr | L_D 1.64 1.46 1.36 1.27 0.90 0.97 L_D 0.99 1.42 1.23 0.84 0.79 0.82 |
td | L_D |
td | 1.64 |
td | 1.46 |
td | 1.36 |
td | 1.27 |
td | 0.90 |
td | 0.97 |
td | L_D |
td | 0.99 |
td | 1.42 |
td | 1.23 |
td | 0.84 |
td | 0.79 |
td | 0.82 |
tr | ORF 1b Alpha Delta Gamma L_A L_B L_C L_D ORF 1a Alpha Delta Gamma L_A L_B L_C L_D |
td | ORF 1b |
td | Alpha |
td | Delta |
td | Gamma |
td | L_A |
td | L_B |
td | L_C |
td | L_D |
td | ORF 1a |
td | Alpha |
td | Delta |
td | Gamma |
td | L_A |
td | L_B |
td | L_C |
td | L_D |
tr | Alpha 0.01 0.01 0.01 0.01 0.01 0.01 Alpha 0.02 0.02 0.01 0.02 0.02 0.03 |
td | Alpha |
td | 0.01 |
td | 0.01 |
td | 0.01 |
td | 0.01 |
td | 0.01 |
td | 0.01 |
td | Alpha |
td | 0.02 |
td | 0.02 |
td | 0.01 |
td | 0.02 |
td | 0.02 |
td | 0.03 |
tr | Delta 0.70 0.01 0.02 0.01 0.01 0.01 Delta 1.32 0.03 0.02 0.03 0.03 0.04 |
td | Delta |
td | 0.70 |
td | 0.01 |
td | 0.02 |
td | 0.01 |
td | 0.01 |
td | 0.01 |
td | Delta |
td | 1.32 |
td | 0.03 |
td | 0.02 |
td | 0.03 |
td | 0.03 |
td | 0.04 |
tr | Gamma 0.62 0.67 0.01 0.01 0.01 0.01 Gamma 1.14 1.33 0.02 0.03 0.02 0.04 |
td | Gamma |
td | 0.62 |
td | 0.67 |
td | 0.01 |
td | 0.01 |
td | 0.01 |
td | 0.01 |
td | Gamma |
td | 1.14 |
td | 1.33 |
td | 0.02 |
td | 0.03 |
td | 0.02 |
td | 0.04 |
tr | L_A 0.61 0.69 0.60 0.01 0.01 0.01 L_A 1.22 1.01 1.30 0.02 0.02 0.04 |
td | L_A |
td | 0.61 |
td | 0.69 |
td | 0.60 |
td | 0.01 |
td | 0.01 |
td | 0.01 |
td | L_A |
td | 1.22 |
td | 1.01 |
td | 1.30 |
td | 0.02 |
td | 0.02 |
td | 0.04 |
tr | L_B 0.60 0.70 0.65 0.54 0.01 0.01 L_B 1.26 1.42 1.41 1.19 0.01 0.02 |
td | L_B |
td | 0.60 |
td | 0.70 |
td | 0.65 |
td | 0.54 |
td | 0.01 |
td | 0.01 |
td | L_B |
td | 1.26 |
td | 1.42 |
td | 1.41 |
td | 1.19 |
td | 0.01 |
td | 0.02 |
tr | L_C 0.58 0.69 0.62 0.53 0.50 0.01 L_C 1.35 1.41 1.44 1.19 0.97 0.03 |
td | L_C |
td | 0.58 |
td | 0.69 |
td | 0.62 |
td | 0.53 |
td | 0.50 |
td | 0.01 |
td | L_C |
td | 1.35 |
td | 1.41 |
td | 1.44 |
td | 1.19 |
td | 0.97 |
td | 0.03 |
tr | L_D 0.60 0.67 0.61 0.53 0.50 0.52 L_D 1.26 1.27 1.39 1.09 0.90 1.03 |
td | L_D |
td | 0.60 |
td | 0.67 |
td | 0.61 |
td | 0.53 |
td | 0.50 |
td | 0.52 |
td | L_D |
td | 1.26 |
td | 1.27 |
td | 1.39 |
td | 1.09 |
td | 0.90 |
td | 1.03 |
tr | S gene Alpha Delta Gamma L_A L_B L_C L_D E gene Alpha Delta Gamma L_A L_B L_C L_D |
td | S gene |
td | Alpha |
td | Delta |
td | Gamma |
td | L_A |
td | L_B |
td | L_C |
td | L_D |
td | E gene |
td | Alpha |
td | Delta |
td | Gamma |
td | L_A |
td | L_B |
td | L_C |
td | L_D |
tr | Alpha 0.02 0.02 0.03 0.03 0.03 0.02 Alpha 0.12 0.18 0.09 0.15 0.15 0.12 |
td | Alpha |
td | 0.02 |
td | 0.02 |
td | 0.03 |
td | 0.03 |
td | 0.03 |
td | 0.02 |
td | Alpha |
td | 0.12 |
td | 0.18 |
td | 0.09 |
td | 0.15 |
td | 0.15 |
td | 0.12 |
tr | Delta 0.86 0.03 0.04 0.04 0.06 0.03 Delta 1.14 0.47 0.22 0.41 0.28 0.17 |
td | Delta |
td | 0.86 |
td | 0.03 |
td | 0.04 |
td | 0.04 |
td | 0.06 |
td | 0.03 |
td | Delta |
td | 1.14 |
td | 0.47 |
td | 0.22 |
td | 0.41 |
td | 0.28 |
td | 0.17 |
tr | Gamma 1.14 0.96 0.04 0.05 0.06 0.04 Gamma 1.59 1.64 0.22 0.24 0.32 0.19 |
td | Gamma |
td | 1.14 |
td | 0.96 |
td | 0.04 |
td | 0.05 |
td | 0.06 |
td | 0.04 |
td | Gamma |
td | 1.59 |
td | 1.64 |
td | 0.22 |
td | 0.24 |
td | 0.32 |
td | 0.19 |
tr | L_A 1.36 1.28 1.43 0.03 0.03 0.02 L_A 1.03 1.58 1.57 0.23 0.21 0.25 |
td | L_A |
td | 1.36 |
td | 1.28 |
td | 1.43 |
td | 0.03 |
td | 0.03 |
td | 0.02 |
td | L_A |
td | 1.03 |
td | 1.58 |
td | 1.57 |
td | 0.23 |
td | 0.21 |
td | 0.25 |
tr | L_B 1.33 1.23 1.34 1.19 0.04 0.02 L_B 1.24 1.75 1.40 1.83 0.11 0.14 |
td | L_B |
td | 1.33 |
td | 1.23 |
td | 1.34 |
td | 1.19 |
td | 0.04 |
td | 0.02 |
td | L_B |
td | 1.24 |
td | 1.75 |
td | 1.40 |
td | 1.83 |
td | 0.11 |
td | 0.14 |
tr | L_C 1.42 1.32 1.46 1.17 1.03 0.03 L_C 1.37 1.64 1.83 2.18 0.94 0.17 |
td | L_C |
td | 1.42 |
td | 1.32 |
td | 1.46 |
td | 1.17 |
td | 1.03 |
td | 0.03 |
td | L_C |
td | 1.37 |
td | 1.64 |
td | 1.83 |
td | 2.18 |
td | 0.94 |
td | 0.17 |
tr | L_D 1.34 1.24 1.41 1.16 1.00 1.11 L_D 1.25 1.42 1.52 1.95 1.16 1.37 |
td | L_D |
td | 1.34 |
td | 1.24 |
td | 1.41 |
td | 1.16 |
td | 1.00 |
td | 1.11 |
td | L_D |
td | 1.25 |
td | 1.42 |
td | 1.52 |
td | 1.95 |
td | 1.16 |
td | 1.37 |
table-wrap-foot | ORF 1a, open reading frame 1a polyprotein; ORF 1b, ORF 1b polyprotein; S, spike glycoprotein; N, nuclocapsid phospoptotein; M, membrane glycoprotein; E, envelope protein |
footnote | ORF 1a, open reading frame 1a polyprotein; ORF 1b, ORF 1b polyprotein; S, spike glycoprotein; N, nuclocapsid phospoptotein; M, membrane glycoprotein; E, envelope protein |
p | ORF 1a, open reading frame 1a polyprotein; ORF 1b, ORF 1b polyprotein; S, spike glycoprotein; N, nuclocapsid phospoptotein; M, membrane glycoprotein; E, envelope protein |
sec | Discussion As per the available information, the BtCoV causing human infection belongs to α- and β-CoV genera of the Coronaviridae family. β-CoV genus has five strains known to infect humans25. The two human-infecting strains (NL63 and 229E) from α-CoV genus which cause mild-to-moderate respiratory infections are believed to have originated in bats25. Two members of the β-CoV genus (HCoV-OC43 and HCoV-HKU1) are known to cause the common cold and lower respiratory tract infections26. The other three are now shown to be pathogenic to humans (SARS-CoV-1, MERS-CoV and SARS-CoV-2). The SARS-CoV-1 and SARS-CoV-2 belong to L_B and MERS CoV belongs to L_C of β-CoV genus27. The phylogenetic analysis for the partial RdRp region revealed the presence of distinct BtCoVs in both the bats. The genomic sequences retrieved from the Indian sequences form a distinct cluster. The three CoV_BtRtBetaCoV/GX2018 sequences retrieved from the Indian Rousettus bats were 5.8-6.7 per cent different from the reference sequence, which was retrieved from Rhinolophus affinis. The two CoV_BtRtBetaCoV/GX2018 sequences retrieved from different bats were 1.2 per cent different from each other. The effect of host influence on the nt usage of the virus cannot be denied; however, it needs to be explored further in detail. Bats are reservoirs for viruses with human pathogenic potential2829, and are known to harbour a broad range of CoVs1. The global distribution of bats, along with the different types of cell receptors present within them, favours virus replication, and is a possible link to their intraspecies transmission. The interspecies spill-over of a BtCoV to humans is thought to occur through an intermediate host, in which the virus replicates through yet completely unidentified routes. In India, regions of the Western Ghats, particularly in Kerala, are reported to have habitat for diverse bat populations. The reports of pathogenic human viruses from bat specimens demand enhanced methods to monitor human exposure to various bat species. Investigations in unexplored regions/States should be focused on gaining further insights into CoV diversity within Indian bat populations. Earlier, we had reported the presence of pathogenic viruses such as the Nipah virus in Pteropus bats in India16. In the present scenario of changing demography and ecological manipulations, it is challenging to have checks on the encounters of bats with other animals and humans. Therefore, active and continuous surveillance remains crucial for outbreak alerts for bat-associated viral agents with epidemic potential, which would be helpful in timely interventions. Although CoVs in the subfamily Coronavirinae do not usually produce clinical symptoms in their natural hosts (bats), accidental transmission of these viruses to humans and other animals may result in respiratory, enteric, hepatic or neurologic diseases of variable severity. It is still not understood as to why only certain CoVs can infect people. There is a need of proactive surveillance of zoonotic infections in bats. Detection and identifications of such aetiological agents will provide leads for the development of diagnostic along with preparedness and readiness to deal with such emergent viruses thereby quickly containing them. The detection and identification of such viruses from bats also recommends cross-sectional antibody surveys (human and domestic animals) in localities where the viruses have been detected. Similarly, if epidemiological situation demands, evidence-based surveillance should also be conducted. There is a need of developing strong mechanisms for working jointly with various stakeholders such as wildlife, poultry, animal husbandry and human health departments. In conclusion, our study showed detection of pathogenic CoVs in two species of Indian bats. Continuous active surveillance is required to identify the emerging novel viruses with epidemic potential. |
title | Discussion |
p | As per the available information, the BtCoV causing human infection belongs to α- and β-CoV genera of the Coronaviridae family. β-CoV genus has five strains known to infect humans25. The two human-infecting strains (NL63 and 229E) from α-CoV genus which cause mild-to-moderate respiratory infections are believed to have originated in bats25. Two members of the β-CoV genus (HCoV-OC43 and HCoV-HKU1) are known to cause the common cold and lower respiratory tract infections26. The other three are now shown to be pathogenic to humans (SARS-CoV-1, MERS-CoV and SARS-CoV-2). The SARS-CoV-1 and SARS-CoV-2 belong to L_B and MERS CoV belongs to L_C of β-CoV genus27. |
p | The phylogenetic analysis for the partial RdRp region revealed the presence of distinct BtCoVs in both the bats. The genomic sequences retrieved from the Indian sequences form a distinct cluster. The three CoV_BtRtBetaCoV/GX2018 sequences retrieved from the Indian Rousettus bats were 5.8-6.7 per cent different from the reference sequence, which was retrieved from Rhinolophus affinis. The two CoV_BtRtBetaCoV/GX2018 sequences retrieved from different bats were 1.2 per cent different from each other. The effect of host influence on the nt usage of the virus cannot be denied; however, it needs to be explored further in detail. |
p | Bats are reservoirs for viruses with human pathogenic potential2829, and are known to harbour a broad range of CoVs1. The global distribution of bats, along with the different types of cell receptors present within them, favours virus replication, and is a possible link to their intraspecies transmission. The interspecies spill-over of a BtCoV to humans is thought to occur through an intermediate host, in which the virus replicates through yet completely unidentified routes. In India, regions of the Western Ghats, particularly in Kerala, are reported to have habitat for diverse bat populations. The reports of pathogenic human viruses from bat specimens demand enhanced methods to monitor human exposure to various bat species. Investigations in unexplored regions/States should be focused on gaining further insights into CoV diversity within Indian bat populations. |
p | Earlier, we had reported the presence of pathogenic viruses such as the Nipah virus in Pteropus bats in India16. In the present scenario of changing demography and ecological manipulations, it is challenging to have checks on the encounters of bats with other animals and humans. Therefore, active and continuous surveillance remains crucial for outbreak alerts for bat-associated viral agents with epidemic potential, which would be helpful in timely interventions. |
p | Although CoVs in the subfamily Coronavirinae do not usually produce clinical symptoms in their natural hosts (bats), accidental transmission of these viruses to humans and other animals may result in respiratory, enteric, hepatic or neurologic diseases of variable severity. It is still not understood as to why only certain CoVs can infect people. |
p | There is a need of proactive surveillance of zoonotic infections in bats. Detection and identifications of such aetiological agents will provide leads for the development of diagnostic along with preparedness and readiness to deal with such emergent viruses thereby quickly containing them. The detection and identification of such viruses from bats also recommends cross-sectional antibody surveys (human and domestic animals) in localities where the viruses have been detected. Similarly, if epidemiological situation demands, evidence-based surveillance should also be conducted. There is a need of developing strong mechanisms for working jointly with various stakeholders such as wildlife, poultry, animal husbandry and human health departments. |
p | In conclusion, our study showed detection of pathogenic CoVs in two species of Indian bats. Continuous active surveillance is required to identify the emerging novel viruses with epidemic potential. |
back | Acknowledgment Authors acknowledge the encouragement and support extended by Prof. (Dr) Balram Bhargava, Secretary, Department of Health Research, Ministry of Health & Family Welfare, Director-General, Indian Council of Medical Research (ICMR), New Delhi, and Dr Raman Gangakhedkar, Division of Epidemiology & Communicable Diseases, ICMR, New Delhi. Authors acknowledge the Principal Conservators of Forests of Kerala, Karnataka, Tamil Nadu, Himachal Pradesh, Punjab, Gujarat, Odisha, Telangana, Chandigarh and Puducherry and other concerned officials for their permission to capture bats. Authors are also thankful to Servshri Rajen Lakra, Prasad Sarkale, Pranita Gawande, Kaumudi Kalele, Shrimati Ashwini Waghmare, Nikita Bankar and Shri Sanjay Thorat for providing technical support. Authors acknowledge the contribution of the field team for the hard work performed by Servshri Sanjay Gopale, Annasaheb Suryawanshi, Manjunath Holeppanavar, Ganesh Chopade, Madhav Acharya, D.K. Singh and Sachin Daigude for technical assistance during the study. Financial support & sponsorship: This work was supported by intramural funding by the Indian Council of Medical Research, New Delhi Conflicts of Interest: None. |
ack | Acknowledgment Authors acknowledge the encouragement and support extended by Prof. (Dr) Balram Bhargava, Secretary, Department of Health Research, Ministry of Health & Family Welfare, Director-General, Indian Council of Medical Research (ICMR), New Delhi, and Dr Raman Gangakhedkar, Division of Epidemiology & Communicable Diseases, ICMR, New Delhi. Authors acknowledge the Principal Conservators of Forests of Kerala, Karnataka, Tamil Nadu, Himachal Pradesh, Punjab, Gujarat, Odisha, Telangana, Chandigarh and Puducherry and other concerned officials for their permission to capture bats. Authors are also thankful to Servshri Rajen Lakra, Prasad Sarkale, Pranita Gawande, Kaumudi Kalele, Shrimati Ashwini Waghmare, Nikita Bankar and Shri Sanjay Thorat for providing technical support. Authors acknowledge the contribution of the field team for the hard work performed by Servshri Sanjay Gopale, Annasaheb Suryawanshi, Manjunath Holeppanavar, Ganesh Chopade, Madhav Acharya, D.K. Singh and Sachin Daigude for technical assistance during the study. |
title | Acknowledgment |
p | Authors acknowledge the encouragement and support extended by Prof. (Dr) Balram Bhargava, Secretary, Department of Health Research, Ministry of Health & Family Welfare, Director-General, Indian Council of Medical Research (ICMR), New Delhi, and Dr Raman Gangakhedkar, Division of Epidemiology & Communicable Diseases, ICMR, New Delhi. Authors acknowledge the Principal Conservators of Forests of Kerala, Karnataka, Tamil Nadu, Himachal Pradesh, Punjab, Gujarat, Odisha, Telangana, Chandigarh and Puducherry and other concerned officials for their permission to capture bats. Authors are also thankful to Servshri Rajen Lakra, Prasad Sarkale, Pranita Gawande, Kaumudi Kalele, Shrimati Ashwini Waghmare, Nikita Bankar and Shri Sanjay Thorat for providing technical support. Authors acknowledge the contribution of the field team for the hard work performed by Servshri Sanjay Gopale, Annasaheb Suryawanshi, Manjunath Holeppanavar, Ganesh Chopade, Madhav Acharya, D.K. Singh and Sachin Daigude for technical assistance during the study. |
footnote | Financial support & sponsorship: This work was supported by intramural funding by the Indian Council of Medical Research, New Delhi |
p | Financial support & sponsorship: This work was supported by intramural funding by the Indian Council of Medical Research, New Delhi |
footnote | Conflicts of Interest: None. |
p | Conflicts of Interest: None. |
Annnotations TAB TSV DIC JSON TextAE
last updated at 2021-04-26 03:49:47 UTC
- Denotations: 1
- Blocks: 0
- Relations: 0