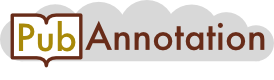
PMC:7079563 / 126-204 JSONTXT 4 Projects
The epidemic of 2019-novel-coronavirus (2019-nCoV) pneumonia and insights for emerging infectious diseases in the future
Abstract
At the end of December 2019, a novel coronavirus, 2019-nCoV, caused an outbreak of pneumonia spreading from Wuhan, Hubei province, to the whole country of China, which has posed great threats to public health and attracted enormous attention around the world. To date, there are no clinically approved vaccines or antiviral drugs available for these human coronavirus infections. Intensive research on the novel emerging human infectious coronaviruses is urgently needed to elucidate their route of transmission and pathogenic mechanisms, and to identify potential drug targets, which would promote the development of effective preventive and therapeutic countermeasures. Herein, we describe the epidemic and etiological characteristics of 2019-nCoV, discuss its essential biological features, including tropism and receptor usage, summarize approaches for disease prevention and treatment, and speculate on the transmission route of 2019-nCoV.
In December 2019, a novel coronavirus was detected in pneumonia patients which was later named 2019-nCoV. Since the first human coronavirus detected in the 1960s, 2019-nCoV is the seventh coronavirus that is known to infect humans [1]. Among all the human coronaviruses, four of them, including NL-63, 229E, HKU1 and OC43, cause mild illness, while the other three, SARS-CoV, MERS-CoV and 2019-nCoV, lead to serious disease [2]. To characterize the new pathogen causing acute infection in humans, researchers have conducted urgent studies on the 2019-nCoV, from its epidemiology and clinical medicine to etiology and virology. This review focuses on the epidemiology and clinical manifestations of 2019-nCoV, the diagnosis and treatment of infected patients, and in particular, evidence regarding the origin of 2019-nCoV.
1 Emergence and epidemic of pneumonias caused by 2019-nCoV
1.1 Pathogen identification and transmission of pneumonia
On 31th December 2019, the first pneumonia case of unknown etiology was reported in Wuhan City, Hubei Province, China. In order to identify the etiology, a number of possible etiological agents were ruled out, including the Severe acute respiratory syndrome coronavirus (SARS-CoV), the Middle-East respiratory syndrome coronavirus (MERS-CoV), avian influenza virus, and other common respiratory pathogens. Finally, a new coronavirus, temporally named 2019-nCoV, was identified to be the pathogen responsible for disease, and it was announced by World Health Organization (WHO) on 12th January [3]. As of January 11, 2020, 41 pneumonia cases were diagnosed, including seven severe cases and one death [2]. At that time, there was no evidence of person-to-person transmission according to the scientific literature. The first “super-spreading” event became a hallmark of the epidemiology of the disease. On January 20, 2020, in epidemiological investigations, two local infections in patients who were infected by 2019-nCoV without physical visits to Wuhan were reported in Guangdong Province, which confirmed the occurrence of human-to-human transmission [4]. Then, 14 medical staff infected by 2019-nCoV from patients further confirmed the human-to-human contagion [5]. On 21th January 2020, WHO announced that 2019-nCoV was possibly sustained through human-to-human transmission, warning the public to take self-protection measures [3]. The first of many cases, this disease was rapidly shown to cause an epidemic [6]. Due to the high transmissibility of 2019-nCoV, the Chinese government has taken quick action and various measures to deal with the epidemic, trying to prevent spread of the outbreak and to treat patients. Researchers put much effort to understand the origin and pathophysiology of this novel coronavirus, and have been testing multiple drugs to screen effective therapy.
1.2 Current situation of the epidemic
Until February 6, 2020, confirmed 2019-nCoV pneumonia cases were increasing with worrisome speed in China. As of 5th February, there were 24,377 cases confirmed in mainland China, including 492 deaths, 3219 severe cases, and 901 cured cases [6]. The fatality rate was about 2%. In addition, 175 cases of 2019-nCoV infection were confirmed in 24 other countries around the world. The typical events during the 2019-nCoV outbreak are summarized in Table 1 . The period from the end of December 2019 to January 2020 was the “Spring Festival travel rush” time in China. Although the government issued an order to control the flow of people, the disease spread rapidly to other cities because of asymptomatic carriers [[7], [8], [9]].
Table 1 Key events of 2019-nCoV.
Date Key events
31 December 2019 The Health Commission of Hubei province, China, first announced a cluster of unexplained cases of pneumonia.
3 January 2020 A total of 44 patients (11 are severely ill, while the remaining 33 patients are in stable condition.) with pneumonia of unknown etiology have been reported to WHO by the national authorities in China.
5 January 2020 The WHO published a document detailing 44 patients had “pneumonia of unknown etiology”, with 121 close contacts under surveillance.
8 January 2020 The genetic sequencing demonstrated that the novel coronavirus is the potential causative organism.
11 January 2020 41 patients have been diagnosed to have infection of the novel coronavirus, with 763 close contacts, 7 severe cases and the first death.
12 January 2020 The virus was tentatively named by WHO as the 2019 novel coronavirus (2019-nCoV).
13 January 2020 The first imported case of 2019-nCoV was found in Thailand. This is the first infected person diagnosed outside China. The patient is a 61-year-old Chinese woman living in Wuhan City, Hubei Province, China.
16 January 2020 The first case reported in Japan. This is the second confirmed case of 2019-nCoV that has been detected outside of China. The person travelled to Wuhan, China.
19 January 2020 The first case reported in Korea. The patient was a Chinese woman who flew from Wuhan to Incheon International Airport. Four other suspected patients were being examined. Furthermore, the first case reported in Shenzhen, China.
20 January 2020 Two local infections in the Chinese province of Guangdong with no direct visit to Wuhan were the first confirmed the occurrence of human-to-human transmission through epidemiological investigation. And the first case reported in Shanghai, China.
21 January 2020 15 health-care workers infected with the 2019-nCoV in Wuhan. Zhejiang Province, Henan Province, Sichuan Province and Taiwa confirmed the first case of 2019-nCoV, respectively. The WHO announced that there was possible sustained human to-human transmission.
22–23 January 2020 17 death in Hubei Province. America and Macao and Many provinces confirmed the first case of 2019-nCoV. Such as Shandong Province, Yunnan Province, Hunan Province, America, Macao, Anhui Province, Fujian Province, Guizhou Province, Shanxi Province, Ningxia Autonomous Region and Hebei Province reported the first case of 2019-nCoV, respectively.
23 January 2020 the local government in Wuhan announced the suspension of public transportation, with closure of airports, railway stations, and highways
24 January 2020 Zhejiang and Shanghai’s first patients diagnosed with 2019-nCoV recover.
5 February 2020 The confirmed pneumonia cases are increasing in 34 provinces, municipalities, and special administrative regions in China. 24,377 cases of 2019-nCoV confirmed in China, including 492 deaths, 3219 serious, and 901 cured. 175 cases of infection were confirmed in 24 other cities around the world.
1.3 Preventive measures
To deal with the 2019-nCoV epidemic, the central and local governments of China took a series of drastic measures. First of all, Chinese health authorities conducted an immediate investigation to characterize and control the disease, including isolation of suspected patients, monitoring of contact clinical status of patients, and developing diagnostic and treatment procedures [5]. In addition, on January 23, 2020, the local government of Wuhan announced the suspension of public transportation, closing airports, railway stations, and highways in the city, in order to prevent further disease transmission [10]. Subsequently, most provinces in China claimed “Level I Emergency Response” by taking a series of measures such as suspending public transportation and setting up community quarantines. Particularly, the list of the first-level designated hospitals was announced, and epidemic prevention training for primary medical staff was started. Since fever is one of typical clinical symptoms of the infection, temperature detection of incoming and outgoing personnel was implemented comprehensively in railway stations, passenger stations and other public places, in order to screen suspected cases as early as possible. In addition, many other compulsory measures limiting population mobility, such as cancellation of mass gatherings, school closures, work-from-home arrangements, was taken to reduce within-population contact rates.
2 Epidemiological and clinical symptoms of 2019-nCoV
2.1 Human coronaviruses
Human coronaviruses (CoVs) could cause respiratory, gastrointestinal, hepatic and central nervous system diseases. Infection outcomes vary from mild, self-limiting disease, to more severe manifestations and even death [1,11]. Among the seven human CoVs, two α-coronaviruses (HCoV-229E and HCoV-NL63) and two β-coronaviruses (HCoV-OC43 and HCoV-HKU1) were thought to cause only mild self-limiting upper respiratory disease, such as common cold in immunocompetent hosts, except for a few cases of severe infections in infants, children and seniors [12,13]; the other three CoVs could cause fatal respiratory diseases. In 2002, the Severe acute respiratory syndrome coronavirus (SARS-CoV) emerged in Guangdong province, China, spreading to 37 countries, and its subsequent global epidemic was associated with 8096 cases and 774 deaths. Ten years later, Middle-East respiratory syndrome coronavirus (MERS-CoV) spread to 27 countries, causing 2494 infected cases and 858 deaths worldwide [1]. And the recently identified novel coronavirus (2019-nCoV), was the third highly pathogenic CoV discovered, with a mortality of 2% which is much lower than that of SARS-CoV (10%) and MERS-CoV (37%) [6]. However, the transmissibility of 2019-nCoV is higher, the mean R0 (R0 is used to estimate the transmissibility of virus) of 2019-nCoV ranged from 3.3 to 5.5, and it appeared (slightly) higher than those of SARS-CoV (2–5) and MERS-CoV (2.7–3.9) [[14], [15], [16]]. Measures on ‘entry, exit and travel’ screening and restrictions are likely to reduce the effective R0, which should benefit 2019-nCoV control and prevention.
2.2 Clinical symptoms of 2019-nCoV infection
The clinical symptoms of 2019-nCoV infection are similar to those of SARS-CoV and MERS-CoV. Most patients present fever, dry cough, dyspnea, and bilateral ground-glass opacities on chest CT scans [4,17,18]. However, patients with 2019-nCoV infection rarely have obvious upper respiratory signs and symptoms (such as snot, sneezing, or sore throat), indicating that the virus primarily infects the lower respiratory tract [4,17]. In addition, about 20–25% of 2019-nCoV patients experience intestinal symptoms and signs (such as diarrhea), similarly to MERS-CoV or SARS-CoV [17]. In severe 2019-nCoV infection cases, the symptoms include acute respiratory distress syndrome, septic shock, metabolic acidosis, and bleeding and coagulation dysfunction. It is worth noting that severe and critically ill patients may have moderate to low fever during the course of the disease, even without obvious fever [3]. Furthermore, like SARS-CoV and MERS-CoV, 2019-nCoV infections induce production of high levels of cytokines [2,17].
The epidemic of 2019-nCoV bears some similarities to SARS-CoV. The outbreaks of the two viruses occurred at about the same time during the year, and they were quite stable in the environment, especially in air-conditioned space, owing to lower ambient temperature and lower humidity [19]. However, SARS-CoV had an aberrant trait that the “viral load” in upper respiratory tract secretions was low in the first 5 days of illness, then increased progressively and peaked early in the second week. Consequently, the transmission rate was relatively low in the first days of illness, providing an opportunity for case detection and isolation to interrupt transmission. On the contrary, for 2019-nCoV, the incubation lasts for an average of 10 days (in a reported range of 2–14 days) from infection to symptoms surfacing [2,4,17,20]. Even worse, 2019-nCoV is able to spread from one person to another even before any actual clinical manifestations, leading to “extremely challenging” conditions for detecting and isolating potential patients, which makes it more difficult to control the epidemic.
2.3 Transmission routes of the disease
2019-nCoV is thought to be transmitted through droplets, close contact, aerosol and maybe fecal-oral transmission, and patients in the incubation period can transmit the virus to other persons [5]. The distribution of the viral receptor can explain the pathogenic mechanisms, clinical manifestations and transmission routes of 2019-nCoV [20,21]. ACE2, a receptor for 2019-nCoV, is necessary for the viral entry of 2019-nCoV. The ubiquitous expression of ACE2 in various cells, such as lung AT2 cells, esophagus upper, stratified epithelial cells, and absorptive enterocytes of ileum and colon may contribute to the multi-tissue infection of 2019-nCoV [22]. Therefore, besides respiratory and bodily contact, fecal-oral transmission is a potential route for 2019-nCoV infection [23,24].
3 Biological features and origin of the 2019-nCoV
3.1 Genomics of 2019-nCoV
According to the genome analysis, 2019-nCoV belongs to Sarbecovirus subgenus, Betacoronavirus genus of the subfamily Orthocoronavirinae in the family Coronaviridae of the suborder cornidovirineae of the order Nidovirales [20,26]. Similarly to other coronaviruses, 2019-nCoV is an enveloped, single-stranded positive-sense RNA (+ssRNA) with around 29,900 nt, showing a typical coronavirus appearance under electron microscopy [20]. The genome organization is 5′-leader-UTR-replicase-S (Spike)-E (Envelope)-M (Membrane)-N (Nucleocapsid)-3′UTRpoly (A) tail with accessory genes interspersed within the structural genes and the 3′ end of the genome [26].
2019-nCoV shares 79.5% nt identity with SARS-CoV and 96% identity with bat-CoV-RaTG13 [20]. The genetic characteristics of 2019-nCoV are significantly different from SARSr-CoV and SARS-CoV, which are closely related only to the specific bat SARS-like coronaviruses, bat-CoV-RaTG13 and bat-SL-CoVZC45, with 96% and 86.9% nucleotide sequence identities, respectively [20]. Therefore 2019-nCoV is considered as a SARS related coronavirus (SARSr-CoV) by pairwise protein sequence analysis (Fig. 1 ) [20].
Fig. 1 The evolutionary position of 2019-nCoV. The phylogenetic tree was constructed based on the complete genomic sequences of 2019-nCoV and related CoVs by using the neighbor-joining (NJ) method with 1000 bootstrap. The novel 2019-nCoV detected strains were indicated in bold with solid squares. Abbreviations are as follows: SARSr coronavirus, SARS-related coronavirus. The accession numbers of CoVs were showed in the figure. Bootstrap values above 50 were shown.
3.2 Receptor usage of 2019-nCoV
The prerequisite of coronaviruses invading the host-cell is to bind to receptors. Following receptor binding, the viral spike protein is cleaved via acid-dependent proteolysis by cathepsin, TMPRRS2 or furin protease, followed by fusion of the viral envelop to the cellular membranes. Spike is a large, clove-shaped trimer, which can be cleaved by proteases into an N-terminal S1 subunit containing the receptor binding domain (RBD) and a C-terminal S2 region. Compared to other proteins of coronavirus, the spike protein has the most variable amino acid sequences which results from the strongest positive selection among all genes of coronavirus to adapt to its hosts [27].The RBD is a major determinant of host range and cross-species transmission. Recently results showed that 2019-nCoV uses the same cell entry receptor, ACE2, as SARS-CoV [21,24,20,28]. Besides human ACE2, 2019-nCoV can use Chinese horseshoe bats, civet, or pig ACE2 but not mouse ACE2 as an entry receptor [24]. One explanation is that human ACE2 has high homology with Chinese horseshoe bats, civet, pig and mouse, which are 80.75%, 83.48%, 81.37%, 82.11% respectively (Table 2 ), while the residues 82 and 353 of mouse ACE2 are different from human ACE2 which are important residues for bind to spike [29]. But the homology of human ACE2 and pangolin ACE2, cat ACE2 are also high with 84.84% and 85.22%. The homology of human ACE2 with other ACE2 varies from 59.73% to 83.23%. In summary, 2019-nCoV may have extensive host ranges. Fig. 2 .
Table 2 The amino acid sequence identities of ACE2 among different species.
Species Pairwise identities (%) of the amino acid sequences of ACE2
African green monkey Civet Bat (R. sinicus) Swine Malayan pangolin Mouse Cat Camel Goat Bos mutus Erinaceus europaeus Cavia porcellus Phascolarctos cinereus Sarcophilus harrisii Tiger Snake Parus major Gallus gallus
Human 93.93 83.48 80.75 81.37 84.84 82.11 85.22 83.23 81.61 81.24 79.01 77.29 71.17 70.51 59.73 67.08 65.31
African green monkey 81.78 79.93 79.93 83.77 81.78 83.15 81.91 81.41 80.92 77.94 76.85 70.38 69.84 59.21 65.6 64.13
Civet 81.78 81.49 82.73 85.59 81.61 93.29 83.85 81.86 81.74 79.75 75.55 71.66 71.63 59.6 67.44 66.3
Bat (R. sinicus) 79.93 81.49 80 82.86 78.26 83.73 81.37 80.37 80 78.51 74.56 69.68 70.02 58.61 65.11 65.19
Swine 79.93 82.73 80 82.61 80.62 83.98 89.81 87.7 87.58 77.64 75.3 71.17 71.01 61.09 66.95 65.56
Malayan pangolin 83.77 85.59 82.86 82.61 82.73 87.33 84.72 82.11 81.86 80.87 77.17 71.66 70.89 59.6 67.44 65.07
Mouse 81.78 81.61 78.26 80.62 82.73 81.74 80.99 80.12 80.37 78.63 78.16 71.05 71.13 61.21 67.2 66.17
Cat 83.15 93.29 83.73 83.98 87.33 81.74 85.71 83.23 83.11 81.49 76.42 72.65 72.25 59.6 67.81 66.79
Camel 81.91 83.85 81.37 89.81 84.72 80.99 85.71 88.2 88.57 79.25 77.29 71.79 72.12 61.34 67.2 66.42
Goat 81.41 81.86 80.37 87.7 82.11 80.12 83.23 88.2 97.76 78.14 76.54 72.04 71.38 60.84 66.09 65.44
Bos mutus 80.92 81.74 80 87.58 81.86 80.37 83.11 88.57 97.76 78.39 77.04 71.42 71.01 61.09 66.09 65.31
Erinaceus europaeus 77.94 79.75 78.51 77.64 80.87 78.63 81.49 79.25 78.14 78.39 74.56 70.67 69.65 60.59 68.8 66.42
Cavia porcellus 76.85 75.55 74.56 75.3 77.17 78.16 76.42 77.29 76.54 77.04 74.56 66.61 66.82 59.38 63.9 63.12
Phascolarctos cinereus 70.38 71.66 69.68 71.17 71.66 71.05 72.65 71.79 72.04 71.42 70.67 66.61 81.92 60.7 67.08 64.83
Sarcophilus harrisii 69.84 71.63 70.02 71.01 70.89 71.13 72.25 72.12 71.38 71.01 69.65 66.82 81.92 60.9 64.62 63.84
Tiger Snake 59.21 59.6 58.61 61.09 59.6 61.21 59.6 61.34 60.84 61.09 60.59 59.38 60.7 60.9 64.5 63.71
Parus major 65.6 67.44 65.11 66.95 67.44 67.2 67.81 67.2 66.09 66.09 68.8 63.9 67.08 64.62 64.5 82.2
Gallus gallus 64.13 66.3 65.19 65.56 65.07 66.17 66.79 66.42 65.44 65.31 66.42 63.12 64.83 63.84 63.71 82.2
Accession numbers: human (NP_068576.1), monkey (AY996037.1), civet (AAX63775.1), bat (R.sinicus) (AGZ48803.1), swine (NP_001116542.1), Malayan pangolin (XP_017505752.1), mouse (NP_001123985.1), cat (NP_001034545.1), camel (XP_010991717.1), goat (NP_001277036.1), Bos mutus (XP_005903173.1), Erinaceus europaeus (XP_007538670.1), Phascolarctos cinereus (XP_020863153.1), Sarcophilus harrisii (XP_031814825.1), tiger Snake (XP_026530754.1), Parus major (XP_015486815.1), Gallus gallus (QEQ50331.1).
Fig. 2 Phylogenetic analysis of ACE2 from humans and 18 other animals. The tree was constructed based on the complete amino acid sequences of ACE2s derived from humans and other animals by using the neighbor-joining (NJ) method with 1000 bootstrap. The accession numbers were as the same as Table 2. Bootstrap values above 50 were shown.
3.3 Origin of 2019-nCoV
The epidemiological investigation of early cases of 2019-nCoV pneumonia showed that many cases had been exposed to the Huanan Seafood Market in Wuhan [2,24,20]. In addition, 33 of the 585 environmental samples from Huanan Seafood Market were positive for 2019-nCoV; however, that market was trading a variety of live animals, such as hedgehog, badger, snake, and bird (turtledoves), probably pangolin, but not bats [24,30]. Thus, bats are less likely to have direct contact with human, and direct transmission of the virus from bat to human is unlikely [1]. Indeed, SARS-CoV and MERS-CoV originated from bats, the natural reservoir, but transmitted to humans via intermediate host civets and camels, respectively. Therefore, 2019-nCoV may also originate from bat but was then transmitted to humans via an intermediate host in the market [[31], [32], [33], [34], [35]]. Currently, 2019-nCoV has been isolated from pangolins and it was found that the genomic seuqence of the isolated strain had 99% identity with that of currently 2019-nCoV infected humans. It has been proposed that the transmission and evolution path of 2019-nCoV was from bat-CoV to pangolins (the intermediate hosts), from where it infected humans [36].
4 Diagnosis and treatment
Diagnosis of coronaviruses is unnecessary in most cases of self-limited infection, as most of the patients have mild or moderate syndromes with good prognosis. However, it may be essential to identify an etiological agent in epidemiological studies, especially during the epidemics outbreak. Since 2019-nCoV has not been found before in humans, there is no vaccine or special treatment for it so far. The number of cases is increasing rapidly, even under the current public health emergency. Thus, diagnosing all suspected cases as soon as possible and immediately isolating them is critical to cutting off the source of infection. Conventional diagnostic testing methods, such as the assays for detection of antiviral antibodies or viral antigens have been developed and used clinically. New diagnostic strategies including multiplex nucleic acid amplification and microarray-based assays may help in epidemiologic monitoring, along with taking effective prevention steps [37,38]. 2019-nCoV nucleic acids can be detected in specimens including nasopharyngeal swabs, sputum, lower respiratory tract secretions, blood and stool [20].
There are currently no approved antiviral treatments or vaccines for human coronavirus infections. The development of safe, stable vaccines is a huge challenge and vaccines would ideally be broad-spectrum, so the research and development of new drugs is a very long process. In such a sudden epidemic, scientists were not able to carry out new drug development by the traditional principles. Therefore, there is another option: a systematic and large-scale screening of existing drugs to see whether they have activity against the 2019-nCoV. Recently drug screening found that nelfinavir has potential antiviral activity against 2019-nCoV. In addition, pitavastatin, perampanel, and praziquantel might also have moderate activities against 2019-nCoV [39]. Based on previous studies, an anti-HIV drug named Kaletra may have therapeutic effect on SARS and MERS, which is composed of two protease inhibitors, ritonavir (CAS#: 155,213-67-5) and lopinavir (CAS#: 192,725-17-0) [[40], [41], [42], [43], [44]]. More recently, Kaletra was also recommended to treat Wuhan pneumonia by the National Health Commission of the People’s Republic of China. Patients with SARS or MERS have used several drugs including ribavirin, interferon, lopinavir-ritonavir, and corticosteroids, but the efficacy of certain drugs is still controversial. Other antiviral drugs, like FAD-approved drugs including ribavirin, penciclovir, nitrazine, nalfamusta, chloroquine, and the two broad-spectrum antiviral drugs redexivir (GS-5734) and favivir (T-705), are being evaluated by measuring the effects of these compounds on cytotoxicity, virus yield and infection rate of 2019-nCoV. Recent results showed that remdesivir and chloroquine are effective in controlling 2019-nCoV infection in vitro and may be evaluated in human patients with 2019-nCoV disease [45]. Remdesivir has recently been recognized as a promising antiviral drug against multiple RNA viruses (including SARS/MERS-CoV) in cultured cells, and in mouse and non-human primate (NHP) models. Currently remdesivir for the treatment of Ebola virus infection is in clinical research. It is promising that these compounds can treat 2019-nCoV infections. Moreover, the fifth edition of infection prevention and control (IPC) guidance announced that severe and critically ill patients could be treated with recovery plasma.
5 Conclusions and future perspectives
The 2019-nCoV epidemic continues. During the past month, huge progress has been made in pathogen monitoring, identifying sources, basic etiology, clinical treatment, drug testing, and vaccine development. With the efforts of China and the helps of other countries in the world, we hope that the epidemic will subside soon. Future research on viral replication, pathogenesis, antiviral drugs and other aspects of 2019-nCoV will contribute to the treatment and prevention of the virus. However, given the emergence of 2019-nCoV pneumonia as a new infectious disease with interspecies transmission from animals, we should reflect on the origin of the human pathogen and learn from our experience. With changes of ecology and human activities, including unlimited invasion of natural habitats of animals, consumption of some of these animals, and modern agricultural practices, the spillover of viruses from natural hosts to humans continues to appear and may even be escalating. Although we have built an unparalleled human civilization and a highly developed information society, invisible viruses can still have devastating effects on human beings. The high lethality viruses such as SARS-CoV, MERS-CoV, H5N1, H7N9, Ebola and emerging 2019-nCoV should serve as an alarm to the world. We should strive to reduce the probability of their occurrence and harm. Strengthening the investigation of animal etiology, normalizing the storage of high risk pathogens, reducing direct contact with wildlife, maintaining the barriers between natural reservoirs and human society and completely eradicating wildlife trading will help to achieve these goals.
Declaration of Competing Interest
The authors declare that they have no conflict of interest.
Acknowledgements
This work was jointly funded by the National Key Research and Development Program of China (grant number 2017YFD0500104), National Natural Science Foundation of China (31470260), and the Science Fund for Distinguished Young Scholars of Hunan Province (grant number 2019JJ20004).
|
Document structure show
article-title | The epidemic of 2019-novel-coronavirus (2019-nCoV) pneumonia and insights for emerging infectious diseases in the future |
abstract | At the end of December 2019, a novel coronavirus, 2019-nCoV, caused an outbreak of pneumonia spreading from Wuhan, Hubei province, to the whole country of China, which has posed great threats to public health and attracted enormous attention around the world. To date, there are no clinically approved vaccines or antiviral drugs available for these human coronavirus infections. Intensive research on the novel emerging human infectious coronaviruses is urgently needed to elucidate their route of transmission and pathogenic mechanisms, and to identify potential drug targets, which would promote the development of effective preventive and therapeutic countermeasures. Herein, we describe the epidemic and etiological characteristics of 2019-nCoV, discuss its essential biological features, including tropism and receptor usage, summarize approaches for disease prevention and treatment, and speculate on the transmission route of 2019-nCoV. |
p | At the end of December 2019, a novel coronavirus, 2019-nCoV, caused an outbreak of pneumonia spreading from Wuhan, Hubei province, to the whole country of China, which has posed great threats to public health and attracted enormous attention around the world. To date, there are no clinically approved vaccines or antiviral drugs available for these human coronavirus infections. Intensive research on the novel emerging human infectious coronaviruses is urgently needed to elucidate their route of transmission and pathogenic mechanisms, and to identify potential drug targets, which would promote the development of effective preventive and therapeutic countermeasures. Herein, we describe the epidemic and etiological characteristics of 2019-nCoV, discuss its essential biological features, including tropism and receptor usage, summarize approaches for disease prevention and treatment, and speculate on the transmission route of 2019-nCoV. |
body | In December 2019, a novel coronavirus was detected in pneumonia patients which was later named 2019-nCoV. Since the first human coronavirus detected in the 1960s, 2019-nCoV is the seventh coronavirus that is known to infect humans [1]. Among all the human coronaviruses, four of them, including NL-63, 229E, HKU1 and OC43, cause mild illness, while the other three, SARS-CoV, MERS-CoV and 2019-nCoV, lead to serious disease [2]. To characterize the new pathogen causing acute infection in humans, researchers have conducted urgent studies on the 2019-nCoV, from its epidemiology and clinical medicine to etiology and virology. This review focuses on the epidemiology and clinical manifestations of 2019-nCoV, the diagnosis and treatment of infected patients, and in particular, evidence regarding the origin of 2019-nCoV. 1 Emergence and epidemic of pneumonias caused by 2019-nCoV 1.1 Pathogen identification and transmission of pneumonia On 31th December 2019, the first pneumonia case of unknown etiology was reported in Wuhan City, Hubei Province, China. In order to identify the etiology, a number of possible etiological agents were ruled out, including the Severe acute respiratory syndrome coronavirus (SARS-CoV), the Middle-East respiratory syndrome coronavirus (MERS-CoV), avian influenza virus, and other common respiratory pathogens. Finally, a new coronavirus, temporally named 2019-nCoV, was identified to be the pathogen responsible for disease, and it was announced by World Health Organization (WHO) on 12th January [3]. As of January 11, 2020, 41 pneumonia cases were diagnosed, including seven severe cases and one death [2]. At that time, there was no evidence of person-to-person transmission according to the scientific literature. The first “super-spreading” event became a hallmark of the epidemiology of the disease. On January 20, 2020, in epidemiological investigations, two local infections in patients who were infected by 2019-nCoV without physical visits to Wuhan were reported in Guangdong Province, which confirmed the occurrence of human-to-human transmission [4]. Then, 14 medical staff infected by 2019-nCoV from patients further confirmed the human-to-human contagion [5]. On 21th January 2020, WHO announced that 2019-nCoV was possibly sustained through human-to-human transmission, warning the public to take self-protection measures [3]. The first of many cases, this disease was rapidly shown to cause an epidemic [6]. Due to the high transmissibility of 2019-nCoV, the Chinese government has taken quick action and various measures to deal with the epidemic, trying to prevent spread of the outbreak and to treat patients. Researchers put much effort to understand the origin and pathophysiology of this novel coronavirus, and have been testing multiple drugs to screen effective therapy. 1.2 Current situation of the epidemic Until February 6, 2020, confirmed 2019-nCoV pneumonia cases were increasing with worrisome speed in China. As of 5th February, there were 24,377 cases confirmed in mainland China, including 492 deaths, 3219 severe cases, and 901 cured cases [6]. The fatality rate was about 2%. In addition, 175 cases of 2019-nCoV infection were confirmed in 24 other countries around the world. The typical events during the 2019-nCoV outbreak are summarized in Table 1 . The period from the end of December 2019 to January 2020 was the “Spring Festival travel rush” time in China. Although the government issued an order to control the flow of people, the disease spread rapidly to other cities because of asymptomatic carriers [[7], [8], [9]]. Table 1 Key events of 2019-nCoV. Date Key events 31 December 2019 The Health Commission of Hubei province, China, first announced a cluster of unexplained cases of pneumonia. 3 January 2020 A total of 44 patients (11 are severely ill, while the remaining 33 patients are in stable condition.) with pneumonia of unknown etiology have been reported to WHO by the national authorities in China. 5 January 2020 The WHO published a document detailing 44 patients had “pneumonia of unknown etiology”, with 121 close contacts under surveillance. 8 January 2020 The genetic sequencing demonstrated that the novel coronavirus is the potential causative organism. 11 January 2020 41 patients have been diagnosed to have infection of the novel coronavirus, with 763 close contacts, 7 severe cases and the first death. 12 January 2020 The virus was tentatively named by WHO as the 2019 novel coronavirus (2019-nCoV). 13 January 2020 The first imported case of 2019-nCoV was found in Thailand. This is the first infected person diagnosed outside China. The patient is a 61-year-old Chinese woman living in Wuhan City, Hubei Province, China. 16 January 2020 The first case reported in Japan. This is the second confirmed case of 2019-nCoV that has been detected outside of China. The person travelled to Wuhan, China. 19 January 2020 The first case reported in Korea. The patient was a Chinese woman who flew from Wuhan to Incheon International Airport. Four other suspected patients were being examined. Furthermore, the first case reported in Shenzhen, China. 20 January 2020 Two local infections in the Chinese province of Guangdong with no direct visit to Wuhan were the first confirmed the occurrence of human-to-human transmission through epidemiological investigation. And the first case reported in Shanghai, China. 21 January 2020 15 health-care workers infected with the 2019-nCoV in Wuhan. Zhejiang Province, Henan Province, Sichuan Province and Taiwa confirmed the first case of 2019-nCoV, respectively. The WHO announced that there was possible sustained human to-human transmission. 22–23 January 2020 17 death in Hubei Province. America and Macao and Many provinces confirmed the first case of 2019-nCoV. Such as Shandong Province, Yunnan Province, Hunan Province, America, Macao, Anhui Province, Fujian Province, Guizhou Province, Shanxi Province, Ningxia Autonomous Region and Hebei Province reported the first case of 2019-nCoV, respectively. 23 January 2020 the local government in Wuhan announced the suspension of public transportation, with closure of airports, railway stations, and highways 24 January 2020 Zhejiang and Shanghai’s first patients diagnosed with 2019-nCoV recover. 5 February 2020 The confirmed pneumonia cases are increasing in 34 provinces, municipalities, and special administrative regions in China. 24,377 cases of 2019-nCoV confirmed in China, including 492 deaths, 3219 serious, and 901 cured. 175 cases of infection were confirmed in 24 other cities around the world. 1.3 Preventive measures To deal with the 2019-nCoV epidemic, the central and local governments of China took a series of drastic measures. First of all, Chinese health authorities conducted an immediate investigation to characterize and control the disease, including isolation of suspected patients, monitoring of contact clinical status of patients, and developing diagnostic and treatment procedures [5]. In addition, on January 23, 2020, the local government of Wuhan announced the suspension of public transportation, closing airports, railway stations, and highways in the city, in order to prevent further disease transmission [10]. Subsequently, most provinces in China claimed “Level I Emergency Response” by taking a series of measures such as suspending public transportation and setting up community quarantines. Particularly, the list of the first-level designated hospitals was announced, and epidemic prevention training for primary medical staff was started. Since fever is one of typical clinical symptoms of the infection, temperature detection of incoming and outgoing personnel was implemented comprehensively in railway stations, passenger stations and other public places, in order to screen suspected cases as early as possible. In addition, many other compulsory measures limiting population mobility, such as cancellation of mass gatherings, school closures, work-from-home arrangements, was taken to reduce within-population contact rates. 2 Epidemiological and clinical symptoms of 2019-nCoV 2.1 Human coronaviruses Human coronaviruses (CoVs) could cause respiratory, gastrointestinal, hepatic and central nervous system diseases. Infection outcomes vary from mild, self-limiting disease, to more severe manifestations and even death [1,11]. Among the seven human CoVs, two α-coronaviruses (HCoV-229E and HCoV-NL63) and two β-coronaviruses (HCoV-OC43 and HCoV-HKU1) were thought to cause only mild self-limiting upper respiratory disease, such as common cold in immunocompetent hosts, except for a few cases of severe infections in infants, children and seniors [12,13]; the other three CoVs could cause fatal respiratory diseases. In 2002, the Severe acute respiratory syndrome coronavirus (SARS-CoV) emerged in Guangdong province, China, spreading to 37 countries, and its subsequent global epidemic was associated with 8096 cases and 774 deaths. Ten years later, Middle-East respiratory syndrome coronavirus (MERS-CoV) spread to 27 countries, causing 2494 infected cases and 858 deaths worldwide [1]. And the recently identified novel coronavirus (2019-nCoV), was the third highly pathogenic CoV discovered, with a mortality of 2% which is much lower than that of SARS-CoV (10%) and MERS-CoV (37%) [6]. However, the transmissibility of 2019-nCoV is higher, the mean R0 (R0 is used to estimate the transmissibility of virus) of 2019-nCoV ranged from 3.3 to 5.5, and it appeared (slightly) higher than those of SARS-CoV (2–5) and MERS-CoV (2.7–3.9) [[14], [15], [16]]. Measures on ‘entry, exit and travel’ screening and restrictions are likely to reduce the effective R0, which should benefit 2019-nCoV control and prevention. 2.2 Clinical symptoms of 2019-nCoV infection The clinical symptoms of 2019-nCoV infection are similar to those of SARS-CoV and MERS-CoV. Most patients present fever, dry cough, dyspnea, and bilateral ground-glass opacities on chest CT scans [4,17,18]. However, patients with 2019-nCoV infection rarely have obvious upper respiratory signs and symptoms (such as snot, sneezing, or sore throat), indicating that the virus primarily infects the lower respiratory tract [4,17]. In addition, about 20–25% of 2019-nCoV patients experience intestinal symptoms and signs (such as diarrhea), similarly to MERS-CoV or SARS-CoV [17]. In severe 2019-nCoV infection cases, the symptoms include acute respiratory distress syndrome, septic shock, metabolic acidosis, and bleeding and coagulation dysfunction. It is worth noting that severe and critically ill patients may have moderate to low fever during the course of the disease, even without obvious fever [3]. Furthermore, like SARS-CoV and MERS-CoV, 2019-nCoV infections induce production of high levels of cytokines [2,17]. The epidemic of 2019-nCoV bears some similarities to SARS-CoV. The outbreaks of the two viruses occurred at about the same time during the year, and they were quite stable in the environment, especially in air-conditioned space, owing to lower ambient temperature and lower humidity [19]. However, SARS-CoV had an aberrant trait that the “viral load” in upper respiratory tract secretions was low in the first 5 days of illness, then increased progressively and peaked early in the second week. Consequently, the transmission rate was relatively low in the first days of illness, providing an opportunity for case detection and isolation to interrupt transmission. On the contrary, for 2019-nCoV, the incubation lasts for an average of 10 days (in a reported range of 2–14 days) from infection to symptoms surfacing [2,4,17,20]. Even worse, 2019-nCoV is able to spread from one person to another even before any actual clinical manifestations, leading to “extremely challenging” conditions for detecting and isolating potential patients, which makes it more difficult to control the epidemic. 2.3 Transmission routes of the disease 2019-nCoV is thought to be transmitted through droplets, close contact, aerosol and maybe fecal-oral transmission, and patients in the incubation period can transmit the virus to other persons [5]. The distribution of the viral receptor can explain the pathogenic mechanisms, clinical manifestations and transmission routes of 2019-nCoV [20,21]. ACE2, a receptor for 2019-nCoV, is necessary for the viral entry of 2019-nCoV. The ubiquitous expression of ACE2 in various cells, such as lung AT2 cells, esophagus upper, stratified epithelial cells, and absorptive enterocytes of ileum and colon may contribute to the multi-tissue infection of 2019-nCoV [22]. Therefore, besides respiratory and bodily contact, fecal-oral transmission is a potential route for 2019-nCoV infection [23,24]. 3 Biological features and origin of the 2019-nCoV 3.1 Genomics of 2019-nCoV According to the genome analysis, 2019-nCoV belongs to Sarbecovirus subgenus, Betacoronavirus genus of the subfamily Orthocoronavirinae in the family Coronaviridae of the suborder cornidovirineae of the order Nidovirales [20,26]. Similarly to other coronaviruses, 2019-nCoV is an enveloped, single-stranded positive-sense RNA (+ssRNA) with around 29,900 nt, showing a typical coronavirus appearance under electron microscopy [20]. The genome organization is 5′-leader-UTR-replicase-S (Spike)-E (Envelope)-M (Membrane)-N (Nucleocapsid)-3′UTRpoly (A) tail with accessory genes interspersed within the structural genes and the 3′ end of the genome [26]. 2019-nCoV shares 79.5% nt identity with SARS-CoV and 96% identity with bat-CoV-RaTG13 [20]. The genetic characteristics of 2019-nCoV are significantly different from SARSr-CoV and SARS-CoV, which are closely related only to the specific bat SARS-like coronaviruses, bat-CoV-RaTG13 and bat-SL-CoVZC45, with 96% and 86.9% nucleotide sequence identities, respectively [20]. Therefore 2019-nCoV is considered as a SARS related coronavirus (SARSr-CoV) by pairwise protein sequence analysis (Fig. 1 ) [20]. Fig. 1 The evolutionary position of 2019-nCoV. The phylogenetic tree was constructed based on the complete genomic sequences of 2019-nCoV and related CoVs by using the neighbor-joining (NJ) method with 1000 bootstrap. The novel 2019-nCoV detected strains were indicated in bold with solid squares. Abbreviations are as follows: SARSr coronavirus, SARS-related coronavirus. The accession numbers of CoVs were showed in the figure. Bootstrap values above 50 were shown. 3.2 Receptor usage of 2019-nCoV The prerequisite of coronaviruses invading the host-cell is to bind to receptors. Following receptor binding, the viral spike protein is cleaved via acid-dependent proteolysis by cathepsin, TMPRRS2 or furin protease, followed by fusion of the viral envelop to the cellular membranes. Spike is a large, clove-shaped trimer, which can be cleaved by proteases into an N-terminal S1 subunit containing the receptor binding domain (RBD) and a C-terminal S2 region. Compared to other proteins of coronavirus, the spike protein has the most variable amino acid sequences which results from the strongest positive selection among all genes of coronavirus to adapt to its hosts [27].The RBD is a major determinant of host range and cross-species transmission. Recently results showed that 2019-nCoV uses the same cell entry receptor, ACE2, as SARS-CoV [21,24,20,28]. Besides human ACE2, 2019-nCoV can use Chinese horseshoe bats, civet, or pig ACE2 but not mouse ACE2 as an entry receptor [24]. One explanation is that human ACE2 has high homology with Chinese horseshoe bats, civet, pig and mouse, which are 80.75%, 83.48%, 81.37%, 82.11% respectively (Table 2 ), while the residues 82 and 353 of mouse ACE2 are different from human ACE2 which are important residues for bind to spike [29]. But the homology of human ACE2 and pangolin ACE2, cat ACE2 are also high with 84.84% and 85.22%. The homology of human ACE2 with other ACE2 varies from 59.73% to 83.23%. In summary, 2019-nCoV may have extensive host ranges. Fig. 2 . Table 2 The amino acid sequence identities of ACE2 among different species. Species Pairwise identities (%) of the amino acid sequences of ACE2 African green monkey Civet Bat (R. sinicus) Swine Malayan pangolin Mouse Cat Camel Goat Bos mutus Erinaceus europaeus Cavia porcellus Phascolarctos cinereus Sarcophilus harrisii Tiger Snake Parus major Gallus gallus Human 93.93 83.48 80.75 81.37 84.84 82.11 85.22 83.23 81.61 81.24 79.01 77.29 71.17 70.51 59.73 67.08 65.31 African green monkey 81.78 79.93 79.93 83.77 81.78 83.15 81.91 81.41 80.92 77.94 76.85 70.38 69.84 59.21 65.6 64.13 Civet 81.78 81.49 82.73 85.59 81.61 93.29 83.85 81.86 81.74 79.75 75.55 71.66 71.63 59.6 67.44 66.3 Bat (R. sinicus) 79.93 81.49 80 82.86 78.26 83.73 81.37 80.37 80 78.51 74.56 69.68 70.02 58.61 65.11 65.19 Swine 79.93 82.73 80 82.61 80.62 83.98 89.81 87.7 87.58 77.64 75.3 71.17 71.01 61.09 66.95 65.56 Malayan pangolin 83.77 85.59 82.86 82.61 82.73 87.33 84.72 82.11 81.86 80.87 77.17 71.66 70.89 59.6 67.44 65.07 Mouse 81.78 81.61 78.26 80.62 82.73 81.74 80.99 80.12 80.37 78.63 78.16 71.05 71.13 61.21 67.2 66.17 Cat 83.15 93.29 83.73 83.98 87.33 81.74 85.71 83.23 83.11 81.49 76.42 72.65 72.25 59.6 67.81 66.79 Camel 81.91 83.85 81.37 89.81 84.72 80.99 85.71 88.2 88.57 79.25 77.29 71.79 72.12 61.34 67.2 66.42 Goat 81.41 81.86 80.37 87.7 82.11 80.12 83.23 88.2 97.76 78.14 76.54 72.04 71.38 60.84 66.09 65.44 Bos mutus 80.92 81.74 80 87.58 81.86 80.37 83.11 88.57 97.76 78.39 77.04 71.42 71.01 61.09 66.09 65.31 Erinaceus europaeus 77.94 79.75 78.51 77.64 80.87 78.63 81.49 79.25 78.14 78.39 74.56 70.67 69.65 60.59 68.8 66.42 Cavia porcellus 76.85 75.55 74.56 75.3 77.17 78.16 76.42 77.29 76.54 77.04 74.56 66.61 66.82 59.38 63.9 63.12 Phascolarctos cinereus 70.38 71.66 69.68 71.17 71.66 71.05 72.65 71.79 72.04 71.42 70.67 66.61 81.92 60.7 67.08 64.83 Sarcophilus harrisii 69.84 71.63 70.02 71.01 70.89 71.13 72.25 72.12 71.38 71.01 69.65 66.82 81.92 60.9 64.62 63.84 Tiger Snake 59.21 59.6 58.61 61.09 59.6 61.21 59.6 61.34 60.84 61.09 60.59 59.38 60.7 60.9 64.5 63.71 Parus major 65.6 67.44 65.11 66.95 67.44 67.2 67.81 67.2 66.09 66.09 68.8 63.9 67.08 64.62 64.5 82.2 Gallus gallus 64.13 66.3 65.19 65.56 65.07 66.17 66.79 66.42 65.44 65.31 66.42 63.12 64.83 63.84 63.71 82.2 Accession numbers: human (NP_068576.1), monkey (AY996037.1), civet (AAX63775.1), bat (R.sinicus) (AGZ48803.1), swine (NP_001116542.1), Malayan pangolin (XP_017505752.1), mouse (NP_001123985.1), cat (NP_001034545.1), camel (XP_010991717.1), goat (NP_001277036.1), Bos mutus (XP_005903173.1), Erinaceus europaeus (XP_007538670.1), Phascolarctos cinereus (XP_020863153.1), Sarcophilus harrisii (XP_031814825.1), tiger Snake (XP_026530754.1), Parus major (XP_015486815.1), Gallus gallus (QEQ50331.1). Fig. 2 Phylogenetic analysis of ACE2 from humans and 18 other animals. The tree was constructed based on the complete amino acid sequences of ACE2s derived from humans and other animals by using the neighbor-joining (NJ) method with 1000 bootstrap. The accession numbers were as the same as Table 2. Bootstrap values above 50 were shown. 3.3 Origin of 2019-nCoV The epidemiological investigation of early cases of 2019-nCoV pneumonia showed that many cases had been exposed to the Huanan Seafood Market in Wuhan [2,24,20]. In addition, 33 of the 585 environmental samples from Huanan Seafood Market were positive for 2019-nCoV; however, that market was trading a variety of live animals, such as hedgehog, badger, snake, and bird (turtledoves), probably pangolin, but not bats [24,30]. Thus, bats are less likely to have direct contact with human, and direct transmission of the virus from bat to human is unlikely [1]. Indeed, SARS-CoV and MERS-CoV originated from bats, the natural reservoir, but transmitted to humans via intermediate host civets and camels, respectively. Therefore, 2019-nCoV may also originate from bat but was then transmitted to humans via an intermediate host in the market [[31], [32], [33], [34], [35]]. Currently, 2019-nCoV has been isolated from pangolins and it was found that the genomic seuqence of the isolated strain had 99% identity with that of currently 2019-nCoV infected humans. It has been proposed that the transmission and evolution path of 2019-nCoV was from bat-CoV to pangolins (the intermediate hosts), from where it infected humans [36]. 4 Diagnosis and treatment Diagnosis of coronaviruses is unnecessary in most cases of self-limited infection, as most of the patients have mild or moderate syndromes with good prognosis. However, it may be essential to identify an etiological agent in epidemiological studies, especially during the epidemics outbreak. Since 2019-nCoV has not been found before in humans, there is no vaccine or special treatment for it so far. The number of cases is increasing rapidly, even under the current public health emergency. Thus, diagnosing all suspected cases as soon as possible and immediately isolating them is critical to cutting off the source of infection. Conventional diagnostic testing methods, such as the assays for detection of antiviral antibodies or viral antigens have been developed and used clinically. New diagnostic strategies including multiplex nucleic acid amplification and microarray-based assays may help in epidemiologic monitoring, along with taking effective prevention steps [37,38]. 2019-nCoV nucleic acids can be detected in specimens including nasopharyngeal swabs, sputum, lower respiratory tract secretions, blood and stool [20]. There are currently no approved antiviral treatments or vaccines for human coronavirus infections. The development of safe, stable vaccines is a huge challenge and vaccines would ideally be broad-spectrum, so the research and development of new drugs is a very long process. In such a sudden epidemic, scientists were not able to carry out new drug development by the traditional principles. Therefore, there is another option: a systematic and large-scale screening of existing drugs to see whether they have activity against the 2019-nCoV. Recently drug screening found that nelfinavir has potential antiviral activity against 2019-nCoV. In addition, pitavastatin, perampanel, and praziquantel might also have moderate activities against 2019-nCoV [39]. Based on previous studies, an anti-HIV drug named Kaletra may have therapeutic effect on SARS and MERS, which is composed of two protease inhibitors, ritonavir (CAS#: 155,213-67-5) and lopinavir (CAS#: 192,725-17-0) [[40], [41], [42], [43], [44]]. More recently, Kaletra was also recommended to treat Wuhan pneumonia by the National Health Commission of the People’s Republic of China. Patients with SARS or MERS have used several drugs including ribavirin, interferon, lopinavir-ritonavir, and corticosteroids, but the efficacy of certain drugs is still controversial. Other antiviral drugs, like FAD-approved drugs including ribavirin, penciclovir, nitrazine, nalfamusta, chloroquine, and the two broad-spectrum antiviral drugs redexivir (GS-5734) and favivir (T-705), are being evaluated by measuring the effects of these compounds on cytotoxicity, virus yield and infection rate of 2019-nCoV. Recent results showed that remdesivir and chloroquine are effective in controlling 2019-nCoV infection in vitro and may be evaluated in human patients with 2019-nCoV disease [45]. Remdesivir has recently been recognized as a promising antiviral drug against multiple RNA viruses (including SARS/MERS-CoV) in cultured cells, and in mouse and non-human primate (NHP) models. Currently remdesivir for the treatment of Ebola virus infection is in clinical research. It is promising that these compounds can treat 2019-nCoV infections. Moreover, the fifth edition of infection prevention and control (IPC) guidance announced that severe and critically ill patients could be treated with recovery plasma. 5 Conclusions and future perspectives The 2019-nCoV epidemic continues. During the past month, huge progress has been made in pathogen monitoring, identifying sources, basic etiology, clinical treatment, drug testing, and vaccine development. With the efforts of China and the helps of other countries in the world, we hope that the epidemic will subside soon. Future research on viral replication, pathogenesis, antiviral drugs and other aspects of 2019-nCoV will contribute to the treatment and prevention of the virus. However, given the emergence of 2019-nCoV pneumonia as a new infectious disease with interspecies transmission from animals, we should reflect on the origin of the human pathogen and learn from our experience. With changes of ecology and human activities, including unlimited invasion of natural habitats of animals, consumption of some of these animals, and modern agricultural practices, the spillover of viruses from natural hosts to humans continues to appear and may even be escalating. Although we have built an unparalleled human civilization and a highly developed information society, invisible viruses can still have devastating effects on human beings. The high lethality viruses such as SARS-CoV, MERS-CoV, H5N1, H7N9, Ebola and emerging 2019-nCoV should serve as an alarm to the world. We should strive to reduce the probability of their occurrence and harm. Strengthening the investigation of animal etiology, normalizing the storage of high risk pathogens, reducing direct contact with wildlife, maintaining the barriers between natural reservoirs and human society and completely eradicating wildlife trading will help to achieve these goals. Declaration of Competing Interest The authors declare that they have no conflict of interest. |
p | In December 2019, a novel coronavirus was detected in pneumonia patients which was later named 2019-nCoV. Since the first human coronavirus detected in the 1960s, 2019-nCoV is the seventh coronavirus that is known to infect humans [1]. Among all the human coronaviruses, four of them, including NL-63, 229E, HKU1 and OC43, cause mild illness, while the other three, SARS-CoV, MERS-CoV and 2019-nCoV, lead to serious disease [2]. To characterize the new pathogen causing acute infection in humans, researchers have conducted urgent studies on the 2019-nCoV, from its epidemiology and clinical medicine to etiology and virology. This review focuses on the epidemiology and clinical manifestations of 2019-nCoV, the diagnosis and treatment of infected patients, and in particular, evidence regarding the origin of 2019-nCoV. |
sec | 1 Emergence and epidemic of pneumonias caused by 2019-nCoV 1.1 Pathogen identification and transmission of pneumonia On 31th December 2019, the first pneumonia case of unknown etiology was reported in Wuhan City, Hubei Province, China. In order to identify the etiology, a number of possible etiological agents were ruled out, including the Severe acute respiratory syndrome coronavirus (SARS-CoV), the Middle-East respiratory syndrome coronavirus (MERS-CoV), avian influenza virus, and other common respiratory pathogens. Finally, a new coronavirus, temporally named 2019-nCoV, was identified to be the pathogen responsible for disease, and it was announced by World Health Organization (WHO) on 12th January [3]. As of January 11, 2020, 41 pneumonia cases were diagnosed, including seven severe cases and one death [2]. At that time, there was no evidence of person-to-person transmission according to the scientific literature. The first “super-spreading” event became a hallmark of the epidemiology of the disease. On January 20, 2020, in epidemiological investigations, two local infections in patients who were infected by 2019-nCoV without physical visits to Wuhan were reported in Guangdong Province, which confirmed the occurrence of human-to-human transmission [4]. Then, 14 medical staff infected by 2019-nCoV from patients further confirmed the human-to-human contagion [5]. On 21th January 2020, WHO announced that 2019-nCoV was possibly sustained through human-to-human transmission, warning the public to take self-protection measures [3]. The first of many cases, this disease was rapidly shown to cause an epidemic [6]. Due to the high transmissibility of 2019-nCoV, the Chinese government has taken quick action and various measures to deal with the epidemic, trying to prevent spread of the outbreak and to treat patients. Researchers put much effort to understand the origin and pathophysiology of this novel coronavirus, and have been testing multiple drugs to screen effective therapy. 1.2 Current situation of the epidemic Until February 6, 2020, confirmed 2019-nCoV pneumonia cases were increasing with worrisome speed in China. As of 5th February, there were 24,377 cases confirmed in mainland China, including 492 deaths, 3219 severe cases, and 901 cured cases [6]. The fatality rate was about 2%. In addition, 175 cases of 2019-nCoV infection were confirmed in 24 other countries around the world. The typical events during the 2019-nCoV outbreak are summarized in Table 1 . The period from the end of December 2019 to January 2020 was the “Spring Festival travel rush” time in China. Although the government issued an order to control the flow of people, the disease spread rapidly to other cities because of asymptomatic carriers [[7], [8], [9]]. Table 1 Key events of 2019-nCoV. Date Key events 31 December 2019 The Health Commission of Hubei province, China, first announced a cluster of unexplained cases of pneumonia. 3 January 2020 A total of 44 patients (11 are severely ill, while the remaining 33 patients are in stable condition.) with pneumonia of unknown etiology have been reported to WHO by the national authorities in China. 5 January 2020 The WHO published a document detailing 44 patients had “pneumonia of unknown etiology”, with 121 close contacts under surveillance. 8 January 2020 The genetic sequencing demonstrated that the novel coronavirus is the potential causative organism. 11 January 2020 41 patients have been diagnosed to have infection of the novel coronavirus, with 763 close contacts, 7 severe cases and the first death. 12 January 2020 The virus was tentatively named by WHO as the 2019 novel coronavirus (2019-nCoV). 13 January 2020 The first imported case of 2019-nCoV was found in Thailand. This is the first infected person diagnosed outside China. The patient is a 61-year-old Chinese woman living in Wuhan City, Hubei Province, China. 16 January 2020 The first case reported in Japan. This is the second confirmed case of 2019-nCoV that has been detected outside of China. The person travelled to Wuhan, China. 19 January 2020 The first case reported in Korea. The patient was a Chinese woman who flew from Wuhan to Incheon International Airport. Four other suspected patients were being examined. Furthermore, the first case reported in Shenzhen, China. 20 January 2020 Two local infections in the Chinese province of Guangdong with no direct visit to Wuhan were the first confirmed the occurrence of human-to-human transmission through epidemiological investigation. And the first case reported in Shanghai, China. 21 January 2020 15 health-care workers infected with the 2019-nCoV in Wuhan. Zhejiang Province, Henan Province, Sichuan Province and Taiwa confirmed the first case of 2019-nCoV, respectively. The WHO announced that there was possible sustained human to-human transmission. 22–23 January 2020 17 death in Hubei Province. America and Macao and Many provinces confirmed the first case of 2019-nCoV. Such as Shandong Province, Yunnan Province, Hunan Province, America, Macao, Anhui Province, Fujian Province, Guizhou Province, Shanxi Province, Ningxia Autonomous Region and Hebei Province reported the first case of 2019-nCoV, respectively. 23 January 2020 the local government in Wuhan announced the suspension of public transportation, with closure of airports, railway stations, and highways 24 January 2020 Zhejiang and Shanghai’s first patients diagnosed with 2019-nCoV recover. 5 February 2020 The confirmed pneumonia cases are increasing in 34 provinces, municipalities, and special administrative regions in China. 24,377 cases of 2019-nCoV confirmed in China, including 492 deaths, 3219 serious, and 901 cured. 175 cases of infection were confirmed in 24 other cities around the world. 1.3 Preventive measures To deal with the 2019-nCoV epidemic, the central and local governments of China took a series of drastic measures. First of all, Chinese health authorities conducted an immediate investigation to characterize and control the disease, including isolation of suspected patients, monitoring of contact clinical status of patients, and developing diagnostic and treatment procedures [5]. In addition, on January 23, 2020, the local government of Wuhan announced the suspension of public transportation, closing airports, railway stations, and highways in the city, in order to prevent further disease transmission [10]. Subsequently, most provinces in China claimed “Level I Emergency Response” by taking a series of measures such as suspending public transportation and setting up community quarantines. Particularly, the list of the first-level designated hospitals was announced, and epidemic prevention training for primary medical staff was started. Since fever is one of typical clinical symptoms of the infection, temperature detection of incoming and outgoing personnel was implemented comprehensively in railway stations, passenger stations and other public places, in order to screen suspected cases as early as possible. In addition, many other compulsory measures limiting population mobility, such as cancellation of mass gatherings, school closures, work-from-home arrangements, was taken to reduce within-population contact rates. |
label | 1 |
title | Emergence and epidemic of pneumonias caused by 2019-nCoV |
sec | 1.1 Pathogen identification and transmission of pneumonia On 31th December 2019, the first pneumonia case of unknown etiology was reported in Wuhan City, Hubei Province, China. In order to identify the etiology, a number of possible etiological agents were ruled out, including the Severe acute respiratory syndrome coronavirus (SARS-CoV), the Middle-East respiratory syndrome coronavirus (MERS-CoV), avian influenza virus, and other common respiratory pathogens. Finally, a new coronavirus, temporally named 2019-nCoV, was identified to be the pathogen responsible for disease, and it was announced by World Health Organization (WHO) on 12th January [3]. As of January 11, 2020, 41 pneumonia cases were diagnosed, including seven severe cases and one death [2]. At that time, there was no evidence of person-to-person transmission according to the scientific literature. The first “super-spreading” event became a hallmark of the epidemiology of the disease. On January 20, 2020, in epidemiological investigations, two local infections in patients who were infected by 2019-nCoV without physical visits to Wuhan were reported in Guangdong Province, which confirmed the occurrence of human-to-human transmission [4]. Then, 14 medical staff infected by 2019-nCoV from patients further confirmed the human-to-human contagion [5]. On 21th January 2020, WHO announced that 2019-nCoV was possibly sustained through human-to-human transmission, warning the public to take self-protection measures [3]. The first of many cases, this disease was rapidly shown to cause an epidemic [6]. Due to the high transmissibility of 2019-nCoV, the Chinese government has taken quick action and various measures to deal with the epidemic, trying to prevent spread of the outbreak and to treat patients. Researchers put much effort to understand the origin and pathophysiology of this novel coronavirus, and have been testing multiple drugs to screen effective therapy. |
label | 1.1 |
title | Pathogen identification and transmission of pneumonia |
p | On 31th December 2019, the first pneumonia case of unknown etiology was reported in Wuhan City, Hubei Province, China. In order to identify the etiology, a number of possible etiological agents were ruled out, including the Severe acute respiratory syndrome coronavirus (SARS-CoV), the Middle-East respiratory syndrome coronavirus (MERS-CoV), avian influenza virus, and other common respiratory pathogens. Finally, a new coronavirus, temporally named 2019-nCoV, was identified to be the pathogen responsible for disease, and it was announced by World Health Organization (WHO) on 12th January [3]. As of January 11, 2020, 41 pneumonia cases were diagnosed, including seven severe cases and one death [2]. At that time, there was no evidence of person-to-person transmission according to the scientific literature. The first “super-spreading” event became a hallmark of the epidemiology of the disease. On January 20, 2020, in epidemiological investigations, two local infections in patients who were infected by 2019-nCoV without physical visits to Wuhan were reported in Guangdong Province, which confirmed the occurrence of human-to-human transmission [4]. Then, 14 medical staff infected by 2019-nCoV from patients further confirmed the human-to-human contagion [5]. On 21th January 2020, WHO announced that 2019-nCoV was possibly sustained through human-to-human transmission, warning the public to take self-protection measures [3]. The first of many cases, this disease was rapidly shown to cause an epidemic [6]. Due to the high transmissibility of 2019-nCoV, the Chinese government has taken quick action and various measures to deal with the epidemic, trying to prevent spread of the outbreak and to treat patients. Researchers put much effort to understand the origin and pathophysiology of this novel coronavirus, and have been testing multiple drugs to screen effective therapy. |
sec | 1.2 Current situation of the epidemic Until February 6, 2020, confirmed 2019-nCoV pneumonia cases were increasing with worrisome speed in China. As of 5th February, there were 24,377 cases confirmed in mainland China, including 492 deaths, 3219 severe cases, and 901 cured cases [6]. The fatality rate was about 2%. In addition, 175 cases of 2019-nCoV infection were confirmed in 24 other countries around the world. The typical events during the 2019-nCoV outbreak are summarized in Table 1 . The period from the end of December 2019 to January 2020 was the “Spring Festival travel rush” time in China. Although the government issued an order to control the flow of people, the disease spread rapidly to other cities because of asymptomatic carriers [[7], [8], [9]]. Table 1 Key events of 2019-nCoV. Date Key events 31 December 2019 The Health Commission of Hubei province, China, first announced a cluster of unexplained cases of pneumonia. 3 January 2020 A total of 44 patients (11 are severely ill, while the remaining 33 patients are in stable condition.) with pneumonia of unknown etiology have been reported to WHO by the national authorities in China. 5 January 2020 The WHO published a document detailing 44 patients had “pneumonia of unknown etiology”, with 121 close contacts under surveillance. 8 January 2020 The genetic sequencing demonstrated that the novel coronavirus is the potential causative organism. 11 January 2020 41 patients have been diagnosed to have infection of the novel coronavirus, with 763 close contacts, 7 severe cases and the first death. 12 January 2020 The virus was tentatively named by WHO as the 2019 novel coronavirus (2019-nCoV). 13 January 2020 The first imported case of 2019-nCoV was found in Thailand. This is the first infected person diagnosed outside China. The patient is a 61-year-old Chinese woman living in Wuhan City, Hubei Province, China. 16 January 2020 The first case reported in Japan. This is the second confirmed case of 2019-nCoV that has been detected outside of China. The person travelled to Wuhan, China. 19 January 2020 The first case reported in Korea. The patient was a Chinese woman who flew from Wuhan to Incheon International Airport. Four other suspected patients were being examined. Furthermore, the first case reported in Shenzhen, China. 20 January 2020 Two local infections in the Chinese province of Guangdong with no direct visit to Wuhan were the first confirmed the occurrence of human-to-human transmission through epidemiological investigation. And the first case reported in Shanghai, China. 21 January 2020 15 health-care workers infected with the 2019-nCoV in Wuhan. Zhejiang Province, Henan Province, Sichuan Province and Taiwa confirmed the first case of 2019-nCoV, respectively. The WHO announced that there was possible sustained human to-human transmission. 22–23 January 2020 17 death in Hubei Province. America and Macao and Many provinces confirmed the first case of 2019-nCoV. Such as Shandong Province, Yunnan Province, Hunan Province, America, Macao, Anhui Province, Fujian Province, Guizhou Province, Shanxi Province, Ningxia Autonomous Region and Hebei Province reported the first case of 2019-nCoV, respectively. 23 January 2020 the local government in Wuhan announced the suspension of public transportation, with closure of airports, railway stations, and highways 24 January 2020 Zhejiang and Shanghai’s first patients diagnosed with 2019-nCoV recover. 5 February 2020 The confirmed pneumonia cases are increasing in 34 provinces, municipalities, and special administrative regions in China. 24,377 cases of 2019-nCoV confirmed in China, including 492 deaths, 3219 serious, and 901 cured. 175 cases of infection were confirmed in 24 other cities around the world. |
label | 1.2 |
title | Current situation of the epidemic |
p | Until February 6, 2020, confirmed 2019-nCoV pneumonia cases were increasing with worrisome speed in China. As of 5th February, there were 24,377 cases confirmed in mainland China, including 492 deaths, 3219 severe cases, and 901 cured cases [6]. The fatality rate was about 2%. In addition, 175 cases of 2019-nCoV infection were confirmed in 24 other countries around the world. The typical events during the 2019-nCoV outbreak are summarized in Table 1 . The period from the end of December 2019 to January 2020 was the “Spring Festival travel rush” time in China. Although the government issued an order to control the flow of people, the disease spread rapidly to other cities because of asymptomatic carriers [[7], [8], [9]]. Table 1 Key events of 2019-nCoV. Date Key events 31 December 2019 The Health Commission of Hubei province, China, first announced a cluster of unexplained cases of pneumonia. 3 January 2020 A total of 44 patients (11 are severely ill, while the remaining 33 patients are in stable condition.) with pneumonia of unknown etiology have been reported to WHO by the national authorities in China. 5 January 2020 The WHO published a document detailing 44 patients had “pneumonia of unknown etiology”, with 121 close contacts under surveillance. 8 January 2020 The genetic sequencing demonstrated that the novel coronavirus is the potential causative organism. 11 January 2020 41 patients have been diagnosed to have infection of the novel coronavirus, with 763 close contacts, 7 severe cases and the first death. 12 January 2020 The virus was tentatively named by WHO as the 2019 novel coronavirus (2019-nCoV). 13 January 2020 The first imported case of 2019-nCoV was found in Thailand. This is the first infected person diagnosed outside China. The patient is a 61-year-old Chinese woman living in Wuhan City, Hubei Province, China. 16 January 2020 The first case reported in Japan. This is the second confirmed case of 2019-nCoV that has been detected outside of China. The person travelled to Wuhan, China. 19 January 2020 The first case reported in Korea. The patient was a Chinese woman who flew from Wuhan to Incheon International Airport. Four other suspected patients were being examined. Furthermore, the first case reported in Shenzhen, China. 20 January 2020 Two local infections in the Chinese province of Guangdong with no direct visit to Wuhan were the first confirmed the occurrence of human-to-human transmission through epidemiological investigation. And the first case reported in Shanghai, China. 21 January 2020 15 health-care workers infected with the 2019-nCoV in Wuhan. Zhejiang Province, Henan Province, Sichuan Province and Taiwa confirmed the first case of 2019-nCoV, respectively. The WHO announced that there was possible sustained human to-human transmission. 22–23 January 2020 17 death in Hubei Province. America and Macao and Many provinces confirmed the first case of 2019-nCoV. Such as Shandong Province, Yunnan Province, Hunan Province, America, Macao, Anhui Province, Fujian Province, Guizhou Province, Shanxi Province, Ningxia Autonomous Region and Hebei Province reported the first case of 2019-nCoV, respectively. 23 January 2020 the local government in Wuhan announced the suspension of public transportation, with closure of airports, railway stations, and highways 24 January 2020 Zhejiang and Shanghai’s first patients diagnosed with 2019-nCoV recover. 5 February 2020 The confirmed pneumonia cases are increasing in 34 provinces, municipalities, and special administrative regions in China. 24,377 cases of 2019-nCoV confirmed in China, including 492 deaths, 3219 serious, and 901 cured. 175 cases of infection were confirmed in 24 other cities around the world. |
table-wrap | Table 1 Key events of 2019-nCoV. Date Key events 31 December 2019 The Health Commission of Hubei province, China, first announced a cluster of unexplained cases of pneumonia. 3 January 2020 A total of 44 patients (11 are severely ill, while the remaining 33 patients are in stable condition.) with pneumonia of unknown etiology have been reported to WHO by the national authorities in China. 5 January 2020 The WHO published a document detailing 44 patients had “pneumonia of unknown etiology”, with 121 close contacts under surveillance. 8 January 2020 The genetic sequencing demonstrated that the novel coronavirus is the potential causative organism. 11 January 2020 41 patients have been diagnosed to have infection of the novel coronavirus, with 763 close contacts, 7 severe cases and the first death. 12 January 2020 The virus was tentatively named by WHO as the 2019 novel coronavirus (2019-nCoV). 13 January 2020 The first imported case of 2019-nCoV was found in Thailand. This is the first infected person diagnosed outside China. The patient is a 61-year-old Chinese woman living in Wuhan City, Hubei Province, China. 16 January 2020 The first case reported in Japan. This is the second confirmed case of 2019-nCoV that has been detected outside of China. The person travelled to Wuhan, China. 19 January 2020 The first case reported in Korea. The patient was a Chinese woman who flew from Wuhan to Incheon International Airport. Four other suspected patients were being examined. Furthermore, the first case reported in Shenzhen, China. 20 January 2020 Two local infections in the Chinese province of Guangdong with no direct visit to Wuhan were the first confirmed the occurrence of human-to-human transmission through epidemiological investigation. And the first case reported in Shanghai, China. 21 January 2020 15 health-care workers infected with the 2019-nCoV in Wuhan. Zhejiang Province, Henan Province, Sichuan Province and Taiwa confirmed the first case of 2019-nCoV, respectively. The WHO announced that there was possible sustained human to-human transmission. 22–23 January 2020 17 death in Hubei Province. America and Macao and Many provinces confirmed the first case of 2019-nCoV. Such as Shandong Province, Yunnan Province, Hunan Province, America, Macao, Anhui Province, Fujian Province, Guizhou Province, Shanxi Province, Ningxia Autonomous Region and Hebei Province reported the first case of 2019-nCoV, respectively. 23 January 2020 the local government in Wuhan announced the suspension of public transportation, with closure of airports, railway stations, and highways 24 January 2020 Zhejiang and Shanghai’s first patients diagnosed with 2019-nCoV recover. 5 February 2020 The confirmed pneumonia cases are increasing in 34 provinces, municipalities, and special administrative regions in China. 24,377 cases of 2019-nCoV confirmed in China, including 492 deaths, 3219 serious, and 901 cured. 175 cases of infection were confirmed in 24 other cities around the world. |
label | Table 1 |
caption | Key events of 2019-nCoV. |
p | Key events of 2019-nCoV. |
table | Date Key events 31 December 2019 The Health Commission of Hubei province, China, first announced a cluster of unexplained cases of pneumonia. 3 January 2020 A total of 44 patients (11 are severely ill, while the remaining 33 patients are in stable condition.) with pneumonia of unknown etiology have been reported to WHO by the national authorities in China. 5 January 2020 The WHO published a document detailing 44 patients had “pneumonia of unknown etiology”, with 121 close contacts under surveillance. 8 January 2020 The genetic sequencing demonstrated that the novel coronavirus is the potential causative organism. 11 January 2020 41 patients have been diagnosed to have infection of the novel coronavirus, with 763 close contacts, 7 severe cases and the first death. 12 January 2020 The virus was tentatively named by WHO as the 2019 novel coronavirus (2019-nCoV). 13 January 2020 The first imported case of 2019-nCoV was found in Thailand. This is the first infected person diagnosed outside China. The patient is a 61-year-old Chinese woman living in Wuhan City, Hubei Province, China. 16 January 2020 The first case reported in Japan. This is the second confirmed case of 2019-nCoV that has been detected outside of China. The person travelled to Wuhan, China. 19 January 2020 The first case reported in Korea. The patient was a Chinese woman who flew from Wuhan to Incheon International Airport. Four other suspected patients were being examined. Furthermore, the first case reported in Shenzhen, China. 20 January 2020 Two local infections in the Chinese province of Guangdong with no direct visit to Wuhan were the first confirmed the occurrence of human-to-human transmission through epidemiological investigation. And the first case reported in Shanghai, China. 21 January 2020 15 health-care workers infected with the 2019-nCoV in Wuhan. Zhejiang Province, Henan Province, Sichuan Province and Taiwa confirmed the first case of 2019-nCoV, respectively. The WHO announced that there was possible sustained human to-human transmission. 22–23 January 2020 17 death in Hubei Province. America and Macao and Many provinces confirmed the first case of 2019-nCoV. Such as Shandong Province, Yunnan Province, Hunan Province, America, Macao, Anhui Province, Fujian Province, Guizhou Province, Shanxi Province, Ningxia Autonomous Region and Hebei Province reported the first case of 2019-nCoV, respectively. 23 January 2020 the local government in Wuhan announced the suspension of public transportation, with closure of airports, railway stations, and highways 24 January 2020 Zhejiang and Shanghai’s first patients diagnosed with 2019-nCoV recover. 5 February 2020 The confirmed pneumonia cases are increasing in 34 provinces, municipalities, and special administrative regions in China. 24,377 cases of 2019-nCoV confirmed in China, including 492 deaths, 3219 serious, and 901 cured. 175 cases of infection were confirmed in 24 other cities around the world. |
tr | Date Key events |
th | Date |
th | Key events |
tr | 31 December 2019 The Health Commission of Hubei province, China, first announced a cluster of unexplained cases of pneumonia. |
td | 31 December 2019 |
td | The Health Commission of Hubei province, China, first announced a cluster of unexplained cases of pneumonia. |
tr | 3 January 2020 A total of 44 patients (11 are severely ill, while the remaining 33 patients are in stable condition.) with pneumonia of unknown etiology have been reported to WHO by the national authorities in China. |
td | 3 January 2020 |
td | A total of 44 patients (11 are severely ill, while the remaining 33 patients are in stable condition.) with pneumonia of unknown etiology have been reported to WHO by the national authorities in China. |
tr | 5 January 2020 The WHO published a document detailing 44 patients had “pneumonia of unknown etiology”, with 121 close contacts under surveillance. |
td | 5 January 2020 |
td | The WHO published a document detailing 44 patients had “pneumonia of unknown etiology”, with 121 close contacts under surveillance. |
tr | 8 January 2020 The genetic sequencing demonstrated that the novel coronavirus is the potential causative organism. |
td | 8 January 2020 |
td | The genetic sequencing demonstrated that the novel coronavirus is the potential causative organism. |
tr | 11 January 2020 41 patients have been diagnosed to have infection of the novel coronavirus, with 763 close contacts, 7 severe cases and the first death. |
td | 11 January 2020 |
td | 41 patients have been diagnosed to have infection of the novel coronavirus, with 763 close contacts, 7 severe cases and the first death. |
tr | 12 January 2020 The virus was tentatively named by WHO as the 2019 novel coronavirus (2019-nCoV). |
td | 12 January 2020 |
td | The virus was tentatively named by WHO as the 2019 novel coronavirus (2019-nCoV). |
tr | 13 January 2020 The first imported case of 2019-nCoV was found in Thailand. This is the first infected person diagnosed outside China. The patient is a 61-year-old Chinese woman living in Wuhan City, Hubei Province, China. |
td | 13 January 2020 |
td | The first imported case of 2019-nCoV was found in Thailand. This is the first infected person diagnosed outside China. The patient is a 61-year-old Chinese woman living in Wuhan City, Hubei Province, China. |
tr | 16 January 2020 The first case reported in Japan. This is the second confirmed case of 2019-nCoV that has been detected outside of China. The person travelled to Wuhan, China. |
td | 16 January 2020 |
td | The first case reported in Japan. This is the second confirmed case of 2019-nCoV that has been detected outside of China. The person travelled to Wuhan, China. |
tr | 19 January 2020 The first case reported in Korea. The patient was a Chinese woman who flew from Wuhan to Incheon International Airport. Four other suspected patients were being examined. Furthermore, the first case reported in Shenzhen, China. |
td | 19 January 2020 |
td | The first case reported in Korea. The patient was a Chinese woman who flew from Wuhan to Incheon International Airport. Four other suspected patients were being examined. Furthermore, the first case reported in Shenzhen, China. |
tr | 20 January 2020 Two local infections in the Chinese province of Guangdong with no direct visit to Wuhan were the first confirmed the occurrence of human-to-human transmission through epidemiological investigation. And the first case reported in Shanghai, China. |
td | 20 January 2020 |
td | Two local infections in the Chinese province of Guangdong with no direct visit to Wuhan were the first confirmed the occurrence of human-to-human transmission through epidemiological investigation. And the first case reported in Shanghai, China. |
tr | 21 January 2020 15 health-care workers infected with the 2019-nCoV in Wuhan. Zhejiang Province, Henan Province, Sichuan Province and Taiwa confirmed the first case of 2019-nCoV, respectively. The WHO announced that there was possible sustained human to-human transmission. |
td | 21 January 2020 |
td | 15 health-care workers infected with the 2019-nCoV in Wuhan. Zhejiang Province, Henan Province, Sichuan Province and Taiwa confirmed the first case of 2019-nCoV, respectively. The WHO announced that there was possible sustained human to-human transmission. |
tr | 22–23 January 2020 17 death in Hubei Province. America and Macao and Many provinces confirmed the first case of 2019-nCoV. Such as Shandong Province, Yunnan Province, Hunan Province, America, Macao, Anhui Province, Fujian Province, Guizhou Province, Shanxi Province, Ningxia Autonomous Region and Hebei Province reported the first case of 2019-nCoV, respectively. |
td | 22–23 January 2020 |
td | 17 death in Hubei Province. America and Macao and Many provinces confirmed the first case of 2019-nCoV. Such as Shandong Province, Yunnan Province, Hunan Province, America, Macao, Anhui Province, Fujian Province, Guizhou Province, Shanxi Province, Ningxia Autonomous Region and Hebei Province reported the first case of 2019-nCoV, respectively. |
tr | 23 January 2020 the local government in Wuhan announced the suspension of public transportation, with closure of airports, railway stations, and highways |
td | 23 January 2020 |
td | the local government in Wuhan announced the suspension of public transportation, with closure of airports, railway stations, and highways |
tr | 24 January 2020 Zhejiang and Shanghai’s first patients diagnosed with 2019-nCoV recover. |
td | 24 January 2020 |
td | Zhejiang and Shanghai’s first patients diagnosed with 2019-nCoV recover. |
tr | 5 February 2020 The confirmed pneumonia cases are increasing in 34 provinces, municipalities, and special administrative regions in China. 24,377 cases of 2019-nCoV confirmed in China, including 492 deaths, 3219 serious, and 901 cured. 175 cases of infection were confirmed in 24 other cities around the world. |
td | 5 February 2020 |
td | The confirmed pneumonia cases are increasing in 34 provinces, municipalities, and special administrative regions in China. 24,377 cases of 2019-nCoV confirmed in China, including 492 deaths, 3219 serious, and 901 cured. 175 cases of infection were confirmed in 24 other cities around the world. |
sec | 1.3 Preventive measures To deal with the 2019-nCoV epidemic, the central and local governments of China took a series of drastic measures. First of all, Chinese health authorities conducted an immediate investigation to characterize and control the disease, including isolation of suspected patients, monitoring of contact clinical status of patients, and developing diagnostic and treatment procedures [5]. In addition, on January 23, 2020, the local government of Wuhan announced the suspension of public transportation, closing airports, railway stations, and highways in the city, in order to prevent further disease transmission [10]. Subsequently, most provinces in China claimed “Level I Emergency Response” by taking a series of measures such as suspending public transportation and setting up community quarantines. Particularly, the list of the first-level designated hospitals was announced, and epidemic prevention training for primary medical staff was started. Since fever is one of typical clinical symptoms of the infection, temperature detection of incoming and outgoing personnel was implemented comprehensively in railway stations, passenger stations and other public places, in order to screen suspected cases as early as possible. In addition, many other compulsory measures limiting population mobility, such as cancellation of mass gatherings, school closures, work-from-home arrangements, was taken to reduce within-population contact rates. |
label | 1.3 |
title | Preventive measures |
p | To deal with the 2019-nCoV epidemic, the central and local governments of China took a series of drastic measures. First of all, Chinese health authorities conducted an immediate investigation to characterize and control the disease, including isolation of suspected patients, monitoring of contact clinical status of patients, and developing diagnostic and treatment procedures [5]. In addition, on January 23, 2020, the local government of Wuhan announced the suspension of public transportation, closing airports, railway stations, and highways in the city, in order to prevent further disease transmission [10]. Subsequently, most provinces in China claimed “Level I Emergency Response” by taking a series of measures such as suspending public transportation and setting up community quarantines. Particularly, the list of the first-level designated hospitals was announced, and epidemic prevention training for primary medical staff was started. Since fever is one of typical clinical symptoms of the infection, temperature detection of incoming and outgoing personnel was implemented comprehensively in railway stations, passenger stations and other public places, in order to screen suspected cases as early as possible. In addition, many other compulsory measures limiting population mobility, such as cancellation of mass gatherings, school closures, work-from-home arrangements, was taken to reduce within-population contact rates. |
sec | 2 Epidemiological and clinical symptoms of 2019-nCoV 2.1 Human coronaviruses Human coronaviruses (CoVs) could cause respiratory, gastrointestinal, hepatic and central nervous system diseases. Infection outcomes vary from mild, self-limiting disease, to more severe manifestations and even death [1,11]. Among the seven human CoVs, two α-coronaviruses (HCoV-229E and HCoV-NL63) and two β-coronaviruses (HCoV-OC43 and HCoV-HKU1) were thought to cause only mild self-limiting upper respiratory disease, such as common cold in immunocompetent hosts, except for a few cases of severe infections in infants, children and seniors [12,13]; the other three CoVs could cause fatal respiratory diseases. In 2002, the Severe acute respiratory syndrome coronavirus (SARS-CoV) emerged in Guangdong province, China, spreading to 37 countries, and its subsequent global epidemic was associated with 8096 cases and 774 deaths. Ten years later, Middle-East respiratory syndrome coronavirus (MERS-CoV) spread to 27 countries, causing 2494 infected cases and 858 deaths worldwide [1]. And the recently identified novel coronavirus (2019-nCoV), was the third highly pathogenic CoV discovered, with a mortality of 2% which is much lower than that of SARS-CoV (10%) and MERS-CoV (37%) [6]. However, the transmissibility of 2019-nCoV is higher, the mean R0 (R0 is used to estimate the transmissibility of virus) of 2019-nCoV ranged from 3.3 to 5.5, and it appeared (slightly) higher than those of SARS-CoV (2–5) and MERS-CoV (2.7–3.9) [[14], [15], [16]]. Measures on ‘entry, exit and travel’ screening and restrictions are likely to reduce the effective R0, which should benefit 2019-nCoV control and prevention. 2.2 Clinical symptoms of 2019-nCoV infection The clinical symptoms of 2019-nCoV infection are similar to those of SARS-CoV and MERS-CoV. Most patients present fever, dry cough, dyspnea, and bilateral ground-glass opacities on chest CT scans [4,17,18]. However, patients with 2019-nCoV infection rarely have obvious upper respiratory signs and symptoms (such as snot, sneezing, or sore throat), indicating that the virus primarily infects the lower respiratory tract [4,17]. In addition, about 20–25% of 2019-nCoV patients experience intestinal symptoms and signs (such as diarrhea), similarly to MERS-CoV or SARS-CoV [17]. In severe 2019-nCoV infection cases, the symptoms include acute respiratory distress syndrome, septic shock, metabolic acidosis, and bleeding and coagulation dysfunction. It is worth noting that severe and critically ill patients may have moderate to low fever during the course of the disease, even without obvious fever [3]. Furthermore, like SARS-CoV and MERS-CoV, 2019-nCoV infections induce production of high levels of cytokines [2,17]. The epidemic of 2019-nCoV bears some similarities to SARS-CoV. The outbreaks of the two viruses occurred at about the same time during the year, and they were quite stable in the environment, especially in air-conditioned space, owing to lower ambient temperature and lower humidity [19]. However, SARS-CoV had an aberrant trait that the “viral load” in upper respiratory tract secretions was low in the first 5 days of illness, then increased progressively and peaked early in the second week. Consequently, the transmission rate was relatively low in the first days of illness, providing an opportunity for case detection and isolation to interrupt transmission. On the contrary, for 2019-nCoV, the incubation lasts for an average of 10 days (in a reported range of 2–14 days) from infection to symptoms surfacing [2,4,17,20]. Even worse, 2019-nCoV is able to spread from one person to another even before any actual clinical manifestations, leading to “extremely challenging” conditions for detecting and isolating potential patients, which makes it more difficult to control the epidemic. 2.3 Transmission routes of the disease 2019-nCoV is thought to be transmitted through droplets, close contact, aerosol and maybe fecal-oral transmission, and patients in the incubation period can transmit the virus to other persons [5]. The distribution of the viral receptor can explain the pathogenic mechanisms, clinical manifestations and transmission routes of 2019-nCoV [20,21]. ACE2, a receptor for 2019-nCoV, is necessary for the viral entry of 2019-nCoV. The ubiquitous expression of ACE2 in various cells, such as lung AT2 cells, esophagus upper, stratified epithelial cells, and absorptive enterocytes of ileum and colon may contribute to the multi-tissue infection of 2019-nCoV [22]. Therefore, besides respiratory and bodily contact, fecal-oral transmission is a potential route for 2019-nCoV infection [23,24]. |
label | 2 |
title | Epidemiological and clinical symptoms of 2019-nCoV |
sec | 2.1 Human coronaviruses Human coronaviruses (CoVs) could cause respiratory, gastrointestinal, hepatic and central nervous system diseases. Infection outcomes vary from mild, self-limiting disease, to more severe manifestations and even death [1,11]. Among the seven human CoVs, two α-coronaviruses (HCoV-229E and HCoV-NL63) and two β-coronaviruses (HCoV-OC43 and HCoV-HKU1) were thought to cause only mild self-limiting upper respiratory disease, such as common cold in immunocompetent hosts, except for a few cases of severe infections in infants, children and seniors [12,13]; the other three CoVs could cause fatal respiratory diseases. In 2002, the Severe acute respiratory syndrome coronavirus (SARS-CoV) emerged in Guangdong province, China, spreading to 37 countries, and its subsequent global epidemic was associated with 8096 cases and 774 deaths. Ten years later, Middle-East respiratory syndrome coronavirus (MERS-CoV) spread to 27 countries, causing 2494 infected cases and 858 deaths worldwide [1]. And the recently identified novel coronavirus (2019-nCoV), was the third highly pathogenic CoV discovered, with a mortality of 2% which is much lower than that of SARS-CoV (10%) and MERS-CoV (37%) [6]. However, the transmissibility of 2019-nCoV is higher, the mean R0 (R0 is used to estimate the transmissibility of virus) of 2019-nCoV ranged from 3.3 to 5.5, and it appeared (slightly) higher than those of SARS-CoV (2–5) and MERS-CoV (2.7–3.9) [[14], [15], [16]]. Measures on ‘entry, exit and travel’ screening and restrictions are likely to reduce the effective R0, which should benefit 2019-nCoV control and prevention. |
label | 2.1 |
title | Human coronaviruses |
p | Human coronaviruses (CoVs) could cause respiratory, gastrointestinal, hepatic and central nervous system diseases. Infection outcomes vary from mild, self-limiting disease, to more severe manifestations and even death [1,11]. Among the seven human CoVs, two α-coronaviruses (HCoV-229E and HCoV-NL63) and two β-coronaviruses (HCoV-OC43 and HCoV-HKU1) were thought to cause only mild self-limiting upper respiratory disease, such as common cold in immunocompetent hosts, except for a few cases of severe infections in infants, children and seniors [12,13]; the other three CoVs could cause fatal respiratory diseases. In 2002, the Severe acute respiratory syndrome coronavirus (SARS-CoV) emerged in Guangdong province, China, spreading to 37 countries, and its subsequent global epidemic was associated with 8096 cases and 774 deaths. Ten years later, Middle-East respiratory syndrome coronavirus (MERS-CoV) spread to 27 countries, causing 2494 infected cases and 858 deaths worldwide [1]. And the recently identified novel coronavirus (2019-nCoV), was the third highly pathogenic CoV discovered, with a mortality of 2% which is much lower than that of SARS-CoV (10%) and MERS-CoV (37%) [6]. However, the transmissibility of 2019-nCoV is higher, the mean R0 (R0 is used to estimate the transmissibility of virus) of 2019-nCoV ranged from 3.3 to 5.5, and it appeared (slightly) higher than those of SARS-CoV (2–5) and MERS-CoV (2.7–3.9) [[14], [15], [16]]. Measures on ‘entry, exit and travel’ screening and restrictions are likely to reduce the effective R0, which should benefit 2019-nCoV control and prevention. |
sec | 2.2 Clinical symptoms of 2019-nCoV infection The clinical symptoms of 2019-nCoV infection are similar to those of SARS-CoV and MERS-CoV. Most patients present fever, dry cough, dyspnea, and bilateral ground-glass opacities on chest CT scans [4,17,18]. However, patients with 2019-nCoV infection rarely have obvious upper respiratory signs and symptoms (such as snot, sneezing, or sore throat), indicating that the virus primarily infects the lower respiratory tract [4,17]. In addition, about 20–25% of 2019-nCoV patients experience intestinal symptoms and signs (such as diarrhea), similarly to MERS-CoV or SARS-CoV [17]. In severe 2019-nCoV infection cases, the symptoms include acute respiratory distress syndrome, septic shock, metabolic acidosis, and bleeding and coagulation dysfunction. It is worth noting that severe and critically ill patients may have moderate to low fever during the course of the disease, even without obvious fever [3]. Furthermore, like SARS-CoV and MERS-CoV, 2019-nCoV infections induce production of high levels of cytokines [2,17]. The epidemic of 2019-nCoV bears some similarities to SARS-CoV. The outbreaks of the two viruses occurred at about the same time during the year, and they were quite stable in the environment, especially in air-conditioned space, owing to lower ambient temperature and lower humidity [19]. However, SARS-CoV had an aberrant trait that the “viral load” in upper respiratory tract secretions was low in the first 5 days of illness, then increased progressively and peaked early in the second week. Consequently, the transmission rate was relatively low in the first days of illness, providing an opportunity for case detection and isolation to interrupt transmission. On the contrary, for 2019-nCoV, the incubation lasts for an average of 10 days (in a reported range of 2–14 days) from infection to symptoms surfacing [2,4,17,20]. Even worse, 2019-nCoV is able to spread from one person to another even before any actual clinical manifestations, leading to “extremely challenging” conditions for detecting and isolating potential patients, which makes it more difficult to control the epidemic. |
label | 2.2 |
title | Clinical symptoms of 2019-nCoV infection |
p | The clinical symptoms of 2019-nCoV infection are similar to those of SARS-CoV and MERS-CoV. Most patients present fever, dry cough, dyspnea, and bilateral ground-glass opacities on chest CT scans [4,17,18]. However, patients with 2019-nCoV infection rarely have obvious upper respiratory signs and symptoms (such as snot, sneezing, or sore throat), indicating that the virus primarily infects the lower respiratory tract [4,17]. In addition, about 20–25% of 2019-nCoV patients experience intestinal symptoms and signs (such as diarrhea), similarly to MERS-CoV or SARS-CoV [17]. In severe 2019-nCoV infection cases, the symptoms include acute respiratory distress syndrome, septic shock, metabolic acidosis, and bleeding and coagulation dysfunction. It is worth noting that severe and critically ill patients may have moderate to low fever during the course of the disease, even without obvious fever [3]. Furthermore, like SARS-CoV and MERS-CoV, 2019-nCoV infections induce production of high levels of cytokines [2,17]. |
p | The epidemic of 2019-nCoV bears some similarities to SARS-CoV. The outbreaks of the two viruses occurred at about the same time during the year, and they were quite stable in the environment, especially in air-conditioned space, owing to lower ambient temperature and lower humidity [19]. However, SARS-CoV had an aberrant trait that the “viral load” in upper respiratory tract secretions was low in the first 5 days of illness, then increased progressively and peaked early in the second week. Consequently, the transmission rate was relatively low in the first days of illness, providing an opportunity for case detection and isolation to interrupt transmission. On the contrary, for 2019-nCoV, the incubation lasts for an average of 10 days (in a reported range of 2–14 days) from infection to symptoms surfacing [2,4,17,20]. Even worse, 2019-nCoV is able to spread from one person to another even before any actual clinical manifestations, leading to “extremely challenging” conditions for detecting and isolating potential patients, which makes it more difficult to control the epidemic. |
sec | 2.3 Transmission routes of the disease 2019-nCoV is thought to be transmitted through droplets, close contact, aerosol and maybe fecal-oral transmission, and patients in the incubation period can transmit the virus to other persons [5]. The distribution of the viral receptor can explain the pathogenic mechanisms, clinical manifestations and transmission routes of 2019-nCoV [20,21]. ACE2, a receptor for 2019-nCoV, is necessary for the viral entry of 2019-nCoV. The ubiquitous expression of ACE2 in various cells, such as lung AT2 cells, esophagus upper, stratified epithelial cells, and absorptive enterocytes of ileum and colon may contribute to the multi-tissue infection of 2019-nCoV [22]. Therefore, besides respiratory and bodily contact, fecal-oral transmission is a potential route for 2019-nCoV infection [23,24]. |
label | 2.3 |
title | Transmission routes of the disease |
p | 2019-nCoV is thought to be transmitted through droplets, close contact, aerosol and maybe fecal-oral transmission, and patients in the incubation period can transmit the virus to other persons [5]. The distribution of the viral receptor can explain the pathogenic mechanisms, clinical manifestations and transmission routes of 2019-nCoV [20,21]. ACE2, a receptor for 2019-nCoV, is necessary for the viral entry of 2019-nCoV. The ubiquitous expression of ACE2 in various cells, such as lung AT2 cells, esophagus upper, stratified epithelial cells, and absorptive enterocytes of ileum and colon may contribute to the multi-tissue infection of 2019-nCoV [22]. Therefore, besides respiratory and bodily contact, fecal-oral transmission is a potential route for 2019-nCoV infection [23,24]. |
sec | 3 Biological features and origin of the 2019-nCoV 3.1 Genomics of 2019-nCoV According to the genome analysis, 2019-nCoV belongs to Sarbecovirus subgenus, Betacoronavirus genus of the subfamily Orthocoronavirinae in the family Coronaviridae of the suborder cornidovirineae of the order Nidovirales [20,26]. Similarly to other coronaviruses, 2019-nCoV is an enveloped, single-stranded positive-sense RNA (+ssRNA) with around 29,900 nt, showing a typical coronavirus appearance under electron microscopy [20]. The genome organization is 5′-leader-UTR-replicase-S (Spike)-E (Envelope)-M (Membrane)-N (Nucleocapsid)-3′UTRpoly (A) tail with accessory genes interspersed within the structural genes and the 3′ end of the genome [26]. 2019-nCoV shares 79.5% nt identity with SARS-CoV and 96% identity with bat-CoV-RaTG13 [20]. The genetic characteristics of 2019-nCoV are significantly different from SARSr-CoV and SARS-CoV, which are closely related only to the specific bat SARS-like coronaviruses, bat-CoV-RaTG13 and bat-SL-CoVZC45, with 96% and 86.9% nucleotide sequence identities, respectively [20]. Therefore 2019-nCoV is considered as a SARS related coronavirus (SARSr-CoV) by pairwise protein sequence analysis (Fig. 1 ) [20]. Fig. 1 The evolutionary position of 2019-nCoV. The phylogenetic tree was constructed based on the complete genomic sequences of 2019-nCoV and related CoVs by using the neighbor-joining (NJ) method with 1000 bootstrap. The novel 2019-nCoV detected strains were indicated in bold with solid squares. Abbreviations are as follows: SARSr coronavirus, SARS-related coronavirus. The accession numbers of CoVs were showed in the figure. Bootstrap values above 50 were shown. 3.2 Receptor usage of 2019-nCoV The prerequisite of coronaviruses invading the host-cell is to bind to receptors. Following receptor binding, the viral spike protein is cleaved via acid-dependent proteolysis by cathepsin, TMPRRS2 or furin protease, followed by fusion of the viral envelop to the cellular membranes. Spike is a large, clove-shaped trimer, which can be cleaved by proteases into an N-terminal S1 subunit containing the receptor binding domain (RBD) and a C-terminal S2 region. Compared to other proteins of coronavirus, the spike protein has the most variable amino acid sequences which results from the strongest positive selection among all genes of coronavirus to adapt to its hosts [27].The RBD is a major determinant of host range and cross-species transmission. Recently results showed that 2019-nCoV uses the same cell entry receptor, ACE2, as SARS-CoV [21,24,20,28]. Besides human ACE2, 2019-nCoV can use Chinese horseshoe bats, civet, or pig ACE2 but not mouse ACE2 as an entry receptor [24]. One explanation is that human ACE2 has high homology with Chinese horseshoe bats, civet, pig and mouse, which are 80.75%, 83.48%, 81.37%, 82.11% respectively (Table 2 ), while the residues 82 and 353 of mouse ACE2 are different from human ACE2 which are important residues for bind to spike [29]. But the homology of human ACE2 and pangolin ACE2, cat ACE2 are also high with 84.84% and 85.22%. The homology of human ACE2 with other ACE2 varies from 59.73% to 83.23%. In summary, 2019-nCoV may have extensive host ranges. Fig. 2 . Table 2 The amino acid sequence identities of ACE2 among different species. Species Pairwise identities (%) of the amino acid sequences of ACE2 African green monkey Civet Bat (R. sinicus) Swine Malayan pangolin Mouse Cat Camel Goat Bos mutus Erinaceus europaeus Cavia porcellus Phascolarctos cinereus Sarcophilus harrisii Tiger Snake Parus major Gallus gallus Human 93.93 83.48 80.75 81.37 84.84 82.11 85.22 83.23 81.61 81.24 79.01 77.29 71.17 70.51 59.73 67.08 65.31 African green monkey 81.78 79.93 79.93 83.77 81.78 83.15 81.91 81.41 80.92 77.94 76.85 70.38 69.84 59.21 65.6 64.13 Civet 81.78 81.49 82.73 85.59 81.61 93.29 83.85 81.86 81.74 79.75 75.55 71.66 71.63 59.6 67.44 66.3 Bat (R. sinicus) 79.93 81.49 80 82.86 78.26 83.73 81.37 80.37 80 78.51 74.56 69.68 70.02 58.61 65.11 65.19 Swine 79.93 82.73 80 82.61 80.62 83.98 89.81 87.7 87.58 77.64 75.3 71.17 71.01 61.09 66.95 65.56 Malayan pangolin 83.77 85.59 82.86 82.61 82.73 87.33 84.72 82.11 81.86 80.87 77.17 71.66 70.89 59.6 67.44 65.07 Mouse 81.78 81.61 78.26 80.62 82.73 81.74 80.99 80.12 80.37 78.63 78.16 71.05 71.13 61.21 67.2 66.17 Cat 83.15 93.29 83.73 83.98 87.33 81.74 85.71 83.23 83.11 81.49 76.42 72.65 72.25 59.6 67.81 66.79 Camel 81.91 83.85 81.37 89.81 84.72 80.99 85.71 88.2 88.57 79.25 77.29 71.79 72.12 61.34 67.2 66.42 Goat 81.41 81.86 80.37 87.7 82.11 80.12 83.23 88.2 97.76 78.14 76.54 72.04 71.38 60.84 66.09 65.44 Bos mutus 80.92 81.74 80 87.58 81.86 80.37 83.11 88.57 97.76 78.39 77.04 71.42 71.01 61.09 66.09 65.31 Erinaceus europaeus 77.94 79.75 78.51 77.64 80.87 78.63 81.49 79.25 78.14 78.39 74.56 70.67 69.65 60.59 68.8 66.42 Cavia porcellus 76.85 75.55 74.56 75.3 77.17 78.16 76.42 77.29 76.54 77.04 74.56 66.61 66.82 59.38 63.9 63.12 Phascolarctos cinereus 70.38 71.66 69.68 71.17 71.66 71.05 72.65 71.79 72.04 71.42 70.67 66.61 81.92 60.7 67.08 64.83 Sarcophilus harrisii 69.84 71.63 70.02 71.01 70.89 71.13 72.25 72.12 71.38 71.01 69.65 66.82 81.92 60.9 64.62 63.84 Tiger Snake 59.21 59.6 58.61 61.09 59.6 61.21 59.6 61.34 60.84 61.09 60.59 59.38 60.7 60.9 64.5 63.71 Parus major 65.6 67.44 65.11 66.95 67.44 67.2 67.81 67.2 66.09 66.09 68.8 63.9 67.08 64.62 64.5 82.2 Gallus gallus 64.13 66.3 65.19 65.56 65.07 66.17 66.79 66.42 65.44 65.31 66.42 63.12 64.83 63.84 63.71 82.2 Accession numbers: human (NP_068576.1), monkey (AY996037.1), civet (AAX63775.1), bat (R.sinicus) (AGZ48803.1), swine (NP_001116542.1), Malayan pangolin (XP_017505752.1), mouse (NP_001123985.1), cat (NP_001034545.1), camel (XP_010991717.1), goat (NP_001277036.1), Bos mutus (XP_005903173.1), Erinaceus europaeus (XP_007538670.1), Phascolarctos cinereus (XP_020863153.1), Sarcophilus harrisii (XP_031814825.1), tiger Snake (XP_026530754.1), Parus major (XP_015486815.1), Gallus gallus (QEQ50331.1). Fig. 2 Phylogenetic analysis of ACE2 from humans and 18 other animals. The tree was constructed based on the complete amino acid sequences of ACE2s derived from humans and other animals by using the neighbor-joining (NJ) method with 1000 bootstrap. The accession numbers were as the same as Table 2. Bootstrap values above 50 were shown. 3.3 Origin of 2019-nCoV The epidemiological investigation of early cases of 2019-nCoV pneumonia showed that many cases had been exposed to the Huanan Seafood Market in Wuhan [2,24,20]. In addition, 33 of the 585 environmental samples from Huanan Seafood Market were positive for 2019-nCoV; however, that market was trading a variety of live animals, such as hedgehog, badger, snake, and bird (turtledoves), probably pangolin, but not bats [24,30]. Thus, bats are less likely to have direct contact with human, and direct transmission of the virus from bat to human is unlikely [1]. Indeed, SARS-CoV and MERS-CoV originated from bats, the natural reservoir, but transmitted to humans via intermediate host civets and camels, respectively. Therefore, 2019-nCoV may also originate from bat but was then transmitted to humans via an intermediate host in the market [[31], [32], [33], [34], [35]]. Currently, 2019-nCoV has been isolated from pangolins and it was found that the genomic seuqence of the isolated strain had 99% identity with that of currently 2019-nCoV infected humans. It has been proposed that the transmission and evolution path of 2019-nCoV was from bat-CoV to pangolins (the intermediate hosts), from where it infected humans [36]. |
label | 3 |
title | Biological features and origin of the 2019-nCoV |
sec | 3.1 Genomics of 2019-nCoV According to the genome analysis, 2019-nCoV belongs to Sarbecovirus subgenus, Betacoronavirus genus of the subfamily Orthocoronavirinae in the family Coronaviridae of the suborder cornidovirineae of the order Nidovirales [20,26]. Similarly to other coronaviruses, 2019-nCoV is an enveloped, single-stranded positive-sense RNA (+ssRNA) with around 29,900 nt, showing a typical coronavirus appearance under electron microscopy [20]. The genome organization is 5′-leader-UTR-replicase-S (Spike)-E (Envelope)-M (Membrane)-N (Nucleocapsid)-3′UTRpoly (A) tail with accessory genes interspersed within the structural genes and the 3′ end of the genome [26]. 2019-nCoV shares 79.5% nt identity with SARS-CoV and 96% identity with bat-CoV-RaTG13 [20]. The genetic characteristics of 2019-nCoV are significantly different from SARSr-CoV and SARS-CoV, which are closely related only to the specific bat SARS-like coronaviruses, bat-CoV-RaTG13 and bat-SL-CoVZC45, with 96% and 86.9% nucleotide sequence identities, respectively [20]. Therefore 2019-nCoV is considered as a SARS related coronavirus (SARSr-CoV) by pairwise protein sequence analysis (Fig. 1 ) [20]. Fig. 1 The evolutionary position of 2019-nCoV. The phylogenetic tree was constructed based on the complete genomic sequences of 2019-nCoV and related CoVs by using the neighbor-joining (NJ) method with 1000 bootstrap. The novel 2019-nCoV detected strains were indicated in bold with solid squares. Abbreviations are as follows: SARSr coronavirus, SARS-related coronavirus. The accession numbers of CoVs were showed in the figure. Bootstrap values above 50 were shown. |
label | 3.1 |
title | Genomics of 2019-nCoV |
p | According to the genome analysis, 2019-nCoV belongs to Sarbecovirus subgenus, Betacoronavirus genus of the subfamily Orthocoronavirinae in the family Coronaviridae of the suborder cornidovirineae of the order Nidovirales [20,26]. Similarly to other coronaviruses, 2019-nCoV is an enveloped, single-stranded positive-sense RNA (+ssRNA) with around 29,900 nt, showing a typical coronavirus appearance under electron microscopy [20]. The genome organization is 5′-leader-UTR-replicase-S (Spike)-E (Envelope)-M (Membrane)-N (Nucleocapsid)-3′UTRpoly (A) tail with accessory genes interspersed within the structural genes and the 3′ end of the genome [26]. |
p | 2019-nCoV shares 79.5% nt identity with SARS-CoV and 96% identity with bat-CoV-RaTG13 [20]. The genetic characteristics of 2019-nCoV are significantly different from SARSr-CoV and SARS-CoV, which are closely related only to the specific bat SARS-like coronaviruses, bat-CoV-RaTG13 and bat-SL-CoVZC45, with 96% and 86.9% nucleotide sequence identities, respectively [20]. Therefore 2019-nCoV is considered as a SARS related coronavirus (SARSr-CoV) by pairwise protein sequence analysis (Fig. 1 ) [20]. Fig. 1 The evolutionary position of 2019-nCoV. The phylogenetic tree was constructed based on the complete genomic sequences of 2019-nCoV and related CoVs by using the neighbor-joining (NJ) method with 1000 bootstrap. The novel 2019-nCoV detected strains were indicated in bold with solid squares. Abbreviations are as follows: SARSr coronavirus, SARS-related coronavirus. The accession numbers of CoVs were showed in the figure. Bootstrap values above 50 were shown. |
figure | Fig. 1 The evolutionary position of 2019-nCoV. The phylogenetic tree was constructed based on the complete genomic sequences of 2019-nCoV and related CoVs by using the neighbor-joining (NJ) method with 1000 bootstrap. The novel 2019-nCoV detected strains were indicated in bold with solid squares. Abbreviations are as follows: SARSr coronavirus, SARS-related coronavirus. The accession numbers of CoVs were showed in the figure. Bootstrap values above 50 were shown. |
label | Fig. 1 |
caption | The evolutionary position of 2019-nCoV. The phylogenetic tree was constructed based on the complete genomic sequences of 2019-nCoV and related CoVs by using the neighbor-joining (NJ) method with 1000 bootstrap. The novel 2019-nCoV detected strains were indicated in bold with solid squares. Abbreviations are as follows: SARSr coronavirus, SARS-related coronavirus. The accession numbers of CoVs were showed in the figure. Bootstrap values above 50 were shown. |
p | The evolutionary position of 2019-nCoV. The phylogenetic tree was constructed based on the complete genomic sequences of 2019-nCoV and related CoVs by using the neighbor-joining (NJ) method with 1000 bootstrap. The novel 2019-nCoV detected strains were indicated in bold with solid squares. Abbreviations are as follows: SARSr coronavirus, SARS-related coronavirus. The accession numbers of CoVs were showed in the figure. Bootstrap values above 50 were shown. |
sec | 3.2 Receptor usage of 2019-nCoV The prerequisite of coronaviruses invading the host-cell is to bind to receptors. Following receptor binding, the viral spike protein is cleaved via acid-dependent proteolysis by cathepsin, TMPRRS2 or furin protease, followed by fusion of the viral envelop to the cellular membranes. Spike is a large, clove-shaped trimer, which can be cleaved by proteases into an N-terminal S1 subunit containing the receptor binding domain (RBD) and a C-terminal S2 region. Compared to other proteins of coronavirus, the spike protein has the most variable amino acid sequences which results from the strongest positive selection among all genes of coronavirus to adapt to its hosts [27].The RBD is a major determinant of host range and cross-species transmission. Recently results showed that 2019-nCoV uses the same cell entry receptor, ACE2, as SARS-CoV [21,24,20,28]. Besides human ACE2, 2019-nCoV can use Chinese horseshoe bats, civet, or pig ACE2 but not mouse ACE2 as an entry receptor [24]. One explanation is that human ACE2 has high homology with Chinese horseshoe bats, civet, pig and mouse, which are 80.75%, 83.48%, 81.37%, 82.11% respectively (Table 2 ), while the residues 82 and 353 of mouse ACE2 are different from human ACE2 which are important residues for bind to spike [29]. But the homology of human ACE2 and pangolin ACE2, cat ACE2 are also high with 84.84% and 85.22%. The homology of human ACE2 with other ACE2 varies from 59.73% to 83.23%. In summary, 2019-nCoV may have extensive host ranges. Fig. 2 . Table 2 The amino acid sequence identities of ACE2 among different species. Species Pairwise identities (%) of the amino acid sequences of ACE2 African green monkey Civet Bat (R. sinicus) Swine Malayan pangolin Mouse Cat Camel Goat Bos mutus Erinaceus europaeus Cavia porcellus Phascolarctos cinereus Sarcophilus harrisii Tiger Snake Parus major Gallus gallus Human 93.93 83.48 80.75 81.37 84.84 82.11 85.22 83.23 81.61 81.24 79.01 77.29 71.17 70.51 59.73 67.08 65.31 African green monkey 81.78 79.93 79.93 83.77 81.78 83.15 81.91 81.41 80.92 77.94 76.85 70.38 69.84 59.21 65.6 64.13 Civet 81.78 81.49 82.73 85.59 81.61 93.29 83.85 81.86 81.74 79.75 75.55 71.66 71.63 59.6 67.44 66.3 Bat (R. sinicus) 79.93 81.49 80 82.86 78.26 83.73 81.37 80.37 80 78.51 74.56 69.68 70.02 58.61 65.11 65.19 Swine 79.93 82.73 80 82.61 80.62 83.98 89.81 87.7 87.58 77.64 75.3 71.17 71.01 61.09 66.95 65.56 Malayan pangolin 83.77 85.59 82.86 82.61 82.73 87.33 84.72 82.11 81.86 80.87 77.17 71.66 70.89 59.6 67.44 65.07 Mouse 81.78 81.61 78.26 80.62 82.73 81.74 80.99 80.12 80.37 78.63 78.16 71.05 71.13 61.21 67.2 66.17 Cat 83.15 93.29 83.73 83.98 87.33 81.74 85.71 83.23 83.11 81.49 76.42 72.65 72.25 59.6 67.81 66.79 Camel 81.91 83.85 81.37 89.81 84.72 80.99 85.71 88.2 88.57 79.25 77.29 71.79 72.12 61.34 67.2 66.42 Goat 81.41 81.86 80.37 87.7 82.11 80.12 83.23 88.2 97.76 78.14 76.54 72.04 71.38 60.84 66.09 65.44 Bos mutus 80.92 81.74 80 87.58 81.86 80.37 83.11 88.57 97.76 78.39 77.04 71.42 71.01 61.09 66.09 65.31 Erinaceus europaeus 77.94 79.75 78.51 77.64 80.87 78.63 81.49 79.25 78.14 78.39 74.56 70.67 69.65 60.59 68.8 66.42 Cavia porcellus 76.85 75.55 74.56 75.3 77.17 78.16 76.42 77.29 76.54 77.04 74.56 66.61 66.82 59.38 63.9 63.12 Phascolarctos cinereus 70.38 71.66 69.68 71.17 71.66 71.05 72.65 71.79 72.04 71.42 70.67 66.61 81.92 60.7 67.08 64.83 Sarcophilus harrisii 69.84 71.63 70.02 71.01 70.89 71.13 72.25 72.12 71.38 71.01 69.65 66.82 81.92 60.9 64.62 63.84 Tiger Snake 59.21 59.6 58.61 61.09 59.6 61.21 59.6 61.34 60.84 61.09 60.59 59.38 60.7 60.9 64.5 63.71 Parus major 65.6 67.44 65.11 66.95 67.44 67.2 67.81 67.2 66.09 66.09 68.8 63.9 67.08 64.62 64.5 82.2 Gallus gallus 64.13 66.3 65.19 65.56 65.07 66.17 66.79 66.42 65.44 65.31 66.42 63.12 64.83 63.84 63.71 82.2 Accession numbers: human (NP_068576.1), monkey (AY996037.1), civet (AAX63775.1), bat (R.sinicus) (AGZ48803.1), swine (NP_001116542.1), Malayan pangolin (XP_017505752.1), mouse (NP_001123985.1), cat (NP_001034545.1), camel (XP_010991717.1), goat (NP_001277036.1), Bos mutus (XP_005903173.1), Erinaceus europaeus (XP_007538670.1), Phascolarctos cinereus (XP_020863153.1), Sarcophilus harrisii (XP_031814825.1), tiger Snake (XP_026530754.1), Parus major (XP_015486815.1), Gallus gallus (QEQ50331.1). Fig. 2 Phylogenetic analysis of ACE2 from humans and 18 other animals. The tree was constructed based on the complete amino acid sequences of ACE2s derived from humans and other animals by using the neighbor-joining (NJ) method with 1000 bootstrap. The accession numbers were as the same as Table 2. Bootstrap values above 50 were shown. |
label | 3.2 |
title | Receptor usage of 2019-nCoV |
p | The prerequisite of coronaviruses invading the host-cell is to bind to receptors. Following receptor binding, the viral spike protein is cleaved via acid-dependent proteolysis by cathepsin, TMPRRS2 or furin protease, followed by fusion of the viral envelop to the cellular membranes. Spike is a large, clove-shaped trimer, which can be cleaved by proteases into an N-terminal S1 subunit containing the receptor binding domain (RBD) and a C-terminal S2 region. Compared to other proteins of coronavirus, the spike protein has the most variable amino acid sequences which results from the strongest positive selection among all genes of coronavirus to adapt to its hosts [27].The RBD is a major determinant of host range and cross-species transmission. Recently results showed that 2019-nCoV uses the same cell entry receptor, ACE2, as SARS-CoV [21,24,20,28]. Besides human ACE2, 2019-nCoV can use Chinese horseshoe bats, civet, or pig ACE2 but not mouse ACE2 as an entry receptor [24]. One explanation is that human ACE2 has high homology with Chinese horseshoe bats, civet, pig and mouse, which are 80.75%, 83.48%, 81.37%, 82.11% respectively (Table 2 ), while the residues 82 and 353 of mouse ACE2 are different from human ACE2 which are important residues for bind to spike [29]. But the homology of human ACE2 and pangolin ACE2, cat ACE2 are also high with 84.84% and 85.22%. The homology of human ACE2 with other ACE2 varies from 59.73% to 83.23%. In summary, 2019-nCoV may have extensive host ranges. Fig. 2 . Table 2 The amino acid sequence identities of ACE2 among different species. Species Pairwise identities (%) of the amino acid sequences of ACE2 African green monkey Civet Bat (R. sinicus) Swine Malayan pangolin Mouse Cat Camel Goat Bos mutus Erinaceus europaeus Cavia porcellus Phascolarctos cinereus Sarcophilus harrisii Tiger Snake Parus major Gallus gallus Human 93.93 83.48 80.75 81.37 84.84 82.11 85.22 83.23 81.61 81.24 79.01 77.29 71.17 70.51 59.73 67.08 65.31 African green monkey 81.78 79.93 79.93 83.77 81.78 83.15 81.91 81.41 80.92 77.94 76.85 70.38 69.84 59.21 65.6 64.13 Civet 81.78 81.49 82.73 85.59 81.61 93.29 83.85 81.86 81.74 79.75 75.55 71.66 71.63 59.6 67.44 66.3 Bat (R. sinicus) 79.93 81.49 80 82.86 78.26 83.73 81.37 80.37 80 78.51 74.56 69.68 70.02 58.61 65.11 65.19 Swine 79.93 82.73 80 82.61 80.62 83.98 89.81 87.7 87.58 77.64 75.3 71.17 71.01 61.09 66.95 65.56 Malayan pangolin 83.77 85.59 82.86 82.61 82.73 87.33 84.72 82.11 81.86 80.87 77.17 71.66 70.89 59.6 67.44 65.07 Mouse 81.78 81.61 78.26 80.62 82.73 81.74 80.99 80.12 80.37 78.63 78.16 71.05 71.13 61.21 67.2 66.17 Cat 83.15 93.29 83.73 83.98 87.33 81.74 85.71 83.23 83.11 81.49 76.42 72.65 72.25 59.6 67.81 66.79 Camel 81.91 83.85 81.37 89.81 84.72 80.99 85.71 88.2 88.57 79.25 77.29 71.79 72.12 61.34 67.2 66.42 Goat 81.41 81.86 80.37 87.7 82.11 80.12 83.23 88.2 97.76 78.14 76.54 72.04 71.38 60.84 66.09 65.44 Bos mutus 80.92 81.74 80 87.58 81.86 80.37 83.11 88.57 97.76 78.39 77.04 71.42 71.01 61.09 66.09 65.31 Erinaceus europaeus 77.94 79.75 78.51 77.64 80.87 78.63 81.49 79.25 78.14 78.39 74.56 70.67 69.65 60.59 68.8 66.42 Cavia porcellus 76.85 75.55 74.56 75.3 77.17 78.16 76.42 77.29 76.54 77.04 74.56 66.61 66.82 59.38 63.9 63.12 Phascolarctos cinereus 70.38 71.66 69.68 71.17 71.66 71.05 72.65 71.79 72.04 71.42 70.67 66.61 81.92 60.7 67.08 64.83 Sarcophilus harrisii 69.84 71.63 70.02 71.01 70.89 71.13 72.25 72.12 71.38 71.01 69.65 66.82 81.92 60.9 64.62 63.84 Tiger Snake 59.21 59.6 58.61 61.09 59.6 61.21 59.6 61.34 60.84 61.09 60.59 59.38 60.7 60.9 64.5 63.71 Parus major 65.6 67.44 65.11 66.95 67.44 67.2 67.81 67.2 66.09 66.09 68.8 63.9 67.08 64.62 64.5 82.2 Gallus gallus 64.13 66.3 65.19 65.56 65.07 66.17 66.79 66.42 65.44 65.31 66.42 63.12 64.83 63.84 63.71 82.2 Accession numbers: human (NP_068576.1), monkey (AY996037.1), civet (AAX63775.1), bat (R.sinicus) (AGZ48803.1), swine (NP_001116542.1), Malayan pangolin (XP_017505752.1), mouse (NP_001123985.1), cat (NP_001034545.1), camel (XP_010991717.1), goat (NP_001277036.1), Bos mutus (XP_005903173.1), Erinaceus europaeus (XP_007538670.1), Phascolarctos cinereus (XP_020863153.1), Sarcophilus harrisii (XP_031814825.1), tiger Snake (XP_026530754.1), Parus major (XP_015486815.1), Gallus gallus (QEQ50331.1). Fig. 2 Phylogenetic analysis of ACE2 from humans and 18 other animals. The tree was constructed based on the complete amino acid sequences of ACE2s derived from humans and other animals by using the neighbor-joining (NJ) method with 1000 bootstrap. The accession numbers were as the same as Table 2. Bootstrap values above 50 were shown. |
table-wrap | Table 2 The amino acid sequence identities of ACE2 among different species. Species Pairwise identities (%) of the amino acid sequences of ACE2 African green monkey Civet Bat (R. sinicus) Swine Malayan pangolin Mouse Cat Camel Goat Bos mutus Erinaceus europaeus Cavia porcellus Phascolarctos cinereus Sarcophilus harrisii Tiger Snake Parus major Gallus gallus Human 93.93 83.48 80.75 81.37 84.84 82.11 85.22 83.23 81.61 81.24 79.01 77.29 71.17 70.51 59.73 67.08 65.31 African green monkey 81.78 79.93 79.93 83.77 81.78 83.15 81.91 81.41 80.92 77.94 76.85 70.38 69.84 59.21 65.6 64.13 Civet 81.78 81.49 82.73 85.59 81.61 93.29 83.85 81.86 81.74 79.75 75.55 71.66 71.63 59.6 67.44 66.3 Bat (R. sinicus) 79.93 81.49 80 82.86 78.26 83.73 81.37 80.37 80 78.51 74.56 69.68 70.02 58.61 65.11 65.19 Swine 79.93 82.73 80 82.61 80.62 83.98 89.81 87.7 87.58 77.64 75.3 71.17 71.01 61.09 66.95 65.56 Malayan pangolin 83.77 85.59 82.86 82.61 82.73 87.33 84.72 82.11 81.86 80.87 77.17 71.66 70.89 59.6 67.44 65.07 Mouse 81.78 81.61 78.26 80.62 82.73 81.74 80.99 80.12 80.37 78.63 78.16 71.05 71.13 61.21 67.2 66.17 Cat 83.15 93.29 83.73 83.98 87.33 81.74 85.71 83.23 83.11 81.49 76.42 72.65 72.25 59.6 67.81 66.79 Camel 81.91 83.85 81.37 89.81 84.72 80.99 85.71 88.2 88.57 79.25 77.29 71.79 72.12 61.34 67.2 66.42 Goat 81.41 81.86 80.37 87.7 82.11 80.12 83.23 88.2 97.76 78.14 76.54 72.04 71.38 60.84 66.09 65.44 Bos mutus 80.92 81.74 80 87.58 81.86 80.37 83.11 88.57 97.76 78.39 77.04 71.42 71.01 61.09 66.09 65.31 Erinaceus europaeus 77.94 79.75 78.51 77.64 80.87 78.63 81.49 79.25 78.14 78.39 74.56 70.67 69.65 60.59 68.8 66.42 Cavia porcellus 76.85 75.55 74.56 75.3 77.17 78.16 76.42 77.29 76.54 77.04 74.56 66.61 66.82 59.38 63.9 63.12 Phascolarctos cinereus 70.38 71.66 69.68 71.17 71.66 71.05 72.65 71.79 72.04 71.42 70.67 66.61 81.92 60.7 67.08 64.83 Sarcophilus harrisii 69.84 71.63 70.02 71.01 70.89 71.13 72.25 72.12 71.38 71.01 69.65 66.82 81.92 60.9 64.62 63.84 Tiger Snake 59.21 59.6 58.61 61.09 59.6 61.21 59.6 61.34 60.84 61.09 60.59 59.38 60.7 60.9 64.5 63.71 Parus major 65.6 67.44 65.11 66.95 67.44 67.2 67.81 67.2 66.09 66.09 68.8 63.9 67.08 64.62 64.5 82.2 Gallus gallus 64.13 66.3 65.19 65.56 65.07 66.17 66.79 66.42 65.44 65.31 66.42 63.12 64.83 63.84 63.71 82.2 Accession numbers: human (NP_068576.1), monkey (AY996037.1), civet (AAX63775.1), bat (R.sinicus) (AGZ48803.1), swine (NP_001116542.1), Malayan pangolin (XP_017505752.1), mouse (NP_001123985.1), cat (NP_001034545.1), camel (XP_010991717.1), goat (NP_001277036.1), Bos mutus (XP_005903173.1), Erinaceus europaeus (XP_007538670.1), Phascolarctos cinereus (XP_020863153.1), Sarcophilus harrisii (XP_031814825.1), tiger Snake (XP_026530754.1), Parus major (XP_015486815.1), Gallus gallus (QEQ50331.1). |
label | Table 2 |
caption | The amino acid sequence identities of ACE2 among different species. |
p | The amino acid sequence identities of ACE2 among different species. |
table | Species Pairwise identities (%) of the amino acid sequences of ACE2 African green monkey Civet Bat (R. sinicus) Swine Malayan pangolin Mouse Cat Camel Goat Bos mutus Erinaceus europaeus Cavia porcellus Phascolarctos cinereus Sarcophilus harrisii Tiger Snake Parus major Gallus gallus Human 93.93 83.48 80.75 81.37 84.84 82.11 85.22 83.23 81.61 81.24 79.01 77.29 71.17 70.51 59.73 67.08 65.31 African green monkey 81.78 79.93 79.93 83.77 81.78 83.15 81.91 81.41 80.92 77.94 76.85 70.38 69.84 59.21 65.6 64.13 Civet 81.78 81.49 82.73 85.59 81.61 93.29 83.85 81.86 81.74 79.75 75.55 71.66 71.63 59.6 67.44 66.3 Bat (R. sinicus) 79.93 81.49 80 82.86 78.26 83.73 81.37 80.37 80 78.51 74.56 69.68 70.02 58.61 65.11 65.19 Swine 79.93 82.73 80 82.61 80.62 83.98 89.81 87.7 87.58 77.64 75.3 71.17 71.01 61.09 66.95 65.56 Malayan pangolin 83.77 85.59 82.86 82.61 82.73 87.33 84.72 82.11 81.86 80.87 77.17 71.66 70.89 59.6 67.44 65.07 Mouse 81.78 81.61 78.26 80.62 82.73 81.74 80.99 80.12 80.37 78.63 78.16 71.05 71.13 61.21 67.2 66.17 Cat 83.15 93.29 83.73 83.98 87.33 81.74 85.71 83.23 83.11 81.49 76.42 72.65 72.25 59.6 67.81 66.79 Camel 81.91 83.85 81.37 89.81 84.72 80.99 85.71 88.2 88.57 79.25 77.29 71.79 72.12 61.34 67.2 66.42 Goat 81.41 81.86 80.37 87.7 82.11 80.12 83.23 88.2 97.76 78.14 76.54 72.04 71.38 60.84 66.09 65.44 Bos mutus 80.92 81.74 80 87.58 81.86 80.37 83.11 88.57 97.76 78.39 77.04 71.42 71.01 61.09 66.09 65.31 Erinaceus europaeus 77.94 79.75 78.51 77.64 80.87 78.63 81.49 79.25 78.14 78.39 74.56 70.67 69.65 60.59 68.8 66.42 Cavia porcellus 76.85 75.55 74.56 75.3 77.17 78.16 76.42 77.29 76.54 77.04 74.56 66.61 66.82 59.38 63.9 63.12 Phascolarctos cinereus 70.38 71.66 69.68 71.17 71.66 71.05 72.65 71.79 72.04 71.42 70.67 66.61 81.92 60.7 67.08 64.83 Sarcophilus harrisii 69.84 71.63 70.02 71.01 70.89 71.13 72.25 72.12 71.38 71.01 69.65 66.82 81.92 60.9 64.62 63.84 Tiger Snake 59.21 59.6 58.61 61.09 59.6 61.21 59.6 61.34 60.84 61.09 60.59 59.38 60.7 60.9 64.5 63.71 Parus major 65.6 67.44 65.11 66.95 67.44 67.2 67.81 67.2 66.09 66.09 68.8 63.9 67.08 64.62 64.5 82.2 Gallus gallus 64.13 66.3 65.19 65.56 65.07 66.17 66.79 66.42 65.44 65.31 66.42 63.12 64.83 63.84 63.71 82.2 |
tr | Species Pairwise identities (%) of the amino acid sequences of ACE2 |
th | Species |
th | Pairwise identities (%) of the amino acid sequences of ACE2 |
tr | African green monkey Civet Bat (R. sinicus) Swine Malayan pangolin Mouse Cat Camel Goat Bos mutus Erinaceus europaeus Cavia porcellus Phascolarctos cinereus Sarcophilus harrisii Tiger Snake Parus major Gallus gallus |
th | African green monkey |
th | Civet |
th | Bat (R. sinicus) |
th | Swine |
th | Malayan pangolin |
th | Mouse |
th | Cat |
th | Camel |
th | Goat |
th | Bos mutus |
th | Erinaceus europaeus |
th | Cavia porcellus |
th | Phascolarctos cinereus |
th | Sarcophilus harrisii |
th | Tiger Snake |
th | Parus major |
th | Gallus gallus |
tr | Human 93.93 83.48 80.75 81.37 84.84 82.11 85.22 83.23 81.61 81.24 79.01 77.29 71.17 70.51 59.73 67.08 65.31 |
td | Human |
td | 93.93 |
td | 83.48 |
td | 80.75 |
td | 81.37 |
td | 84.84 |
td | 82.11 |
td | 85.22 |
td | 83.23 |
td | 81.61 |
td | 81.24 |
td | 79.01 |
td | 77.29 |
td | 71.17 |
td | 70.51 |
td | 59.73 |
td | 67.08 |
td | 65.31 |
tr | African green monkey 81.78 79.93 79.93 83.77 81.78 83.15 81.91 81.41 80.92 77.94 76.85 70.38 69.84 59.21 65.6 64.13 |
td | African green monkey |
td | 81.78 |
td | 79.93 |
td | 79.93 |
td | 83.77 |
td | 81.78 |
td | 83.15 |
td | 81.91 |
td | 81.41 |
td | 80.92 |
td | 77.94 |
td | 76.85 |
td | 70.38 |
td | 69.84 |
td | 59.21 |
td | 65.6 |
td | 64.13 |
tr | Civet 81.78 81.49 82.73 85.59 81.61 93.29 83.85 81.86 81.74 79.75 75.55 71.66 71.63 59.6 67.44 66.3 |
td | Civet |
td | 81.78 |
td | 81.49 |
td | 82.73 |
td | 85.59 |
td | 81.61 |
td | 93.29 |
td | 83.85 |
td | 81.86 |
td | 81.74 |
td | 79.75 |
td | 75.55 |
td | 71.66 |
td | 71.63 |
td | 59.6 |
td | 67.44 |
td | 66.3 |
tr | Bat (R. sinicus) 79.93 81.49 80 82.86 78.26 83.73 81.37 80.37 80 78.51 74.56 69.68 70.02 58.61 65.11 65.19 |
td | Bat (R. sinicus) |
td | 79.93 |
td | 81.49 |
td | 80 |
td | 82.86 |
td | 78.26 |
td | 83.73 |
td | 81.37 |
td | 80.37 |
td | 80 |
td | 78.51 |
td | 74.56 |
td | 69.68 |
td | 70.02 |
td | 58.61 |
td | 65.11 |
td | 65.19 |
tr | Swine 79.93 82.73 80 82.61 80.62 83.98 89.81 87.7 87.58 77.64 75.3 71.17 71.01 61.09 66.95 65.56 |
td | Swine |
td | 79.93 |
td | 82.73 |
td | 80 |
td | 82.61 |
td | 80.62 |
td | 83.98 |
td | 89.81 |
td | 87.7 |
td | 87.58 |
td | 77.64 |
td | 75.3 |
td | 71.17 |
td | 71.01 |
td | 61.09 |
td | 66.95 |
td | 65.56 |
tr | Malayan pangolin 83.77 85.59 82.86 82.61 82.73 87.33 84.72 82.11 81.86 80.87 77.17 71.66 70.89 59.6 67.44 65.07 |
td | Malayan pangolin |
td | 83.77 |
td | 85.59 |
td | 82.86 |
td | 82.61 |
td | 82.73 |
td | 87.33 |
td | 84.72 |
td | 82.11 |
td | 81.86 |
td | 80.87 |
td | 77.17 |
td | 71.66 |
td | 70.89 |
td | 59.6 |
td | 67.44 |
td | 65.07 |
tr | Mouse 81.78 81.61 78.26 80.62 82.73 81.74 80.99 80.12 80.37 78.63 78.16 71.05 71.13 61.21 67.2 66.17 |
td | Mouse |
td | 81.78 |
td | 81.61 |
td | 78.26 |
td | 80.62 |
td | 82.73 |
td | 81.74 |
td | 80.99 |
td | 80.12 |
td | 80.37 |
td | 78.63 |
td | 78.16 |
td | 71.05 |
td | 71.13 |
td | 61.21 |
td | 67.2 |
td | 66.17 |
tr | Cat 83.15 93.29 83.73 83.98 87.33 81.74 85.71 83.23 83.11 81.49 76.42 72.65 72.25 59.6 67.81 66.79 |
td | Cat |
td | 83.15 |
td | 93.29 |
td | 83.73 |
td | 83.98 |
td | 87.33 |
td | 81.74 |
td | 85.71 |
td | 83.23 |
td | 83.11 |
td | 81.49 |
td | 76.42 |
td | 72.65 |
td | 72.25 |
td | 59.6 |
td | 67.81 |
td | 66.79 |
tr | Camel 81.91 83.85 81.37 89.81 84.72 80.99 85.71 88.2 88.57 79.25 77.29 71.79 72.12 61.34 67.2 66.42 |
td | Camel |
td | 81.91 |
td | 83.85 |
td | 81.37 |
td | 89.81 |
td | 84.72 |
td | 80.99 |
td | 85.71 |
td | 88.2 |
td | 88.57 |
td | 79.25 |
td | 77.29 |
td | 71.79 |
td | 72.12 |
td | 61.34 |
td | 67.2 |
td | 66.42 |
tr | Goat 81.41 81.86 80.37 87.7 82.11 80.12 83.23 88.2 97.76 78.14 76.54 72.04 71.38 60.84 66.09 65.44 |
td | Goat |
td | 81.41 |
td | 81.86 |
td | 80.37 |
td | 87.7 |
td | 82.11 |
td | 80.12 |
td | 83.23 |
td | 88.2 |
td | 97.76 |
td | 78.14 |
td | 76.54 |
td | 72.04 |
td | 71.38 |
td | 60.84 |
td | 66.09 |
td | 65.44 |
tr | Bos mutus 80.92 81.74 80 87.58 81.86 80.37 83.11 88.57 97.76 78.39 77.04 71.42 71.01 61.09 66.09 65.31 |
td | Bos mutus |
td | 80.92 |
td | 81.74 |
td | 80 |
td | 87.58 |
td | 81.86 |
td | 80.37 |
td | 83.11 |
td | 88.57 |
td | 97.76 |
td | 78.39 |
td | 77.04 |
td | 71.42 |
td | 71.01 |
td | 61.09 |
td | 66.09 |
td | 65.31 |
tr | Erinaceus europaeus 77.94 79.75 78.51 77.64 80.87 78.63 81.49 79.25 78.14 78.39 74.56 70.67 69.65 60.59 68.8 66.42 |
td | Erinaceus europaeus |
td | 77.94 |
td | 79.75 |
td | 78.51 |
td | 77.64 |
td | 80.87 |
td | 78.63 |
td | 81.49 |
td | 79.25 |
td | 78.14 |
td | 78.39 |
td | 74.56 |
td | 70.67 |
td | 69.65 |
td | 60.59 |
td | 68.8 |
td | 66.42 |
tr | Cavia porcellus 76.85 75.55 74.56 75.3 77.17 78.16 76.42 77.29 76.54 77.04 74.56 66.61 66.82 59.38 63.9 63.12 |
td | Cavia porcellus |
td | 76.85 |
td | 75.55 |
td | 74.56 |
td | 75.3 |
td | 77.17 |
td | 78.16 |
td | 76.42 |
td | 77.29 |
td | 76.54 |
td | 77.04 |
td | 74.56 |
td | 66.61 |
td | 66.82 |
td | 59.38 |
td | 63.9 |
td | 63.12 |
tr | Phascolarctos cinereus 70.38 71.66 69.68 71.17 71.66 71.05 72.65 71.79 72.04 71.42 70.67 66.61 81.92 60.7 67.08 64.83 |
td | Phascolarctos cinereus |
td | 70.38 |
td | 71.66 |
td | 69.68 |
td | 71.17 |
td | 71.66 |
td | 71.05 |
td | 72.65 |
td | 71.79 |
td | 72.04 |
td | 71.42 |
td | 70.67 |
td | 66.61 |
td | 81.92 |
td | 60.7 |
td | 67.08 |
td | 64.83 |
tr | Sarcophilus harrisii 69.84 71.63 70.02 71.01 70.89 71.13 72.25 72.12 71.38 71.01 69.65 66.82 81.92 60.9 64.62 63.84 |
td | Sarcophilus harrisii |
td | 69.84 |
td | 71.63 |
td | 70.02 |
td | 71.01 |
td | 70.89 |
td | 71.13 |
td | 72.25 |
td | 72.12 |
td | 71.38 |
td | 71.01 |
td | 69.65 |
td | 66.82 |
td | 81.92 |
td | 60.9 |
td | 64.62 |
td | 63.84 |
tr | Tiger Snake 59.21 59.6 58.61 61.09 59.6 61.21 59.6 61.34 60.84 61.09 60.59 59.38 60.7 60.9 64.5 63.71 |
td | Tiger Snake |
td | 59.21 |
td | 59.6 |
td | 58.61 |
td | 61.09 |
td | 59.6 |
td | 61.21 |
td | 59.6 |
td | 61.34 |
td | 60.84 |
td | 61.09 |
td | 60.59 |
td | 59.38 |
td | 60.7 |
td | 60.9 |
td | 64.5 |
td | 63.71 |
tr | Parus major 65.6 67.44 65.11 66.95 67.44 67.2 67.81 67.2 66.09 66.09 68.8 63.9 67.08 64.62 64.5 82.2 |
td | Parus major |
td | 65.6 |
td | 67.44 |
td | 65.11 |
td | 66.95 |
td | 67.44 |
td | 67.2 |
td | 67.81 |
td | 67.2 |
td | 66.09 |
td | 66.09 |
td | 68.8 |
td | 63.9 |
td | 67.08 |
td | 64.62 |
td | 64.5 |
td | 82.2 |
tr | Gallus gallus 64.13 66.3 65.19 65.56 65.07 66.17 66.79 66.42 65.44 65.31 66.42 63.12 64.83 63.84 63.71 82.2 |
td | Gallus gallus |
td | 64.13 |
td | 66.3 |
td | 65.19 |
td | 65.56 |
td | 65.07 |
td | 66.17 |
td | 66.79 |
td | 66.42 |
td | 65.44 |
td | 65.31 |
td | 66.42 |
td | 63.12 |
td | 64.83 |
td | 63.84 |
td | 63.71 |
td | 82.2 |
table-wrap-foot | Accession numbers: human (NP_068576.1), monkey (AY996037.1), civet (AAX63775.1), bat (R.sinicus) (AGZ48803.1), swine (NP_001116542.1), Malayan pangolin (XP_017505752.1), mouse (NP_001123985.1), cat (NP_001034545.1), camel (XP_010991717.1), goat (NP_001277036.1), Bos mutus (XP_005903173.1), Erinaceus europaeus (XP_007538670.1), Phascolarctos cinereus (XP_020863153.1), Sarcophilus harrisii (XP_031814825.1), tiger Snake (XP_026530754.1), Parus major (XP_015486815.1), Gallus gallus (QEQ50331.1). |
footnote | Accession numbers: human (NP_068576.1), monkey (AY996037.1), civet (AAX63775.1), bat (R.sinicus) (AGZ48803.1), swine (NP_001116542.1), Malayan pangolin (XP_017505752.1), mouse (NP_001123985.1), cat (NP_001034545.1), camel (XP_010991717.1), goat (NP_001277036.1), Bos mutus (XP_005903173.1), Erinaceus europaeus (XP_007538670.1), Phascolarctos cinereus (XP_020863153.1), Sarcophilus harrisii (XP_031814825.1), tiger Snake (XP_026530754.1), Parus major (XP_015486815.1), Gallus gallus (QEQ50331.1). |
p | Accession numbers: human (NP_068576.1), monkey (AY996037.1), civet (AAX63775.1), bat (R.sinicus) (AGZ48803.1), swine (NP_001116542.1), Malayan pangolin (XP_017505752.1), mouse (NP_001123985.1), cat (NP_001034545.1), camel (XP_010991717.1), goat (NP_001277036.1), Bos mutus (XP_005903173.1), Erinaceus europaeus (XP_007538670.1), Phascolarctos cinereus (XP_020863153.1), Sarcophilus harrisii (XP_031814825.1), tiger Snake (XP_026530754.1), Parus major (XP_015486815.1), Gallus gallus (QEQ50331.1). |
figure | Fig. 2 Phylogenetic analysis of ACE2 from humans and 18 other animals. The tree was constructed based on the complete amino acid sequences of ACE2s derived from humans and other animals by using the neighbor-joining (NJ) method with 1000 bootstrap. The accession numbers were as the same as Table 2. Bootstrap values above 50 were shown. |
label | Fig. 2 |
caption | Phylogenetic analysis of ACE2 from humans and 18 other animals. The tree was constructed based on the complete amino acid sequences of ACE2s derived from humans and other animals by using the neighbor-joining (NJ) method with 1000 bootstrap. The accession numbers were as the same as Table 2. Bootstrap values above 50 were shown. |
p | Phylogenetic analysis of ACE2 from humans and 18 other animals. The tree was constructed based on the complete amino acid sequences of ACE2s derived from humans and other animals by using the neighbor-joining (NJ) method with 1000 bootstrap. The accession numbers were as the same as Table 2. Bootstrap values above 50 were shown. |
sec | 3.3 Origin of 2019-nCoV The epidemiological investigation of early cases of 2019-nCoV pneumonia showed that many cases had been exposed to the Huanan Seafood Market in Wuhan [2,24,20]. In addition, 33 of the 585 environmental samples from Huanan Seafood Market were positive for 2019-nCoV; however, that market was trading a variety of live animals, such as hedgehog, badger, snake, and bird (turtledoves), probably pangolin, but not bats [24,30]. Thus, bats are less likely to have direct contact with human, and direct transmission of the virus from bat to human is unlikely [1]. Indeed, SARS-CoV and MERS-CoV originated from bats, the natural reservoir, but transmitted to humans via intermediate host civets and camels, respectively. Therefore, 2019-nCoV may also originate from bat but was then transmitted to humans via an intermediate host in the market [[31], [32], [33], [34], [35]]. Currently, 2019-nCoV has been isolated from pangolins and it was found that the genomic seuqence of the isolated strain had 99% identity with that of currently 2019-nCoV infected humans. It has been proposed that the transmission and evolution path of 2019-nCoV was from bat-CoV to pangolins (the intermediate hosts), from where it infected humans [36]. |
label | 3.3 |
title | Origin of 2019-nCoV |
p | The epidemiological investigation of early cases of 2019-nCoV pneumonia showed that many cases had been exposed to the Huanan Seafood Market in Wuhan [2,24,20]. In addition, 33 of the 585 environmental samples from Huanan Seafood Market were positive for 2019-nCoV; however, that market was trading a variety of live animals, such as hedgehog, badger, snake, and bird (turtledoves), probably pangolin, but not bats [24,30]. Thus, bats are less likely to have direct contact with human, and direct transmission of the virus from bat to human is unlikely [1]. Indeed, SARS-CoV and MERS-CoV originated from bats, the natural reservoir, but transmitted to humans via intermediate host civets and camels, respectively. Therefore, 2019-nCoV may also originate from bat but was then transmitted to humans via an intermediate host in the market [[31], [32], [33], [34], [35]]. Currently, 2019-nCoV has been isolated from pangolins and it was found that the genomic seuqence of the isolated strain had 99% identity with that of currently 2019-nCoV infected humans. It has been proposed that the transmission and evolution path of 2019-nCoV was from bat-CoV to pangolins (the intermediate hosts), from where it infected humans [36]. |
sec | 4 Diagnosis and treatment Diagnosis of coronaviruses is unnecessary in most cases of self-limited infection, as most of the patients have mild or moderate syndromes with good prognosis. However, it may be essential to identify an etiological agent in epidemiological studies, especially during the epidemics outbreak. Since 2019-nCoV has not been found before in humans, there is no vaccine or special treatment for it so far. The number of cases is increasing rapidly, even under the current public health emergency. Thus, diagnosing all suspected cases as soon as possible and immediately isolating them is critical to cutting off the source of infection. Conventional diagnostic testing methods, such as the assays for detection of antiviral antibodies or viral antigens have been developed and used clinically. New diagnostic strategies including multiplex nucleic acid amplification and microarray-based assays may help in epidemiologic monitoring, along with taking effective prevention steps [37,38]. 2019-nCoV nucleic acids can be detected in specimens including nasopharyngeal swabs, sputum, lower respiratory tract secretions, blood and stool [20]. There are currently no approved antiviral treatments or vaccines for human coronavirus infections. The development of safe, stable vaccines is a huge challenge and vaccines would ideally be broad-spectrum, so the research and development of new drugs is a very long process. In such a sudden epidemic, scientists were not able to carry out new drug development by the traditional principles. Therefore, there is another option: a systematic and large-scale screening of existing drugs to see whether they have activity against the 2019-nCoV. Recently drug screening found that nelfinavir has potential antiviral activity against 2019-nCoV. In addition, pitavastatin, perampanel, and praziquantel might also have moderate activities against 2019-nCoV [39]. Based on previous studies, an anti-HIV drug named Kaletra may have therapeutic effect on SARS and MERS, which is composed of two protease inhibitors, ritonavir (CAS#: 155,213-67-5) and lopinavir (CAS#: 192,725-17-0) [[40], [41], [42], [43], [44]]. More recently, Kaletra was also recommended to treat Wuhan pneumonia by the National Health Commission of the People’s Republic of China. Patients with SARS or MERS have used several drugs including ribavirin, interferon, lopinavir-ritonavir, and corticosteroids, but the efficacy of certain drugs is still controversial. Other antiviral drugs, like FAD-approved drugs including ribavirin, penciclovir, nitrazine, nalfamusta, chloroquine, and the two broad-spectrum antiviral drugs redexivir (GS-5734) and favivir (T-705), are being evaluated by measuring the effects of these compounds on cytotoxicity, virus yield and infection rate of 2019-nCoV. Recent results showed that remdesivir and chloroquine are effective in controlling 2019-nCoV infection in vitro and may be evaluated in human patients with 2019-nCoV disease [45]. Remdesivir has recently been recognized as a promising antiviral drug against multiple RNA viruses (including SARS/MERS-CoV) in cultured cells, and in mouse and non-human primate (NHP) models. Currently remdesivir for the treatment of Ebola virus infection is in clinical research. It is promising that these compounds can treat 2019-nCoV infections. Moreover, the fifth edition of infection prevention and control (IPC) guidance announced that severe and critically ill patients could be treated with recovery plasma. |
label | 4 |
title | Diagnosis and treatment |
p | Diagnosis of coronaviruses is unnecessary in most cases of self-limited infection, as most of the patients have mild or moderate syndromes with good prognosis. However, it may be essential to identify an etiological agent in epidemiological studies, especially during the epidemics outbreak. Since 2019-nCoV has not been found before in humans, there is no vaccine or special treatment for it so far. The number of cases is increasing rapidly, even under the current public health emergency. Thus, diagnosing all suspected cases as soon as possible and immediately isolating them is critical to cutting off the source of infection. Conventional diagnostic testing methods, such as the assays for detection of antiviral antibodies or viral antigens have been developed and used clinically. New diagnostic strategies including multiplex nucleic acid amplification and microarray-based assays may help in epidemiologic monitoring, along with taking effective prevention steps [37,38]. 2019-nCoV nucleic acids can be detected in specimens including nasopharyngeal swabs, sputum, lower respiratory tract secretions, blood and stool [20]. |
p | There are currently no approved antiviral treatments or vaccines for human coronavirus infections. The development of safe, stable vaccines is a huge challenge and vaccines would ideally be broad-spectrum, so the research and development of new drugs is a very long process. In such a sudden epidemic, scientists were not able to carry out new drug development by the traditional principles. Therefore, there is another option: a systematic and large-scale screening of existing drugs to see whether they have activity against the 2019-nCoV. Recently drug screening found that nelfinavir has potential antiviral activity against 2019-nCoV. In addition, pitavastatin, perampanel, and praziquantel might also have moderate activities against 2019-nCoV [39]. Based on previous studies, an anti-HIV drug named Kaletra may have therapeutic effect on SARS and MERS, which is composed of two protease inhibitors, ritonavir (CAS#: 155,213-67-5) and lopinavir (CAS#: 192,725-17-0) [[40], [41], [42], [43], [44]]. More recently, Kaletra was also recommended to treat Wuhan pneumonia by the National Health Commission of the People’s Republic of China. Patients with SARS or MERS have used several drugs including ribavirin, interferon, lopinavir-ritonavir, and corticosteroids, but the efficacy of certain drugs is still controversial. Other antiviral drugs, like FAD-approved drugs including ribavirin, penciclovir, nitrazine, nalfamusta, chloroquine, and the two broad-spectrum antiviral drugs redexivir (GS-5734) and favivir (T-705), are being evaluated by measuring the effects of these compounds on cytotoxicity, virus yield and infection rate of 2019-nCoV. Recent results showed that remdesivir and chloroquine are effective in controlling 2019-nCoV infection in vitro and may be evaluated in human patients with 2019-nCoV disease [45]. Remdesivir has recently been recognized as a promising antiviral drug against multiple RNA viruses (including SARS/MERS-CoV) in cultured cells, and in mouse and non-human primate (NHP) models. Currently remdesivir for the treatment of Ebola virus infection is in clinical research. It is promising that these compounds can treat 2019-nCoV infections. Moreover, the fifth edition of infection prevention and control (IPC) guidance announced that severe and critically ill patients could be treated with recovery plasma. |
sec | 5 Conclusions and future perspectives The 2019-nCoV epidemic continues. During the past month, huge progress has been made in pathogen monitoring, identifying sources, basic etiology, clinical treatment, drug testing, and vaccine development. With the efforts of China and the helps of other countries in the world, we hope that the epidemic will subside soon. Future research on viral replication, pathogenesis, antiviral drugs and other aspects of 2019-nCoV will contribute to the treatment and prevention of the virus. However, given the emergence of 2019-nCoV pneumonia as a new infectious disease with interspecies transmission from animals, we should reflect on the origin of the human pathogen and learn from our experience. With changes of ecology and human activities, including unlimited invasion of natural habitats of animals, consumption of some of these animals, and modern agricultural practices, the spillover of viruses from natural hosts to humans continues to appear and may even be escalating. Although we have built an unparalleled human civilization and a highly developed information society, invisible viruses can still have devastating effects on human beings. The high lethality viruses such as SARS-CoV, MERS-CoV, H5N1, H7N9, Ebola and emerging 2019-nCoV should serve as an alarm to the world. We should strive to reduce the probability of their occurrence and harm. Strengthening the investigation of animal etiology, normalizing the storage of high risk pathogens, reducing direct contact with wildlife, maintaining the barriers between natural reservoirs and human society and completely eradicating wildlife trading will help to achieve these goals. |
label | 5 |
title | Conclusions and future perspectives |
p | The 2019-nCoV epidemic continues. During the past month, huge progress has been made in pathogen monitoring, identifying sources, basic etiology, clinical treatment, drug testing, and vaccine development. With the efforts of China and the helps of other countries in the world, we hope that the epidemic will subside soon. Future research on viral replication, pathogenesis, antiviral drugs and other aspects of 2019-nCoV will contribute to the treatment and prevention of the virus. However, given the emergence of 2019-nCoV pneumonia as a new infectious disease with interspecies transmission from animals, we should reflect on the origin of the human pathogen and learn from our experience. With changes of ecology and human activities, including unlimited invasion of natural habitats of animals, consumption of some of these animals, and modern agricultural practices, the spillover of viruses from natural hosts to humans continues to appear and may even be escalating. Although we have built an unparalleled human civilization and a highly developed information society, invisible viruses can still have devastating effects on human beings. The high lethality viruses such as SARS-CoV, MERS-CoV, H5N1, H7N9, Ebola and emerging 2019-nCoV should serve as an alarm to the world. We should strive to reduce the probability of their occurrence and harm. Strengthening the investigation of animal etiology, normalizing the storage of high risk pathogens, reducing direct contact with wildlife, maintaining the barriers between natural reservoirs and human society and completely eradicating wildlife trading will help to achieve these goals. |
sec | Declaration of Competing Interest The authors declare that they have no conflict of interest. |
title | Declaration of Competing Interest |
p | The authors declare that they have no conflict of interest. |
back | Acknowledgements This work was jointly funded by the National Key Research and Development Program of China (grant number 2017YFD0500104), National Natural Science Foundation of China (31470260), and the Science Fund for Distinguished Young Scholars of Hunan Province (grant number 2019JJ20004). |
ack | Acknowledgements This work was jointly funded by the National Key Research and Development Program of China (grant number 2017YFD0500104), National Natural Science Foundation of China (31470260), and the Science Fund for Distinguished Young Scholars of Hunan Province (grant number 2019JJ20004). |
title | Acknowledgements |
p | This work was jointly funded by the National Key Research and Development Program of China (grant number 2017YFD0500104), National Natural Science Foundation of China (31470260), and the Science Fund for Distinguished Young Scholars of Hunan Province (grant number 2019JJ20004). |
Annnotations TAB TSV DIC JSON TextAE
last updated at 2021-04-26 01:47:10 UTC
- Denotations: 2
- Blocks: 0
- Relations: 0