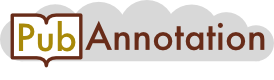
CORD-19:01548ef6d46d6e6d72afe8cbf4e231552b9d2bd7 / 12027-12199 3 Projects
The Presence of Viruses in Uninoculated Tissue Cultures: Sources and Methods of Detection
Abstract
It is a commonplace of in vitro studies of cells that, besides mycoplasmas (Barile, 1968) , tissue cultures often contain extraneous viruses. The latter can come from medium ingredients that have a biological origin, such as serum (Kniazeff, this volume) or trypsin (Hallauer et al., 1971) , can result from accidental contamination of the cultures by the technicians who handle them (Collier, 1957) , or can be introduced in association with the cells used to initiate the cultures (Hsiung, 1968) , the latter being particularly likely to occur when primary tissue cultures are used (Kalter and Heberling, 1968 ). The first two sorts of viral contamination can be considered trivial from a biological point of view, because they constitute a peculiar hazard of the circumstances in which tissue culture is carried out and can be expected to be eliminated when a foolproof methodology is adopted. The third source from which viruses can be unknowingly introduced, namely those viruses inherent to the cultured tissues themselves, is the one with which this chapter is con-cerned. It cannot be considered trivial, because the large extent of its probable occurrence raises the question of whether the presence of viruses can be considered an inevitable, or perhaps essential, accompaniment of the propagation of cells in vitro, especially when continuous cell lines are to be established.
Very many animal species have hidden virus infections, that is, viruses that appear to exist in close association with particular tissues or organs of the species involved, without causing overt symptoms. It follows that primary cultures prepared from almost any tissue may be found infected with viruses in vitro simply because the organ from which they were prepared was infected in vivo (Hsiung, 1968) . In this way, primary cultures from chickens (Burke et al, 1965) , chimpanzees (Rogers et al., 1967) , dogs (Smith et al., 1970) , ducks (Luginbuhl, 1968) , guinea pigs (Hsiung and Kaplow, 1969) , hamsters (Stenback et al., 1966) , horses , man (Hsiung, 1968) , rabbits (Nesburn, 1969) , rats (Melendez et al., 1967) , monkeys (Hsiung, 1968) , and swine (Huygelen and Peetermans, 1967) have all been found to yield viruses spontaneously. More than one agent has been found in the same primary culture (Hsiung, 1968) . Primates have been more intensively studied in this respect that any other group of species, perhaps because of the widespread use of viral vaccines. Tumor cell cultures are also a rich source of adventitious viruses and, in view of what is often an obvious lack of relationship to the etiology of the tumor from which they have been derived, have been termed passenger viruses. By reason of their capacity for continuous synthesis and replication, tumor cells have been assumed to provide a favorable milieu for the multiplication of viruses and therefore to be especially likely to harbor such passengers. At the present time, it may be preferable to restrict the use of the term passenger to viruses carried into culture with the explanted tissue in the form of fully infectious extracellular virions that subsequently become capable of invading and replicating within cells of the culture. In the most obvious cases, this type of association will eventually be manifested as a frank cytopathic effect, as seems to occur with passenger adenoviruses in human adenoids (Rowe et al, 1956 ) and the foamy viruses that are often found in monkey kidney cultures (Hsiung, 1968) . The cytopathic effects observed in vitro may sometimes result from the acquisition of susceptibility to infection and lysis by cells that were originally resistant to the virus in vivo. A striking illustration of an effect on the virus-cell relationship brought about by altering the cells' environment has been given by Kaplan ( 1955) . When monkey kidneys are infected in vivo with polio virus, no cytopathic effect can be observed and no virus can be recovered following subsequent perfusion of the kidney or homogenization of its tissue. However, if parts of an infected kidney found negative for virus by such tests are reduced to cell suspensions by trypsin treatment and used to set up conventional monolayer cultures, cell destruction eventually takes place, accompanied by the appearance of fully infective virus. When the kidney cells are removed from their in vivo situation to an alien environment, they somehow acquire susceptibility to polio virus. The nature of the mechanisms involved-whether an actual change in the cells themselves or merely their removal from a protected environment, such as would be provided by antibody-remains unknown. The so-called conditional lethal mutants are further examples of how extraneous conditions can affect the response of cells to infection. Many strains of polio virus (Cooper, 1964) are now known that multiply normally at 37°C but do not complete their multiplication cycle when incubated at 39.5°C. Such a virus will not be apparent at the higher temperature but will emerge, accompanied by a cytopathic effect, when the temperature is lowered. It is not suggested that temperature-dependent viruses of this type are widespread in nature-most of those known are selected laboratory mutants-but they are mentioned here to illustrate the point that appropriate culture conditions may allow hidden viruses to emerge that would not otherwise be detected.
A passenger association of a similar kind, although a less obvious one, occurs when only a small number of the cells in the culture are or become susceptible to the virus, although the latter is carried into the culture in its fully infectious form. Virus replication will occur, although sufficient cells will not be destroyed at any one time for the culture to show a cytopathic effect. However, the virus never enters a truly latent form. An association of this type between the paramyxovirus SV5 and human fibroblasts has been described by Wong and Kilbourne (1961) . Three different kinds of carrier cultures of this type have also been established deliberately.
/. In the first, almost all the cells in the culture are genetically resistant to the virus, which then replicates only in a minority of susceptible cells. Examples of this type are Coxsackie virus A9 in HeLa cells (Takemoto and Habel, 1959) and polio virus type 3 in HeLa cells (Vogt and Dulbecco, 1958) .
2. In this type of association, all the cells remain susceptible but the spread of the virus is restricted by antibody in the medium, e.g., herpes simplex virus in HeLa cells (Wheeler, 1960) .
A third type is one in which the cells also retain genetic susceptibility but most of them become refractory through the action of interferon produced within the culture, as with Newcastle disease virus infection of murine L cells (Henle, 1963) .
It is doubtful, though, whether the term passenger should be extended to include every virus that appears fortuitously in primary cultures of tissues. A tabulation of recent reports of such isolations given by Smith (1970) shows that they include agents, such as members of the herpes group, that are often present in vivo in a latent noninfectious form, even in the presence of circulating humoral antibody (Andrewes, 1967) . Latent herpes simplex virus infection can be activated by a number of nonspecific stimuli, after which completed virions are released by infected skin cells that show a cytopathic effect (Fenner, 1968) . It is unlikely that the herpes-type viruses that appear predominantly in primary tissue cultures all represent populations stemming from complete virions introduced to the cultures along with the cells. Many may represent latent herpesvirus genomes activated as a result of the stress of culture initiation. In a number of cases, tissue culture propagation for many transfers is necessary before a virus emerges. Primary cultures of cells from children with Burkitt's African lymphoma rarely release Epstein-Barr virus (EBV); its presence cannot be demonstrated with the electron miscroscope, either in the tumor itself or in the early stages of its culture history. Nor do the cells at this stage contain viral antigens detectable by indirect immunofluorescence using EBV-positive human sera. However, following a number of transfers in vitro, cells from many such cultures can be shown to contain microscopically identifiable viral structures and a proportion of them now show positive immunofluorescence (Henle and Henle, 1966) . The virus contained within such cells can also be caused to increase in amount by subjecting the cultured cells to stresses of various kinds, such as argininedepleted media (Henle and Henle, 1968) or a suboptimal temperature of cultivation (Horoszewicz et al., 1968) .
Acquisition of an identifiable herpes-like virus following passage is not a unique property of cells from Burkitt's African lymphoma and has been shown to occur with many floating lymphoid cell lines of varied origin . Indeed, it has been stated that all such continuously transferable lines carry a herpes-like virus (Gerber et al, 1970) . In this instance, the recent establishment from cases of Hodgkin's disease (Eisinger et al., 1971 ) of lymphoblastoid cell lines, capable of growth in suspension and carrying a herpes-like virus resembling, but not identical with, Epstein-Barr virus, is of interest, although there is as yet no evidence of a connection between this virus and the etiology of Hodgkin's disease. In certain other human tumor culture systems, prolonged cultivation may be necessary before antigenic and other changes, possibly indicative of the emergence of viruslike agents, can take place (Sabin, 1965) . It is therefore proposed that the term indigenous rather than passenger be used to describe this group of viruses, indigenous viruses being defined as those that are frankly pathogenic in the appropriate circumstances [Epstein-Barr virus has been identified as the cause of infectious mononucleosis (Henle et al, 1968; Hirshaut et al., 1969) ; herpesvirus Β is latent in the rhesus monkey but causes fatal disease in man (Andrewes, 1964) ] but that are normally introduced into tissue cultures within latently infected cells, later to be induced to proliferate by stimuli at present unknown.
Cells in tissue culture may have viruses associated with them in a third way, which we may term cryptic. Cryptic viruses remain integrated within their host cells throughout their life in culture. They do not cause a cytopathic effect under normal circumstances and do not often become induced or activated under the normal conditions of culture. Naturally, this is a broad class that includes associations between viruses and cells of widely varying degrees of intimacy. On the one hand, there are cryptic viruses that closely resemble indigenous viruses in that prolonged cultivation causes their emergence in identifiable form, although without cytopathic effect. Examples are the type C viral particles that frequently appear in cultures of mouse tissues following prolonged cultivation (Aaronson etal., 1969) . On the other hand, are cells infected by viruses, the only evidence of whose presence is an alteration of cellular properties, such as the acquisition of malignancy [Nil 2E cells transformed by the hamster sarcoma virus HaSV (Albino and Sanders, 1972) ]. An intermediate category is typified by the association between SV40 virus and nonpermissive mouse cells or between Polyomavirus and hamster kidney cells (BHK 21 ) (Dulbecco, 1968) in which the viral presence is signaled not only by altered cellular properties but also by the manufacture of virally specified antigens and the possibility of rescuing complete extracellular virus by fusion with permissive cells (Koprowski et al., 1967) . Temperature-sensitive variants of Polyomavirus are also known that affect the expression of this virus when in the cryptic state (Dulbecco, 1969) . Cells incubated at the nonpermissive temperature appear normal; they do not have the transformed morphology and mode of growth that stamp them as carrying the viral genome. When shifted to the permissive temperature, they transform but, unlike the conditional lethal mutants of polio virus mentioned earlier, do not proceed to the production of complete virus. Treatment of cultured cells with certain nucleoside analogs (bromoor iododeoxyuridine) can cause the activation of apparently cryptic viral RNA genomes, resulting in the appearance of products varying from special antigens thought to be virally specified (Weiss et al., 1971) up to complete infective type C oncornavirus particles (Lowy et al., 1971; Aaronson etal., 1971) . Similar chemical treatment of cells carrying cryptic DNA virus genomes (SV40-transformed 3T3 mouse fibroblasts; Watkins, 1970) causes many more cells to become capable of making virus upon fusion with permissive cells than when they are not so treated.
host cells have been taken from cases in which the association has been deliberately established by treating cells with viruses in tissue culture and then showing later that a cryptic type of association has been set up. There is, however, no a priori reason for assuming that associations of this kind do not occur in vivo and that cells detached from whole organisms and subsequently cultivated in vitro will not prove to be cryptically infected. That this may be so is indicated by the observation that cells derived from tumors caused by the inoculation of polyoma or SV40 viruses into infant hamsters, and subsequently cultured do not manufacture virus but can be shown, nevertheless, to contain viral genomes because complete virus may be rescued by fusion with permissive cells . The transfer of cells from the in vitro to an in vivo situation can show a similar effect. Syrian (Vigier, 1970) or Chinese hamster (Hlozanek et al., 1966) or rat cells (Svoboda, 1964) transformed morphologically in vitro by means of Rous sarcoma virus show a cryptic association between virus and cell, for their injection into chickens results in the growth of a tumor consisting of chicken cells that release the same sarcoma virus.
For over 10 years it has been widely believed that cell cultures initiated from normal tissues have a limited lifespan in vitro (Hayflick, 1965) . Two main hypotheses have been advanced to account for this condition.
1. It can result from inadequacy of the medium used and/or the unnatural stresses to which cells are subject during manipulation in culture (Puck et al., 1958; Todaro and Green, 1964) , as well as from the particular schedule adopted for subculture (Hay, 1970) .
2. Cell cultures exhibit aging in vitro, the time of onset of which reflects the physiological age in vivo of the cells of the tissue from which the cultures were initiated. Each tissue cell is conceived as having an inborn potential for a finite number of cell divisions. Once the limit is reached, the cell dies, supposedly owing to accumulated copying errors in its DNA that lead to associated mistakes in protein synthesis (Orgel, 1963; Hayflick, 1966) .
At the present time, it is still uncertain whether either of these hypotheses is correct. There is a clear difference between species with regard to the ability of their cells to propagate indefinitely in culture. Human diploid fibroblasts appear generally to have a limited lifetime in vitro (Hayflick, 1965) ; those from rats appear capable of long-term cultivation without loss of growth potential (Petursson et al., 1964) . Cells from mice, hamsters, and many other species frequently give rise to cell lines capable of continuous cultivation, the properties of which, such as saturation density and oncogenic potential, depend on the seeding density and transfer interval adopted for their propagation (Macpherson and Stoker, 1962; Todaro and Green, 1963) . Many have a tendency to become heteroploid (e.g., mouse) at the time a capacity for continuous transfer is acquired.
Tissues from other species, such as the Chinese hamster (Yerganian and Leonard, 1961; Hsu et al., 1964) , also readily give cell lines that can be continuously propagated in vitro, although here the cells tend to become pseudodiploid rather than heteroploid when continuously maintained.
The possibility of establishing continuous lines with cells from these species does not seem to depend on their physiological age at the time of explantation. With the mouse, as with both the Chinese and the Syrian hamster, cell lines have been readily established from embryos (Todaro and Green, 1963; Diamond, 1967) , new borns (MacPherson and Stoker, 1962) , and adults (Earle, 1943; Hsu et al., 1964) with equal facility.
The mode of origin of the abnormal chromosome complements of continuous cell lines, whether by selection or by some other means, is at present uncertain. However, it has been claimed that it can itself be a consequence of employing defective media and that by using the proper regimen, continuous lines of euploid cells can be established, even from humans (Puck et ai, 1958).
It has not been ruled out, however, that the ability of tissues to generate continuous cell lines may be linked with the occurrence of indigenous or cryptic viruses. As already mentioned, many human continuous lymphoid cell lines carry a virus of the herpesvirus groups (Gerber et al., 1972) . A few that contain no virus detectable by immunofluorescence or electron microscopy [e.g., Raji cells derived from a Burkitt lymphoma (Pulvertaft, 1965) , NHDL 3 cell cultures from normal human leukocytes (Gerber and Monroe, 1968) , NC 37 from leukocytes of a patient with pneumonia (see Gerber, 1972) ] have been shown to contain cryptic virus by cloning (Maurer et ai, 1969) , nucleic acid hybridization (Zur Hausen andScholte-Holthausen, 1970), complement fixation (Vonka et al., 1970) , or activation by bromodeoxyuridine (BrdU) (Gerber, 1972) .
The readiness with which murine tissues give rise to continuous cell lines can be correlated with the presence of cryptic genomes of type C RNA viruses. Activation of the latter can take place spontaneously in cells propagated in vitro (Aaronson et al, 1969) and in mice as the result either of aging or of certain carcinogenic stimuli (Kaplan, 1967) . Treatment with halogenated pyrimidines can be used to reveal the presence of such viruses in clonal lines of cells from both high and low leukemia incidence strains of mice (Lowy et 0/., 1971 ; Aaronson et al., 1971) . Indeed, cells from embryos of the high leukemic AKR strain of mice normally grow in culture as virusnegative cell lines. In vivo they develop a capacity to produce virus, for cells from newborns or older mice can be shown to release virus when cultured.
Although the above information indicates strongly that mouse cells may contain cryptic type C viral genomes that can be activated by various means, there is as yet no direct evidence that activation of the latter confers upon the cells a capacity to propagate indefinitely in vitro. An indirect suggestion that this may be so, however, is given by the work of Aaronson et al. (1969) , who carried cultures of murine fibroblasts according to the 3T3 schedule of culture transfer. Most of their cultures began to produce type C virus particles after about 35 passages in culture, which represents roughly 50-70 cell generations, the time at which cell cultures are said to enter crisis and then either die out or give rise to continuous cell lines. The question thus remains open as to whether indigenous or cryptic viruses invariably accompany an ability to grow continuously in cell culture, and its answer must await further experiment. In the meantime, it must be kept in mind that the presence of viral particles of abnormal morphology, such as the corona viruses common to hamster cells (Bernhard and Tournier, 1964) , may be a normal feature of cells in culture and not the result of contamination by some extraneous agent.
It is also necessary to be cautious regarding the relationship between the acquisition by cells of hetero-or pseudodiploidy and the onset of the ability to propagate continuously in culture. Viral infection of cells has been shown in many instances to be accompanied by karyotypic changes (Hampar and Ellison, 1963; Nichols et al., 1962; Shein and Enders, 1962; Koprowski etal., 1962; Black and Rowe, 1963) , so that the chromosomal alterations observed at the initiation of continuous cell lines may be secondary to the real cause of the phenomenon.
Apart from the possible role of viruses in the establishment of normal tissue cultures, it is necessary to consider the extent to which the presence of passenger, indigenous, or cryptic viruses can invalidate experimental observations. To the virologist, in particular the viral geneticist, the absence of passenger viruses would seem essential.
The widespread adoption of tissue culture methods in the 1950's brought a curious paradox into modern virology in that we now often have to look for a disease to fit a given virus, rather than the other way round. A great many viruses are now known that can be associated with no known disease, and with some of them it is quite uncertain whether they originated from the tissues used to set up the cultures used or from the materials that were later used to inoculate them. Not only are passenger viruses an obvious source of error when tissue cultures are employed in attempts to isolate viruses from clinical material but extraneous agents can also confuse the results of experiments undertaken to study the behavior of human viruses in tissue culture, by suppressing (Hoggan et al., 1966) or even stimulating (Rabsoneia/., 1964) the multiplication of the agent under study. It is, however, in genetic experiments that their presence is most likely to lead to false conclusions. Hybridization, at the level of the genetic material, between viruses growing in the same cell is well known and subsequent replication of the hybrid agent can give rise to virions of altered properties (Rapp et al., 1965) . Virus stocks containing virions with drastically changed properties can also arise by transcapsidation (Hanafusa et al., 1964) , a condition where the genetic material of one virus becomes enclosed within the protein coat of another. Compared with passenger viruses, indigenous and cryptic agents are more difficult to detect. Indeed, in some cases they may remain undetected during many tissue culture passages. The recovery of viruses following prolonged cultivation of material from human tumors or as a result of exposure of the latter's cells to an alien environment, such as that provided by the tissues of another species (McAllister et al., 1971) , can give rise to false hopes that a tumor etiologic agent has been isolated, whereas in reality an indigenous latent virus has been stimulated to proliferate. Positive chemical stimulation of tumor cells to release hitherto cryptic agents is likewise of doubtful significance, unless it can be shown that normal cells from the same tissue in the same individual do not also respond by the production of virus and/or antigens when so stimulated. Like passenger viruses, stimulated indigenous or activated cryptic viruses have potential to affect the replication of known viruses added to the culture and can also be concerned in hybridization and transcapsidation (Rapp et al., 1965) . The experimental virologist thus has the need to know that his cells are free from all three classes of agents before he can make an unequivocal interpretation of his experimental findings. Methods for the recognition of indigenous and cryptic virus infections have been suggested by Fogh et al. (1971) and will be mentioned in Section V.
The possibility that cryptic viral genomes are already present in the cells used to support virus growth raises difficulties in the interpretation of cell transformation and viral replication brought about by type C oncornaviruses, in particular when an attempt is made to discover the part played by RNA-dependent DNA polymerases (Baltimore, 1970; Temin andMizutani 1970) with respect to these processes. Following demonstration in cell-free systems, it is widely accepted that these enzymes, by means of an RNA-DNA hybrid intermediate, can transcribe part or all of the information in the viral RNA into a DNA copy that then becomes integrated into the host cell DNA, causing cell transformation followed by maintenance of the trans-formed state when this material is replicated in unison with the cellular DNA. In productive infection, it may also serve as a template for the production of further copies of the viral RNA. In support of the theory originally put forward by Huebner and , there is now evidence that normal mouse cells, even from strains with a low incidence of leukemia (Lowy et al, 1971; Aaronson et al, 1971 ), already contain the information for the production of MuLV virions for whose replication they are commonly used as substrate. Similarly, cells from uninfected embryos of the kind used for studying the behavior of avian sarcoma viruses can be induced to form virally specified antigens by treatment with IUdr (Weiss et al., 1971) . Infection of these cells with the appropriate oncornaviruses constitutes a kind of superinfection of cells already cryptically carrying a related viral genome, and the mode of replication of these viruses becomes hard to visualize at a biochemical level. In the case of the type C RNA viruses, it is even possible to suggest that perhaps cancer causes viruses rather than the other way around ! The presumably common occurrence of indigenous and cryptic infection among cells from continuous cell lines as well as early passage cell strains suggests that it is probably safer for the experimenter to assume their presence rather than otherwise.
Viruses defined as passengers by the criteria adopted prove easy to detect. Once the presence of a particular virus is presumed, inoculation of homogenates of suspected cultures into cells known to respond cytopathically should reveal it. In some instances, such cells may be hard to find. Murine leukemia viruses multiply exuberantly in cultures of mouse fibroblasts without cytopathic effect and could thus remain undetected. Cocultivation of the cells of suspected cultures with rat XC fibroblasts (transformed by and cryptically carrying the genome of Rous sarcoma virus) reveals the presence of this virus by means of a cytopathic effect on the latter cells (Klement et al., 1969) . Direct examination of both cultured cells and gradient-centrifuged tissue culture supernatants with the electron microscope, using both thin sectioning and negative staining techniques, should also serve to reveal passenger viruses. However, the relative insensitivity of such methods may fail to reveal minimal contamination. Passenger viruses that do not have an obvious cytopathic effect, such as certain myxoviruses, might be detected by their ability to induce interferon production, causing the appearance of resistance to infection by an interferon-sensitive cytopathic agent, such as vesicular stomatitis or encephalomyocarditis virus. Myxo-or paramyxoviruses may also be demonstrated directly through hemadsorption (Kilbourne, 1969; Marcus and Carver, 1969) . The revelation of indigenous or cryptic viruses may require more sophisticated methods. Prolonged cultivation has already been mentioned as an aid to the evocation of indigenous viruses. Other techniques might include the cocultivation and/or fusion (using ß-propiolactone-inactivated Sendai virus) of cells with indicator cells thought to be permissive for the virus suspected. In this instance, properties of the virus other than its ability to replicate and cause cell destruction could be used to devise a detection system; for example, such cells as skin fibroblasts from human cases of Fanconi's anemia (Todaro et al., 1966) , Down's syndrome (Todaro and Martin, 1967) or Bloom's syndrome (German et al., 1965) may be sensitive indicators for transforming viruses. Cocultivation and cell fusion, combined with chemical activation by nucleoside analogs and, perhaps, water-soluble mutagens and carcinogens Igel etal., 1969) , may prove useful for the detection of cryptic viruses, as will the use of helper viruses to call forth hidden viral genomes from cultured cells. Feline leukemia virus (FeLV) would be an obvious choice, from its ability to multiply without cytopathic effect in many types of mammalian cells. Its most important property isthat it can form a hybrid with murine sarcoma virus that can then infect cat cells and be sarcomagenic for this species. FeLV may thus be used to call forth cryptic sarcoma virus genomes that could then be detected by their effect in cats. More direct tests for indigenous or cryptic viruses or their intracellular manifestations will be to search, by immunofluorescence, for the presence of antigens associated with a specific group of viruses, using selected antiserums. Immunofluorescence may also be used to detect the results of activating cryptic viral genomes by any of the methods suggested in the last paragraph. Less certain, but giving suggestive results, is the technique of molecular hybridization. It can be used in two ways.
1. The first is to see whether messenger RNA (mRNA) from cells suspected of harboring a cryptic virus hybridizes with nucleic acids from viruses already known. Using this method, an RNA specific for adeno-and papovaviruses has been found in tumors induced by these viruses.
2. In the case of type C oncornaviruses, the viral RNA-dependent DNA polymerase has been used to make a "DNA" in vitro, using the viral RNA itself as a template. This DNA is then used as a probe to search for cellular RNA's resembling the viral RNA in uninfected cells (Hehlmann et al., 1972) .
Possibly the best way to determine whether a passenger, indigenous, or activated cryptic virus in a particular tissue culture system is complicating the results of experiments is to determine whether the viral yields comprise a mixed population of virions. This can be done by analyzing the infective dose-response curve to see whether it obeys single-or multiple-hit kinetics. In the case of such systems as MuLV-MSV or RSV-RAV, where the presence of a helper virus is obligatory, double-hit kinetics is the rule. However, it should be pointed out that even in these systems, the dose-response curve has the appearance of being single-hit once the helper virus is present in great excess, so that its presence will not be suspected if this is the only means of testing used.
|
Annnotations
- Denotations: 1
- Blocks: 0
- Relations: 0