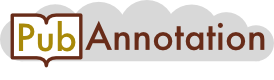
CORD-19:12dd53397f3ddd026cfbfe6dfd1aaefc565b6aeb / 10189-10268 2 Projects
Simultaneous Detection of Antibodies to Mouse Hepatitis Virus Recombinant Structural Proteins by a Microsphere-Based Multiplex Fluorescence Immunoassay ᰔ Downloaded from
Abstract
We describe a new microsphere-based multiplex fluorescent immunoassay (MFI) using recombinant mouse hepatitis virus (MHV) proteins to detect antibodies to coronaviruses in mouse and rat sera. All the recombinant proteins, including nucleocapsid (N) and 3 subunits of spike protein, S1, S2, and Smid, showed positive reactivity in MFI with mouse antisera to 4 MHV strains (MHV-S, -A59, -JHM, and -Nu67) and rat antiserum to a strain of sialodacryoadenitis virus (SDAV-681). The MFI was evaluated for its diagnostic power, with panels of mouse sera classified as positive or negative for anti-MHV antibodies by enzyme-linked immunosorbent assay (ELISA) using MHV virion antigen and indirect fluorescent antibody assay. The reactivities of 236 naturally infected mouse sera were examined; 227 samples were positive by MFI using S2 antigen (96% sensitivity), and 208 samples were positive using N antigen (88% sensitivity). Based on the assessment by MFI using the S2 and N antigens, only 3 serum samples showed double-negative results, indicating a false-negative rate of 1.3%. In 126 uninfected mouse sera, including 34 ELISA false-positive sera, only 7 samples showed false-positive results by MFI using either the S2 or N antigen (94% specificity). Similarly, the S2 and N antigen-based MFI was 98% sensitive and 100% specific in detecting anticoronavirus antibodies in rat sera. Thus, this MFI-based serologic assay using the S2 and N antigens promises to be a reliable diagnostic method, representing a highly sensitive and specific alternative to traditional ELISA for detection of coronavirus infections in laboratory mouse and rat colonies.
Mouse hepatitis virus (MHV) is one of the most prevalent infectious agents of laboratory mice. At present, the most widely used methods for diagnosis of MHV infections are serological tests by enzyme-linked immunosorbent assay (ELISA) and indirect fluorescent antibody assay (IFA). Although IFA is highly specific, it is subjective and is not suitable for screening of large numbers of samples. ELISA has better throughput but requires larger amounts of serum samples than IFA. Moreover, the MHV virion antigen currently used in ELISA is propagated in mouse cell lines, but purified viral antigens have some disadvantages, such as poor yields of purified viruses, less reproducibility of antigens, and contamination with cellular proteins, resulting in difficulties in quality control of antigens and frequent false-positive results in serological tests.
The recombinant antigen-based antibody detection tests are well known to provide high reproducibility, are easy to standardize, and do not require cultivation of infectious agents. Although the use of recombinant antigens in serological tests has been widely reported for a variety of infectious diseases (1, 4, 7, 13, 14, 17, 19, 21, 23) , no serological test using recombinant MHV antigens is currently available. MHV is composed of three major structural proteins: nucleocapsid protein (N), spike protein (S), and membrane protein (M). The N, S, and M proteins all contribute to inducing host immune responses. The N protein is the most abundant protein in the MHV virion, with an amino acid sequence that is highly conserved among MHV strains. The S protein is the major target of the neutralizing antibodies and induces the earliest antibody responses (2, 5, 11, 18) . Therefore, we chose the N and S proteins as candidates to generate recombinant antigens for the detection of antibody responses to MHV infections.
The microsphere assay technology developed by Luminex Corp. is suited to a high-throughput immunoassay for simultaneous detection of antibodies to multiple pathogens and antigens (4, 8, 9, 10, 12, 24, 25) . In a microsphere-based multiplex fluorescent immunoassay (MFI), microspheres function as the solid phase to which protein antigens from various pathogens are bound. One hundred sets of color-coded beads are used, and each bead set is distinguishable by its fluorescent emission when excited by a classification laser. Since each of the bead sets can be conjugated with a unique antigen, each bead represents a separate antibody reaction after incubation with the serum samples to be tested, followed by a fluorescently labeled detection reagent and fluorescent emission by a detection laser. Another distinct advantage of the MFI method is that a trace amount of serum sample is sufficient for multiplex detection.
Here we describe the usefulness of the MFI method using recombinant N and S antigens in serological diagnosis of MHV infections. The sensitivity and specificity of recombinant antigen-based MFI were evaluated in comparison with those of the cell culture virion-based ELISA and IFA using experimentally and naturally infected mouse sera. Furthermore, our MFI system was applied to serological testing of coronavirus infections in rats, including sialodacryoadenitis virus (SDAV) and rat coronavirus (RCV).
The MHV-S strain was obtained from ATCC and propagated in a mouse astrocytoma cell line (DBT) (6) . DBT cells were grown in Eagle's minimum essential medium (MEM) containing 10% fetal bovine serum at 37°C with 5% CO 2 in a humidified incubator.
Animals and serum samples. Antisera to the MHV-S, -A59, -JHM, and -Nu67 (20) strains were prepared from 7-week-old female ICR mice inoculated oronasally with 1 ϫ 10 6 to 1 ϫ 10 7 50% tissue culture infective doses (TCID 50 )/100 l of MHV culture stock. Antiserum to SDAV-681 was prepared from 7-week-old female Wistar rats oronasally inoculated with 1 ϫ 10 6 TCID 50 /100 l of SDAV-681 culture stock. At 4 weeks postinfection, serum was collected from the experimentally infected mice, and rats were euthanized by isoflurane inhalation. For serial serum collection, serum samples were obtained from the tail veins of mice on days 0, 7, 14, 21, and 28 after inoculation with MHV-S. The animal studies were approved by the institutional animal care and use committees of the University of Tsukuba and the Central Institute for Experimental Animals.
Uninfected control sera were collected from ICR, C3H, C57BL/6, and BALB/cA mice, and Wistar rats were purchased from CLEA Japan Inc. (Tokyo, Japan) at 6 to 30 weeks old. Their source colonies were specified to be free of MHV or SDAV/RCV, and the sera collected were confirmed to be negative for MHV or SDAV/RCV by ELISA and IFA as described below.
To evaluate the specificity and sensitivity of the microsphere-based multiplex fluorescent immunoassay (MFI) method for detecting antibodies in mice and rats naturally infected with MHV or SDAV/RCV, mouse and rat sera from a variety of sources submitted to the Central Institute for Experimental Animals for health screening were tested by MFI as well as ELISA and IFA for detection of anti-MHV or anti-SDAV/RCV antibodies.
Viral RNA preparation and RT-PCR amplification. DBT cells were infected with MHV-S and incubated for 2 days. The infected cells were collected, and RNA was extracted with RNAiso Plus reagent (Takara Bio, Otsu, Japan). For cDNA synthesis, aliquots of 100 ng of RNA were incubated at 65°C for 5 min with 0.5 M reverse oligonucleotide primer. After chilling on ice, 200 U of SuperScript III reverse transcriptase (Invitrogen, Carlsbad, CA) was added, followed by incubation at 50°C for 1 h in the presence of 0.5 mM (each) deoxynucleoside triphosphates (dNTPs), 5 mM dithiothreitol, and 1ϫ firststrand buffer in a reaction volume of 20 l. Aliquots of 2 l of cDNA reaction mixture were added to PCR mixtures containing 0.6 M (each) forward and reverse primers, 0.2 mM (each) dNTP, 1ϫ Pyrobest buffer II, and 2.5 U of Pyrobest DNA polymerase (Takara Bio) in a volume of 50 l. PCR was carried out for 35 cycles of 15 s at 94°C for denaturation, 30 s at 60°C for annealing, and 2 min at 72°C for extension using a PCR thermal cycler (TP600; Takara Bio). The sequences of oligonucleotide primers used for reverse transcription-PCR (RT-PCR) were as follows: S1-FW, 5Ј-CCGGATCCATGTTGTCCGTGTTTATT T-3Ј (BamHI site in italics); S1-RV, 5Ј-TAGTCGACTCACGTGCGCGCGCG GTGAGCA-3Ј (SalI site in italics); S2-FW, 5Ј-CCGGATCCTCTGTCAGTAC GGGTTATA-3Ј (BamHI site in italics); S2-RV, 5Ј-GGTCGACCTCAATCCTC ATGGGAGGAAAT-3Ј (SalI site in italics); Smid-FW, 5Ј-ATGGATCCGGAT TTAATGATGTGGC-3Ј (BamHI site in italics); Smid-RV, 5Ј-CCGTCGACTC ATAGAATTTCTTGTA-3Ј (SalI site in italics); N-FW, 5Ј-CTAGAATTCATG TCTTTTGTTCCTGGG-3Ј (EcoRI site in italics); and N-RV, 5Ј-GCGCTCGA GTTACACATTAGAGTCATC-3Ј (XhoI site in italics). PCR products were separated by 1% agarose gel electrophoresis. Bands were excised from the gels, and the products were purified using a QIAquick gel extraction kit (Qiagen, Valencia, CA).
Cloning and sequencing. Purified PCR products were cloned into the plasmid vector pGEM-T Easy (Promega, Madison, WI), and the sequences were determined using a BigDye PCR sequencing kit (Applied Biosystems, Carlsbad, CA) and the ABI Prism 3100-Avant genetic analyzer (Applied Biosystems). The full region of the N gene was subcloned into the EcoRI and XhoI sites of the expression vector pGEX-5X-1 (GE Healthcare, Little Chalfont, United Kingdom). The partial regions of the S gene, S1, S2, and Smid (Fig. 1A) , were subcloned into the BamHI and SalI sites of the expression vector pET28a(ϩ) (Novagen, Darmstadt, Germany). After a ligation reaction carried out using the Ligation High reagent (Toyobo, Osaka, Japan), Escherichia coli BL21 or BL21(DE3) competent cells were transformed with the recombinant plasmids.
Expression and purification of recombinant proteins. To produce the glutathione S-transferase (GST)-fused recombinant N protein, transformed E. coli BL21 was cultured in 1 liter of Luria-Bertani (LB) medium containing 100 g/ml ampicillin at room temperature. When the culture media had reached an optical density at 600 nm (OD 600 ) of 0.6 to 0.8, isopropyl -D-thiogalactopyranoside (IPTG) was added at a final concentration of 0.8 mM, followed by incubation for an additional 2 h at room temperature. Cultures were placed on ice for 10 min and centrifuged at 2,000 ϫ g for 10 min. Next, the supernatant was removed, and the pellet was suspended in 50 ml of Dulbecco's phosphate-buffered saline (PBS). Then, Triton X-100 at a final concentration of 1% and dithiothreitol at a final concentration of 1 mM were added, and the mixture was stored at Ϫ80°C until purification. The bacterial suspension was thawed and sonicated on ice 15 times with 10-s pulses. The soluble fraction was collected after centrifugation at 8,000 ϫ g for 20 min, and then 1 ml of 50% glutathione Sepharose 4B equilibrated with PBS was added. After incubation with gentle inversion at room temperature for 1 h, the matrix was transferred to a disposable column and washed with 20 ml of PBS. Protein was eluted 4 times by incubation with 1 ml of 50 mM Tris-HCl (pH 8.2) containing 20 mM glutathione at room temperature for 20 min. Usually, only fractions 1 and 2 were pooled for further use because they showed OD 280 values of 0.8 or higher. In addition, E. coli BL21 carrying the pGEX-5X-1 vector, which contains the full-length N gene of Sendai virus (SeV), was utilized to prepare the GST-fused SeV N protein as an irrelevant GST control antigen. To produce the His-tagged recombinant S1, S2, and Smid proteins, each transformed E. coli BL21(DE3) clone was cultured in 500 ml of LB medium containing 60 g/ml kanamycin at room temperature. When the culture medium had reached an OD 600 of 0.4 to 0.5, IPTG was added to a final concentration of 0.4 mM, followed by incubation for an additional 4 h at room temperature. Cultures were placed on ice for 10 min and centrifuged at 2,000 ϫ g for 10 min. Next, the supernatant was removed, and the pellet was suspended in 25 ml of PBS containing 1% Triton X-100, 1 mg/ml lysozyme, and 1 mM dithiothreitol, and the mixture was stored at Ϫ80°C until purification. The bacterial suspension was thawed and sonicated on ice with 30-s pulses 10 times. The insoluble fraction was collected by centrifugation at 8,000 ϫ g for 20 min, and the pellet was suspended in 2.5 ml of PBS containing 1 M urea and 1% Nonidet P-40. After sonication with 30-s pulses 10 times on ice, the pellet was collected by centrifugation at 13,000 ϫ g for 20 min, resuspended in 2.5 ml of PBS containing 1 M urea, and sonicated with 30-s pulses 10 times on ice. The pellet was then collected again by centrifugation at 13,000 ϫ g for 20 min, dissolved in 500 l of PBS containing 8 M urea, and sonicated with 30-s pulses 10 times on ice. After centrifugation at 13,000 ϫ g for 10 min, the supernatant was collected as partially purified recombinant proteins. The concentration of the recombinant proteins was determined by the Bradford protein assay (Bio-Rad Laboratories, Hercules, CA). To assess the quality of the recombinant protein, protein samples were subjected to 8% SDS-PAGE and stained with BioSafe Coomassie (Bio-Rad). Immunological reactivity of the recombinant protein was confirmed by immunoblotting analysis with mouse antiserum to the MHV-S strain (1:1,000 dilution), horseradish peroxidase (HRPO)-conjugated anti-mouse IgG (1:10,000 dilution; GE Healthcare), and an ECL Advance Western blotting detection kit (GE Healthcare).
Coupling of viral proteins to fluorescent microspheres. Carboxylated fluorescent microspheres (Luminex, Austin, TX) were suspended in 80 l of 0.1 M NaH 2 PO 4 (pH 6.2) and activated by adding 10 l (each) of 50 mg/ml N-hydroxysuccinimide (Pierce, Rockford, IL) and 50 mg/ml 1-ethyl-3-(3-dimethylaminopropyl) carbodiimide (Pierce). The microsphere mixture was incubated for 20 min at room temperature in the dark, centrifuged at 8,000 ϫ g for 2 min, and washed twice with 250 l of PBS. Then, recombinant N antigen and inactivated MHV virion antigen were coupled to microspheres by incubation for 2 h at room temperature in 500 l of PBS using a rotator in the dark. Recombinant S1, S2, and Smid antigens were coupled to microspheres by incubation for 2 h at room temperature in 500 l of PBS containing 8 M urea using a rotator in the dark. Antigen-coupled microspheres were washed three times with 500 l of 0.05% Tween 20 in PBS and resuspended in 250 l of 1% bovine serum albumin (BSA), 0.05% sodium azide, and 1ϫ Complete Mini protease inhibitor (Roche, Basel, Switzerland) in PBS. After blocking, the microspheres were stored at 4°C in the dark.
MFI. MFI was performed to detect antibodies to coronaviruses in mouse and rat sera. Approximately 2,000 beads (each) of antigen-coupled microsphere sets were added to each well of a multiscreen HTS 96-well filter plate (Millipore, Bedford, MA). Beads were washed with 200 l per well of 0.05% Tween 20-PBS VOL. 18, 2011 RECOMBINANT MHV ANTIGEN-BASED MFI 759
on March 19, 2020 by guest http://cvi.asm.org/ by removing the fluid using a plate vacuum manifold (Millipore). Serum samples were diluted with 1% BSA-PBS, and 100 l was added to each well. The beads were incubated on a plate shaker in the dark for 1 h at room temperature. After incubation, the beads were washed twice by adding 200 l of 0.05% Tween 20-PBS, shaking for 1 min on a plate shaker, and removing the fluid with a plate vacuum manifold. Next, the beads were resuspended in 100 l of biotinylated anti-mouse IgG(HϩL) (Vector Laboratories, Burlingame, CA) diluted 1:2,000 or biotinylated anti-rat IgG(HϩL) (Vector Laboratories) diluted 1:1,000 in 1% BSA-PBS and incubated on a plate shaker in the dark for 1 h at room temperature. The beads were washed twice with 0.05% Tween 20-PBS as described above and then resuspended in 100 l of 1 g/ml R-phycoerythrin (R-PE)conjugated streptavidin (streptavidin-R-PE; Molecular Probes, Eugene, OR) in 1% BSA-PBS. After incubation on a plate shaker in the dark for 30 min at room temperature, the beads were washed three times with 0.05% Tween 20-PBS, resuspended in 100 l of 0.05% Tween 20-PBS, and analyzed in a Luminex-200 instrument. MFI data analysis. A minimum of 100 gated events were acquired for each bead set by using the Luminex-200 instrument. Results of antibody detection are reported as the median fluorescent intensity of R-PE measured from 100 or more counted beads. For each antigen, the optimal protein concentration for coupling and the cutoff value for designating positive or negative were determined. To set the cutoff value, an average median fluorescent intensity for more than 30 normal mouse or rat sera was calculated for each antigen, and three times the standard deviation (SD) value was added to the average. To determine the optimal protein concentration, the signal/noise (S/N) ratio was calculated as the median fluorescent intensity of anti-MHV-S-or anti-SDAV-681-positivecontrol serum diluted 1:100, divided by the cutoff value. The protein concentra-tion at which the highest S/N ratio was obtained was used as the optimal concentration for coupling. ELISA. A commercial ELISA kit (Monilisa MHV; Wakamoto Pharmaceutical, Tokyo, Japan) was used to detect anti-MHV mouse antibody and anti-SDAV/RCV rat antibody in 1:40-diluted test sera. Serum samples with OD 492 values of Ն0.4 were classified as positive by measurement with a microplate reader (model 680; Bio-Rad).
IFA assay. MHV-Nu67-infected DBT cells were mixed with equal numbers of uninfected DBT cells, spotted onto 12-well Teflon printed slides, fixed in acetone at 4°C for 15 min, and stored at Ϫ20°C until use. Aliquots of 10 l of test sera at a dilution of 1:10 in PBS were added to each well and incubated at 37°C for 15 min. The slides were then washed in PBS and incubated with 10 l of fluorescein-conjugated anti-mouse IgG (MP Biomedicals, Irvine, CA) or fluorescein-conjugated anti-rat IgG (MP Biomedicals) diluted 1:100 in PBS containing 1% Evan's blue dye at 37°C for 15 min. After the slides were washed, specific fluorescence was analyzed using a UV epifluorescence microscope.
Nucleotide sequence accession number. The complete nucleotide sequence of the S gene cloned from the MHV-S strain has been submitted to DDBJ/EMBL/ GenBank under accession no. AB593383.
Cloning of MHV-S strain S and N genes. The N gene sequence obtained was identical to that of the MHV-S strain registered in GenBank with the accession number M35255. . Partial-length S proteins were expressed as His-tagged proteins, S1-His (87 kDa), S2-His (68 kDa), and Smid-His (70 kDa). (C) Immunoblot detection of the recombinant proteins, N-GST, S1-His, S2-His, and Smid-His, with anti-MHV-S-strain mouse serum. The N-GST protein was affinity purified using immobilized glutathione, and the S1-His, S2-His, and Smid-His proteins were partially purified from insoluble extracts by serial treatment with urea solutions. The arrows indicate the major recombinant protein bands. MWM, molecular size marker.
The S gene sequence obtained here did not show perfect identity to that of the MHV-S strain registered in GenBank with the accession number GU593319, with 4 nucleotide substitutions all resulting in amino acid alterations. The S gene of the MHV-S strain showed a high degree of similarity with the S genes of the MHV-Y, -TY, -DVIM, -2 and -1 strains, with amino acid identities of 95% to 91%. The degree of S gene similarity was also high between MHV-S and the rat coronavirus strains SDAV-681 and RCV-Parker, with amino acid identities of 92% and 91%, respectively. On the other hand, the degree of S gene similarity between MHV-S and the MHV-3, -RI, -JHM, or -A59 strain was relatively low (amino acid identities of 80% to 82%). The S2 region, i.e., the carboxyterminal half of the S protein, is highly conserved among MHV strains and rat coronaviruses, with identities of 88% to 99%, which were comparable to the degrees of N protein identity (91% to 96%). However, the degrees of identity of the S1 region, i.e., the amino-terminal half of the S protein, among MHV strains and rat coronaviruses was 73% to 94%, which was lower than that of S2.
After sequencing, the full-length N gene was subcloned into the pGEX-5X-1 vector, and partial regions of the S gene, S1, S2, and Smid (Fig. 1A) , were subcloned into the pET28a(ϩ) vector. In E. coli clones transformed with these recombinant plasmids, 4 recombinant proteins, the GST-fused N protein and the His-tagged S1, S2, and Smid proteins, were expressed successfully. At first, we attempted to prepare the recombinant S protein as a soluble GST fusion protein in the same way as the N protein. Since the S protein is too large to be produced efficiently in E. coli, we cloned each DNA fragment encoding the N-terminal S1 subunit, which forms the globular head of the peplomer, and the C-terminal S2 subunit, which forms the stalk region. In addition, the middle part of the S protein near the proteolytic site, which we designated Smid, was prepared. Since all the truncated recombinant S proteins in the GST fusion form were expressed in only small amounts, we finally produced the recombinant S1, S2, and Smid proteins as insoluble His-tagged proteins. Unexpectedly, immobilized metal affinity chromatography did not enable purification of a large amount of these His-tagged proteins efficiently. Therefore, we partially purified the insoluble His-tagged proteins by washing them in 1 M urea solution. Finally, the recombinant S proteins were solubilized in 8 M urea-PBS and used as antigens for further analysis.
SDS-PAGE and immunoblotting analysis of recombinant proteins. Recombinant proteins produced by E. coli were analyzed by SDS-PAGE. As shown in Fig. 1B , the GST-fused N protein and the His-tagged S1, S2, and Smid proteins were detected as major recombinant protein bands, with estimated molecular masses of approximately 76 kDa, 87 kDa, 68 kDa, and 70 kDa, respectively. On immunoblotting analysis, all the major recombinant MHV proteins showed strong reactivity with anti-MHV-S mouse serum (Fig. 1C) . Several bands that do not correspond to the expected molecular masses of the recombinant MHV proteins were detected, and some of those were immunoreactive (Fig. 1B and C) . It is likely that the smaller proteins present in the affinity-purified GST-N protein preparation are degraded recombinant N proteins, since those are immunoreactive with anti-MHV serum. Multiple protein bands present in the His-tagged S protein preparations may be degraded proteins originated from the recombinant S proteins or E. coli proteins contaminating the insoluble inclusion bodies.
Development of recombinant MHV antigen-based MFI. The reactivities of microspheres coupled with 5 to 40 g/ml of each recombinant antigen were analyzed using serially diluted anti-MHV-S mouse serum. As shown in Fig. 2 , antigen dose-dependent reactions were observed for all of the antigens tested. Although the median fluorescent intensity value for MHV virion antigen decreased sharply at low antigen concentrations, all of the recombinant antigens exhibited consistently high median fluorescent intensities even at the lowest antigen concentration used. Specificity of the GST-fused MHV N protein for detection of anti-MHV antibody was confirmed by the lack of cross-reactivity of anti-MHV S serum with the GST-fused SeV N protein, used as an irrelevant GST control antigen ( Fig. 2 ; see also Table 2 ). Furthermore, linear median fluorescent intensity results across a broad range of antibody concentrations were obtained for all of the antigens. This linear reaction property of the MFI and low background binding of microspheres allowed the MFI to be performed at a serum dilution of 1:100.
The optimal protein concentration of each antigen for mouse serum testing was determined based on reactivity in MFI with 1:100-diluted anti-MHV mouse serum and negativecontrol mouse sera. As shown in Table 1 , all the recombinant antigens provided the highest S/N ratio when used at 10 g/ml; therefore, the optimal protein concentration was set at 10 g/ml. The cutoff value to distinguish positive samples was set at a median fluorescent intensity of 750 for the N antigen, 850 for the S1 antigen, 800 for the S2 antigen, and 1,000 for the Smid antigen according to the background median fluorescent intensity values, calculated as the mean plus 3 standard deviations after rounding up to the nearest 50 median fluorescent intensity units. For MHV virion antigen, the optimal protein concentration and cutoff value were set at 40 g/ml and a median fluorescent intensity of 900, respectively. In the same manner, the antigen concentration and cutoff value were optimized for rat serum testing ( Table 1 ). The optimal protein concentration of the S1, S2, and Smid antigens was 10 g/ml, which was the same in mice and rats, while the N antigen was optimal at 5 g/ml and the virion antigen was optimal at 20 g/ml. The cutoff value was set at a median fluorescent intensity of 1,000 for the N antigen, 750 for the S1 antigen, 450 for the S2 antigen, 500 for the Smid antigen, and 600 for virion antigen to measure antibodies in rat sera.
To evaluate cross-reactivities of the recombinant antigens prepared from the MHV-S strain, each recombinant antigen and MHV-S virion antigen was analyzed with mouse antisera raised to several MHV strains and rat antiserum to SDAV-681 by MFI (Fig. 3) . Antisera to MHV-S, MHV-A59, and MHV-JHM showed a similar reaction pattern. These sera reacted strongly with the recombinant N and virion antigens, moderately with the recombinant S2 and Smid antigens, and weakly with the recombinant S1 antigen. Antiserum against MHV-Nu67 showed high reactivity with the S2 antigen, moderate reactivity with the Smid antigen, and weak reactivity with the virion, N, and S1 antigens. High cross-reactivity of anti-SDAV rat serum was observed with the MHV virion, N, S2, and Smid antigens. These results indicated that the recombinant antigens cross-react with antibodies generated to a variety of MHV strains and SDAV, although the strength of reactivity to each antigen varies among viral strains.
A time course of seroconversion to MHV detected by MFI, ELISA, and IFA was compared using serially collected serum samples from 10 mice experimentally infected with MHV-S. As shown in Table 2 , only 2 or 3 of 10 mice seroconverted to MHV on day 7 after inoculation, and all mice seroconverted on day 14, according to the N MFI, S2 MFI, virion MFI, and IFA. In contrast, all mice tested positive for anti-MHV antibodies on day 7 in the ELISA. We also found nonspecific reactivity with day zero serum samples in the ELISA analysis but not in either the MFI or IFA analysis. These results suggest that ELISA is superior in detecting antibodies to MHV at the early stage of infection and that MFI has high sensitivity and specificity, equivalent to those of IFA.
Sensitivity and specificity of MFI. To evaluate the sensitivity of the recombinant MHV antigen-based MFI, 236 mouse sera a By measuring the median fluorescent intensity values of 32 negative mouse sera at a dilution of 1:100, background intensity was defined as the mean ϩ 3 SD after rounding up to the nearest 50 median fluorescent intensity units. Signal/noise (S/N) ratio was calculated as the median fluorescent intensity of 1:100-diluted anti-MHV-S-strain mouse serum divided by the background intensity. The optimal protein concentration of each antigen was determined at the concentration that provided the highest S/N ratio. The background median fluorescent intensity at the optimal concentration was used as a cutoff value to differentiate between the positive and negative samples (indicated by underlined bold letters).
b The optimal protein concentration of each antigen and cutoff median fluorescent intensity value for rat sera were determined in the same manner using 30 negative rat sera. naturally infected with MHV and 50 rat sera naturally infected with SDAV or RCV, confirmed by double-positive results in a commercial MHV-ELISA and IFA, were tested with the MFI. In MHV-infected mouse sera, as shown in Table 3 , the highest sensitivity was obtained with S2 (96%), followed by the Smid (89%), N (88%), virion (76%), and S1 antigens (65%). In SDAV-infected rat sera, as shown in Table 4 , seropositive rates for S2, Smid, and virion were markedly high, with the same sensitivity (98%), followed by the N antigen with a similar high sensitivity (94%), but S1 antigen provided the lowest (60%) sensitivity.
With regard to the specificity of the recombinant MHV antigen-based MFI, non-MHV-infected mouse sera and non-SDAV/RCV-infected rat sera, confirmed by negative results in IFA, were tested and compared with a commercial MHV ELISA (Tables 3 and 4 ). In MFI for detecting mouse and rat antibodies, all the antigens revealed high specificity (Ն90% for mice and 100% for rats). In contrast, the specificity of a commercial MHV-ELISA was 73% for mice and 90% for rats. Figure 4 shows a comparison of S2 antigen-based MFI and N-antigen-based MFI results for 236 sera from mice naturally infected with MHV. Of 236 mouse sera tested, 9 serum samples were negative in S2-antigen-based MFI and 28 serum samples were negative in N-antigen-based MFI. Only three serum samples (1.3%) showed double-negative results for S2 and N by MFI. Of 126 sera from MHV-uninfected mice, 10 serum samples (7.9%) showed false-positive results for either the S2 or N antigen. Taken together, these results indicated that the MFI using the recombinant S2 and N antigens has FIG. 3. Reactivities of recombinant MHV antigens with mouse antisera to MHV strains and rat antiserum to SDAV. Recombinant MHV antigens (N, S1, S2, and Smid) and MHV virion antigen were coupled onto microspheres at the optimal protein concentration for each antigen, and their reactivities with serially diluted mouse antisera to MHV strains and rat antiserum to SDAV were analyzed by microsphere fluorescence immunoassay. }, N antigen; Ⅺ, S1 antigen; F, S2 antigen; ‚, Smid antigen; ,ء virion antigen. Ն98% sensitivity and Ն92% specificity for detecting MHV and SDAV/RCV antibodies in mouse and rat sera.
In this study, the recombinant S and N proteins of MHV produced in E. coli were tested as a substitute for purified virion antigens for the detection of antibodies to rodent coronaviruses. Major disadvantages of virion antigen are the variation in purities of virion proteins, which affects the specificity and sensitivity, laborious work to prepare large amounts of antigen, the possibility of remaining infectivity, and the loss of reactivity during the inactivation process. First, we prepared the recombinant N protein as a soluble GST fusion protein. However, we found that the sensitivity of the N protein-based ELISA was lower than that of the traditional ELISA using virion antigen in a preliminary study. Therefore, we attempted to prepare recombinant S proteins in GST-fused and Histagged forms. Because the S protein is too large to be produced efficiently in E. coli, we prepared truncated molecules of the S protein, including the N-terminal S1 subunit, the C-terminal S2 subunit, and the middle part, designated Smid. In previous studies of MHV (3, 16) , bovine coronavirus (22) , and severe acute respiratory syndrome coronavirus (15, 26) , this middle region was identified as an immunodominant linear neutralization domain by analysis using a prokaryotic protein expression system and monoclonal antibodies or patients' sera. As a result, large amounts of the His-tagged S1, S2, and Smid proteins were produced as insoluble proteins and were solubilized in a high-molar urea solution. All of the recombinant S proteins that were solubilized under denaturing conditions using 8 M urea showed immunoreactivities with anti-MHV antibodies on immunoblotting and in MFI. We found that the MFI reactivity of the microspheres coupled with S proteins in 8 M urea-PBS was stronger than that of the microspheres coupled in PBS. These observations indicated that the recombinant S proteins solubilized with 8 M urea were capable of being cou-pled efficiently to microspheres in 8 M urea-PBS. These findings may reflect the appearance of insoluble aggregates of the recombinant S proteins in PBS, which leads to a decrease in coupling efficiency and MFI reactivity.
All the recombinant proteins were expressed from the genes of the MHV-S strain. Cross-reactivity of these recombinant proteins in detection of antibodies to other MHV strains and SDAV rat coronavirus was confirmed by MFI. According to the calibration curves constructed with the experimentally infected sera to MHV strains and SDAV, cross-reactivity levels of the recombinant proteins were compared. The median fluorescent intensity values corresponding to the 4 recombinant proteins were similar among the antisera. However, antiserum to MHV-JHM reacted very weakly with the S1 antigen. This weak reactivity was unlikely to be a consequence of the relatively low amino acid sequence identity (76%) of S1 between MHV-JHM and MHV-S, because the level of antibody to S1 in anti-MHV-A59 serum was equivalent to or higher than that in anti-MHV-S serum despite the even lower S1 identity (73%) between MHV-A59 and MHV-S. Furthermore, antiserum to MHV-Nu67 was remarkable for its lower level of antibody to the N antigen than to the S2 and Smid antigens. Such low reactivity to the N antigen may be reflective of the slow growth of MHV-Nu67, a low-pathogenicity strain isolated from nude mice (20) . This may be correlated with the observation that the S protein is the earliest recognized antigen by the host immune system, whereas the N protein elicits antibody responses during the late stage of infection (2, 15) . Nevertheless, the possibility that the differences in reaction pattern among the antisera to the virus strains described above were simply due to individual variation among the experimentally infected animals cannot be excluded.
The MFI calibration curve of each antigen constructed with anti-MHV-S serum suggested that MFI provides a broad dynamic range of antibody concentration, which allows accurate analysis of antibody levels at a single point of serum dilution. We assessed 32 mouse sera and 30 rat sera from MHV or SDAV/RCV-free commercial breeder animals at a 1:100 dilution and established an optimal antigen concentration and a cutoff median fluorescent intensity value corresponding to each antigen. The protein concentration providing the highest S/N ratio was determined as the optimal antigen concentration, and the mean median fluorescent intensity plus 3 SD value was set as a cutoff median fluorescent intensity value. According to these criteria, serial serum samples from experimentally infected mice and a large number of serum samples from naturally infected laboratory mice were analyzed to select the favorable antigens in MFI for detecting antibodies to MHV and to evaluate diagnostic predictability. An experimental infection study showed that seroconversion in the MFI took place from 7 to 14 days post-MHV infection in the majority of infected mice. In contrast, all of the infected mice seroconverted in ELISA by day 7. For early detection of MHV antibodies, ELISA is more sensitive than MFI, whereas the sensitivities of N-based MFI and S2-based MFI are equivalent to that of IFA. Because IFA is the primary method used as confirmatory testing for ELISA and MFI-based screening as-says, special attention should be paid to false-negative results in MHV antibody detection within 2 weeks after infection. With regard to the reactivity of sera from naturally infected mice, our results indicated that the S2 antigen has the highest sensitivity, but 4% (9 of 236) of samples still show false-negative results. The recombinant N antigen resembles the MHV virion antigen in immunoreactivity with naturally and experimentally infected sera. While the sensitivity of the N MFI was 88%, 6 of 9 false-negative samples in the S2 MFI showed positive results in the N MFI. These results indicated that multiplex serological screening, consisting of S2 MFI and N MFI, has 98% sensitivity for detection of MHV infection in mice. Naturally infected animal sera used in this study were retrospective serum samples screened for positivity for antibodies to MHV by ELISA and IFA. Therefore, it is likely that the sensitivity of MFI is equivalent to that of the traditional ELISA and IFA. Thirty-four sera that were negative for anti-N or anti-S2 antibodies may represent samples collected from mice at the early stage of infection. It is not likely that the failure to detect antibodies based on the MFI using the N or S2 on March 19, 2020 by guest http://cvi.asm.org/ antigen resulted from a higher serum dilution in MFI (1:100 dilution) than that in ELISA (1:40 dilution), because no significant correlation was seen between ELISA OD values and median fluorescent intensity values in these sera. Many of the singly positive sera showed definite reactivity with either N or S2. Thus, production of antibody to each antigen varies among individuals or strains of animals as well as virus strains, and consequently, multiplex analysis using polyvalent antigens is recommended in recombinant antigen-based serologic testing. Based on the assessment of 126 mouse sera and 59 rat sera from animals uninfected with MHV or SDAV/RCV, which were confirmed by IFA, we found that the MFI had a significantly lower false-positive rate than the ELISA. The lower false-positive rate in MFI may be due to the lower nonspecific binding of serum antibodies to the microspheres than to ELISA plates (12) . In the case of the recombinant antigenbased MFI, the purified recombinant proteins are covalently coupled to microspheres, and antigen purity eliminates nonspecific reactions that often cause high background problems in ELISA (25) . Furthermore, the linear reaction and high sensitivity allow MFI to be used for analysis of serum samples at a dilution of 1:100. Since the MHV ELISA was performed using 1:40-diluted sera, the higher serum dilution in MFI definitely affected the low background.
In summary, the MFI method developed with recombinant MHV S2 and N antigens was as sensitive as and more specific than the traditional MHV ELISA for screening of antibodies to coronaviruses in mouse and rat sera. MFI-format assays have been used for the simultaneous detection of antibodies to a variety of pathogens in laboratory rodents (4, 8, 9) . Although MHV virion antigen was successfully used to develop the MFI system for serodetection of 10 mouse pathogens (9) , this is the first report of using a recombinant antigen-based MFI to detect MHV infection. There are many advantages to MFI over ELISA when screening large rodent colonies. MFI allows screening of animals for serum antibodies to multiple pathogens or antigens simultaneously in one reaction well for each animal. This markedly reduces the time and labor required to perform a separate ELISA for each antigen. The MFI format also requires much less serum than ELISA, since multiple agents can be screened using only 1 l of undiluted serum. In this regard, MFI is suitable for serological screening of small laboratory animals, such as mice, often through survival bleeding. The amounts of antigens required for coupling microspheres for 200 analytes are equal to those used for coating a single well of an ELISA plate, thereby greatly saving cost, time, and labor for preparing antigens. In our experience, antigencoupled microspheres can be stored in the refrigerator for at least 6 months without a significant reduction in immunoreactivity. The multiplexing feature of the MFI method enables high throughput and automated screening for serological detection of many infectious agents in laboratory animals.
|
Annnotations
- Denotations: 1
- Blocks: 0
- Relations: 0