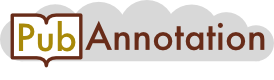
CORD-19:04c300fc145b27f210fe05c52a93de14920c17bc / 0-143 2 Projects
THE APPLICATION OF MONOCLONAL ANTIBODIES I N THE STUDY OF VIRUSES
Abstract
Since their initial description (Kohler and Milstein, 1975) monoclonal antibodies have made a profound impression on all areas of biological research and biotechnology. The basic property of a monoclonal antibody, that of combining specifically with one epitope (or family of related epitopes), can be used to supply a wealth of data. A single monoclonal antibody can provide information on protein "relatedness," structure, function, synthesis, processing, cellular or tissue distribution and on the association between molecules. Any attempt to separate the ways in which these data are gathered must be to some extent artificial since one study frequently yields results relevant to other areas. However, it is appropriate to differentiate between the broad areas of virological investigation. The contribution of monoclonal antibodies within each area can then be assessed. Thus, diagnostic virology, taxonomy, and epidemiology (Section 11) can be considered separately from the biochemical and molecular biological investigations described in Section 111; Section IV deals with the interaction of virus and host at the whole organism level. Consequently, the selection of virus variants is dealt with in Section I11 where information concerning protein structure or function has been obtained, and also in Section IV where pathogenesis was investigated. It is not intended in this review to provide an exhaustive list of all publications in which monoclonal antibodies have been used, but rather to illustrate how monoclonal antibodies can be applied in virology. Other reviews of different aspects have recently been published (Antczak, 1982; Blann, 1981; Kennett, 1981; Nowinski et al., 1983; Sinkovics and Dreesman, 1983; Yewdell and Gerhard, 1981) .
It is important to emphasize that monoclonal antibody techniques are rarely applied in isolation, but are normally backed up with investigations using the more classical biochemical approaches. For instance the recognition of separate active sites for hemagglutination and neuraminidase activity on the Sendai virus HN protein (Portner, 1981) involved experiments using temperature-sensitive mutations and chemical inhibitors as well as competitive antibody binding assays and selection of virus variants, and the proof of relationship between turkey (H1N1) influenza, and swine viruses involved RNA/RNA hybridization and replication studies as well as the observation of monoclonal antibody cross reactions (Hinshaw et al., 1983) . Furthermore, there are limitations to the information that antibody studies can provide; structural studies for instance ultimately depend on the orientation of an antibody binding site on the various structural levels of the protein, amino acid sequence, three-dimensional structure, and even intermolecular associations (Section 111).
The principle of monoclonal antibody production, that of fusing a short lived antibody-producing cell derived from an immunized animal with a permanent myeloma cell line, is well known. This has been recently reviewed (Gerhard et al., 1980) . It is, however, necessary to consider the production of the immunogen, since it may be necessary to enrich for, or preferentially expose, a particular antigen. Thus, in the production of measles virus-specific antibodies (Bohn et al., 1982) , heating of the antigen in the presence of 1% sodium dodecyl sulfate (SDS) led to the derivation of antibodies specific for the matrix (M) protein. Similar treatment in the presence of reducing agents favoured the production of antibodies directed to the phosphoprotein (P) and nucleocapsid protein (N). If the protein against which monoclonal antibodies are desired is sufficiently immunogenic, it is not necessary to use pure preparations. Whole cell antigen was used in the production of antibodies directed against respiratory syncitial virus (RSV) (CGte et al., 1981) and against polyoma virus T antigen (Dilworth and Griffin, 1982) . Also, if cross reacting, e.g., group specific antibodies are required, it is possible to perform the primary inoculation with one antigen, and the boosting inoculation with the cross reacting antigen (Gerhard et al., 1978) .
It is not appropriate to consider here the immunological techniques used in detail, but the interested reader is referred to a review of techniques used in conjunction with polyclonal sera (van Regenmortel, 1981) . However, it is relevant to consider the limitations of these processes when applied to monoclonal antibodies. First, the majority of monoclonal antibodies dc not cause immunoprecipitation of the antigen with which they combine. This may be related to the fact that only one epitope is recognized per molecule. Consequently, extensive cross linking and stabilization of immune complexes is not possible. Therefore, immunoprecipitation by monoclonal antibodies requires the addition of a cross-linking agent such as staphylococcus protein A or antiimmunoglobulin. The antigen is only retained by one binding site and only the most strongly combining monoclonal antibodies will succeed. This problem may be diminished in the case of a multimeric protein. Second, most immune precipitation procedures involve the use of detergent in the precipitation buffer. This is necessary to prevent nonspecific contamination of the precipitate. However, this detergent will have some destabilizing effect on protein structure. Monoclonal antibodies are often sensitive to minor conformational change (Section II1,B) and consequently may be rendered nonreactive in this test. It may be necessary to experiment with detergent type and concentration in order to achieve satisfactory precipitation. The specificity of monoclonal antibodies, compared with hyperimmune serum, is illustrated in Fig. 1 . The adverse effect of alterations in protein conformation may be aggravated in western blot procedures where antigens are often totally denatured for separation on sodium dodecyl sulfate (SDS)polyacrylamide gels before testing with antibody (Towbin et al., 1979) . However, the method does have applications (Braun et al., 1983; Roseto et al., 1983) . Immune precipitation is not suitable for quantitation of antigens. This type of information is extracted from radioimmunoassay (RIA) or enzyme-linked immunosorbent assay (ELISA). These processes are exten-sively applied during the initial screening for successful production of monoclonal antibodies, in diagnostic virology (Section I1,A) or in a modified form in competitive binding assays (Section 111). These procedures score only the combination of antibody and antigen and give no information on the nature of that antigen. Detergent conditions are frequently milder than those used in immunoprecipitation, but the attachment of antigen to the solid support necessary in many cases for these tests can itself result in conformational alteration (Bruck et al., 1982a) .
Information on the histological and intracellular location of antigen is obtained from techniques such as immune fluorescence (IF) and immune electron microscopy (IEM) using electron-dense or enzymatic markers (reviewed by van Regenmortel, 1981) . These techniques have been widely used in diagnostic virology (Section II,A) as well as in studies of viral protein synthesis and virus maturation (Section 111,B). In addition to the traditional techniques of serology, neutralization (NT), hemagglutination inhibition (HI), complement fixation (CF), and hemolysin inhibition (HLI) have been extensively employed. In the following it is shown how application of these techniques in experiments utilizing monoclonal antibodies can be made in the study of all areas of virological research.
The reaction of a single antibody with an epitope is characterized by the equilibrium constant of the combination; this is reflected in the binding assays described above (Section I). Although any one antibody is elicited by the exposure of an epitope to the immune system, it will react with many other epitopes to a greater or lesser extent, depending on the degree of fit between the would-be target site on the antigen and the combining site on the antibody itself. In practical terms the epitope must possess some form of similarity to the parent epitope before any combination at all is detect- Vero cells were infected with measles virus Edmonston and labeled with [35S]methionine. Lysates were prepared and used in immunoprecipitation experiments. Proteins immunoprecipitated by track 1, rabbit hyperimmune antimeasles serum; track 2, rabbit preimmune control serum; tracks 3-5, monoclonal antibodies specific for the H protein; tracks 6-8, monoclonal antibodies specific for the Nprotein; tracks 9-16, monoclonal antibodies specific for the matrix protein; track 17, control monoclonal antibody, raised against coronavirus antigen; track 18, total infected cell lysate without immunoprecipitation. Note that the antibodies used in tracks 6,7, and 8 are strongly mutually competitive in radioimmunoassay competitive binding experiments, but only one is also active in the immunoprecipitation reaction. Reproduced with permission from Carter et al. Nature (London) 1983. able in the binding assays. This specificity of reaction provides the basis for the detection of subtle differences between the gene products of related viruses and thus the differentiation of one virus from another.
An alteration in the efficiency with which any one antibody combines with its target constitutes only a small part of the overall reaction of polyclonal antiserum with antigen and is consequently not detectable. The use of antibodies with uniform specificity thus permits very fine analysis of epitope/antibody interactions and therefore of structural alteration in the target molecule. Indeed this approach greatly exceeds conventional serology in specificity, and has led to the recognition of differences between viruses previously thought identical. Monoclonal antibodies are capable of detecting differences of one amino acid, formerly only demonstrated by recourse to peptide fingerprinting or oligonucleotide mapping of the viral genome. This approach has therefore revolutionized the techniques of virus identification, providing in many cases a simplification in the test procedure and yielding a more rapid result. Used in this way, monoclonal antibodies find application in two main areas, first, in the field of rapid diagnosis of virus disease in man, animals, and plants, and second, in the extension of virus taxonomy. These investigations could lead to more effective vaccination programs or disease treatment, and to a greater understanding of the relationships between viruses, their evolution, and epidemiology.
Rapid and accurate diagnosis of viral infection is of obvious importance to the clinician and to public health. Wands et al. (1981) have summarized the criteria for selection of a monoclonal antibody for use as a diagnostic reagent as follows:
1. High affinity for antigen, both to permit efficient combination with low concentrations of antigen and to more efficiently displace host antibodies which may already coat primary biopsy samples.
2. It may be directed toward an area of the molecule not normally recognized efficiently by the host's own antibodies, and not therefore masked.
3. The antibody should be directed against an often repeated antigenic determinant that is readily accessible, e.g., a virus coat protein.
4. Multivalence: this may increase sensitivity, and IgM was found more effective than IgG.
If the antibody is not carefully chosen, it may give less satisfactory results than usual serological procedures (Phillips et al., 19821 , and cross reaction with host cell polypeptides should be carefully excluded (Fujinami et al., 1983) . The application of monoclonal antibodies in diagnosis can be illustrated by reference to the following viruses; herpes, hepatitis B, rabies, flaviviruses, picornaviruses, and influenza; this list is not exhaustive.
Cytomegalovirus is a human herpesvirus (human beta herpesvirus 5) which has gained prominence in recent years following latent virus reactivation and disease in immunosuppressed, organ transplant recipients or patients with some other immune deficiency syndrome (Peterson et al., 1980; Thomas et al., 1975a,b) . Recently monoclonal antibodies have been developed which are directed against virus structural polypeptides Pereira et al., 1982~) . Some of these have proven able to detect CMV infection in biopsy specimens by the IF test . Antibodies specific for herpes simplex viruses types 1 and 2 (Balachandran et Pereira et al., 1982b; Showalter et al., 1981) have also been used to type these viruses directly in cells from herpetic lesions (Balachandran et al., 1982a; Peterson et al., 1983) . These tests yielded identical results to the more time consuming process of virus isolation and typing by restriction endonuclease mapping, and results were superior to those obtained by standard serology. A similar procedure has also proved successful in the identification of varicella zoster (human herpesvirus 3) in cells obtained from vesicular skin lesions (Forghani et al., 1982) ' and monoclonal antibodies are now available which could be useful for typing Epstein -Barr virus (human gamma herpesvirus 4) (Mueller-Lantzsch et al., 1981) . Herpes viruses can also be distinguished by a rapid enzyme immune filtration test (Richman et al., 1982) . Marek's disease (MD) virus is a herpes virus of chickens normally diagnosed by reference antisera directed against the Marek's associated tumor surface antigen (MATSA). Monoclonal antibodies have been obtained (Lee et al., 1983b) specific for MATSA, andused with success in the identification of Marek's disease in chickens (Lee et al., 1983a) . Use of this test should help prevent confusion with lymphoid leukosis, since both diseases produce similar symptoms and are of economic importance.
Monoclonal antibodies produced against hepatitis B virus (HBV) (Wands et al., 1981) have been used to obtain a similar improvement in sensitivity over conventional diagnosis. The application of monoclonal antibodies in RIA was found to detect HBV surface antigen even in patients who were negative by conventional testing . This was subsequently confirmed by the demonstration that the new test detected antigenantibody complexes invisible by the former procedure. These complexes were shown to be virus related by DNA hybridization techniques (Shafritz et al., 1982) .
All four serotypes of Dengue virus can be distinguished by monoclonal antibodies and have been applied with success to the identification of fresh low passage, virus isolates in an IF test (Henchal et al., 1983) . This was found just as reliable, but faster, than the usual plaque reduction tests. Influenza A and B viruses could be readily distinguished and, in some cases, typed satisfactorily even when there was insufficient virus present to permit hemagglutination inhibition testing . Finally monoclonal antibodies may find widespread future use in the diagnosis of plant virus disease. Recent work with antibodies specific for tobacco mosaic virus was able to distinguish two orchid strains which were previously thought identical with the tabomavirus type strain (Briand et al., 1982) . The further use of monoclonal antibodies in the wider aspects of diagnosis has been reviewed (Nowinski et al., 1983) .
Further application of monoclonal antibodies to virus identification has been made in the fields of virus epidemiology and taxonomy. In this manner the antibodies permit a fine analysis of intervirus relationships at both structural and biological levels. Monoclonal antibodies have been used to compare vaccine strains of yellow fever virus (YFV) derived in different laboratories. This confirmed the required similarity between vaccines in use and these strains could also be differentiated from virulent field isolates . A similar comparison of wild type polio virus andvaccine strains, Saukett and Sabin, has also been conducted (Crainic et al., 1981; Ferguson et al., 1982; Humphrey et al., 1982; Minor et al., 1982; Osterhaus et al., 1981a) . The use of monoclonal antibodies in the study of rabies infection has provided an important insight into the mechanism of virus pathogenicity (Section IV) and into the variation between virus strains. Many workers have compared field isolates using monoclonal antibodies directed against the virus glycoprotein and nucleocapsid protein. In this way differences have been found between viruses previously considered identical Charlton et al., 1982; Digoutte, 1982; Schneider, 1982; Witkor and Koprowski, 1980; . This may have an important bearing on instances in which vaccine failure has been observed. Variation in the nucleocapsid protein is probably not so important in this respect (Charlton et al., 1982) . Such differences also exist between morbilliviruses (Giraudon and Wild, 1981a,b; Server et al., 1982; ter Meulen et aL, 1981) . In addition, monoclonal antibodies have permitted analysis of the similarities and differences between hapadnaviruses (C8te et al., 1982) , parvoviruses (Burtonboy et al., 1982; Parrish et al., 1982) , rotaviruses Gary et al., Greenberg et al., 1983) , bunyaviruses (Gonzalez-Scarano et al., 1982) and to identify Zinga virus as Rift Valley fever (Meegan et al., 1983) .
At the present time the virus best characterized with monoclonal antibodies is that of influenza. The three-dimensional structure has been determined for both the hemagglutinin (HA) and neuraminidase (NA) ; Section III,A,3). The maintenance of influenza within the human population is related to two mechanisms. First, exchange of the hemagglutinin gene with one derived from an animal virus accomplishes a complete antigenic change (antigenic shift) in the virus and permits widespread human infection. Preexisting immunity is thus overcome and a worldwide pandemic may result. In the periods between antigenic shift, a more gradual alteration occurs in the virus proteins (antigenic drift) which in some cases may permit reinfection of the host population. Monoclonal antibodies have naturally been applied to these most interesting phenomena in an attempt to demonstrate the relationships between human, animal, and avian virus species, and also to demonstrate antigenic drift both in the field and the laboratory. Monoclonal antibodies provide the sensitivity required to analyze subtle structural alterations and are therefore well suited to the task. The seal influenza virus A/seal/Massachusetts/l/80 (H7N7) has been compared with viruses of avian origin . A close relationship was noted between hemagglutinin molecules from those sources suggesting avian viruses might have played a role in the evolution of the seal virus strain. In other studies differences have been noted between 25 avian (H4) influenza strains which may be related to the original host (Fukushi et al., 1982) and differences have been observed between neuraminidase (NA) molecules (Holmes et al., 1982) . Antigenic drift has been demonstrated among field isolates of influenza A (Underwood, 1982) and B . In addition nonneutralized HA or NA variants are readily selected by using monoclonal antibodies to apply selection pressure (Natali et al., 1981; Webster et al., 1982) . This demonstrates how such a process might occur under immunological pressure applied by the host. At least some of these laboratory selected variants might have been able to spread in the population since they were not neutralized by many samples of sera from potential hosts which did recognize the parent virus (Natali et al., 1981) . In contrast to the ready demonstration of variability among the influenza viruses, tick-borne encephalitis virus (TBE) isolates have remained very similar over the last 26 years (Heinz et ab, 1982) .
Ready differentiation between viruses permits analysis of virus spread within the population. At least two variants of influenza B could coexist in the population . The respective epidemiologies of herpes simplex virus 1 and 2 have also been studied and one patient may suffer from both viruses simultaneously (Peterson et al., 1983) . Monoclonal antibodies have permitted the analysis of mixed infections with closely related viruses in uitro. Thus, interference and exclusion were demonstrated between Dengue virus types 2 and 3, but a small proportion of cells could be doubly infected (Dittmar et al., 1982) . The use of these reagents permits the identification of the polypeptides carrying virus taxonomic markers (group, type, etc.) as demonstrated by Simian agent 12 , adenovirus 5 (Russell et al., 1981) , and herpes virus (McLean et Showalter et al., 1981) . This type of analysis could also indicate polypeptides which have been structurally conserved during the course of virus evolution.
The advantges of a technique which permits rapid and efficient virus identification are enormous. However, it is essential that the monoclonal antibody is chosen with care. Many will recognize carbohydrate determinants of glycosylated polypeptides, and this modification is influenced by the host cell in which the virus is grown (Klenk and Rott, 1980) . At least one instance is known where the type-specific target present on HSV glycoproteins synthesized in Hep-2 cells was not reactive when the virus was grown in Vero cells .
Second, the ability to detect differences is influenced by the technique used (Kendal et al., 1981) . Binding of monoclonal antibody to antigen may or may not disrupt the biological function of that protein; this could lead to errors if the tests involved monitor antibody binding indirectly by inhibition of target protein function. For this reason, procedures which simply demonstrate antibody binding are to be preferred in diagnostic virology. Immunofluorescence is the method of choice for this purpose since it is rapid and relatively simple. Furthermore, it is applicable directly to biopsy samples and provides additional information on the histological distribution of the antigen examined. However, binding alone is an insufficient guide for research purposes, since the functional significance of the same epitope may vary with the virus strain and mutations may render an antibody unable to inhibit protein function without abolishing antibody binding (Kendal et al., 1981) . It is necessary therefore to perform a number of tests in order to extract the maximum possible information.
Monoclonal antibodies provide us with a tool which is able to examine the individual protein within a structure as complicated as the whole cell.
It is possible to investigate, both in uitro and in viuo, the role of a protein.
It is also possible to determine the active areas of the molecule and to monitor its synthesis and processing within the infected cell. The ability to distinguish related proteins can be used to provide a genetic marker in recombination experiments (Gonzalez-Scarano et al., 1982) .
The combination of antibody with antigen can be used to provide structural information on several levels. First, just as it is possible to identify proteins carrying a taxonomic marker, so it is possible to identify a protein as performing a particular function once an antibody is available which is capable of inhibiting that function. Second, antibodies binding to the same target protein differ in their capacity to produce function inhibition of that protein. A panel of monoclonal antibodies is frequently divided into groups according to their effects on the activity of a target protein, or family of proteins. In this way epitopes may be assigned a functional significance. If certain assumptions are made, it is then possible to conduct competitive binding analyses to arrange the antibody sites relative to each other, and a schematic map of the molecule's surface can be drawn up. Frequently virus variants can be selected to which a given antibody can no longer bind. These may also be analyzed for loss of protein function or loss of capacity to bind other monoclonal antibodies. In this way the map can be refined, or indeed constructed entirely. Third, in order to explain function in terms of structure it is necessary to orientate the map thus obtained with the physical structure of the antigen. This can be done by determination of the amino acid alterations produced in the variants described above, either directly by partial proteolysis and examination of those molecule fragments bound by the antibody, or from genome sequence determination in the region of the alteration. Active amino acid residues can thus be distinguished and positioned in the primary structure of the protein. The location of the antibody binding site is then known if the three-dimensional structure of the protein has been determined, or clues may be obtained from immune electron microscopy (IEM).
The whole process described above has met with varying success in application to a number of viruses. The most complete of such determina-tions has been derived in the study of the influenza virus hemagglutinin and neuraminidase.
The target protein of a monoclonal antibody is usually detected by radioimmune precipitation or western blot procedures (Section I). In this manner, those proteins to which neutralizing antibodies are directed have been determined, e.g., VP1 in poliovirus (Osterhaus et al., 1981b; Thorpe et al., 1982) , VP2 in Bluetongue virus Letchworth and Appleton, 1983) , and gp 56 in Venezuelan equine encephalitis virus (Roehrig et at., 1982a) .
The glycoprotein GP-1 of the coronavirus MHV was proven responsible for cell attachment and virus spread through cell/cell fusion (Collins et al., 1982) . A modified immunoprecipitation experiment has demonstrated that a subset of the SV40 T antigen present in the infected cell is able to bind to the virus origin of DNA replication. The antibody used in this work was apparently capable of distinguishing between T antigen with this activity and T antigen which could not perform this function (Scheller et al., 1982) . A similar analysis has identified the cellular RNA polymerase which binds to the adenovirus 2 promotor in vitro (Dahmus and Kedinger, 1983) , and microinjection techniques have demonstrated that antibody specific to the transformation related protein p53 in 3T3 cells is capable of preventing the seruminduced onset of DNA synthesis (Mercer et al., 1982) . Although the last two experiments are concerned with the function of cellular proteins, this approach has an obvious application in the study of virus replication and transcription. Such an approach could also investigate the involvement of host -cell proteins in these processes. Further insight into the function of tumour virus proteins in transformed cell survival has come from work involving monoclonal antibodies specific for p15 E, a structural component of type C retroviruses. This protein was detected in cancer effusions and found to be highly efficient at blocking monocyte response to chemoattractants, a function which could protect a cancer from attack (Cianciolo et al., 1981) .
This analysis seeks to define active areas of the protein in terms of epitopes recognized by a panel of monoclonal antibodies. In the main these experiments have been conducted on virus surface proteins which have easily monitored biological functions such as hemagglutination, neutralization, hemolysis, or neuraminidase activity. In principle, the same approach could be extended to any protein with a measurable activity. Combination of antibody with antigen can have a variety of effects, de-pending on the site of attachment. Some functions which might previously have been thought associated, have been found separable when highly specific monoclonal antibodies were used. A polyclonal monospecific serum which coats the protein surface with antibody will normally inhibit all protein functions, but a monoclonal antibody, which binds away from the active site need not have any detectable effect at all (Burstin et al., 1982; Massey and Schochetman, 1981; Pinter et al., 1982; Thorpe et al., 1982) .
By means of this function inhibition analysis, a panel of monoclonal antibodies specific for the measles virus hemagglutinin was divided into five groups : group 1 antibodies had no detectable activity, in HI or NT assays; group 2 exhibited HI activity but failed to neutralize virus; group 3 consisted of antibodies with similar activities in both tests. Antibodies of group 4 revealed a significantly higher activity in the HI than the NT tests whereas this was reversed in group 5. The antibodies used in that study were deliberately selected from those which had undetectable HLI activities. This report therefore documents the separation of hemagglutination (a model for virus attachment to its target cell) from hemolysis (a model for virus penetration by fusion reactions). This indicates that the requirements underlying these reactions must differ. Both of these tests in fact are artificial, since the virus does not normally act to cross link cells, or to lyse the cell it is about to attack, and these results indicate that it is possible to prevent stable cell cross linking in circumstances which still permit sufficient virus/cell association for action of the fusion protein. Similarly, antibodies may inhibit hemagglutination but still permit sufficient cell contact such that infection is not inhibited (Burstin et al., 1982; Gentry et al., 1982; Gonzalez-Scarano et al., 1982; Kimura-Kiroda and Yasui, 1983; ter Meulen et al., 1981. This type of analysis provides clues that the functional areas of a multifunctional protein are distinct. For instance the paramyxovirus HN protein has two activities, cell attachment and neuraminidase. These probably reside at different areas of the molecule since some monoclonal antibodies inhibit only neuraminidase (Orvell and Grandien, 1982) , and virus variants have been obtained under monoclonal antibody selection pressure which are deficient only in neuraminidase (Portner, 1981) . In the same way, antibodies are known which neutralize virus without blocking hemagglutination. Presumably these antibodies bind to sites important in virus penetration but not absorption. This phenomenon is exemplified by Sendai virus (Miura et al., 1982) , influenza virus , Sindbis virus (Chanas et al., 1982a) , and reovirus (Burstin et al., 1982) . Other biological functions have been used to characterize epitopes defined by competition ELISA on the envelope glycoprotein of bovine leukemia virus (Bruck et al., 1982a) . This analysis concentrated on BLV/ VSV pseudotype formation inhibition, polykaryotosis inhibition (cell/cell fusion following infection), and complement fixation (Bruck et al., 1982b) .
Monoclonal antibodies directed to virus surface proteins are generally more readily produced, but as more become available specific for virus internal proteins, this approach can be expected to yield further information on their function. A function inhibihon assay has been applied to delineate that area of the SV40 T antigen involved in ATPase activity (Clark et d., 1981) and to demonstrate that some areas of the influenza and VSV nucleocapsid proteins may be associated with the transcription process (De et al., 1982; van Wyke et d., 1981) .
Once epitopes are identified it is necessary to determine their topological relationship to each other on the surface of the molecule. This is conveniently achieved by a competitive binding analysis although selection of variants has also been extensively used.
Competitive binding is frequently examined in RIA or ELISA. One monoclonal antibody carries the appropriate marker and unlabeled antibodies are assessed for their capacity to inhibit the combination of the labeled antibody with antigen. If competition is observed, the antibodies are said to belong to the same binding group. However, several precautions must be taken in the interpretation of data before meaningful conclusions can be reached. It is assumed that two antibodies are mutually competitive if their binding sites overlap. The binding site of a second antibody is then wholly or partially masked by the binding of the first antibody and binding is truly competitive in nature. However, this is not the only explanation for such an observation. Binding sites may be distinct but close together, such that the bulk of the first antibody molecule sterically prevents access of a second to its binding site. These two possibilities cannot be readily distinguished but do at least both imply proximity of attachment sites. In fact, a monoclonal antibody bound to a single epitope on its target protein may have considerable "swivel" movement available to it rendering steric effects relatively unimportant. Stone and Nowinski (1980) reported that two IgG molecules (MW 150K) could bind noncompetitively to a retrovirus 15K protein. However, immunoglobulin class may well be important here since oligomeric IgM would obviously provide a greater steric hindrance than IgG. If steric effects are suspected they may be minimized by the use of monomeric antigen-binding submolecular antibody fragments.
It is however quite possible that allosteric inhibitory effects might also occur which would be indistinguishable from true competitive binding.
Indeed positive allosteric effects have been observed and antibody pairs identified which bind synergistically (Lefrancois and Lyles, 1982a,b; Lubeck and Gerhard, 1982) . Similar effects resulting in competition may be widespread but are difficult to demonstrate. Combination of monoclonal antibody with influenza neuraminidase modifies the reaction kinetics (Mountford et al., 1982) and combination with HSV glycoprotein can alter the pattern obtained by partial proteolysis (Eisenberg et al., 1982a) . These effects could also be mediated by antibody-induced conformational change.
Finally, two antibodies directed at the same site will compete in a manner related to their relative avidities or concentrations. Taking an extreme case, a weakly binding antibody would not show detectable competition with a labeled, strongly binding immunoglobulin, but the reverse is clearly not true. In practice a spectrum of relative interference efficiencies will probably be obtained (Lefrancois and Lyles, 1982a,b; Stone and Nowinski, 1980) . The extent of interference obtained will be influenced by the antibody avidity as well as the proximity of the binding sites. For this reason binding sites should not be distinguished on the basis of the extent of competition, it is the observation of competition itself which is significant. Furthermore, while the observation of competition assigns two antibodies to the same binding group, it is not sufficient to separate them merely on the basis of failure to compete. Any such noncompeting antibodies should be examined in the reverse experiment (i.e., with the other partner carrying the marker) to exclude avidity effects as described above. If this procedure fails to reveal competition, then allocation to separate binding groups is justified. Alternatively, determination of antibody avidity may obviate the need for this analysis but one-way competition effects can still occur even in the case of antibodies of similar avidity and concentration (Carter et al., 1982) . The reasons for this are unknown but might involve some form of allosteric effect. Determination of antibody avidity may be performed by a rapid method (Jackson et al., 1983) or relative antibody avidities may be estimated from RIA saturation curves (Carter et al., 1982; Massey and Schochetman, 1981; Stone and Nowinski, 1980) . The competitive binding procedure has been applied with success to many viruses. Monoclonal antibodies specific for the measles virus hemagglutinin were grouped into five sets on the basis of their ability to influence the biological functions of that molecule and these were then sorted into three binding groups by competition radioimmunoassay (Carter et ul., 1982) . A similar approach has produced a detailed map of the VSV G protein including epitopes instrumental in virus neutralization in which determinants were identified as cross-reacting or specific to the strains Indiana or New Jersey (Lefrancois and Lyles, 1982a,b; Volk et al., 1982) . Japanese encephalitis virus (Kimura-Kiroda and Yasui, 1983) and RNA tumor viruses (Bruck et al., 1982a,b; Massey and Schochetman, 1981; Niman and Elder, 1982; Pinter et al., 1982) have also been extensively analyzed. The binding group analysis forms a sensitive probe for alterations in protein surface structure . The manner in which the sample is prepared can also influence the conformation and/or exposure of the reacting epitopes and this should be considered in the design of such experiments (Bruck et al., 1982b) .
The second approach to the problem of arranging binding sites relative to each other is to select virus variants under monoclonal antibody selection pressure. In the case of neutralizing antibodies this is relatively straightforward. Nonneutralizing antibodies could also be applied to enveloped viruses if used in conjunction with complement. Virus variants may then be isolated to which the selecting antibodies can no longer bind, or in which antibody combination no longer results in neutralization. These viruses are then tested with a panel of monoclonal antibodies to determine which other antibodies also show altered binding characteristics. In this way, a map of epitopes can be constructed . The virus variants are commonly already present in the population at a frequency of (Emini et al., 1982; Portner et aL, 1980; Prabhakar et al., 1982; Webster et al., 1982) which presumably represents the error rate in virus transcription. This results in amino acid substitutions either within the antibody binding site or at a position which influences the structure of the binding site. This type of experiment provides a finer analysis of the relationships between binding sites because steric hindrance is eliminated. Variant selection and mapping has been applied in the main to the HA and NA proteins of influenza. The extremely detailed studies performed by Laver et al., (1979 Laver et al., ( ,1980a and Gerhard et al., (1981) exemplify this approach. However, regardless of the method in which epitope topography is determined, the schematic map thus produced must be oriented on the physical structure of the protein and this necessitates a detailed knowledge of the molecule.
Two approaches have been used to achieve this objective. First, antibody binding sites can be localized to a particular peptide fragment following partial proteolysis, and second, the amino acid substitutions occurring in the antibody-selected variant proteins can be determined. Niman and Elder (1982) have conducted a comprehensive analysis of the Rauscher virus gp 70. Products of partial proteolysis were identified by reactivity with antisera directed against synthetic polypeptides predicted from the gene sequence. The other polypeptides were assigned by peptide map-ping. In this way a linear proteolytic leavage map could be derived for 8 different enzymes. Monoclonal antibodies were then analyzed for their ability to immunoprecipitate various polypeptide fragments and their binding sites could then be assigned in the molecule's primary structure.
It was found that antibody binding sites were closely grouped in two areas which presumably reflected regions of the protein exposed for immunological attack. A similar analysis located eight epitopes, previously defined by their reaction with a panel of monoclonal antibodies, in the proteolytic cleavage map of gp 70 from murine leukemia virus (Pinter et al., 19821, and antibody sites have also been localized on gp 51 of bovine leukemia virus, a 15K molecular weight fragment contained areas involved in biological activity (Bruck et al., 1982b) . Similarly, a herpes simplex virus glycoprotein type common determinant has been localized to a 12K fragment of a larger (38K) peptide which also contained two type-specific determinants (Eisenberg et al., 1982a) . Other studies have located the active site of SV40 T antigen ATPase by means of well characterized deletion mutants. It was found that enzyme activity and antibody binding required the region of the A gene from 0.37 to 0.29 map units (Clark et al., 1981) .
Provided the primary sequence of the molecule is known, virus variants can supply highly specific information on the location of antibody binding sites. Unlike antibody binding to a peptide fragment, however, it is not possible to be absolutely certain that the amino acid affected actually constitutes part of the binding site itself. This method has been extensively applied in the study of influenza. Amino acid alterations can be detected by peptide mapping (Laver et al., 1979 (Laver et al., , 1980b or genome sequencing (Caton et al., 1982) . Since the three-dimensional structure of the influenza hemagglutinin is now available these binding sites could be easily located . Analogy with this model has permitted the location of such sites on other strains of influenza (Caton et ul., 1982; Jackson et al., 1982) . These studies have revealed the presence of distinct areas on the HA molecule within which the amino acid substitutions predominantly occurred, These areas were also implicated in the production of antigenic variation associated with the process of antigenic drift (reviewed by Ward, 1981) .
The three-dimensional structure of the influenza virus NA molecule is now known permitting the location of monoclonal antibody binding sites to be identified . This also provided insight into those areas of the molecule involved in enzyme function and previously identified by monoclonal antibodies as well as locations in which antigenic drift could occur. This level of refinement in our knowledge of structure, function, and anti-genicity has so far been reached only in influenza but a similar approach has located the neutralization sites of poliovirus type 3. This was found to be a small (eight amino acid) sequence in protein VP1 Minor et al., 1983) ; however no three-dimensional structure is yet available for this virus.
A further clue to antibody binding site location may be obtained from immune electron microscopy in which the antibody is used in conjunction with an electron-dense material such as ferritin or hemocyanin (Gonda et al., 1981) . Chanas et al. (1982a) demonstrated that hemagglutination inhibiting monoclonal antibodies attached near the tip of the Sindbis virus peplomer, while hemolysin inhibiting antibodies attached lower down, near the virus membrane. A similar situation has also been demonstrated in influenza and may explain the separation of hemagglutination inhibition and neutralization exhibited by monoclonal antibodies specific for the HA protein of seal influenza .
In just the same way as monoclonal antibodies provide a sensitive probe for virus detection in diagnosis (Section I1,A) so they can also be used to detect low amounts of individual virus proteins within the infectedcell. In this way they can answer questions of molecular and cell biology. They can thus provide information concerning temporal and spatial separation of protein formation and accumulation, and data on protein modification and processing in the infected cell.
Many studies have been performed to demonstrate temporal control of protein synthesis within the lytic virus infection, e.g., Sendai virus (Orvell and Grandien, 1982; and measles virus (Norrby et al., 1982) . It is not the object of this review to list all these studies. Rather we shall consider persistent infections where normal protein synthesis is modified or controlled such that virus and cells may coexist. Interesting information has been gathered in this area which frequently involves low levels of infected cells or proteins within those cells and monoclonal antibodies are thus admirably suited for investigation of this phenomenon.
Measles virus is associated with a persistent slowly progressing fatal infection of the human central nervous system (CNS) termed subacute sclerosing panencephalitis (SSPE) (reviewed by ter . This disease is associated with a failure to produce mature virus or to develop the typical measles virus cytopathology, cell fusion in brain tissue. An examination of the patients' immune response during this illness demonstrated a lack of antibodies directed against the virus matrix (M)
protein . A direct search by immunoprecipitation and western blot techniques has failed to demonstrate the production of this protein in infected brain or brain explants . The clinical importance of this disease has prompted an examination of measles virus polypeptide expression in persistently infected cells in uitro. Studies using several monoclonal antibodies failed to detect M protein in persistently infected tissue culture cell lines (SSPE cell lines) derived from SSPE patient brain (Carter et al., 1984) . Although this method did permit identification of M protein in a laboratory-established persistent infection which was previously thought not to express this polypeptide . Monoclonal antibodies thus provide excellent evidence that matrix protein is not synthesized in SSPE cell lines. The lack of this major structural polypeptide is thought to prevent virus particle maturation and account for the slowly progressing nature of the disease. Measles virus persistently infected cells show a low expression of membrane glycoproteins (Norrby et al., 1982) ' although expression is normally sufficient to permit development of syncytia. The expression of virus polypeptides has also been investigated within mouse brain persistently infected with lymphocytic choriomeningitis (LCM) virus (Oldstone and Buchmeier, 1982; Rodriguez et al., 1983) , Sendai virus , and on the surface of spleen cells from mice infected with Friend leukemia virus (Britt et al., 1981) . In these cases the level of virus glycoproteins detected on the cell surface was also reduced. Perhaps this is one way in which persistently infected cells evade the immune system and thus permit the maintenance of infection.
Monoclonal antibodies permit the selective detection of virus-specific transformation markers on the transformed cell surface, e.g., Epstein -Barr virus (Kintner and Sugden, 1981; Slovin et al., 1982) and Marek's disease virus (Lee et al., 1983b) . Antibodies further permit the detection and characterization of transformation specific proteins as well as some investigations of their function. Monoclonal antibodies directed against the v-ras gene product of Harvey sarcoma virus have been produced. The products of the v-rczs gene family in viruses, transformed and normal cells were shown to share similarities, and the antibodies were able to confirm that the protein is located at the inner surface of the membrane and binds guanine nucleotides (Furth et al., 1982) . In addition, a tumor antigen of adenovirus 5 has been shown to accumulate in the cell nucleus (Sarnow et al., 1982) .
The specificity of the monoclonal antibody confers the ability to react with a family of related proteins. This property is particularly relevant where precursorproduct relationships exist (as in proteolysis), or where similar sequences of proteins might occur in different molecules, e.g., following a recombination or readthrough event in transcription. If the precursors never reach appreciable levels within the cell or the shared sequences are small, the cross reacting proteins are difficult to detect with polyclonal serum.
Small virus-specific polypeptides which reacted with monoclonal antibodies specific for glycoproteins A and B were detected in HSV-infected Vero cells (Pereira et al., 1981) . These were not identified in virus-infected Hep-2 cells and were later shown to be proteolytically related to the intact forms of the glycoproteins (Pereira et al., 1982a) . Families of related proteins were also detected in this manner in CMV infected cells . A 15K cleavage product of the measles virus nucleocapsid protein has also been identified (Carter and Baczko, 1983) . This type of analysis defines aprotein family but does not indicate whether the submolecular fragments serve any purpose. Furthermore, care should always be taken to ensure that the protein precipitation is not caused by a spurious cross reaction with a host cell polypeptide (Section V).
Monoclonal antibodies have also permitted the identification of novel virus proteins. A new cell surface marker was discovered on B cell lines known to harbor EBV (Slovin et al., 1982) , a new HSV glycoprotein has also been detected and characterized (Balachandran et aL, 1981 ) and a new protein (gp 70) was detected in leukemic cells from mice infected with Friend leukemia virus. This protein was apparently derived from a virus produced by a recombination event during infection, and had lost cross reactivity with a monoclonal antibody specific for the ecotropic Friend murine leukemia virus (Britt et al., 1981) . An unusual form of composite protein was demonstrated in measles virus infected BGM cells and was apparently not produced in other infected cells. The protein was detected because it cross-reacted with a monoclonal antibody specific for nucleocapsid protein, but the new molecule was 24K larger. Peptide mapping analysis showed that it apparently contained all the nucleocapsid peptides plus some new ones whose origin could not be determined (Wild and Giraudon, 1982) . No precursor -product relationship could be demonstrated between this new protein and the nucleocapsid protein. The origin of this protein is still obscure but a readthrough mechanism was suggested. A similar finding has also been reported using monoclonal antibodies in the study of HSV 1 and 2 infections (Zweig et al., 1980) . A polypeptide was observed which was larger than the nucleocapsid protein and contained additional peptides but apparently had no precursorproduct relationship to the smaller nucleocapsid protein.
Following its synthesis, a protein may mature through posttranslational processing. Processing in the sense used here includes covalent modification through glycosylation, posttranslational cleavage etc, as well as maturation by conformational changes often associated with the formation of intermolecular associations. This concept is particularly relevant in the context of virus assembly where stable associations must occur leading to the production of a viable particle. Conformational change is probably involved in this process and may perform a regulatory function as in tobacco mosaic virus assembly (Butler, 1971; Klug and Durham, 1971 ).
a. Modification and Transport. The novel proteins formed by proteolysis in some HSV-infected cells have already been mentioned (Section III,B,2). These proteins are degraded only in some cell types (Pereira et al., ,1982a which suggests this process is not a functionally important processing phenomenon. However, monoclonal antibodies have been used in pulse-chase experiments to demonstrate genuine modification of proteins through glycosylation (Balachandran et al., 1981) and also to demonstrate temporal regulation of their synthesis (Showalter et al., 1981) . Misra et al., (1982) have studied the maturation of an HSV-1 early glycoprotein (GVP-11). These workers discovered that tunicamycin-sensitive glycosylation was necessary for the cell surface expression of this protein. However, monoclonal antibodies may not react with the unmodified form of a protein (only one antibody from a panel of 65 directed against measles virus H protein reacted with the unmodified product formed in vitro; M. J. Carter, unpublished observation) and consequently might not always provide an adequate probe for the expression of the polypeptide moiety. A polyclonal serum should therefore always be used. Opposite results have been obtained with measles virus H protein. Glycosylation was not required for insertion into the rough endoplasmic reticulum and intracellular transport of the protein to the plasma membrane. This detailed study also elucidated intermediates formed in the protein modification pathway leading to the mature molecule (Bellini et al., 1983) .
A comprehensive immunofluorescence study using monoclonal antibodies has examined the distribution of a number of Moloney murine leukemia proteins on the infected cell membrane (Satake et al., 1981; Satake and Luftig, 1983) . These workers were able to demonstrate that the mem-brane sites at which virus antigens accumulated were often distinct and differentially controlled by the cellular microtubule system. In addition, they were able to demonstrate by double dot immunofluorescence that two virus-specific antigens, P15 and P15 E, accumulated at the same sites. This association between a gag and an env gene product may be important for virus assembly.
In the formation of a virus particle stable intermolecular associations must be formed. It is likely that protein combination to form virus structural components will involve conformational changes. Protein conformation could also be altered artifactually during extraction procedures. Although some antibodies seem to recognize a sequence of amino acids, most monoclonal antibodies possess a sensitivity to the conformation of the epitope that cannot be visualized using a polyclonal serum, and this permit investigations into this complex field of structural isomerism. Alternatively the development of intermolecular associations must result in the masking of some epitopes and therefore the inhibition of combination with antibody directed toward that area of the protein.
Monoclonal antibodies could distinguish readily between native and unfolded forms of the Sindbis virus glycoprotein El (Roehrig et al., 1982b) and between native (N) and heated (H) forms of the poliovirus capsid Icenogle et al., 1981) . In the latter case it was also shown that the irreversible conversion of N to H could be accomplished by some acidic isolation procedures . This explained an earlier finding that some capsids were in the H form inside the infected cell (Ferguson et al., 1981) . More biologically relevant information concerning virus assembly has also been derived from studies of poliovirus. Two monoclonal antibodies were obtained which bound to poliovirion precursor structures. The sites recognized were different, one antibody bound to infectious virions, 80 S empty capsids in the N form and to 14 S precursor subunits, the other bound only to virions and empty capsids. Neither antibody bound to the heat-treated particles. Furthermore, the antibody binding sites were both shown to be located on VP1 by chemical cross linking, but neither antibody bound well to free VP1 in the native or denatured form. This indicated the sites recognized were formed only in the capsid structure. However, variants could readily be selected which did not express these sites, so it is likely that the areas recognized by these antibodies are not vital for structural integrity (Emini et al., 1982) . Similar data have been reported for foot and mouth disease virus (FMDV) where monoclonal antibodies have been obtained which can distinguish between complete (146s) virions and their precursor (12 S) subunits (McCullogh and Butcher, 1982) . These two studies demonstrate that unique antigenic sites are created during picornavirus maturation and explain earlier reports that neutralizing antibodies could only be elicited by using the mature polio virion as immunogen (Dernick, 1981) . A similar effect has been demonstrated in the case of TMV where some monoclonal antibodies react only with the nucleocapsid, and others only with the protein monomer (Dietzgen and Sander, 1982) . Although the former class must recognize a new site caused by conformational change or formed at molecular junctions, the latter class of antibodies could be directed at a site which is either masked in the protein complex or destroyed through conformational change.
The pathway of adenovirus hexon polymerization has also been investigated with conformation-specific monoclonal antibodies. The monomeric or denatured hexon protein lacks antigenic determinants in common with the trimeric capsomere, a situation reminiscent of the picornavirus capsid. Using these conformation-sensitive monoclonal antibodies, Cepko and Sharp (1982) have shown that trimerization of the hexon protein involves a nonstructural lOOK virus-specific polypeptide. Three hexon molecules bind to this protein in the monomeric configuration and are released as a trimeric capsomere. The capsomeric conformation is adopted concomitantly with release from the 100K "scaffolding" protein.
A structural rearrangement may also occur during release of CMV since some antibodies are able to react with determinants and neutralize infectious virus but are unable to bind to the same proteins on the surface of infected cells . Conformational changes are believed important in the development of fusion protein activity by the influenza virus hemagglutinin at low pH (Skehel et al., 1982) . Monoclonal antibodies have been used to demonstrate this structural change directly, and to locate areas involved in the molecule's structure (Daniels et al., 1983) .
Virus architecture, that is the proximity of structural proteins, is also amenable to examination. For instance gp 56 and gp 50 have been shown closely associated on the Venezuelan equine encephalitis (VEE) virus envelope by means of competitive binding studies (Roehrig et al., 1982a) . A similar examination showed that the influenza hemagglutinin was close to the cellular H-2K histocompatibility antigen on the surface of the infected cell. A complex is not apparently formed since these antigens did not cocap (Hackett and Askonas, 1982) . On the mature influenza virus envelope however, the HA protein is often found in close association with the NA protein, since monoclonal antibodies specific for hemagglutinin may have a neuraminidase inhibiting activity . Frequently, virus-specific products associate with cellular proteins and this can be demonstrated by competitive binding assays or coprecipitation experiments (Carroll and Gurney, 1982; Ferracini et al., 1982; Sarnow et al., 1982) . c. Mutations. Structural change can also result from the acquisition of mutations by the virus genome. During persistent infection a virus genome is able to accumulate mutations rapidly (Holland et al., 1979) . The resulting structural alterations in virus proteins can be detected by monoclonal antibodies. Differences have been found in the glycoproteins synthesized by lytic transforming EBV and by the nontransforming virus shed from persistent infections . Such changes have also been demonstrated in the measles virus hemagglutinin by immunofluorescence . A similar process has been detected by alterations in competitive binding behavior using monoclonal antibodies specific for the measles virus matrix protein . In this study an apparent separation of binding groups was observed. A monoclonal antibody was found to have lost its capacity to compete with a second even though both still immunoprecipitated the M protein. This example illustrates that the detection of structural change is dependent on the method used. An examination based solely on radioimmune precipitation may be superior in detecting certain changes which "loosen" the protein and render it more susceptible to the action of detergents in the buffer used. However, changes in relative avidity, topography, or allostery would not be detected provided antibody avidity was still sufficiently high to permit precipitation (Section I). Similarly, detergent produced effects might not be detected in the competition RIA alone.
In any analysis of virus-specific protein structure and function, it is always desirable to obtain pure preparations of the protein in question to permit in uitro experimentation or vaccine production. One obvious example of the use to which monoclonal antibodies can be put is in the preparation of affinity columns for such purifications. It is not appropriate to list here all instances in which this has been performed, but any review of the application of monoclonal antibody technology would be incomplete without mention of this subject. The application of the technique is exemplified in the purification of glycoproteins from HSV 1 and 2 (Eisenberg et al., 1982b) and the measles virus hemagglutinin (Bellini et al., 1981) .
When used in RIA or ELISA monoclonal antibodies provide a convenient and accurate method for the quantitation of viral proteins (Lutz et al., 1983) .
The study of a virus as a pathogen is more complicated than studies conducted in uitro. These examinations frequently cross the boundary between virology and immunology. Monoclonal antibodies may be used to
The isolation of nonneutralizable virus variants has already been discussed (Section II1,A). These variants can also be applied in studies of pathogenicity as well as protein structure. Spontaneous virus variants occur at a high frequency (lo-*) in Coxsackie B4 virus populations (Prabhaker et al., 1982) and this may explain the high variation in disease pattern produced by these viruses. Possibly alterations in the virus attachment protein might result in an altered tissue tropism. However, the most interesting data have so far emerged from studies of rabies and influenza virus.
The selection of naturally arising antigenic variants in the rabies virus glycoprotein was first reported by . Coulon et al.(1982a,b) applied this method to select variants using three monoclonal antibodies and noted that while two antibodies led to the isolation of variants indistinguishable in virulence from the parent virus stock, one antibody selected avirulent viruses.
These variants were very similar to the parental virus by all other criteria, but differed in their capacity to evoke a strong and rapid immune response which could lead to successful defense against the infection (Coulon et al., 1982~) . More monoclonal antibodies were then used to locate the virulence-related epitope on an antigenic map (Coulon et al., 1983) . Similar experiments led Dietzschold et al., (1983) to the identification of a single amino acid change in nonpathogenic variants of rabies virus. The arginine residue present in the virulent virus glycoprotein at position 333 was substituted in all avirulent variants tested. Such differences have been identified (Section I1,B) at other positions in the virus proteins. Broadly similar results have also been obtained in studies of reovirus. Monoclonal antibodies which neutralized the virus (Burstin et al., 1982) were used in the selection of variants. These variants were found to exhibit reduced neurovirulence compared with the parent strain . The altered epitope was also demonstrated to be the major site of recognition for virus specific cytotoxic lymphocytes (Finberg et al., 1982) .
The use of monoclonal antibodies in the study of antigenic drift in influenza virus has already been discussed in the context of the structural information derived from such experiments (Section II1,A). Although antigenic drift has been studied mainly in the HA molecule (Natali et al., 1981; Underwood, 1982; it may also occur in the neuraminidase but its extent may be restricted in some species of avian virus . Knowledge of the basis for such drift is now approaching that for the HA molecule (Colman et aZ., 1983) . The subject is mentioned again here since it has a direct bearing on the pathogenesis and survival of influenza viruses in the population. The role of the HA in this drift has recently been reviewed (Ward, 1981) .
Monoclonal antibodies can be applied in order to mimic the immune response and to gain insight into the effect of immunological pressure on the virus. Experiments using monoclonal antibodies to block cytotoxic lymphocyte action have demonstrated that the epitopes recognized by these cells may be identical to (Finberg et al., 1982) or distinct from (Lefrancois and Lyles, 1983b) those regions to which neutralizing antibodies are directed.
The host immune response has been implicated in the maintenance of measles virus persistence leading to SSPE. As described in Section JII,B, the disease is associated with a defect in the production of virus M protein. Some evidence has been gathered that suggests the hosts' immune response itself may actually modulate the synthesis of virus polypeptides and consequently help to bring this situation about (Fujinami and Oldstone, 1979 ). This has been modelled in uiuo where inoculation of a hyperimmune anti-measles virus antiserum was found to alter the characteristics of disease in intracerebrally inoculated BALB/c mice, a delayed disease was then produced. Inoculation of monoclonal antibody specific for the virus hemagglutinin protein was also able to induce this effect although monoclonal antibody directed against the nucleocapsid protein could not (Rammohan et al., 1981) . A similar process has been demonstrated with a measles virus persistent infection in uitto. Antiserum treatment of C6 cells persistently infected with measles virus led to a drastic reduction in the expression of virus polypeptides (Barrett and Koschel, 1983) . Once again monoclonal antibody to H, but not N could produce this effect (P. N. Barrett, personal communication) . Antibody to surface glycoprotein can also prolong experimental Sindbis virus infection (Chanas et al., 1982a) . Antibodies with neutralizing activity were active in this respect while those which lacked this property were not. This is also reflected in the measles virus system described above. Monoclonal antibodies which neutralized the virus seemed to be exclusively active in producing the alteration in virus polypeptide expression (P. N. Barrett, personal communication) . However, it is also true that under some circumstances monoclonal antibodies can enhance virus infection. Flaviviruses, in combination with antibody, are able to infect cells bearing Fc receptors through a cross bridging mediated by the antibody (Chanas et al., 1982b; Peiris et al., 1982; Schlesinger et al., 1983) . However, use of monoclonal antibodies has demonstrated that the virus epitope to which the antibody is bound is also important. Brandt et al. (1982) found only flavivirus type common determinants were effective, and second, that some cells were unable to support YFV replication even when antibody enhancement was attempted. Consequently the presence of the Fc receptor on the target cell surface is not alone sufficient for productive infection.
Use of monoclonal antibodies for therapeutic purposes is as yet in its infancy. It is likely that the antibodies would be used in three main areas. First, in emergency, injection of a neutralizing antibody can aid recovery from disease. Human monoclonal antibodies are now becoming available (Crawford et al., 1983; Sikora and Neville, 1982) and are likely to produce fewer problems with allergic reaction. Animal experiments in the mouse have indicated that monoclonal antibodies will be beneficial in this area. Neutralizing antibodies are able to localize HSV infection and prevent spread to the nervous system (Kapoor et al., 1982) , promote recovery from ocular infection (Rector et al., 1982) or protect against a later footpad challenge (Dix et al., 1981) , and antibodies to any herpesvirus glycoprotein had protective activity. Intravenous inoculation of monoclonal antibody specific for the virus hemagglutinin was found to protect mice from the invariably lethal consequences of intracerebral injection with influenza WSN. This action was however dependent upon the breakdown of the blood-brain barrier (Doherty and Gerhard, 1981) . The ability of antibody to function in this way is not simply correlated with its anti-virus action measured by in uitro assays. Nonneutralizing antibodies protect mice from HSV (Balachandran et al., 1982c; Rector et al., 1982) or Sindbis virus (Schmaljohn et al., 1982) , and Mathews and Roehrig (1982) found nonneutralizing antibodies could also protect mice from Japanese equine encephalitis infection. Antibodies of high avidity protected with greater efficiency than those of lower avidity, but antibodies directed against epitopes instrumental in neutralizing the virus were effective at lower doses. This work therefore implicates the tightness of attachment as well as site of combination with antigen in efficiency of protection.
Some evidence suggests that complement is not involved in protection by monoclonal antibodies and suggests the process is mediated by an antibody-dependent cell-mediated cytolysis (Balachandran et al., 1982~) . Second, monoclonal antibodies will be applied to the selection of avirulent viruses for vaccine purposes. Rabies virus has already been described in this context (Section IV,A). Heterogeneity among Coxsackie (Prabhakar et al., 1982) or bluetongue viruses ) may permit such an approach. The antibodies could also be used in the preparation of pure subunit vaccines (Osterhaus et al., 1981b) . Finally, when combined with a cytotoxic agent, monoclonal antibodies provide a specific cell killing tool (Davies, 1981) . Specific cytotoxicity has already been demonstrated using this technique (Blythman et al., 1981; Krolick et al., 1980; Youle and Neville, 1980) .
Monoclonal antibodies allow us to view the fate of a protein in the infected cell, from synthesis to virus assembly, as a continuous process and hint at the interaction between virus-specific gene products and the cellular machinery. The use of monoclonal antibodies provides valuable insight into the working of the protein both as an enzyme and as a target for the host immune response, evolving in reaction to that response. As three-dimensional structures of more molecules are determined, so this work should extend our understanding of virus protein structure and function.
In the foregoing discussion it has been emphasized how the specificity of a monoclonal antibody can be applied to the study of all areas of virology. In each case it is the specificity of the antibody which has proved useful, and even permitted the identification of related but hitherto unknown proteins. However, this very specificity also provides the basis for spurious cross reactivity not normally present in polyclonal serum. In general, an appropriately absorbed monospecific antiserum will recognize many epitopes and thus coat a considerable area of a protein's exposed surface. A single epitope repeated on another protein would cross react, but this would be masked by the vast majority of antibodies reacting with the target antigen. In the case of a monoclonal antibody this is not so. A target molecule may have only one site for the antibody and if this is shared with another protein a considerable cross reaction could be detected, even in the abscence of extensive structural homology. It has been calculated (Crawford et al., 1982 ) that a primary sequence of four amino acids (a not unusual size for an antibody binding site) might occur some 15 times in the average cell's complement of protein sequences. However, monoclonal antibody cross reactions would be expected much less frequently than this because (1) the amino acid sequence must be expressed on the surface of the molecule, (2) antibodies may recognize a sequence in a given conformation or state of modification, (3) protein sequence does not normally occur at random, and (4) antibody binding sites are often larger than this (Atassi, 1975) .
Furthermore, it would be very difficult for an animal to produce an antibody response to an epitope which was likely to cross react, merely on a random basis, with a "self" epitope. However, this could perhaps be tolerated as a small constituent of a total non-self-cross-reacting immune response. Consequently, it is likely that fortuitous cross reaction may be comparatively rare. Nevertheless, many cross reactions have been reported which are at present not understood (reviewed by Lane and Koprowski, 1982) . Several of these (Crawfordet al., 1982; Lane and Hoeffler, 1980) involve cross rections between SV40 T antigen and host proteins or between SV40 T and t antigens (Harlow et al., 1981) . In the former case there is good reason to believe that some of these antibodies recognize epitopes on functionally related host proteins, and which therefore may be constrained to some degree of overall structural similarity. Whether this represents an evolutionarily conserved structure, or one which has arisen by independent convergent evolutionary processes is unknown. However, cross reaction at the monoclonal antibody level cannot be assumed indicative of any extensive structural or functional similarity without considerable supporting evidence.
The application of monoclonal antibodies in conventional serological reactions, permitting the differentiation of closely related, serologically cross-reacting proteins has already been discussed in this review. In addition, the process described above provides an entirely new dimension to these procedures and might thus permit the identification of functionally related proteins which bear no evidence of cross reaction with polyclonal sera. Consequently application of monoclonal antibodies has not only improved information obtained from well established assay procedures, but may also lead to processes yielding an entirely new type of information.
|
Annnotations
- Denotations: 2
- Blocks: 0
- Relations: 0