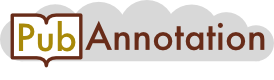
CORD-19:02a37452d5c5906b46021fbfa99a96df71704b65 / 0-169 2 Projects
What roles do growth factors play in CNS remyelination?
Abstract
The substantial advances that have been made during the last two decades on the developmental biology of the oligodendrocyte lineage have undoubtedly provided a major impetus to the study of CNS remyelination. Specific markers have been recognised that allow the identification of distinctive phenotypes within the oligodendrocyte lineage . Armed with these markers, it has been possible to elucidate the lineage relationships between these phenotypes both in vitro and during development, and to describe the migratory, proliferative and differentiation properties of each. This has led to a conceptual framework of oligodendrogliogenesis that in recent years has been applied to studies on the dynamics of the oligodendrocyte lineage in adulthood, and the need to generate replacement oligodendrocytes and new myelin sheaths following demyelination. Using stage-specific markers to identify cells associated with spontaneous remyelination, and to obtain purified population of oligodendrocyte lineage cells for transplantation into experimental models of demyelination, the long-standing debate about the origin of remyelinating oligodendrocytes is being resolved in favour of the adult oligodendrocyte progenitor (OP) (Groves et al., 1993; Warrington et al., 1993; Keirstead and Blakemore, 1997; Car-roll et al., 1998; Crang et al., 1998; Redwine and Armstrong, 1998; Levine and Reynolds, 1999) .
This chapter reviews the role that growth factors play in orchestrating the behaviour of the adult OP in remyelination, and discusses how this information may be relevant to formulating new ways of promoting remyelination in clinical disease.
Areas of complete remyelination contain more oligodendrocytes than were present in the same area of white matter before it was demyelinated. Remyelination must therefore involve the generation of new oligodendrocytes, regardless of whether previously myelinating mature oligodendrocytes that survive within an area of demyelination can generate new myelin sheaths, a view for which experimental data provides little support (Keirstead and Blakemore, 1997; Crang et al., 1998) . The weight of evidence from experimental models of remyelination indicates that OPs are the major and probably exclusive source of the new cells (Godfraind et al., 1989; Gensert and Goldman, 1997; Cenci di Bello et al., 1999; Levine and Reynolds, 1999) . A critical early event in remyelination is the recruitment of OPs, a process that involves their proliferation and migration both within and into a demyelinated area. The zone of recruitment from intact white matter around the area of demyelination appears to be very narrow , although may be greater if the demyelination is close to a germinal region such as the sub-ventricular zone (Nait-Oumesmar et al., 1999) . Once recruited, the OPs then need to engage the de-myelinated axons and differentiate into myelinating oligodendrocytes for the process of remyelination to be completed.
Thus, the key sequence of events effecting the oligodendrocyte lineage during remyelination is the proliferation and migration of OPs, and finally their d@erentiation into myelinating oligodendrocytes. Clearly, an essential component in understanding how remyelination works is the identification of the factors involved in governing these three properties of OPs. Here again, developmental studies have pointed the way, revealing a number of growth factors that effect the proliferation, migration and differentiation of neonatal OPs during myelination (reviewed in Woodruff and Franklin, 1997) . These studies have led investigators to ask whether adult OPs, the cell responsible for mediating remyelination, are similarly influenced by growth factors both in vitro, and more recently, in vivo.
Tissue culture studies on OPs isolated from the adult rodent CNS indicate that PDGF, FGF-2, GGF-2 and NT-3 are all mitogenic for these cells (Wolswijk et al., 1991; Wolswijk and Noble, 1992; Engel and Wolswijk, 1996; Shi et al., 1998) . In some instances, this effect can be synergistic, such as the combination of PDGF with either FGF-2 (Wolswijk and Noble, 1992) or GGF-2 (Shi et al., 1998) . PDGF and FGF-2 also increase the motility of OPs, and again the combination of these two growth factors has a synergistic effect. On the basis of these effects, one could imagine these factors, singly or in combination, being involved in the recruitment phase of remyelination. The ability of FGF-2 to inhibit differentiation of adult OPs is also consistent with its playing a role in OP recruitment (Gard and Pfeiffer, 1993) . What then of growth factors that induce differentiation of OPs into myelinating cells? Here there is less direct evidence from adult-derived OPs, but one can make a case based on observations using neonatal derived OPs and transgenics that both IGF-I and TGF-fll might have such an effect. During post-natal myelination, maximal levels of IGF-I immediately precede periods of active myelination (Carson et al., 1983; Rotwein et al., 1988; Bach et al., 1991) , while the phenotype of transgenic mice that over-express IGF-I also indicate a key role in myelin sheath formation (Carson et al., 1993; Ye et al., 1995) . Similarly, in vitro studies on the effects of TGF-81 on OPs suggest that it may have a role in inducing differentiation, by inhibiting mitogen-driven OP proliferation (Mckinnon et al., 1993) .
Growth factor expression during remyelination in experimental models
Having described growth factor effects on adult OPs in tissue culture, a next step is to establish whether there are changes in the expression levels of any of these growth factors in experimental models of remyelination, and whether these patterns are consistent with the predicted roles in OP recruitment and differentiation. This has been addressed using a number of different models, and using both protein and mRNA detection methods (summarised in Table 1 ). In an early study, Komoly and colleagues used in situ hybridisation and immunocytochemistry to detect changes in IGF-I expression during remyelination of cuprizone-induced demyelination of the mouse brain (Komoly et al., 1992) . The levels of IGF-I mRNA were highest at the beginning of remyelination and gradually decreased as MBP mRNA accumulated and remyelination proceeded. The IGF-I mRNA was localised to astrocytes, although the possibility that macrophages, a feature of this and other demyelinating lesions (Morel1 et al., 2000) , might also be a source was not specifically addressed. A similar association between IGF-I expression and remyelination was subsequently demonstrated using a cryogenic demyelinating lesion (Yao et al., 1995b) and in EAE (Liu et al., 1994) . In cryogenic lesions, evidence was presented that in the early stages of repair most of the IGF mRNA expression was in areas from which GFAP mRNA was absent, indicating that there are cells other than astrocytes that are a source of IGF-I in remyelinating lesions.
Another murine model has been used to demonstrate the expression of PDGF-A during remyelination (Redwine and Armstrong, 1998) . In this model, demyelination is induced by intracranial injection of mouse hepatitis virus (strain A-59), a coronavirus that causes glial cell lysis following infection. This results in widespread demyelination throughout the Komoly et al., 1992 Yao et al., 1995b Liu et al., 1994 Hinks and Franklin, 1999 Copelman et al., 2000 Redwine and Armstrong, 1998 Hinks and Franklin, 1999 Copelman et al., 2000 Liu et al., 1998 Hinks and Franklin, 1999 Copelman et al., 2000 Tourbah et al., 1992 Hinks and Franklin, 1999 Copelman et al., 2000 Copelman et al., 2000 Hinks and Franklin, 1999 Hinks and Franklin, 1999 Hinks and Franklin, 1999 Copelman et al., 2000 Copelman et al., 2000 neuraxis that does not start to remyelinate until virus is cleared at 4 weeks post-injection. Redwine and Armstrong have shown that there is an increase in the number of cells expressing PDGF-A mRNA during the early stages of remyelination when there was extensive proliferation of OPs, and that there is a corresponding increase in PDGF-A immunoreactivity during this period. The PDGF-A immunoreactivity was localised to GFAP+ astrocytes, suggesting that these cells are a primary source of PDGF-A chain-containing dimers during remyelination. A third rodent model used to study growth factor expression is that which involves the direct injection of the demyelinating chemical lysolecithin into white matter. Injection of lysolecithin creates a focal area of demyelination characterised by the presence of demyelinated axons, loss of myelin protein mRNAs and a robust macrophage response (Blakemore, 1976; Woodruff and Franklin, 1999; Ousman and David, 2000) . This is followed by a stereotypic pattern of remyelination that is determined by the species, sex and age of the animal (Blakemore, 1978; Gilson and Blakemore, 1993; Shields et al., 1999) .
In young adult rat spinal cord, new myelin sheaths do not appear in the lesion until 10 days after lesion induction, shortly after the reappearance of MBP and PLP mRNA transcripts within the area of demyelination, and by 21 days the lesion is extensively remyelinated (Woodruff and Franklin, 1999) . One can therefore divide the remyelination process into a recruitment phase when the lesion is repopulated with remyelinating cells, which gives way around day 10 to a differentiation phase when the recruited cells differentiate into myelinating oligodendrocytes. Using this model, we have examined the mRNA expression of a range of growth factors that influence the behaviour of perinatal and adult oligodendrocyte progenitors in vitro or during development. Although we could find no change in the expression levels of GGF-2, NT-3 or CNTF, there were distinctive patterns of expression of PDGF-A, FGF-2, IGF-I and TGF-PI (Hinks and Franklin, 1999) . The levels of expression of IGF-I and TGF-PI gradually rise during the recruitment phase, peak at 10 days and then gradually decline during the differentiation phase, returning to control levels at 28 days when the lesion is fully remyelinated. By contrast, both PDGF-A and FGF-2 have much lower and more restricted levels of expression than either IGF-I or TGF-l31 with different temporal profiles. Both PDGF-A and FGF-2 rapidly reach peak levels at the beginning of the recruitment phase, which in the case of PDGF-A are sustained throughout remyelination but for FGF-2 gradually decline during the differentiation phase. By comparing the growth factor mRNA expression patterns with those of astrocyte and macrophage markers, our data suggest that reactive astrocytes produce increased levels of all four mRNAs, and that the macrophages produce particularly high levels of IGF-I and TGF-bl mRNA. The significant contribution that macrophages are making to the expression of IGF-I mRNA largely determines the expression profile of this growth factor and of TGF-pl, and differs from the observation made by Komoly and co-workers using the cuprizone model, which suggested that astrocytes were the principal source of IGF-I (Komoly et al., 1992) .
What roles for growth factors can we infer from the in vitro and expression studies?
The predictions that can be made for specific growth factor roles on the basis of their in vitro effects on adult OPs are largely upheld by the observations summarised in the previous section on the expression patterns of growth factors in a range of experimental models. Although it is not always possible to separate distinct recruitment and differentiation phases, and in many situations the two events are occurring concurrently during much of the remyelination process, nevertheless, particular associations can be made. For example, PDGF and FGF-2, both of which promote proliferation and motility of adult OPs, and might therefore be expected to be involved in mediating OP recruitment, are expressed early during the repair process when expansion of OPs is occurring (Redwine and Armstrong, 1998; Hinks and Franklin, 1999) . FGF-1, whose effects on adult OPs have not been directly assessed but may have similar, albeit less potent effects to FGF-2, is also expressed during the early stages of remyelination (Tourbah et al., 1992) . IGF-I and TGF-l3 1, whose in vitro effects suggest they may be involved in the differentiation phase of remyelination, have increased levels of expression occurring at a time when differentiated remyelinating oligodendrocytes appear within the lesion (Komoly et al., 1992; Hinks and Franklin, 1999) .
Thus, one can propose a schema of remyelination in which the initial proliferation of OPs is driven by astrocyte-derived PDGF-A and FGF, and that the subsequent differentiation of the recruited OPs is triggered by the expression of astrocyte and macrophage derived IGF-I and TGF-PI (Fig. 1) . If this schema were true then one would predict that in situations where the rate of remyelination is slowed then there ought to be corresponding changes in the expression patterns of these growth factors. For example, slowing of remyelination might arise from a delayed increase in expression of the putative recruitment factors PDGF-A and FGF-2, or from a delay in the maximal expression of the putative differentiation factors IGF-I and TGF-bl, or a combination of both of these. In a recent study, we have tested this prediction by comparing the growth factor mRNA expression in rapidly remyelinating lysolecithin lesions made in young adult rats with the expression in slowly remyelinating lesions in old adult rats (Shields et al., 1999) . This study revealed delay in the onset of increased PDGF-A mRNA expression and a delay in the peak expression in the IGF-I and TGF-pl mRNA (Hinks and Franklin, 2000) . Both these results are consistent with our prediction and add support for the proposed schema.
Although most studies support the proposed schema, this is not universally the case. Copelman and colleagues have recently published data on growth factor mRNA expression in an intriguing brain aggregate tissue culture model of remyelination that are curiously at variance with the data from in vivo models of remyelination (Copelman et al., 2000) . In this model, the increase in PDGF-A mRNA, detected by RT-PCR, occurs after an increase in IGF-I and FGF-2 mRNA levels, a sequence of change that is difficult to reconcile with the proposed schema. One interpretation of this data is that while PDGF may be required for OP generation in the in vivo models of remyelination there may not be a similar requirement in the aggregate model where sufficient numbers of OPs may remain in the aggregate following demyelination. Another possibility is that PDGF is only one of a number of factors, including FGF-2 and possibly other as yet unrecog- nised factors, that are required for OP expansion and indicated that growth factor signalling is an importhat OP recruitment can proceed in its absence. tant part of efficient remyelination.
Although plausible hypotheses regarding the roles of growth factors can be devised on the basis of expression studies such as those described, the evidence that they do indeed have an important determining role comes from studies in which changes in remyelination can be induced by changing growth factor levels. Such evidence has come from studies in which growth factors have been both supplemented and inhibited. The latter has been achieved using a pharmacological antagonist, trapidil, which decreased the extent of oligodendrocyte remyelination of lysolecithin-induced demyelination in rats following systemic delivery (McKay et al., 1997; McKay et al., 1998) . Despite being widely described as a PDGF antagonist, it was not possible to infer a specific role for PDGF since other data (including our own unpublished observations) indicate that trapidil inhibits a range of other growth factors. The pharmacological approach suffers from its lack of specificity, but nevertheless, the trapidil experiments If remyelination can be inhibited by removing growth factor effects is it possible to enhance remyelination by adding growth factors? This is inherently harder to achieve for a variety of reasons including the possibility that growth factor levels are saturated even though remyelination is sub-optimal. It is also worth noting that there are very few rodent models of demyelination where remyelination is incomplete, meaning that most studies will, at best, be able to demonstrate an enhancement of the rate rather than the extent of remyelination. Even so, this approach has attracted some attention because of the possibility that growth factor-based therapies may be helpful in promoting remyelination in demyelinating diseases such as multiple sclerosis.
The first attempts to enhance remyelination using growth factors stemmed from the work on IGF-I expression in the cuprizone, cryogenic and EAE models, and involved daily intravenous or sub-cutaneous delivery of IGF-I after induction of EAE by passive transfer of MBP-reactive T-lymphocytes in Lewis rats (Yao et al., 1995a (Yao et al., , 1996 . The results of these studies showed that IGF-I was not only able to reduce the number of demyelinating foci, but also changed a number of parameters that were associated with remyelination, including the number of PLP mRNA expressing cells present within lesions. Since the IGF-I was delivered systemically and not directly into a demyelinating lesion, it was unclear whether the effects of IGF-I delivery were due to direct effects on oligodendrocyte lineage cells, or an indirect effect via a change in, for example, the inflammatory response. Subsequent studies indicated that the observed effects were probably due to the latter given the reduced damage to the blood-brain barrier and the reduction in the number of inflammatory cells within the CNS responsible for demyelination (Liu et al., 1997 (Liu et al., , 1998 . It is likely therefore that the improvement in remyelination was not a result of direct stimulation of OL cells by IGF-I, but rather that it was able to proceed in a less hostile environment.
A similar approach was used to assess the remyelination-enhancing properties of GGF-2, an adult OP mitogen. GGF-2 was delivered systemically by daily sub-cutaneous injection to mice in whom chronic relapsing EAE had been induced (Cannella et al., 1998) . Similar to the IGF-I studies in rat EAE, the GGF-2 treated animals showed clinical improvements compared to the control animals. Moreover, the increase in expression of MBP exon 2-containing mBNAs together with histopathological data, indicated improved remyelination in the treated animals. However, since the GGF-2 was delivered systemically, it remains unclear whether the observed effects of GGF-2 were as a result of direct effects on oligodendrocyte lineage cells within the lesion.
A strategy for promoting CNS remyelination by direct delivery of growth factors to the CNS has recently been reported by McTigue et al. (1998) . In this study, fibroblasts engineered to produce NT-3, BDNF, CNTF, NGF and FGF-2 were transplanted into contusion lesions in adult rat spinal cord. In the lesions that received NT-3 and BDNF supplementation, there was increased axonal regrowth and a corresponding increase in the myelination. Important though these results undoubtedly are, it is not entirely resolved whether the increase in myelination is simply a reflection of their being more 'axon' available for myelination or whether there is a direct effect of the two neurotrophins on oligodendrocyte lineage cells. The effects of NT-3 on adult OPs equivocal and difficult to reconcile with the effects described since it would appear to be mitogenic for cells derived from the brain (Shi et al., 1998) , but not for those derived from the spinal cord (Engel and Wolswijk, 1996) . There is little known about the effects of BDNF on adult oligodendrocyte lineage cells.
The feasibility of growth factor-based therapies for promoting remyelination in multiple sclerosis
The failure of remyelination, which becomes an increasingly characteristic feature of multiple sclerosis as the disease progresses, has stimulated the quest for methods by which remyelination might be promoted. Transplantation of myelinogenic cells is one approach in which much effort has been invested and one that gets ever closer to clinical implementation (Blakemore and Franklin, 2000) . An alternative approach is to try and enhance or reactivate intrinsic remyelination. The notion that growth factors may provide a means of achieving has often been discussed (e.g. McMorris and Mckinnon, 1996; Woodruff and Franklin, 1997; Wolswijk, 1998a) . Although much remains to be learnt about the precise roles of growth factors in remyelination, it is becoming clear from the existing experimental data that the process requires the sequential expression of different growth factor combinations. Moreover, the timing of this sequence critically determines the rate and efficiency of remyelination. An implication of this is that the effects of growth factor supplementation will be determined by the stage of remyelination at which they are administered and that inappropriate supplementation may actually impede remyelination. For example, if a lesion were failing to remyelinate because of a shortage of OPs, then recruitment-associated growths factors would be beneficial. On the other hand, differentiation-associated growth factors would be disadvantageous by inducing premature differentiation at the expense of the required OP expansion. Conversely, if a lesion was replete with OPs that were failing to differentiate, a situation that exists in at least some non-remyelinating MS lesions (Wolswijk, 1998a,b) , then differentiation-associated growth factors may allow remyelination to proceed to completion, while recruitment-associated growth factors would exacerbate the problem. The potential for inhibiting myelination by growth factor deliv-ery has recently been demonstrated in developmental myelination of the rat anterior medullary vellum (Goddard et al., 1999) . With current technology, it seems unlikely that it would be possible to know what growth factor strategy would be required for a particular plaque or for a particular patient without recourse to biopsy. It is also imperative to gain a deeper understanding of the effects of different growth factors on oligodendrocyte lineage cells derived from the adult human CNS, an issue for which recent advances in isolation protocols will undoubtedly help (Roy et al., 1999) . Without this information, coupled with a more detailed analysis of growth factor roles that will emerge from experimental data, the handful of studies that have described expression of a number of growth factors in MS lesions are difficult to assess (Gveric et al., 1999) .
Thus, although the concept of growth factor therapies for promoting remyelination has much to commend it, there are still substantial issues to be resolved before a rational approach based on an understanding of the mechanisms of remyelination can be devised. It is perhaps worth remembering that although the role of growth factors in regeneration of other tissue, such as skin, has progressed considerably further than our understanding of growths factors in remyelination, there are few widely used therapies for wound healing based on growth factor delivery. Nevertheless, there can be little doubt that a clearer path to achieving therapeutic remyelination will emerge from a careful dissection of the roles performed by growth factors and the other key mediators of this important repair process.
Abbreviations BDNF CNS CNTF EAE FGF GFAP GGF IGF MBP MOG NT-3 OL brain-derived neurotrophic factor central nervous system ciliary neurotrophic factor experimental allergic encephalomyelitis fibroblast growth factor glial fibrillary acidic protein glial growth factor insulin-like growth factor myelin basic protein myelin oligodendrocyte glycoprotein neurotrophin-3 oligodendrocyte lineage OP oligodendrocyte progenitor PDGF platelet-derived growth factor TGF transforming growth factor
|
Annnotations
- Denotations: 4
- Blocks: 0
- Relations: 0