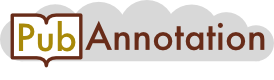
CORD-19:ded7d3ae6f8bbe7866a224433f9aac0471e758ae / 18910-18927
Diagnosis of human respiratory syncytial virus infection using reverse transcription loop-mediated isothermal amplification
Abstract
Human respiratory syncytial virus (RSV) is a major causative agent of lower respiratory tract infections in children and the elderly. A reverse transcription-loop-mediated isothermal amplification (RT-LAMP) was developed assay to amplify the genome of RSV subgroups A and B, in order to improve current diagnostic methods for RSV infection. The primer sets for RT-LAMP were designed using highly conserved nucleotide sequences in the matrix protein region of subgroups A and B, and were specific for each subgroup. The RT-LAMP efficiency was compared to virus isolation and a commercially available enzyme immunoassay (EIA) for RSV detection (BD Directigen EZ RSV test TM ), using nasopharyngeal aspirates from 59 children with respiratory tract infections. The RT-LAMP was specific for RSV and could not detect other respiratory pathogens. 61% (36/59) of children were positive by RT-LAMP, 34% (20/59) by viral isolation, and 56% (26/46) by EZ RSV. Of 16 specimens that were negative by both antigen detection and virus isolation, 12.5% (2/16) were RT-LAMP positive. These results suggest that the RT-LAMP is more sensitive than other methods used to detect RSV. The RT-LAMP assay developed in this study may be useful for diagnostic and epidemiological studies of RSV infection.
Human respiratory syncytial virus (RSV) belongs to the genus Pneumovirus in the subfamily Pneumovirinae of the family Paramyxoviridae, and is a major causative agent of respiratory tract infections in children worldwide. Children born preterm or with underlying cardiopulmonary disorders are at especially high risk of developing severe and lethal RSV respiratory tract infections (American Academy of Pediatrics, 2003; Girard et al., 2005; Saijo et al., 1993 Saijo et al., , 1994 . Elderly and immunocompromised individuals are also susceptible to severe respiratory diseases, highlighting the importance of preventing nasocomial and in-house RSV infections in hospitals and care centers for the elderly (Englund et al., 1988; Falsey and Walsh, 2000) . Palivizumab, a monoclonal antibody with neutralizing activity against RSV, was developed recently and made available commercially as an effective prophylaxis against disease (American Academy of Pediatrics, 2003) . Thus, precise diagnostic and epidemiological tests are important for the prevention of RSV infections. The diagnosis of RSV infection is generally undertaken by virus isolation and/or antigen detection using an enzyme immunoassay (EIA) (Dayan et al., 2002; Falsey et al., 2002; Reina et al., 2004) . However, because of the thermolability of RSV, the rate of isolation is dependent on conditions such as sample collection and cold chain from sample collection to cell inoculation. EIAs are useful as bed-side diagnostic tools in clinical settings, however their sensitivity and specificity are generally between 70 and 90%, suggesting that misdiagnosis of RSV infection is common (10-30%) (Grondahl et al., 2005; Ohm-Smith et al., 2004; Wren et al., 1990) . In addition, it is difficult to detect RSV antigen in nasopharyngeal aspirates collected from adults, since only a small amount of RSV antigen can be found (Casiano-Colon et al., 2003; Falsey et al., 2002; Falsey and Walsh, 2000) . The development of more sensitive and specific detection methods is therefore essential for more precise diagnoses of RSV. As a result, molecular methods such as reverse transcription (RT)-polymerase chain reaction (PCR) and quantitative real-time RT-PCR have been developed for the detection of RSV (Falsey et al., 2002 Gilbert et al., 1996) .
Loop-mediated isothermal amplification (LAMP) was developed recently to amplify specific nucleic acid sequences using a designated primer set (Nagamine et al., 2002; Notomi et al., 2000) . Amplification by LAMP is simple, since the reaction mixture is incubated at a single temperature for less than 1 h, and specificity is high because at least six specific primer region sequences are required for construction of the LAMP primer set (Nagamine et al., 2002; Notomi et al., 2000) . The amplified product level can be monitored in a real-time quantitative manner by measuring turbidity since nucleic acid amplification results in the precipitation of magnesium pyrophosphate (Mori et al., 2001) . The LAMP assay can also be used with RNA templates by combining reverse transcription reactions with LAMP (RT-LAMP) (Notomi et al., 2000) . Genetic diagnosis by RT-LAMP has been developed for infections caused by RNA viruses, such as severe acute respiratory syndrome (SARS), West Nile encephalitis, and Dengue fever (Hong et al., 2004; Parida et al., 2004 Parida et al., , 2005 . Ushio et al. (2005) recently developed RT-LAMP for detection of the RSV genome and the primers used in their method were designed according to the nucleotide sequence of the nucleoprotein region. Highly conserved sequences of the nucleotide sequence are located in the matrix protein region of RSV isolates, suggesting that this region was a suitable target for designing LAMP primers. In the present study, a RT-LAMP method was developed in which the LAMP primers were designed from the matrix protein gene, and the competence of this assay was compared to virus isolation and EIA.
59 nasopharyngeal aspirates were collected from children with respiratory tract infections from November 2004 to November 2005 at Sendai Medical Center, Sendai, Japan. Nasopharyngeal aspirates were suspended in transport medium containing Eagle's minimum essential medium (MEM, Invitrogen Corp., Carlsbad, CA, USA) supplemented with 0.5% of gelatin, and antibiotics (Numazaki et al., 1987) and stored at −20 • C prior to use. The nasopharyngeal aspirates were centrifuged at 3000 rpm for 15 min, and the supernatant was used for virus isolation. Detection of RSV antigen was analyzed in 46 of the 59 nasopharyngeal aspirates using the enzyme immunoassay (EIA), BD Directigen EZ RSV test TM (Becton, Dickinson and Company, Franklin Lakes, NJ, USA). The nasopharyngeal aspirates were also used for RNA extraction. Virus isolation was performed using six kinds of cells, MDCK, HEp-2, Vero, HMV-II, LLC-MK2, and human embryo fibroblasts, by seeding them in 96-well plate as described previously (Numazaki et al., 1987; Suzuki et al., 2005) .
The RSV strains used in this study were laboratory strains (Long and CH-18537) and wild type viruses isolated in 1996 (N96-77, N97-23, N91-730, N96-51, and N96-728) . Human metapneumovirus was isolated in the Virus Research Center at Sendai Medical Center (hMPV; 2003-2404), human coronaviruses (HCoV) strain NL63 was supplied by Dr. Hoek (University of Amsterdam, the Netherlands), HCoV strain 229E was supplied by Dr. Nomura (FUJITA Health University, Japan), SARS coronavirus strain Frankfurt was supplied by Dr. J. Ziebuhr (University of Würzburg, Germany), parainfluenza virus 3 was purchased from the American Tissue Culture Collection (ATCC) (C-245), influenza viruses strains A:H5N1, A:H3N2, A:H1N1, and B were stored in the National Institute of Infectious Diseases in Japan, and adenoviruses serotype 3, strain O.B., serotype 4, strain R163, and serotype 7, strain Gomen were stored in National Institute of Infectious Diseases in Japan. Bacterial pathogens that induce respiratory tract infections, like Bordetella pertussis (Tohama), Bordetella parapertussis (ATCC15311), Haemophils influenza biotype 3, Haemophils parainfluenza biotype 1, and Mycoplasma pneumonia (M129), were also stored in NIID. RSV was propagated in HEp-2 cells and titrated using the standard plaque assay on HEp-2 cells cultured in 12-well culture plates.
RSV strains are divided into two subgroups (A and B) based on antigenic variation (Gimenez et al., 1986; Mufson et al., 1985) . The primer sets for the RT-LAMP assay were designed from the alignment sequences of three subgroup A RSV strains (GenBank accession Nos. NC 001803, U39662, U50362) and three subgroup B RSV strains (NC 001781, AF013254, and AY353550) using the online LAMP primer design software (PrimerExplorer; http://primerexplorer.jp/index.html). The loop primer set was also designed with this software. The RSV RT-LAMP primer set described by Ushio et al. (2005) was also used for experiments.
RNA was extracted from virus stocks using TRIzol reagent (Invitrogen), according to the manufacturer's instructions. DNA was extracted from stocks of adenovirus or bacteria using QIA-GEN Genomic-tips (Qiagen, Hilden, Germany) according to the manufacturer's instructions. RNA was extracted from 140 l of each nasopharyngeal aspirate and eluted with 50 l of elute buffer using the QIAamp Viral RNA Mini Kit (Qiagen). Total RNA and genomic DNA were quantitated by measuring the OD value.
The RT-LAMP assay was performed using the Loopamp RNA amplification reagents kit (RT-LAMP; Eiken, Tokyo, Japan) as described. 5 l of each sample of extracted RNA or DNA was mixed with 40 pmol of the FIP and BIP primers, 20 pmol of the Loop-F and Loop-B primers, 5 pmol of the F3 and B3 primers, 1 l of Enzyme Mix, and 12.5 l of Reaction Mix, and distilled water was added to make a final volume of 25 l. For real-time monitoring of the RT-LAMP assay, the reaction mixture was incubated at 63 • C for 45 min in the Loopamp real-time turbidimeter Teramecs, Kyoto, Japan) . A no template negative control was included in each assay. For the purposes of this study, samples in which the magnitude of the turbidity signal was greater than 0.1 within 45 min were considered positive. Time during which the turbidity was greater than 0.1 was expressed as time of positivity in minutes. The minimum copy number of the target regions produced using the RT-LAMP assay was determined from the RT-PCR product. Target regions of the RT-LAMP assay were amplified with specific primers and Platinum Taq High Fidelity DNA polymerase (Invitrogen). The PCR products were gel-purified with a QIAquick Gel Purification Kit (Qiagen) and the concentration was determined by measuring the OD value. The DNA copy number was calculated using the following formula: copies (number/ml) = ((6.023 × l0 23 × 5 × l0 −5 × OD 260 )/molecular weight). RT-LAMP assay was performed using serially diluted target DNA, and the copy number detection limit was determined.
One-step RT-PCR was also carried out to detect RSV from clinical specimens as described. 5 l of each sample was mixed with 1 l of each primer set (sense: GARGTRGCTCCAGAATAYAGGCAT, anti-sense: AGCAT-CACTTGCCCTGAACCATAG; Osiowy, 1998) , 25.0 l of 2× AccessQuik RT-PCR Master Mix (Promega Corporation, Madison, WI, USA), and 1 l of supplemented RT enzyme, and distilled water was added to make a final volume of 50 l. The amplification program consisted of an RT step at 45 • C for 45 min, followed by a denature step at 94 • C for 2 min, and 30 cycles of 93 • C for 30 s, 55 • C for 30 s, and 72 • C for 50 s, with a final extension step of 72 • C for 7 min (Gruteke et al., 2004) . After amplification, amplicons were separated by electrophoresis on a 2% agarose gel, stained with ethidium bromide, and the bands were visualized under ultraviolet light.
RT-LAMP products were purified using the QIAquick PCR Purification Kit (Qiagen), and the purified amplicons were digested with the restriction enzyme, NlaIII or XbaI, under optimal conditions (New England Biolabs, Ipswich, MA, USA). After 2 h of digestion, RT-LAMP products were separated by electrophoresis on a 3% agarose gel, stained with ethidium bromide, and the bands were visualized under ultraviolet light. The amplicon from subgroup A RSV was digested with NlaIII, while that from subgroup B RSV was digested with XbaI.
The primer sets used in this study are shown in Table 1 . The primer set for RT-LAMP was first designed with the aim of amplifying both the A and B subgroups of RSV. This proved difficult to design, however, because the LAMP assay uses at least six specific primer regions. Thus, primer sets were designed that had specificity for subgroup A RSV (primer set A) or subgroup B RSV (primer set B). Based on alignment analysis of RSV genomic sequences, highly conserved sequences in the matrix protein coding region were selected as the target genomic sequence (Fig. 1) .
A negative control using water was analyzed by RT-LAMP for 60 min to define the value of the turbidity signal that would discriminate positives from negatives in the RT-LAMP assay. None of the negative controls had a turbidity signal that was greater than 0.1 within a 45 min reaction time. Thus, turbid- ity values exceeding 0.1 within the 45 min reaction time were defined as positive.
Specific reactions of the designed primer set were tested on various respiratory pathogens ( Table 2 ). The RT-LAMP assay was performed using 100 ng of total RNA or 1 ng of extracted genomic DNA. The RT-LAMP using primer set A and primer set B were only able to detect subgroup A RSV and subgroup B RSV, respectively. Samples prepared from other respiratory pathogens, including human coronaviruses, influenza viruses, metapneumovirus, adenoviruses, and bacteria, showed a negative reaction by RT-LAMP. Importantly, these pathogens were positive by PCR or RT-PCR when primer sets were used that were specific for each organism (data not shown). Thus, the primer set described in this report specifically reacts with RSV. Amplicons were digested with the restriction enzyme NlaIII or XbaI and separated by electrophoresis on a 3% agarose gel to confirm specific amplification and differentiation of subgroups A and B. As predicted, the RT-LAMP products amplified from subgroup A RSV were only digested with NlaIII, while the products amplified from subgroup B RSV were only digested with XbaI (data not shown).
The sensitivity of RT-LAMP was next evaluated using serially diluted RNA samples prepared from an RSV stock (Table 3 ). The infectivity of the RSV stock was determined by plaque assay using HEp-2 cells prior to RNA extraction. The entire process of preparing the samples including virus propagation, virus titration, RNA extraction, and the RT-LAMP assay, were carried out three times independently. 10 −1 to 10 −2 PFU of RSV was required to provide enough RNA for detection using the RT- LAMP assay. The detection limit of nucleic acid copy number was determined using PCR products of the target region as a template. At least 10 2 copies/5 l of the template were required for detection by RT-LAMP (data not shown). The RT-LAMP assay was carried out using a mixed sample of subgroups A and B RSV to evaluate potential interference between them. RT-LAMP was able to differentiate between RSV subgroups, and each primer set detected specifically its own target subgroup (data not shown).
RT-LAMP assay was performed to evaluate whether the primer set used in this study could detect RSV in clinical specimens using 59 nasopharyngeal aspirates from children with respiratory tract infections, and the results were compared with those obtained using virus isolation and the EIA test, BD Directigen EZ RSV test (Table 4 ). 61% (36/59) were positive by RT-LAMP, 34% (20/59) by viral isolation, 56% (26/46) by EZ RSV, and 58% (34/59) by RT-PCR. The success rate of RSV detection using the RT-LAMP assay was higher than virus isolation and the EIA test. Of the 39 RSV isolation-negative samples, 4 had parainfluenza virus, 3 had influenza virus, and RSV isolation negative (n =2 6 ) 9 1 7 1 1 1 5 EZ RSV positive (n = 1 0 ) 9 1 9 1 EZ RSV negative (n = 16) 0 16 2 14 1 had enterovirus. These other respiratory viruses isolated were RT-LAMP-negative except for one influenza virus-positive specimen that was RSV positive using the primer set described above as well as the primer set reported by Ushio et al. Comparison between the ability of EZ RSV, RSV isolation, and the RT-LAMP assay to detect RSV from clinical specimens is shown in Table 5 .
Of the 20 RSV isolation-positive specimens, 100% (20/20) were positive by RT-LAMP and 80% were positive (16/20) by EZ RSV. Of the 26 RSV isolation-negative specimens, 42% (11/26) were positive by RT-LAMP and 35% (9/26) by EZ RSV. The sensitivity of RT-PCR was similar to that of EIA. However, 12.5% (2/16) of specimens that were both antigen detection and virus isolation negative were positive by RT-LAMP.
This paper describes a highly sensitive and specific genetic diagnostic method that can detect human RSV using the RT-LAMP assay. Subgroup A and B RSV were both detected by RT-LAMP using primer sets A or B, while other respiratory pathogens were not. The assay developed in this study can detect viral RNA in 10 −1 to 10 −2 PFU of RSV. RSV was also detected in nasopharyngeal aspirates from children with respiratory tract infections, suggesting that the RSV RT-LAMP developed in the present study may be used for diagnosing RSV infections in children.
RSV infections are usually detected by virus isolation or EIAs. The virus isolation method, however, is only able to detect infectious virus. RSV is thermolabile and the quantity of infectious particles depends on sample preparation and storage conditions. Thus, isolation of RSV can be difficult and RSV infection runs the risk of being overlooked if this is the only method used. EIA kits do not require infectious viral antigens for detection and can be carried out easily in a short time (approximately 15 min), and are considered useful for general clinical diagnosis of young children (Ohm-Smith et al., 2004; Wren et al., 1990) . However, misdiagnosis can occur in 10-30% of RSV infections when the EIA kit is used alone (Grondahl et al., 2005) . RSV is not detected easily in specimens from adult patients using either virus isolation or the EIA kit, because neutralizing antibodies maintain the virus titer low (Casiano-Colon et al., 2003; Falsey et al., 2002; Falsey and Walsh, 2000) . Molecular diagnostic methods can detect viruses as long as genomic sequences exist, regardless of the integrity of the virus particle. As a result, molecular diagnostics method should be undertaken for definitive diagnosis of RSV. The LAMP assay is simpler and quicker than RT-PCR (Hong et al., 2004; Parida et al., 2004) , and results can be obtained by viewing fluorescence or determining turbidity values without opening the sample tubes, minimizing concerns about contaminating amplified products. In addition, expensive fluorescent probes and detection instruments that are required for real-time PCR, are unnecessary for the LAMP assay. Therefore, the LAMP assay is useful for qualitative detection of RSV, and RT-LAMP can be used to amplify quantitatively the virus genome in real-time (Hong et al., 2004) . The RT-LAMP assay may be useful not only for diagnostic tests but also for prognosis. In this study, nasopharyngeal aspirates were collected from children with respiratory tract infections, and RT-LAMP was able to detect the RSV genome. The RT-LAMP assay developed in this study also detected RSV genomes in samples that were negative by virus isolation and EIA, suggesting that RT-LAMP is a more sensitive assay for the detection of RSV antigens and is more useful in diagnosing RSV infections than classical methods. The RT-LAMP assay may also be used for diagnosing RSV infections in adults, although further studies are required to test this. In this study, supernatants of nasopharyngeal aspirates were used to detect RSV using the RT-LAMP assay. Since some paramyxovirus virions are cell associated (Wechsler et al., 1985) , this assay may be even more sensitive if the cells are not spun out.
Of the clinical specimens, one was RT-LAMP positive using the RSV primer sets described above and the primer set described by Ushio et al. (2005) as well as being RSV isolation negative, and EIA negative. Viral isolation was influenza virus positive, however, so it is thought that this specimen may have been coinfected with both viruses. Perhaps the RSV gene was present in such small amounts that it could only be detected using the RT-LAMP assay.
Recently, Ushio et al. (2005) developed a diagnostic method for detecting the RSV genome using RT-LAMP using the nucleoprotein region sequences as primers. However, the specificity of this method of RSV detection was only tested against three influenza virus strains. In contrast, we examined the specificity of the RT-LAMP technique against multiple respiratory pathogens, including metapneumovirus, influenza viruses, human coronaviruses, adenoviruses, and bacteria, and found that they were not amplified. When clinical specimens were tested using the RT-LAMP primer set described above and that described by Ushio et al. (2005) RSV genome detection was comparable. Thus, the sensitivity of our RT-LAMP assay described is equivalent to Ushio's method (2005) . Since RSV is a negative strand RNA virus that develops frequent mutations, two or more primer sets may be preferable for diagnosis. The RT-LAMP assay presented in this report should aid laboratory diagnosis and epidemiological surveillance of RSV infection.
of Blood and Biologics at the National Institute of Infectious Disease for providing genomic DNA of respiratory bacteria.
|
Annnotations
- Denotations: 1
- Blocks: 0
- Relations: 0