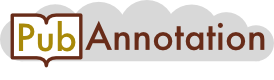
CORD-19:f1fd5f33dcbe0aae1ce2efb7b7d22eafb4247f02 / 0-147 4 Projects
State of the Art Bugs and Asthma A Different Disease?
Abstract
The prevalence of asthma has dramatically increased in recent decades. Exacerbations of asthma are a large contributor to asthma-related costs, and are primarily caused by viral and atypical bacterial infections. Rhinoviruses (RVs) are the most common viruses detected after an asthma exacerbation. RVs, respiratory syncytial virus (RSV), and human metapneumovirus (hMPV) viral infections early in life can induce wheezing and are associated with the development of asthma later in life. Atypical bacterial infections from Mycoplasma pneumoniae and Chlamydia pneumoniae have also been linked to chronic asthma and potential asthma exacerbations. In this article, we will discuss recent developments in viral infections, specifically RV, RSV, and hMPV, and atypical bacterial infections as causes of asthma exacerbations, including new data focusing on the host immune response in airway epithelial cells and animal models of infection.
Asthma is a complex disease consisting of both genetic and environmental factors that is characterized by dyspnea, coughing, wheezing, reversible episodes of bronchoconstriction, airway hyperreactivity, chronic eosinophilic inflammation, and mucus hypersecretion (1) . Each year 11 million asthma exacerbations occur in the United States, with viral infections causing the majority of these exacerbations (Table 1) (2) . Atypical bacterial infections, such as Mycoplasma pneumoniae and Chlamydia pneumoniae, have also been associated with asthma exacerbations as well as chronic asthma (Table 1) (3, 4) . In this review article we will focus on the role of viral infections; specifically rhinoviruses (RVs), respiratory syncytial virus (RSV), and human metapneumovirus (hMPV), as causes of asthma exacerbations and also how these viral infections early in life lead to wheezing and the potential development of asthma. In addition, we will review recent data suggesting that atypical bacterial infections have a pathogenic role in chronic asthma.
For many years viral infections were associated with asthma exacerbations (5) , but determining the specific virus present during the exacerbation was difficult, as serology and tissue culture infectivity techniques were not very sensitive. However, the development and use of reverse transcriptase-polymerase chain reaction (PCR) has greatly facilitated an accurate, reliable, and specific way to distinguish between respiratory viruses and also to determine virus type. Using PCR, it has been suggested that respiratory viral infections are responsible for approximately 80% of asthma exacerbations in children and 75% of exacerbations in adults (6, 7) .
Many types of viruses, such as RVs, RSV, hMPV, influenza, parainfluenza, and coronavirus, have been detected in the respiratory tract secretions from patients with asthma exacerbations. While RV infections do not present with dramatic clinical outcomes in a normal individual, it is the most common virus detected in patients with asthma exacerbations. In fact, approximately 60% of patients with asthma exacerbations have an RV infection (6) . Further, wheezing induced by RV, RSV, and hMPV infections early in childhood is associated with the development of asthma later in childhood (8) (9) (10) (11) .
RVs are positive-sense, icosahedral-shaped, nonenveloped, single-stranded RNA viruses from the Picornaviridae family of viruses. RV serotypes are categorized by many different parameters, such as receptor specificity and homology in nucleotide sequence. Discrimination based on receptor specificity results in two groups of RVs, the major and the minor group. The major group of RVs (e.g., RV14, 16, 39) comprises 90% of the serotypes and uses the intercellular adhesion molecule (ICAM)-1 as a means of entry into the cell. The minor group of RVs (e.g., RV1B, 2) uses the low-density lipoprotein family of receptors as a means of entry.
Using nucleotide sequence homology and phylogenetic analysis, three species of RVs have been described. RV serotypes were traditionally classified into two groups, RV-A and RV-B, based on the VP1 and VP4/VP2 sequences. RV-A is composed of 74 different serotypes and RV-B is composed of 25 different serotypes. Recently, however, using more sophisticated PCR techniques, a third species of RVs has been described that is a distinct RV-C species which contains at least nine serotypes (12) (13) (14) .
Numerous studies suggest that RV potentiates preexisting allergic inflammation, resulting in asthma exacerbations (4). Grunberg and colleagues showed that experimental intranasal RV16 infection in adults with mild atopic asthma caused a decrease in FEV 1 that was maximal 2 days after infection and was significantly greater compared with placebo-infected individuals with mild atopic asthma (15) . Further studies with experimental RV16 infection have shown that patients with asthma have increased levels of interleukin (IL)-8 in their nasal lavage fluid that correlates with the level of airway responsiveness (16) , in contrast to unaffected individuals in whom IL-8 does not increase (17) .
Message and colleagues showed that patients with atopic asthma experimentally infected with RV16 had increased eosinophils and increased bronchial hypersensitivity as measured by the provocative concentration of histamine required to reduce FEV 1 by 10% or 20% (18) . While the exact mechanism remains unknown, in this study patients with asthma with the highest viral load had decreased levels of IFN-g and IL-10 and increased levels of IL-4, IL-5, and IL-13 in CD41 cells from BAL fluid (18) . These data suggest that individuals with asthma, with increased levels of Th2 cytokines, may have increased viral replication and a subsequent increase in airway inflammation and decrease in lung function.
RV infections during early childhood are also associated with lower respiratory tract infections, wheezing, and the development of asthma (9, 10, 19) . RV was detected in 30% of the patients with lower respiratory tract infections, and was associated with wheezing (9, 10). Further, Miller and colleagues found that children under the age of 5 admitted to the hospital for respiratory symptoms or fever had RV-C infections in over 46% of the RV positive samples, and that patients with RV-C infections were more likely to have a diagnosis of asthma at hospital discharge compared with patients with RV-A infections (55% to 36%, respectively) (20) . However, while RV infections during the first year of life were associated with wheezing at age 3 and asthma at age 6 (10, 19), more RSV infections induced wheezing in the first year of life compared with rhinovirus infections (50% and 25%, respectively) (10). This may provide an explanation for why RVs were not previously determined to induce wheeze from infections during infancy.
RV infections are strongly associated with hospitalization for asthma. In one study, adults who had been hospitalized for asthma had respiratory tract secretions examined at the time of admission and also at a 3-month follow-up; RVs were significantly associated with hospitalization for asthma, with an adjusted odds ratio of 15.1 (21) . In this population, after adjustment for severity of asthma, subjects with RV infections were more likely to be smokers not using inhaled corticosteroids (adjusted odds ratio, 11.18; P 5 0.002) than subjects without RV infections (21) .
Green and colleagues also revealed that individuals with allergic asthma infected with RV had an increase in admission to the hospital for asthma (22) . Further, in these patients, dust mite allergen was the primary allergen detected when these subjects were skin tested to a panel of aeroallergens (22) . Therefore, to determine if RV infection during ongoing low dose of dust mite exposure resulted in an increase in asthma symptoms, de Kluijver and colleagues conducted a three-armed double-blind study with low-dose dust mite exposure and RV16 infections in patients with and without asthma (23) . Dust mite exposure alone resulted in decreased FEV 1 and an increase in eosinophils in BAL fluid. RV infection alone had decreased FEV 1 and increased production of IL-8 and neutrophils. However, the study did not show a synergistic decrease in FEV 1 or a synergistic increase in sputum neutrophils, IL-8, or neutrophil elastase with low-dose allergen exposure and RV infection in the populations with mild to moderate asthma (23) . While this study did not successfully develop an experimental model including both allergen exposure and RV infection, epidemiologic data and clinical data from patients with atopic asthma experimentally infected with RV show an association between RV infections and asthma exacerbations.
RSV is a single-stranded, enveloped, negative-sense RNA virus with a peak of infection during the winter months. RSV usually results in an upper respiratory infection, but in 30 to 70% of infants, RSV infections cause lower respiratory infection leading to pneumonia or bronchiolitis.
RSV-induced bronchiolitis is the leading cause of wheeze in infants and young children, and children infected with RSV resulting in bronchiolitis are up to four times more likely to develop wheezing and asthma later in childhood (11) . It is unknown whether RSV increases the chances of developing asthma or if people predisposed to having asthma are more likely to develop RSV-induced bronchiolitis. Nonetheless, studies have shown a correlation between severe RSV bronchiolitis and development of asthma. Sigurs and colleagues reported that 43% of infants with RSV bronchiolitis resulting in hospitalization had asthma or recurrent wheeze, with recurrent wheeze defined as three or more episodes of wheezing not verified by a physician in the prior year, at the age of 13, compared with 8% of control subjects (24) . hMPV hMPV, a recently isolated virus, is a paramyxovirus that is closely related to RSV by phylogenetic analysis and causes respiratory tract infections in both children and adults (25) . hMPV was isolated from children in 2001, and hMPV is associated with asthma exacerbations in both children and adults (25) (26) (27) (28) . Studies examining respiratory viruses in nasalwash specimens from children with respiratory tract infections showed that hMPV was detected in approximately 5 to 12% of children tested (25) (26) (27) . hMPV infection was not associated with asthma exacerbations in children greater than 5 years of age (25) , but was found to be associated with wheezing in children younger than 5 years of age (26, 27, 29) . Further, patients with a co-infection of RSV/hMPV had increased use of supplemental oxygen (26) and were associated with severe bronchiolitis (30) . In adults, Williams and colleagues detected hMPV in the nasal washings in 6.9% of patients admitted to the hospital for asthma exacerbations (27) . These studies show an association between hMPV and wheezing in children less than 5 years of age and also an association between hMPV and asthma exacerbations in adults. However, children greater than 5 years of age show no significant association between hMPV infection and asthma exacerbations (25) , and the reasons for these observed differences remain unclear.
Airway epithelial cells are polarized cells with an apical and basolateral surface that provide the first line of defense for pathogens entering the airway. RV and RSV primarily infect airway epithelial cells, activating inflammatory pathways and increasing proinflammatory cytokine and chemokine production, such as tumor necrosis factor (TNF), IL-6, CXCL8 (IL-8), CCL5 (RANTES), granulocyte macrophage colony-stimulating factor (GM-CSF), and CCL3 (MIP-1a) (31) . This increase in cytokine and chemokine production is due to specific activation of the nuclear factor (NF)-kB and the mitogen-activated protein kinase (MAPK) pathways (32, 33) . Increases in cytokine and chemokine production result in inflammation from the influx of leukocytes and provide a possible mechanism for the exacerbation of asthma. Cultured 16HBE14o-airway epithelial cells and mucociliarydifferentiated primary human bronchial epithelial cells, simultaneously exposed to the proinflammatory cytokine TNF and infected with RV39, had a synergistic increase in IL-8 production and an additive increase in the transactivation of transcription factors required for maximal CXCL-8 production, NF-kB, and activator protein-1 (34) . These data correlate with increased bronchoalveolar TNF levels and a cooperative increase with experimental RV16 infection in segmental allergen challenges of allergic patients (35) . Together, these findings suggest that airway epithelial cells may be responsible for initiating a robust or possible synergistic immune response to an RV or RSV infection in the presence of pre-existing allergic inflammation through the activation of the NF-kB and MAPK pathways and production of cytokines and chemokines.
Until very recently a nonprimate model of RV infection was not available. The main problem in developing a mouse model of RV infection was ICAM-1, the receptor for the major group of RVs, would not bind mouse ICAM-1. However, in 2008 mouse models were developed for both major and minor serotypes of RV (36, 37) . Transgenic BALB/c mice with a mouse/human ICAM-1 chimera were successfully infected with the major group virus, RV16 (36) . Further, the minor group virus, RV1B, was capable of binding the mouse low-density lipoprotein receptor and generated a successful infection in vivo (36, 37) . Infection with RV1B in wild-type mice or RV16 in the ICAM-1 chimera transgenic mice caused an increase in type I IFN production, augmented production of the chemokines CXCL2 and CCL5, an influx of inflammatory cells, and an increase in MUC5B expression in lung homogenates and/or BAL fluid (36, 37) . BALB/c mice were sensitized and challenged intranasally with the allergen ovalbumin (OVA) and infected with RV1B (RV-OVA mice). These RV-OVA mice had an increase in BAL fluid neutrophils at 1 day after infection and in lymphocytes 3 to 14 days after infection, compared with RV-infected mice or OVA-sensitized and -challenged mice receiving ultraviolet-irradiated RV1B. Airway hyperresponsiveness was also increased in RV-OVA mice 1 day after infection in response. Further, MUCAC and MUC5B were both increased in mice with RV1B infection during OVA-induced inflammation (36) .
Mouse models of RSV have elucidated some of the mechanisms by which virus causes inflammation and airway reactivity. These studies reveal that RSV strain differences are an important contributor to airway inflammation and physiologic parameters after infection. RSV strain A2 is the strain most commonly used in the laboratory, and in the absence of pre-existing allergic inflammation RSV A2 produced no lung IL-13, mucus, or airway reactivity with infection (38) . However, infection with another RSV strain, line 19, resulted in abundant production of IL-13 in the lung, airway mucus, and an increase in airway reactivity when challenged with methacholine (38) .
Other models in which RSV infection occurs in the setting of ongoing allergic inflammation have also been useful in understanding how this virus may cause asthma exacerbations in humans. For instance, RSV infection increased airway reactivity in response to methacholine challenge in the presence of ongoing allergic inflammation induced with OVA or cockroach antigen (39, 40) . Mice lacking CCR1, one of the chemokine receptors for CCL3 and CCL5, had a decrease in airway resistance and CD41 or CD81 T cell infiltration into the lung with RSV infection during cockroach antigen-induced allergic inflammation (41) . Mucus production was also increased in RSV A2 infection during ongoing allergic airway inflammation in BALB/c mice. Unexpectedly, there was a decrease in lung IL-13, while IL-17A, a cytokine produced from Th17 cells that is also involved with mucus production, was synergistically increased in the lungs of mice infected with RSV A2 during ongoing allergic inflammation (42) . Collectively, these data suggest that both the strain of RSV and the host immune response may be responsible for increases in mucus production and airway inflammation.
hMPV is able to infect BALB/c mice and has been shown to cause weight loss and ruffling of fur in infected mice similar to RSV (43). However, hMPV does not replicate well in mice, a tenfold lower viral replication compared with mice infected with RSV, and does not produce a robust inflammatory response (43) . Therefore, comparison studies between RSV and hMPV have been difficult to interpret.
Viruses have developed mechanisms to evade the immune system to establish a successful infection by down-regulating cellular transcription and the production of type I IFN. The nonstructural (NS) proteins of RSV, NS1, and NS2 have been shown to inhibit IFN-a and IFN-b production by decreasing proinflammatory transcription factors interferon regulatory factor (IRF)-3, NF-kB, and signal transducers and activators of transcription protein (STAT)-2 (4, 44, 45) . hMPV does not contain NS proteins and has detectable levels of IFN-a in BAL fluid from 6 hours to 5 days after infection, whereas RSV does not have detectable levels at 3 days after infection (46) . This prolonged IFN-a response provides a potential explanation for the small amount of viral replication and the low number of inflammatory cells in BAL fluid of hMPV-infected mice.
Recently, it was shown that cultured airway epithelial cells from patients with asthma had decreased IFN-b in response to RV16 infection compared with cultured airway epithelial cells from normal control subjects (47) . This decreased type I IFN response led to an increase in viral titer and diminished airway epithelial cell apoptosis (47) . Further, similar findings were seen in the mRNA levels of the type III interferons, IFN-l1 and IFN-l2/3 (48) . Therefore, these data combined suggest that preexisting allergic inflammation or the host immune response in patients with asthma may lead to a decreased interferon response, resulting in a more successful infection and perhaps greater symptoms.
M. pneumoniae and C. pneumoniae are atypical bacteria that attach to airway epithelial cells and cause cell damage. Similar to respiratory viruses, M. pneumoniae and C. pneumoniae were very difficult to detect with varying results from serology and culture. Using PCR has made detection more sensitive, although invasive procedures, such as an endobronchial biopsy, are still normally required for definitive diagnosis. While known to cause respiratory tract infections, the role of M. pneumoniae and C. pneumoniae in exacerbations of asthma remains controversial.
Lieberman and colleagues found that 18% of adult patients with acute asthma exacerbations had atypical bacteria infections of M. pneumoniae compared with 3% of control patients without asthma, as detected by paired sera testing using immunofluorescence or enzyme immunoassay methods (49) . In this study there was no association with C. pneumoniae and asthma exacerbations (49) . However, other studies revealed that C. pneumoniae infection was correlated with asthma exacerbations. In 2001, Martin and colleagues examined C. pneumoniae or M. pneumoniae infection in the upper or lower airway of patients with chronic asthma. Using PCR, 31 of the 55 patients with asthma (56.4%) were positive for C. pneumoniae, M. pneumoniae, or both, compared with 1 of the 11 control patients without asthma (9%) (3). M. pneumoniae was found in 25 of the patients with asthma and in only 1 control patient by PCR, while 6 patients with asthma and no control patients were PCR-positive for C. pneumoniae (3). Therefore, while C. pneumoniae is increased in patients with asthma compared with control patients; these data suggest that it may not be as prevalent as M. pneumoniae infections. More clinical studies are still needed to fully determine the link between atypical bacteria infections and asthma exacerbations. However, an animal model of OVA-induced allergic inflammation before infection with M. pneumoniae had increased collagen deposition 42 days after infection that was not seen in M. pneumoniae infection in the absence of pre-existing allergic-induced inflammation (50) .
The mechanisms describing why asthmatic airways are more susceptible to M. pneumoniae still remains largely unknown. Wu and colleagues found that Toll-like receptor (TLR)-2 expression and IL-6 production were down-regulated with M. pneumoniae infection during ongoing OVA-induced allergic inflammation (51) . Further, blocking IL-4 or IL-13 with neutralizing antibodies restored TLR2 expression and IL-6 production (51). Together, these data suggest that preexisting allergic inflammation impairs the ability to up-regulate TLR-2 and IL-6 in the lungs, leading to decreased clearance of M. pneumoniae and an increase in airway inflammation (51) . In addition, airway epithelial cells from subjects with asthma infected with mycoplasma had increased MUC5AC expression compared with epithelial cells from subjects without asthma (52) .
While there is a positive association between atypical bacteria infections and asthma exacerbations, antibiotics are generally not given in response to chronic asthma and are not recommended for asthma exacerbations (53) . Further, macrolides and ketolides that are prescribed for M. pneumoniae and C. pneumoniae infections have antiinflammatory properties, making it difficult to determine if atypical bacteria are playing a role in asthma exacerbations (54, 55) . However, Kraft and colleagues have shown that patients positive for M. pneumoniae and C. pneumoniae with chronic asthma had an increase in FEV 1 when treated with clarithromycin for 6 weeks (56). The TELICAST study by Johnston and colleagues showed that patients with asthma exacerbations that were given telithromycin, a ketolide, had an increase in symptom-free days and lung function tests conducted in the clinic compared with control subjects given placebo, but did not have an increase in lung function tests conducted at home (57) . Therefore, despite the correlation between mycoplasma infections and asthma exacerbations or chronic asthma, more research needs to be conducted to determine if atypical bacteria infections are causing the exacerbations or just another factor that persists simultaneously with, yet independent of, the exacerbation.
Although viral infections and atypical bacterial infections are associated with asthma exacerbations, not all individuals with asthma with a viral infection or atypical bacteria infection have an asthma exacerbation. Therefore, the precise mechanisms leading to these conditions are not fully understood, and more research needs to be conducted.
|
Annnotations
- Denotations: 4
- Blocks: 0
- Relations: 0