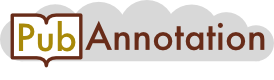
CORD-19:1319a4dbbc3d7b9001f67f9739ec378b7362ac8c / 0-121 4 Projects
Impact of rhinoviruses on pediatric community-acquired pneumonia
Abstract
This study of 592 children seen in our Emergency Department with radiographically confirmed communityacquired pneumonia (CAP) was designed to evaluate the role of rhinoviruses (RVs) in the disease. The respiratory secretions of each child were assayed using RVP Fast in order to detect 17 respiratory viruses, and the RV-positive samples were characterised by means of real-time polymerase chain reaction and sequencing. RVs were identified in 172 cases (29.0%): 48/132 children aged <1 year (36.3%), 80/293 aged 1-3 years (27.3%), and 44/167 aged ≥4 years (26.3%). Sequencing demonstrated that 82 RVs (49.1%) were group A, 17 (10.1%) group B, and 52 (31.1%) group C; 21 (12.2%) were untyped. RVs were found as single agents in 99 cases, and together with two or more other viruses in 73 (40.7%). There were only marginal differences between the different RV groups and between single RV infection and RV coinfections. RV CAP is frequent not only in younger but also in older children, and RV-A is the most common strain associated with it. The clinical relevance of RV CAP seems to be mild to moderate without any major differences between the A and B strains and the recently identified RV C.
Rhinoviruses (RVs) have been known for a long time since they were first isolated in 1956 [1, 2] . It was long thought that they were of little medical significance because they were only associated with upper respiratory tract infections, mainly the common cold. About 20 years ago, the findings of cultures and bacterial antigen detection tests in patients with lower respiratory tract infections (LRTIs) suggested that RVs may sometimes be associated with LRTIs and the induction of asthma exacerbations, particularly in patients with underlying chronic severe disease [3] [4] [5] .
The introduction of more specific and sensitive methods of respiratory viral screening, including polymerase chain reaction (PCR), made it possible to evaluate the role of RVs in LRTIs more precisely, and a number of studies using these methods have clearly shown that the association between RVs and LRTIs is significantly more frequent than previously thought, that RVs could be identified in the respiratory secretions of many infants and young children with bronchiolitis and community-acquired pneumonia (CAP), and that RVs were the agents most frequently associated with asthma exacerbations [6] [7] [8] [9] . Moreover, analysis of full-genome sequences demonstrated that, in addition to the group A (RV-A) and B RVs (RV-B) classified on the basis of the similarity of partial genetic sequences and responses to certain antiviral drugs, there is also a third group of RVs (RV-C) [10] . Together with genetic differences, these new RVs were characterised by the fact that they could not be grown in standard tissue cultures, which may explain why they were not discovered before the development of molecular diagnostics.
These methods have led to breakthroughs in our understanding of the causative role played by RVs in lower airway diseases, but most of the data so far available have come from subjects with wheezing, asthma and chronic lung diseases [11] , and little is known about other LRTIs such as pediatric CAP in otherwise healthy children. Furthermore, the role of the different groups of RVs in pediatric CAP has not been fully defined. The aim of this study was to evaluate the role of the three groups of RVs in pediatric CAP.
The study was approved by the Institutional Review Board of the Fondazione IRCCS Ca' Granda Ospedale Maggiore Policlinico, Milan, Italy, and was carried out in the Department of Maternal and Pediatric Sciences of the University of Milan between 1 November and 30 April of four consecutive winter and early spring seasons (2007-2008, 2008-2009, 2009-2010, and 2010-2011) . The written informed consent of a parent or legal guardian was required, and the older children were asked to give their assent.
All of the children aged between one month and 14 years seen in the Emergency Room of the Department of Maternal and Pediatric Sciences with fever (an axillary temperature >38°C), signs and symptoms (i.e. cough, tachypnea, dyspnea or respiratory distress, and breathing with grunting or wheezing sounds with rales) and a chest radiograph consistent with CAP were considered eligible for the study. The exclusion criteria were chronic diseases increasing the risk of respiratory infections, including premature birth; chronic disorders of the pulmonary or cardiovascular systems, including asthma; chronic metabolic diseases, including diabetes mellitus; neoplasia; kidney or liver dysfunction; hemoglobinopathies; immunosuppression; diseases requiring long-term aspirin therapy; and genetic or neurological disorders. The children with presumed nosocomial pneumonia (i.e. pneumonia appearing more than 48 hours after admission or within two weeks of hospital discharge) were also excluded.
All of the chest radiographs were evaluated by an independent expert radiologist who classified the findings as alveolar pneumonia, non-alveolar pneumonia or no pneumonia in accordance with the World Health Organisation (WHO) criteria for a standardised interpretation of pediatric chest radiographs for the diagnosis of pneumonia [12] . Alveolar pneumonia was defined as dense opacity appearing as fluffy consolidation of a part or all of a lobe, or an entire lung, often containing air on bronchography and sometimes associated with pleural effusion. Any pathologic density in the lung different from that considered alveolar was defined as interstitial pneumonia [12] .
The children for whom informed consent of parents was obtained were enrolled. Their respiratory secretions were taken using a pernasal flocked swab, and stored in a tube of UTM-RT (Kit Cat. No. 360c, Copan Italia, Brescia, Italy). Viral tests were performed for study purposes and were always available after patient's discharge. Upon enrolment, detailed information regarding their demographics, clinical history and the clinical characteristics of the disease was collected together with a blood sample for the evaluation of laboratory variables including white blood cell (WBC) counts, C-reactive protein (CRP) levels and blood cultures. Drug treatment was chosen by the pediatrician in charge on the basis of the guidelines of the Italian Society of Pediatrics. In particular, these guidelines recommend the use of antibiotics in all the cases of radiographically confirmed CAP. In mild cases, ten days of oral therapy with amoxicillin (80-90 mg/kg/day in 3 doses) for children< 5 years or with an oral macrolide (dosage according to the chosen drug; clarithromycin 15 mg/kg/day in 2 doses is the macrolide used in our hospital) for children ≥5 years are recommended. For severe cases, therapy for 10-14 days with a combination of a third generation cephalosporin (cefotaxime 100 mg/kg/day i.v. in 3 doses is the antibiotic used in our hospital) plus a macrolide (clarithromycin 7.5 mg/kg/day i.v., in 2 doses or 15 mg/kg/day orally in 2 doses) is prescribed.
The data collected during hospitalisation were recorded daily, and all of the enrolled children (whether they were hospitalised or sent home immediately after enrolment) were re-evaluated 15±2 days later by means of interviews and clinical examinations carried out by trained investigators using standardised questionnaires that also collected information regarding household illnesses and related morbidities. No additional samples were obtained at the follow-up visit.
Viral RNA or DNA was extracted immediately after collection of the respiratory secretions by means of a NuclisensEasyMAG automated extraction system (Biomeriéux, Craponne, France), and maintained in a freezer at −80°C. They were subsequently tested using the RVP Fast assay in accordance with the manufacturer's instructions (Luminex Molecular Diagnostics Inc., Toronto, Canada). All of the xTAG RVP FAST reagents were provided by Abbott GmbH & Co. (Wiesbaden-Delkenheim, Germany). The RVP Fast assay consists of a single multiplex polymerase chain reaction (PCR) with labelled primers, followed by the single-step hybridisation of the PCR products with the fluorescent bead array, and incubation with reporter reagents. The plate was analysed using a Bio-Plex 200 System (Bio-rad Laboratories, Milan, Italy) and its associated software Luminex x PONENT version 3.1 (Luminex Molecular Diagnostics Inc., provided by Abbott), and the median fluorescent intensity (MFI) was determined. An MFI above the threshold level determined by the manufacturer for a particular target indicated a positive result for that target. The mean positive fluorescence intensities were established using Tag-It Data Analysis Software (TDAS, Luminex). The RVP Fast assay simultaneously detects influenza A virus (subtyped H1 or H3), influenza B virus, respiratory syncytial virus (RSV)-A and -B, parainflunzavirus-1, -2, -3 and −4, adenovirus, human metapneumovirus (hMPV), coronaviruses 229E, NL63, OC43 and HKU1, enterovirus/rhinovirus, and human bocavirus [13, 14] . It also tests an internal positive control added to each specimen at the extraction stage (Escherichia coli phage MS2 RNA) and a positive run control added to each plate (bacteriophage lambda DNA).
The enterovirus/rhinovirus-positive samples were retested in order to identify the RVs. This RT-PCR assay was performed using the iAg-Path-ID one-step RT-PCR Kit (Applied Biosystems, Foster City, CA), and the primers and probe sequences were those reported by Lu et al. [15] . Briefly, each 25-μL reaction mixture contained 1 μM forward and reverse primers, 0.1 μM probe, and 5 μL of nucleic acid extract. The samples were amplified using a 7900 HT Fast Real-Time PCR detection system (Applied Biosystems) and the following thermocycling conditions: 10 min at 48°C for RT, 3 min at 95°C for polymerase activation, and then 45 cycles of 15 s at 95°C and 1 min at 60°C. Each run included template and non-template controls.
The hypervariable part of the 5' NCR, the entire VP4 gene, and the 5′ terminus of the VP2 gene of RVs was amplified using a RT-PCR assay as previously described [16] , with minor modifications. The eluted RNA was transcribed into cDNA using Moloney Murine Reverse Trascriptase (MMLV-RT, Invitrogen) and random hexamers for one hour at 37°C, after which the MMLV-RT was denaturated at 70°C. The PCR was carried out in a final volume of 50 μL, which contained the virus-specific oligonucleotide primers (0.2 μM each), 2 U AmpliTaq Gold 360 DNA Polymerase (Applied Biosystems), 1x reaction Buffer, 0.2 mM of each dNTP, 2 mM MgCl 2 and 2 μL of c-DNA template. The 40 PCR cycles consisted of a denaturation step (45 s at 95°C), an annealing step (45 s at 61°C), and a DNA extension step (1 min at 72°C). The 549 bp PCR products were visualised after electrophoresis on an ethidium bromide-stained 2% agarose gel. Positive and negative controls were tested in all of the reactions.
The PCR products were purified using the Wizard SV Gel and PCR Clean-Up System (Promega), and the purified products were sequenced in both directions using the same forward and reverse primers as those used in the PCR. Nucleotide sequences were obtained by means of automated DNA sequencing using an ABI PRISM 3730 genetic analyser (Applied Biosystems, Foster City, CA). When identifying the RV species, newly determined sequences were checked and aligned with the BioEdit program, and the resulting consensus sequences were compared with sequences from GenBank using the nucleotide-nucleotide BLAST algorithm (http:// www.ncbi.nlm.nih.gov). The analysed fragment was 400 nt in the VP4/VP2 region.
Descriptive statistics were generated. The continuous variables are given as mean values ± standard deviation (SD), and were analysed using a two-sided, non-parametric Wilcoxon rank-sum test or, when the data were normally distributed (on the basis of the Shapiro-Wilks statistic), a two-sided Student's t-test; the categorical variables are given as absolute numbers and percentages, and were compared between groups using contingency table analysis with the χ 2 test or Fisher's exact test as appropriate. Odds ratios (ORs) and 95% confidence intervals (CIs) were calculated to measure the association between positivity for the selected RV type and (1) the presence of high fever, (2) hospitalisation, (3) the presence of rales, (4) the presence of wheezes, (5) having a relative with a similar illness, and (6) radiological evidence of alveolar pneumonia. The ORs were obtained using unconditional multiple logistic regression, and adjusted for age (three groups: <1, 1-3 and ≥4 years) and gender. We also analysed the association between the presence of RVs together with another viral infection and the same clinical and radiological characteristics, adjusting for age, gender and type of RV. All of the analyses were made using SAS version 9.1 (Cary, NC, USA).
A total of 607 children were considered eligible for the study. Parents did not give their consent for the inclusion of their child in 15 cases so that 592 children with radiographically confirmed CAP (311 males; mean age±SD, 3.2±3.0 years) were enrolled during the four years of the study. More than 50% of them were hospitalized taking into account the severity of the disease (respiratory distress, SpO 2 and radiological findings), and for infants, even in case of mild respiratory involvement, the presence of high fever (axillary temperature ≥39°C) was considered. Information regarding clinical evolution of the disease and involvement of family members were available for all the children at follow-up. More than 97% of the children received the antibiotic therapy according to the guidelines of the Italian Society of Pediatrics. Among enrolled patients, 435 (73.5%) were positive for at least one virus. RVs were identified in 172 cases (29.0%) and were the second most common infectious agents after respiratory syncytial virus (RSV), which was found in 188 children (31.7%). A preliminary analysis of the prevalence of RVs and the RV A, B and C subgroups showed that there were no statistically significant differences by year of sample collection; consequently, all of the data collected in the four years were considered together. Table 1 Table 2 shows the RV co-infections. Dual infections were observed in 60 cases (10.1%), triple infections in 11 (1.9%), and quadruple infections in two (0.3%). The most common dual infection was RV + RSV (35 cases) followed by RV + bocavirus (12 cases). RSV was also found in six cases with triple infection and both cases of quadruple infection. The only RV-infected child who had a bacteriapositive blood culture (Streptoccoccus pneumoniae) was co-infected with RV-A and RSV. Table 3 shows the characteristics of the CAP associated with single RV infections by RV group. The differences between the groups were generally marginal. All of the considered demographic and clinical variables were similarly distributed in the three groups with the exception of highgrade fever, which was significantly less frequent in the children infected by RV-C than in those infected with RV-A (p<0.001). Hospitalisation rates and duration were also similar in the three groups, as was drug use. The data regarding the social impact of RV infection showed that, although the rates of absence from the community of the infected child were similar in the three groups, the number of similar illnesses within the family was significantly lower in the children infected with RV-C than in those infected by RV-A (p=0.02). Analysis of the laboratory data showed that neutrophil cell counts were significantly higher in the children infected with RV-C than those infected with RV-A (p=0.02), whereas monocyte counts and CRP levels were significantly lower in the children infected with RV-C than in those infected with RV-A (p=0.04 and p=0.01, respectively). There was no difference in the radiographic characteristics of the three groups. Multivariate analysis confirmed the similarity of the RV groups: the only differences between them related to the RV-C positive cases, which were significantly less frequently associated with high fever (OR 0.13, 95% CI 0.04-0.40) or a similar illness within the family (OR 0.16, 95% CI 0.03-0.82) than the RV-A positive cases. Table 4 shows the demographic, clinical, laboratory and radiographic variables associated with single RV infections and co-infections. The only significant differences were in mean age (the children with a single RV infection were older; p=0.0002), and neutrophil and lymphocyte counts, which were, respectively, significantly lower (p=0.001) and significantly higher (p<0.001) in the children with coinfections. Multivariate analysis showed that the only differences between the groups were related to RV-B positive co-infections, which were significantly less frequently associated with high-grade fever (OR 0.08, 95% CI 0.01-0.82) than RV-A co-infections.
Our data confirm the findings of epidemiological studies of RV infections using molecular diagnostics, i.e. the high frequency of the association between RVs and pediatric CAP [17] . However, given the very large number of infectious agents sought by us, they add significant information concerning the potential causative role of RVs. Using molecular methods capable of identifying 17 different respiratory viruses, we found RVs in more than 28% of our patients with radiographically confirmed pediatric CAP, second only to RSV. The presence of a virus in the nasopharynx of a child with CAP does not necessarily mean that it is the etiological agent because it may only indicate a coincidental upper airways infection, or be due to a carrier state or the prolonged shedding of a pathogen that caused a previous infection. This may be particularly important in the case of RVs because a number of epidemiological studies have shown that they can be found in the respiratory secretions of 12-22% of asymptomatic subjects [18] [19] [20] [21] . We looked for most of the known respiratory viruses with methods able to give reliable results in a short time. However, because all the viral samples were evaluated some days after respiratory specimens' collection, we did not ascertain the impact that the availability of the information due to this method could have had on physician's behavior, particularly as far as antibiotic prescriptions are concerned. This can explain why in almost all our patients antibiotic therapy was initiated and maintained for the entire period recommended by Italian pediatric guidelines for the treatment of CAP. Further studies that evaluate the impact on antimicrobial prescribing of viral findings with methods able to identify multiple viruses should be performed. In our study population, we found RVs as a single agent in about 60% of the children in which RVs were identified. This strongly suggests that RVs were the cause of the CAP diagnosed in many of our study patients, particularly in those in whom very low values of WBC counts and CRP were found. However, it cannot be excluded that in some of these cases RVs could have been associated with bacteria as recently found by Honkinen et al. [22] . Unfortunately, definition of bacterial etiology of lower respiratory tract infections is very difficult, particularly in younger children because the poor co-operation of the patient does not easily permit the collection of the secretions coming from the lower respiratory tract. Moreover, microbiological evaluation of the sputum, as performed by Honkinen et al. [22] , does not necessarily lead to the identification of the true etiology because bacteria might be contaminants from the nasopharynx and are frequently carried by young children. On the other hand, blood culture, that was performed in this study in all the enrolled children, or molecular methods specifically devoted to the identification of bacterial pathogens in blood samples can give only a partial contribution to the solution of the problem because bacteremic CAPs are only the smallest part of the total number of CAPs diagnosed in children.
The incidence of CAP associated with RV infection was a little higher in the children aged <1 year than in the other age groups, but the prevalence of cases due to RV alone was highest among the older children: more than one-third of these cases was found in the patients aged ≥4 years. This is in line with the finding of Miller et al. [23] who found that CAP is the most frequent cause of RV-associated hospitalisations in children aged 24-50 months, whereas other diseases are more frequent causes in younger patients, and this seems to suggest that, unlike RSV (whose importance is strictly age-related) [24] , RVs are also a frequent cause of CAP in older children. The role of RVs as causes of CAP in older children is further supported by the frequency of RVassociated LRTIs in children aged 1-15 years, including pneumonia severe enough to require mechanical ventilation [25] .
In our study population, the most frequently detected RVs associated with pediatric CAP were in the RV-A group, followed by RV-C and RV-B pathogens. In this regard, our data are similar to those of Xiang et al. [26] , but are different from those of Calvo et al. [27] and Linsuwanon et al. [28] , who studied patients with LRTIs and found that RV-B pathogens were predominant when CAP was diagnosed. However, these studies enrolled a and included patients whose diagnosis of CAP was not confirmed by chest radiography [28] . We only enrolled children whose radiographic findings were consistent with CAP, and the study was carried out in the winter and early spring. It is also worth noting that the circulation of RV strains can significantly differ from place to place and from month to month [3] , and that their importance in causing LRTIs other than CAP may vary [29] . Consequently, it is possible that some studies enrolled children but with an LRTI other than CAP, and that the final prevalence rates may have been affected by the different circulation of the various RVs during the year and/or in different places [27, 28] .
Regardless of the type of RV involved in causing the disease, the clinical and laboratory characteristics of CAP were only slightly different in the children with single RV infection. Most of the cases were relatively mild as only CRP C-reactive protein, RV rhinovirus, SD standard deviation, SpO 2 peripheral oxygen saturation a 38.0°C or more any time during the illness (before or upon enrolment, or during follow-up) b 39.0°C or more any time during the illness (before or upon enrolment, or during follow-up) *p<0.05 vs RV-C. No other significant between-group differences two-thirds of the children were hospitalised and none of them needed admission to an intensive care unit. In terms of the clinical role of the different types of RV, our findings are similar to those of by Xiang et al. [26] , but once again, different from those of Linsuwanon et al. [28] , who found a significantly higher incidence of severe CAP in children infected by RV-B. However, as said above, this difference may be explained by the possible inclusion of cases with a diagnosis other than CAP. Interestingly, the chest radiographs of a considerable number of the children with CAP and RV infection (regardless of the type of RV) suggested alveolar involvement, a finding that is usually considered indicative of bacterial etiology. However, none of our children with RV infection alone had a blood culture suggesting a concomitant bacterial infection. The fact that some of the patients had received antibiotics before they were enrolled would have limited the possibility of identifying bacteria, but it is reasonable to think that the alveolar CAP was directly due to the RV infection in a large number of cases. On the other hand, alveolar involvement has been previously found in patients with LRTIs due to other viruses such as human metapneumovirus [30] .
In terms of the social impact of RV infection, our analyses showed that RV-C was associated with a significantly lower incidence of similar illnesses within the family. This suggests that RV-C pathogens are less widespread in the community but it is difficult to explain as there are no published data concerning possible differences in the duration of shedding of the various RV strains. Further studies should clarify whether ours is a chance finding or due to a difference in the shedding of RV-C strains.
The clinical importance of viral co-infections is questioned because the data regarding the severity of single and multiple infections are conflicting. It has been found that RVs have no illness-promoting effect in the case of coinfections with RSV or influenza viruses [11] , but it has also been demonstrated that RV and adenovirus coinfections can cause severely obstructive LRTIs [31] . There are few data concerning the possible additive effect of multiple viral infections including RVs in children with CAP. Most of the co-infections observed during our study involved RSV, which may explain why we found only marginal clinical differences between single and multiple infections. The high incidence of co-infections with RSV, a virus that mainly causes lower respiratory tract involvement (including bronchiolitis) in the first months of life, also reasonably explains why the co-infected children had a younger mean age than those with single infections. However, the lack of any significant difference in almost all of the clinical and laboratory findings between the children with single RV infections of all types and RV coinfections confirms that all of the RV strains played a similar clinical role in determining CAP.
This study has three limitations. First of all, it covered only some months of a single year and the activity of respiratory viruses can significantly vary from season to season. Second, it was confined to a single centre and so the cohort of enrolled children may not reflect nationwide RV infection. Third, it only enrolled children attending a hospital and, as it is highly likely that hospital assistance was not sought for some children with milder CAP, our data may not correctly estimate the true importance of RV as a potential cause of pediatric CAP. However, despite these limitations, we believe that our findings extend current knowledge of the clinical importance of RVs as causes of CAP in children. RV-associated CAP seems to be common not only in the first years of life but also in older children, and RV-A is the strain that is most frequently associated with the disease. The clinical relevance of RV-associated CAP seems to be mild-moderate, without any substantial differences between the old A and B strains and the recently identified RV-C. Co-infections with other viruses do not seem to increase the severity of RV-associated CAP. All of these data are important for the management of pediatric CAP, the identification of the pathogens that require further study, and the development of safe and effective vaccines.
|
Annnotations
- Denotations: 2
- Blocks: 0
- Relations: 0