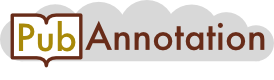
PMC:7299399 / 11390-49391
Annnotations
LitCovid-PD-FMA-UBERON
{"project":"LitCovid-PD-FMA-UBERON","denotations":[{"id":"T46","span":{"begin":95,"end":112},"obj":"Body_part"},{"id":"T47","span":{"begin":129,"end":133},"obj":"Body_part"},{"id":"T48","span":{"begin":190,"end":193},"obj":"Body_part"},{"id":"T49","span":{"begin":195,"end":201},"obj":"Body_part"},{"id":"T50","span":{"begin":319,"end":326},"obj":"Body_part"},{"id":"T51","span":{"begin":393,"end":397},"obj":"Body_part"},{"id":"T52","span":{"begin":430,"end":435},"obj":"Body_part"},{"id":"T53","span":{"begin":526,"end":530},"obj":"Body_part"},{"id":"T54","span":{"begin":564,"end":569},"obj":"Body_part"},{"id":"T55","span":{"begin":630,"end":638},"obj":"Body_part"},{"id":"T56","span":{"begin":658,"end":663},"obj":"Body_part"},{"id":"T57","span":{"begin":758,"end":763},"obj":"Body_part"},{"id":"T58","span":{"begin":836,"end":840},"obj":"Body_part"},{"id":"T59","span":{"begin":857,"end":861},"obj":"Body_part"},{"id":"T60","span":{"begin":975,"end":979},"obj":"Body_part"},{"id":"T61","span":{"begin":1212,"end":1220},"obj":"Body_part"},{"id":"T62","span":{"begin":2058,"end":2087},"obj":"Body_part"},{"id":"T63","span":{"begin":2058,"end":2073},"obj":"Body_part"},{"id":"T64","span":{"begin":2089,"end":2093},"obj":"Body_part"},{"id":"T65","span":{"begin":2126,"end":2130},"obj":"Body_part"},{"id":"T66","span":{"begin":2225,"end":2239},"obj":"Body_part"},{"id":"T67","span":{"begin":2225,"end":2229},"obj":"Body_part"},{"id":"T68","span":{"begin":3067,"end":3074},"obj":"Body_part"},{"id":"T69","span":{"begin":5037,"end":5050},"obj":"Body_part"},{"id":"T70","span":{"begin":5484,"end":5492},"obj":"Body_part"},{"id":"T71","span":{"begin":5558,"end":5561},"obj":"Body_part"},{"id":"T72","span":{"begin":5566,"end":5569},"obj":"Body_part"},{"id":"T73","span":{"begin":5645,"end":5653},"obj":"Body_part"},{"id":"T74","span":{"begin":5684,"end":5691},"obj":"Body_part"},{"id":"T75","span":{"begin":5781,"end":5788},"obj":"Body_part"},{"id":"T76","span":{"begin":5860,"end":5867},"obj":"Body_part"},{"id":"T77","span":{"begin":5962,"end":5969},"obj":"Body_part"},{"id":"T78","span":{"begin":5974,"end":5977},"obj":"Body_part"},{"id":"T79","span":{"begin":6221,"end":6226},"obj":"Body_part"},{"id":"T80","span":{"begin":6334,"end":6342},"obj":"Body_part"},{"id":"T81","span":{"begin":6383,"end":6390},"obj":"Body_part"},{"id":"T82","span":{"begin":6690,"end":6695},"obj":"Body_part"},{"id":"T83","span":{"begin":6700,"end":6705},"obj":"Body_part"},{"id":"T84","span":{"begin":6795,"end":6803},"obj":"Body_part"},{"id":"T85","span":{"begin":7498,"end":7502},"obj":"Body_part"},{"id":"T86","span":{"begin":7532,"end":7540},"obj":"Body_part"},{"id":"T87","span":{"begin":7559,"end":7566},"obj":"Body_part"},{"id":"T88","span":{"begin":7820,"end":7825},"obj":"Body_part"},{"id":"T89","span":{"begin":8146,"end":8150},"obj":"Body_part"},{"id":"T90","span":{"begin":8160,"end":8164},"obj":"Body_part"},{"id":"T91","span":{"begin":8187,"end":8192},"obj":"Body_part"},{"id":"T92","span":{"begin":8606,"end":8609},"obj":"Body_part"},{"id":"T93","span":{"begin":9168,"end":9175},"obj":"Body_part"},{"id":"T94","span":{"begin":9556,"end":9561},"obj":"Body_part"},{"id":"T95","span":{"begin":9625,"end":9629},"obj":"Body_part"},{"id":"T96","span":{"begin":9940,"end":9947},"obj":"Body_part"},{"id":"T97","span":{"begin":10079,"end":10082},"obj":"Body_part"},{"id":"T98","span":{"begin":10087,"end":10091},"obj":"Body_part"},{"id":"T99","span":{"begin":10309,"end":10313},"obj":"Body_part"},{"id":"T100","span":{"begin":10511,"end":10515},"obj":"Body_part"},{"id":"T101","span":{"begin":10530,"end":10538},"obj":"Body_part"},{"id":"T102","span":{"begin":10926,"end":10933},"obj":"Body_part"},{"id":"T103","span":{"begin":10969,"end":10976},"obj":"Body_part"},{"id":"T104","span":{"begin":12024,"end":12027},"obj":"Body_part"},{"id":"T105","span":{"begin":12283,"end":12290},"obj":"Body_part"},{"id":"T106","span":{"begin":12794,"end":12801},"obj":"Body_part"},{"id":"T107","span":{"begin":13870,"end":13875},"obj":"Body_part"},{"id":"T108","span":{"begin":14350,"end":14363},"obj":"Body_part"},{"id":"T109","span":{"begin":14803,"end":14816},"obj":"Body_part"},{"id":"T110","span":{"begin":14986,"end":14995},"obj":"Body_part"},{"id":"T111","span":{"begin":15002,"end":15007},"obj":"Body_part"},{"id":"T112","span":{"begin":15042,"end":15046},"obj":"Body_part"},{"id":"T113","span":{"begin":15069,"end":15079},"obj":"Body_part"},{"id":"T114","span":{"begin":16116,"end":16123},"obj":"Body_part"},{"id":"T115","span":{"begin":16177,"end":16189},"obj":"Body_part"},{"id":"T116","span":{"begin":17065,"end":17069},"obj":"Body_part"},{"id":"T117","span":{"begin":17065,"end":17067},"obj":"Body_part"},{"id":"T118","span":{"begin":17074,"end":17085},"obj":"Body_part"},{"id":"T119","span":{"begin":17183,"end":17191},"obj":"Body_part"},{"id":"T120","span":{"begin":17195,"end":17206},"obj":"Body_part"},{"id":"T121","span":{"begin":18142,"end":18150},"obj":"Body_part"},{"id":"T122","span":{"begin":18218,"end":18226},"obj":"Body_part"},{"id":"T123","span":{"begin":18240,"end":18244},"obj":"Body_part"},{"id":"T124","span":{"begin":18260,"end":18268},"obj":"Body_part"},{"id":"T125","span":{"begin":18546,"end":18551},"obj":"Body_part"},{"id":"T126","span":{"begin":18653,"end":18664},"obj":"Body_part"},{"id":"T127","span":{"begin":18666,"end":18668},"obj":"Body_part"},{"id":"T128","span":{"begin":18806,"end":18808},"obj":"Body_part"},{"id":"T129","span":{"begin":18833,"end":18835},"obj":"Body_part"},{"id":"T130","span":{"begin":18847,"end":18855},"obj":"Body_part"},{"id":"T131","span":{"begin":18875,"end":18877},"obj":"Body_part"},{"id":"T132","span":{"begin":18926,"end":18934},"obj":"Body_part"},{"id":"T133","span":{"begin":19128,"end":19132},"obj":"Body_part"},{"id":"T134","span":{"begin":19205,"end":19213},"obj":"Body_part"},{"id":"T135","span":{"begin":19382,"end":19390},"obj":"Body_part"},{"id":"T136","span":{"begin":19501,"end":19506},"obj":"Body_part"},{"id":"T137","span":{"begin":19580,"end":19587},"obj":"Body_part"},{"id":"T138","span":{"begin":19688,"end":19701},"obj":"Body_part"},{"id":"T139","span":{"begin":20054,"end":20065},"obj":"Body_part"},{"id":"T140","span":{"begin":20123,"end":20134},"obj":"Body_part"},{"id":"T141","span":{"begin":20215,"end":20217},"obj":"Body_part"},{"id":"T142","span":{"begin":20245,"end":20251},"obj":"Body_part"},{"id":"T143","span":{"begin":20256,"end":20267},"obj":"Body_part"},{"id":"T144","span":{"begin":20256,"end":20261},"obj":"Body_part"},{"id":"T145","span":{"begin":20336,"end":20347},"obj":"Body_part"},{"id":"T146","span":{"begin":20336,"end":20341},"obj":"Body_part"},{"id":"T147","span":{"begin":20352,"end":20358},"obj":"Body_part"},{"id":"T148","span":{"begin":20359,"end":20365},"obj":"Body_part"},{"id":"T149","span":{"begin":20431,"end":20442},"obj":"Body_part"},{"id":"T150","span":{"begin":20458,"end":20465},"obj":"Body_part"},{"id":"T151","span":{"begin":20564,"end":20575},"obj":"Body_part"},{"id":"T152","span":{"begin":20642,"end":20652},"obj":"Body_part"},{"id":"T153","span":{"begin":20775,"end":20783},"obj":"Body_part"},{"id":"T154","span":{"begin":20812,"end":20822},"obj":"Body_part"},{"id":"T155","span":{"begin":20827,"end":20835},"obj":"Body_part"},{"id":"T156","span":{"begin":20850,"end":20854},"obj":"Body_part"},{"id":"T157","span":{"begin":20855,"end":20866},"obj":"Body_part"},{"id":"T158","span":{"begin":21179,"end":21183},"obj":"Body_part"},{"id":"T159","span":{"begin":21342,"end":21353},"obj":"Body_part"},{"id":"T160","span":{"begin":21426,"end":21436},"obj":"Body_part"},{"id":"T161","span":{"begin":21714,"end":21727},"obj":"Body_part"},{"id":"T162","span":{"begin":22149,"end":22153},"obj":"Body_part"},{"id":"T163","span":{"begin":22276,"end":22285},"obj":"Body_part"},{"id":"T164","span":{"begin":22408,"end":22417},"obj":"Body_part"},{"id":"T165","span":{"begin":22423,"end":22428},"obj":"Body_part"},{"id":"T166","span":{"begin":22568,"end":22573},"obj":"Body_part"},{"id":"T167","span":{"begin":22677,"end":22686},"obj":"Body_part"},{"id":"T168","span":{"begin":22698,"end":22700},"obj":"Body_part"},{"id":"T169","span":{"begin":22847,"end":22856},"obj":"Body_part"},{"id":"T170","span":{"begin":22862,"end":22867},"obj":"Body_part"},{"id":"T171","span":{"begin":23082,"end":23091},"obj":"Body_part"},{"id":"T172","span":{"begin":23252,"end":23262},"obj":"Body_part"},{"id":"T173","span":{"begin":23513,"end":23518},"obj":"Body_part"},{"id":"T174","span":{"begin":23778,"end":23782},"obj":"Body_part"},{"id":"T175","span":{"begin":24017,"end":24025},"obj":"Body_part"},{"id":"T176","span":{"begin":24356,"end":24362},"obj":"Body_part"},{"id":"T177","span":{"begin":26135,"end":26138},"obj":"Body_part"},{"id":"T178","span":{"begin":27013,"end":27017},"obj":"Body_part"},{"id":"T179","span":{"begin":27067,"end":27074},"obj":"Body_part"},{"id":"T180","span":{"begin":27211,"end":27215},"obj":"Body_part"},{"id":"T181","span":{"begin":27335,"end":27344},"obj":"Body_part"},{"id":"T182","span":{"begin":27454,"end":27458},"obj":"Body_part"},{"id":"T183","span":{"begin":27567,"end":27571},"obj":"Body_part"},{"id":"T184","span":{"begin":27583,"end":27589},"obj":"Body_part"},{"id":"T185","span":{"begin":27593,"end":27604},"obj":"Body_part"},{"id":"T186","span":{"begin":27776,"end":27783},"obj":"Body_part"},{"id":"T187","span":{"begin":27908,"end":27916},"obj":"Body_part"},{"id":"T188","span":{"begin":27995,"end":27999},"obj":"Body_part"},{"id":"T189","span":{"begin":29192,"end":29196},"obj":"Body_part"},{"id":"T190","span":{"begin":29331,"end":29335},"obj":"Body_part"},{"id":"T191","span":{"begin":29345,"end":29350},"obj":"Body_part"},{"id":"T192","span":{"begin":29708,"end":29716},"obj":"Body_part"},{"id":"T193","span":{"begin":29786,"end":29790},"obj":"Body_part"},{"id":"T194","span":{"begin":30445,"end":30449},"obj":"Body_part"},{"id":"T195","span":{"begin":30856,"end":30860},"obj":"Body_part"},{"id":"T196","span":{"begin":31506,"end":31514},"obj":"Body_part"},{"id":"T197","span":{"begin":31928,"end":31933},"obj":"Body_part"},{"id":"T198","span":{"begin":32146,"end":32150},"obj":"Body_part"},{"id":"T199","span":{"begin":32550,"end":32558},"obj":"Body_part"},{"id":"T200","span":{"begin":32581,"end":32586},"obj":"Body_part"},{"id":"T201","span":{"begin":32628,"end":32638},"obj":"Body_part"},{"id":"T202","span":{"begin":32671,"end":32689},"obj":"Body_part"},{"id":"T203","span":{"begin":32691,"end":32695},"obj":"Body_part"},{"id":"T204","span":{"begin":32700,"end":32715},"obj":"Body_part"},{"id":"T205","span":{"begin":32717,"end":32719},"obj":"Body_part"},{"id":"T206","span":{"begin":33031,"end":33035},"obj":"Body_part"},{"id":"T207","span":{"begin":33148,"end":33160},"obj":"Body_part"},{"id":"T208","span":{"begin":33148,"end":33152},"obj":"Body_part"},{"id":"T209","span":{"begin":33170,"end":33185},"obj":"Body_part"},{"id":"T210","span":{"begin":33311,"end":33315},"obj":"Body_part"},{"id":"T211","span":{"begin":33489,"end":33494},"obj":"Body_part"},{"id":"T212","span":{"begin":33750,"end":33755},"obj":"Body_part"},{"id":"T213","span":{"begin":33805,"end":33812},"obj":"Body_part"},{"id":"T214","span":{"begin":33845,"end":33858},"obj":"Body_part"},{"id":"T215","span":{"begin":33845,"end":33849},"obj":"Body_part"},{"id":"T216","span":{"begin":34018,"end":34020},"obj":"Body_part"},{"id":"T217","span":{"begin":34125,"end":34129},"obj":"Body_part"},{"id":"T218","span":{"begin":34357,"end":34364},"obj":"Body_part"},{"id":"T219","span":{"begin":34650,"end":34657},"obj":"Body_part"},{"id":"T220","span":{"begin":36561,"end":36569},"obj":"Body_part"},{"id":"T221","span":{"begin":36591,"end":36596},"obj":"Body_part"},{"id":"T222","span":{"begin":37032,"end":37037},"obj":"Body_part"},{"id":"T223","span":{"begin":37047,"end":37052},"obj":"Body_part"},{"id":"T224","span":{"begin":37412,"end":37427},"obj":"Body_part"},{"id":"T225","span":{"begin":37496,"end":37501},"obj":"Body_part"}],"attributes":[{"id":"A46","pred":"fma_id","subj":"T46","obj":"http://purl.org/sig/ont/fma/fma265130"},{"id":"A47","pred":"fma_id","subj":"T47","obj":"http://purl.org/sig/ont/fma/fma7195"},{"id":"A48","pred":"fma_id","subj":"T48","obj":"http://purl.org/sig/ont/fma/fma7199"},{"id":"A49","pred":"fma_id","subj":"T49","obj":"http://purl.org/sig/ont/fma/fma7203"},{"id":"A50","pred":"fma_id","subj":"T50","obj":"http://purl.org/sig/ont/fma/fma9637"},{"id":"A51","pred":"fma_id","subj":"T51","obj":"http://purl.org/sig/ont/fma/fma7195"},{"id":"A52","pred":"fma_id","subj":"T52","obj":"http://purl.org/sig/ont/fma/fma67498"},{"id":"A53","pred":"fma_id","subj":"T53","obj":"http://purl.org/sig/ont/fma/fma7195"},{"id":"A54","pred":"fma_id","subj":"T54","obj":"http://purl.org/sig/ont/fma/fma68646"},{"id":"A55","pred":"fma_id","subj":"T55","obj":"http://purl.org/sig/ont/fma/fma264783"},{"id":"A56","pred":"fma_id","subj":"T56","obj":"http://purl.org/sig/ont/fma/fma68646"},{"id":"A57","pred":"fma_id","subj":"T57","obj":"http://purl.org/sig/ont/fma/fma68646"},{"id":"A58","pred":"fma_id","subj":"T58","obj":"http://purl.org/sig/ont/fma/fma7195"},{"id":"A59","pred":"fma_id","subj":"T59","obj":"http://purl.org/sig/ont/fma/fma7195"},{"id":"A60","pred":"fma_id","subj":"T60","obj":"http://purl.org/sig/ont/fma/fma7195"},{"id":"A61","pred":"fma_id","subj":"T61","obj":"http://purl.org/sig/ont/fma/fma67257"},{"id":"A62","pred":"fma_id","subj":"T62","obj":"http://purl.org/sig/ont/fma/fma82834"},{"id":"A63","pred":"fma_id","subj":"T63","obj":"http://purl.org/sig/ont/fma/fma63023"},{"id":"A64","pred":"fma_id","subj":"T64","obj":"http://purl.org/sig/ont/fma/fma82834"},{"id":"A65","pred":"fma_id","subj":"T65","obj":"http://purl.org/sig/ont/fma/fma82834"},{"id":"A66","pred":"fma_id","subj":"T66","obj":"http://purl.org/sig/ont/fma/fma63841"},{"id":"A67","pred":"fma_id","subj":"T67","obj":"http://purl.org/sig/ont/fma/fma68646"},{"id":"A68","pred":"fma_id","subj":"T68","obj":"http://purl.org/sig/ont/fma/fma67257"},{"id":"A69","pred":"fma_id","subj":"T69","obj":"http://purl.org/sig/ont/fma/fma9825"},{"id":"A70","pred":"fma_id","subj":"T70","obj":"http://purl.org/sig/ont/fma/fma67257"},{"id":"A71","pred":"fma_id","subj":"T71","obj":"http://purl.org/sig/ont/fma/fma67095"},{"id":"A72","pred":"fma_id","subj":"T72","obj":"http://purl.org/sig/ont/fma/fma74412"},{"id":"A73","pred":"fma_id","subj":"T73","obj":"http://purl.org/sig/ont/fma/fma67257"},{"id":"A74","pred":"fma_id","subj":"T74","obj":"http://purl.org/sig/ont/fma/fma67257"},{"id":"A75","pred":"fma_id","subj":"T75","obj":"http://purl.org/sig/ont/fma/fma67257"},{"id":"A76","pred":"fma_id","subj":"T76","obj":"http://purl.org/sig/ont/fma/fma67257"},{"id":"A77","pred":"fma_id","subj":"T77","obj":"http://purl.org/sig/ont/fma/fma67257"},{"id":"A78","pred":"fma_id","subj":"T78","obj":"http://purl.org/sig/ont/fma/fma67095"},{"id":"A79","pred":"fma_id","subj":"T79","obj":"http://purl.org/sig/ont/fma/fma67264"},{"id":"A80","pred":"fma_id","subj":"T80","obj":"http://purl.org/sig/ont/fma/fma67257"},{"id":"A81","pred":"fma_id","subj":"T81","obj":"http://purl.org/sig/ont/fma/fma67257"},{"id":"A82","pred":"fma_id","subj":"T82","obj":"http://purl.org/sig/ont/fma/fma67264"},{"id":"A83","pred":"fma_id","subj":"T83","obj":"http://purl.org/sig/ont/fma/fma67264"},{"id":"A84","pred":"fma_id","subj":"T84","obj":"http://purl.org/sig/ont/fma/fma67257"},{"id":"A85","pred":"fma_id","subj":"T85","obj":"http://purl.org/sig/ont/fma/fma67122"},{"id":"A86","pred":"fma_id","subj":"T86","obj":"http://purl.org/sig/ont/fma/fma67257"},{"id":"A87","pred":"fma_id","subj":"T87","obj":"http://purl.org/sig/ont/fma/fma67257"},{"id":"A88","pred":"fma_id","subj":"T88","obj":"http://purl.org/sig/ont/fma/fma68646"},{"id":"A89","pred":"fma_id","subj":"T89","obj":"http://purl.org/sig/ont/fma/fma67122"},{"id":"A90","pred":"fma_id","subj":"T90","obj":"http://purl.org/sig/ont/fma/fma67122"},{"id":"A91","pred":"fma_id","subj":"T91","obj":"http://purl.org/sig/ont/fma/fma67264"},{"id":"A92","pred":"fma_id","subj":"T92","obj":"http://purl.org/sig/ont/fma/fma74412"},{"id":"A93","pred":"fma_id","subj":"T93","obj":"http://purl.org/sig/ont/fma/fma67257"},{"id":"A94","pred":"fma_id","subj":"T94","obj":"http://purl.org/sig/ont/fma/fma68877"},{"id":"A95","pred":"fma_id","subj":"T95","obj":"http://purl.org/sig/ont/fma/fma7195"},{"id":"A96","pred":"fma_id","subj":"T96","obj":"http://purl.org/sig/ont/fma/fma67257"},{"id":"A97","pred":"fma_id","subj":"T97","obj":"http://purl.org/sig/ont/fma/fma74412"},{"id":"A98","pred":"fma_id","subj":"T98","obj":"http://purl.org/sig/ont/fma/fma67122"},{"id":"A99","pred":"fma_id","subj":"T99","obj":"http://purl.org/sig/ont/fma/fma67122"},{"id":"A100","pred":"fma_id","subj":"T100","obj":"http://purl.org/sig/ont/fma/fma67122"},{"id":"A101","pred":"fma_id","subj":"T101","obj":"http://purl.org/sig/ont/fma/fma62871"},{"id":"A102","pred":"fma_id","subj":"T102","obj":"http://purl.org/sig/ont/fma/fma67257"},{"id":"A103","pred":"fma_id","subj":"T103","obj":"http://purl.org/sig/ont/fma/fma67257"},{"id":"A104","pred":"fma_id","subj":"T104","obj":"http://purl.org/sig/ont/fma/fma74412"},{"id":"A105","pred":"fma_id","subj":"T105","obj":"http://purl.org/sig/ont/fma/fma67257"},{"id":"A106","pred":"fma_id","subj":"T106","obj":"http://purl.org/sig/ont/fma/fma67257"},{"id":"A107","pred":"fma_id","subj":"T107","obj":"http://purl.org/sig/ont/fma/fma67264"},{"id":"A108","pred":"fma_id","subj":"T108","obj":"http://purl.org/sig/ont/fma/fma9825"},{"id":"A109","pred":"fma_id","subj":"T109","obj":"http://purl.org/sig/ont/fma/fma9825"},{"id":"A110","pred":"fma_id","subj":"T110","obj":"http://purl.org/sig/ont/fma/fma62864"},{"id":"A111","pred":"fma_id","subj":"T111","obj":"http://purl.org/sig/ont/fma/fma68646"},{"id":"A112","pred":"fma_id","subj":"T112","obj":"http://purl.org/sig/ont/fma/fma68646"},{"id":"A113","pred":"fma_id","subj":"T113","obj":"http://purl.org/sig/ont/fma/fma63261"},{"id":"A114","pred":"fma_id","subj":"T114","obj":"http://purl.org/sig/ont/fma/fma67257"},{"id":"A115","pred":"fma_id","subj":"T115","obj":"http://purl.org/sig/ont/fma/fma62925"},{"id":"A116","pred":"fma_id","subj":"T116","obj":"http://purl.org/sig/ont/fma/fma86583"},{"id":"A117","pred":"fma_id","subj":"T117","obj":"http://purl.org/sig/ont/fma/fma86578"},{"id":"A118","pred":"fma_id","subj":"T118","obj":"http://purl.org/sig/ont/fma/fma63261"},{"id":"A119","pred":"fma_id","subj":"T119","obj":"http://purl.org/sig/ont/fma/fma84050"},{"id":"A120","pred":"fma_id","subj":"T120","obj":"http://purl.org/sig/ont/fma/fma63261"},{"id":"A121","pred":"fma_id","subj":"T121","obj":"http://purl.org/sig/ont/fma/fma84050"},{"id":"A122","pred":"fma_id","subj":"T122","obj":"http://purl.org/sig/ont/fma/fma84050"},{"id":"A123","pred":"fma_id","subj":"T123","obj":"http://purl.org/sig/ont/fma/fma256135"},{"id":"A124","pred":"fma_id","subj":"T124","obj":"http://purl.org/sig/ont/fma/fma84050"},{"id":"A125","pred":"fma_id","subj":"T125","obj":"http://purl.org/sig/ont/fma/fma67498"},{"id":"A126","pred":"fma_id","subj":"T126","obj":"http://purl.org/sig/ont/fma/fma86578"},{"id":"A127","pred":"fma_id","subj":"T127","obj":"http://purl.org/sig/ont/fma/fma86578"},{"id":"A128","pred":"fma_id","subj":"T128","obj":"http://purl.org/sig/ont/fma/fma86578"},{"id":"A129","pred":"fma_id","subj":"T129","obj":"http://purl.org/sig/ont/fma/fma86578"},{"id":"A130","pred":"fma_id","subj":"T130","obj":"http://purl.org/sig/ont/fma/fma62871"},{"id":"A131","pred":"fma_id","subj":"T131","obj":"http://purl.org/sig/ont/fma/fma86578"},{"id":"A132","pred":"fma_id","subj":"T132","obj":"http://purl.org/sig/ont/fma/fma84050"},{"id":"A133","pred":"fma_id","subj":"T133","obj":"http://purl.org/sig/ont/fma/fma256135"},{"id":"A134","pred":"fma_id","subj":"T134","obj":"http://purl.org/sig/ont/fma/fma84050"},{"id":"A135","pred":"fma_id","subj":"T135","obj":"http://purl.org/sig/ont/fma/fma84050"},{"id":"A136","pred":"fma_id","subj":"T136","obj":"http://purl.org/sig/ont/fma/fma68646"},{"id":"A137","pred":"fma_id","subj":"T137","obj":"http://purl.org/sig/ont/fma/fma9637"},{"id":"A138","pred":"fma_id","subj":"T138","obj":"http://purl.org/sig/ont/fma/fma9825"},{"id":"A139","pred":"fma_id","subj":"T139","obj":"http://purl.org/sig/ont/fma/fma63261"},{"id":"A140","pred":"fma_id","subj":"T140","obj":"http://purl.org/sig/ont/fma/fma63261"},{"id":"A141","pred":"fma_id","subj":"T141","obj":"http://purl.org/sig/ont/fma/fma86578"},{"id":"A142","pred":"fma_id","subj":"T142","obj":"http://purl.org/sig/ont/fma/fma7196"},{"id":"A143","pred":"fma_id","subj":"T143","obj":"http://purl.org/sig/ont/fma/fma5034"},{"id":"A144","pred":"fma_id","subj":"T144","obj":"http://purl.org/sig/ont/fma/fma9671"},{"id":"A145","pred":"fma_id","subj":"T145","obj":"http://purl.org/sig/ont/fma/fma5034"},{"id":"A146","pred":"fma_id","subj":"T146","obj":"http://purl.org/sig/ont/fma/fma9671"},{"id":"A147","pred":"fma_id","subj":"T147","obj":"http://purl.org/sig/ont/fma/fma7196"},{"id":"A148","pred":"fma_id","subj":"T148","obj":"http://purl.org/sig/ont/fma/fma9637"},{"id":"A149","pred":"fma_id","subj":"T149","obj":"http://purl.org/sig/ont/fma/fma62863"},{"id":"A150","pred":"fma_id","subj":"T150","obj":"http://purl.org/sig/ont/fma/fma9637"},{"id":"A151","pred":"fma_id","subj":"T151","obj":"http://purl.org/sig/ont/fma/fma63261"},{"id":"A152","pred":"fma_id","subj":"T152","obj":"http://purl.org/sig/ont/fma/fma62863"},{"id":"A153","pred":"fma_id","subj":"T153","obj":"http://purl.org/sig/ont/fma/fma264783"},{"id":"A154","pred":"fma_id","subj":"T154","obj":"http://purl.org/sig/ont/fma/fma62860"},{"id":"A155","pred":"fma_id","subj":"T155","obj":"http://purl.org/sig/ont/fma/fma62864"},{"id":"A156","pred":"fma_id","subj":"T156","obj":"http://purl.org/sig/ont/fma/fma7195"},{"id":"A157","pred":"fma_id","subj":"T157","obj":"http://purl.org/sig/ont/fma/fma63194"},{"id":"A158","pred":"fma_id","subj":"T158","obj":"http://purl.org/sig/ont/fma/fma68646"},{"id":"A159","pred":"fma_id","subj":"T159","obj":"http://purl.org/sig/ont/fma/fma63261"},{"id":"A160","pred":"fma_id","subj":"T160","obj":"http://purl.org/sig/ont/fma/fma63261"},{"id":"A161","pred":"fma_id","subj":"T161","obj":"http://purl.org/sig/ont/fma/fma9825"},{"id":"A162","pred":"fma_id","subj":"T162","obj":"http://purl.org/sig/ont/fma/fma68646"},{"id":"A163","pred":"fma_id","subj":"T163","obj":"http://purl.org/sig/ont/fma/fma84050"},{"id":"A164","pred":"fma_id","subj":"T164","obj":"http://purl.org/sig/ont/fma/fma84050"},{"id":"A165","pred":"fma_id","subj":"T165","obj":"http://purl.org/sig/ont/fma/fma63083"},{"id":"A166","pred":"fma_id","subj":"T166","obj":"http://purl.org/sig/ont/fma/fma9670"},{"id":"A167","pred":"fma_id","subj":"T167","obj":"http://purl.org/sig/ont/fma/fma84050"},{"id":"A168","pred":"fma_id","subj":"T168","obj":"http://purl.org/sig/ont/fma/fma86578"},{"id":"A169","pred":"fma_id","subj":"T169","obj":"http://purl.org/sig/ont/fma/fma84050"},{"id":"A170","pred":"fma_id","subj":"T170","obj":"http://purl.org/sig/ont/fma/fma9670"},{"id":"A171","pred":"fma_id","subj":"T171","obj":"http://purl.org/sig/ont/fma/fma84050"},{"id":"A172","pred":"fma_id","subj":"T172","obj":"http://purl.org/sig/ont/fma/fma305853"},{"id":"A173","pred":"fma_id","subj":"T173","obj":"http://purl.org/sig/ont/fma/fma68646"},{"id":"A174","pred":"fma_id","subj":"T174","obj":"http://purl.org/sig/ont/fma/fma68646"},{"id":"A175","pred":"fma_id","subj":"T175","obj":"http://purl.org/sig/ont/fma/fma67257"},{"id":"A176","pred":"fma_id","subj":"T176","obj":"http://purl.org/sig/ont/fma/fma9637"},{"id":"A177","pred":"fma_id","subj":"T177","obj":"http://purl.org/sig/ont/fma/fma278683"},{"id":"A178","pred":"fma_id","subj":"T178","obj":"http://purl.org/sig/ont/fma/fma256135"},{"id":"A179","pred":"fma_id","subj":"T179","obj":"http://purl.org/sig/ont/fma/fma9637"},{"id":"A180","pred":"fma_id","subj":"T180","obj":"http://purl.org/sig/ont/fma/fma256135"},{"id":"A181","pred":"fma_id","subj":"T181","obj":"http://purl.org/sig/ont/fma/fma9639"},{"id":"A182","pred":"fma_id","subj":"T182","obj":"http://purl.org/sig/ont/fma/fma256135"},{"id":"A183","pred":"fma_id","subj":"T183","obj":"http://purl.org/sig/ont/fma/fma24728"},{"id":"A184","pred":"fma_id","subj":"T184","obj":"http://purl.org/sig/ont/fma/fma9637"},{"id":"A185","pred":"fma_id","subj":"T185","obj":"http://purl.org/sig/ont/fma/fma7153"},{"id":"A186","pred":"fma_id","subj":"T186","obj":"http://purl.org/sig/ont/fma/fma67257"},{"id":"A187","pred":"fma_id","subj":"T187","obj":"http://purl.org/sig/ont/fma/fma67257"},{"id":"A188","pred":"fma_id","subj":"T188","obj":"http://purl.org/sig/ont/fma/fma68646"},{"id":"A189","pred":"fma_id","subj":"T189","obj":"http://purl.org/sig/ont/fma/fma74402"},{"id":"A190","pred":"fma_id","subj":"T190","obj":"http://purl.org/sig/ont/fma/fma74402"},{"id":"A191","pred":"fma_id","subj":"T191","obj":"http://purl.org/sig/ont/fma/fma68646"},{"id":"A192","pred":"fma_id","subj":"T192","obj":"http://purl.org/sig/ont/fma/fma13478"},{"id":"A193","pred":"fma_id","subj":"T193","obj":"http://purl.org/sig/ont/fma/fma68646"},{"id":"A194","pred":"fma_id","subj":"T194","obj":"http://purl.org/sig/ont/fma/fma74402"},{"id":"A195","pred":"fma_id","subj":"T195","obj":"http://purl.org/sig/ont/fma/fma74402"},{"id":"A196","pred":"fma_id","subj":"T196","obj":"http://purl.org/sig/ont/fma/fma67257"},{"id":"A197","pred":"fma_id","subj":"T197","obj":"http://purl.org/sig/ont/fma/fma9670"},{"id":"A198","pred":"fma_id","subj":"T198","obj":"http://purl.org/sig/ont/fma/fma68646"},{"id":"A199","pred":"fma_id","subj":"T199","obj":"http://purl.org/sig/ont/fma/fma67257"},{"id":"A200","pred":"fma_id","subj":"T200","obj":"http://purl.org/sig/ont/fma/fma82737"},{"id":"A201","pred":"fma_id","subj":"T201","obj":"http://purl.org/sig/ont/fma/fma82739"},{"id":"A202","pred":"fma_id","subj":"T202","obj":"http://purl.org/sig/ont/fma/fma63011"},{"id":"A203","pred":"fma_id","subj":"T203","obj":"http://purl.org/sig/ont/fma/fma63011"},{"id":"A204","pred":"fma_id","subj":"T204","obj":"http://purl.org/sig/ont/fma/fma63023"},{"id":"A205","pred":"fma_id","subj":"T205","obj":"http://purl.org/sig/ont/fma/fma63023"},{"id":"A206","pred":"fma_id","subj":"T206","obj":"http://purl.org/sig/ont/fma/fma68646"},{"id":"A207","pred":"fma_id","subj":"T207","obj":"http://purl.org/sig/ont/fma/fma67653"},{"id":"A208","pred":"fma_id","subj":"T208","obj":"http://purl.org/sig/ont/fma/fma68646"},{"id":"A209","pred":"fma_id","subj":"T209","obj":"http://purl.org/sig/ont/fma/fma63023"},{"id":"A210","pred":"fma_id","subj":"T210","obj":"http://purl.org/sig/ont/fma/fma68646"},{"id":"A211","pred":"fma_id","subj":"T211","obj":"http://purl.org/sig/ont/fma/fma68646"},{"id":"A212","pred":"fma_id","subj":"T212","obj":"http://purl.org/sig/ont/fma/fma68646"},{"id":"A213","pred":"fma_id","subj":"T213","obj":"http://purl.org/sig/ont/fma/fma67257"},{"id":"A214","pred":"fma_id","subj":"T214","obj":"http://purl.org/sig/ont/fma/fma63841"},{"id":"A215","pred":"fma_id","subj":"T215","obj":"http://purl.org/sig/ont/fma/fma68646"},{"id":"A216","pred":"fma_id","subj":"T216","obj":"http://purl.org/sig/ont/fma/fma63023"},{"id":"A217","pred":"fma_id","subj":"T217","obj":"http://purl.org/sig/ont/fma/fma7195"},{"id":"A218","pred":"fma_id","subj":"T218","obj":"http://purl.org/sig/ont/fma/fma67257"},{"id":"A219","pred":"fma_id","subj":"T219","obj":"http://purl.org/sig/ont/fma/fma67257"},{"id":"A220","pred":"fma_id","subj":"T220","obj":"http://purl.org/sig/ont/fma/fma67257"},{"id":"A221","pred":"fma_id","subj":"T221","obj":"http://purl.org/sig/ont/fma/fma68646"},{"id":"A222","pred":"fma_id","subj":"T222","obj":"http://purl.org/sig/ont/fma/fma68646"},{"id":"A223","pred":"fma_id","subj":"T223","obj":"http://purl.org/sig/ont/fma/fma68646"},{"id":"A224","pred":"fma_id","subj":"T224","obj":"http://purl.org/sig/ont/fma/fma63841"},{"id":"A225","pred":"fma_id","subj":"T225","obj":"http://purl.org/sig/ont/fma/fma68646"}],"text":"Nanotechnology Tools to Inactivate SARS-CoV-2 in Patients\nThe main target of SARS-CoV-2 is the respiratory tract (upper airways, lung),36 although other organs might also be infected (e.g., gut, kidney) and vasculature21 also appears to be a prime target. The expression of ACE2 probably determines uptake by different tissues.37\nIn addition to discussing immune-based approaches, because the lung is the most critically affected organ, we will center our discussion on the various options to inactivate the virus in the deep lung and to target the essential host cells for drug delivery. The virus reaches the alveoli and enters alveolar epithelial type II cells (AECII), due to the relatively high abundance of ACE2 and a permissive cellular milieu. These cells serve as a reservoir of the virus, which finally spreads throughout the lung, leading to the lung function impairment seen in severe cases. Airborne nanomaterials are optimally suited to penetrate into the deep lung due to the physicochemical properties of such aerosols, existing on the same size scale particles that penetrate most readily to the deep airways. Hence, nanomedicine is already actively pursuing ideas to deliver drugs, therapeutic proteins, and mRNAs by exploiting nanodevices for pulmonary delivery.38,39\nMoreover, the rapid emergence of SARS-CoV-2 has exposed one of the main weaknesses in the current medical landscape: the lack of broad-spectrum antiviral drugs. At present, there are only a handful of approved antivirals, and they are mostly virus-specific. Hence, when a new virus emerges, little can be done pharmacologically to slow down its spread. Some research efforts have been focused on the development of broad-spectrum drugs, which could potentially offer some efficacy against future emerging viruses (and maybe SARS-CoV-2). The various approaches developed over the years are mainly based on the creation of entry inhibitors.40,41 A highly conserved part in viruses is the attachment ligand (VAL). In most known respiratory viruses,42 the VAL targets either heparan sulfate proteoglycans (HSPG)43 or sialic acids (SA).44 Both HSPG and SA mimics have shown in vitro ability to bind to viruses, blocking their interaction with cell membranes, and often in a broad-spectrum way.45−47\nIn the context of nanomedicine, many nanomaterials have been developed, ranging from polymers48 to dendrimers,49 oligomers, NPs,50 liposomes,51 and small molecules.52 However, successful clinical translation has been hindered by the fact that, upon dilution, these compounds lose efficacy as the virus-compound complex dissociates leaving viruses free to restart their replication cycle. Recently, it has been shown that this limitation can be overcome by synthesizing NPs that, after binding, are able to inhibit viral infectivity irreversibly by permanently damaging the virion, refueling the hope for a true, broad-spectrum antiviral drug.53 Because the focus is also on the development of a drug specific to SARS-CoV-2, a good entry inhibitor could be based on blocking the S spike protein interaction with the cellular ACE2 receptor.19,21−23 Regardless of the specific approach, it is imperative that novel, effective antivirals be based on compounds that exhibit very low or negligible toxicity profiles, as patients will most likely need to receive those drugs for extended periods of time and will already be weakened. For these reasons, when designing antiviral drugs, clearance mechanisms have to be kept in mind. An example of this process is the recent redesign of broad-spectrum antiviral NPs into equally effective modified cyclodextrins.54 Moreover, nanotechnology may offer nanotheranostic approaches to fill the existing gap between diagnostics and therapy.55−57 The simultaneous management of both diagnostics and therapy for those suffering from COVID-19 or in future pandemics, as for many other diseases, is an additional potential strategy to take into consideration in which nanomaterials have proven to be effective tools. The advantages of the capabilities of nanotechnology and nanomaterials for combined therapeutics and diagnostics has been widely explored in cancer research; however, there have been considerable efforts in the past few years to extend the scope of this approach to other areas including infectious diseases.58\n\nNanomaterial-Based Vaccine Development and Immunomodulation\nFollowing the publication of the genetic sequence of SARS-CoV-2 on January 11, 2020, intense research efforts have been devoted to developing a vaccine against COVID-19. With unprecedented speed, this extraordinary scientific mobilization led the first vaccine candidate to enter the Phase I human clinical trial on March 16, 2020, and other novel candidates are rapidly following.59 Up to May 22, 2020, there are 10 COVID-19 candidate vaccines in clinical evaluations and 114 in preclinical development.60\nConcerning vaccine and immunization research, nanomaterials can assist in multiple ways to boost the upregulation required by the immune system and to direct the immune response specifically against antigens. Immune-targeted nanotherapeutics can be developed through their rational manufacture at the nanoscale level by designing nanomaterials that are able to amplify host’s immune response, for instance as adjuvants in the context of vaccination.\nThe development of a vaccine will rely either on the direct administration of viral antigens (e.g., in the form of recombinant proteins, vectored vaccines, or whole inactivated or attenuated virus) or RNA- or DNA-encoding viral antigens.27 Candidate antigens for immunization are surface proteins such as the immunogenic spike protein (S1), which is already targeted by antibodies of convalescent patients.27 Because the S1 protein is also essential for cellular uptake, many researchers are using this protein as the primary target for a vaccine.\nThere are many issues related to the delivery of a drug, protein, or RNA into the patient as the cargo is often degraded, not bioavailable, or is swiftly cleared. Nanotechnology provides multiple solutions to these challenges though, as nanocarriers can overcome some of these limitations.\nBiocompatible polymeric-, lipid-based, or inorganic NPs can be tuned with respect to their physicochemical properties to encapsulate cargo proteins with high loading efficiency, improving protein delivery and pharmacokinetics over conventional approaches.38 Intranasal delivery of polymer-encapsulated antigen triggers a strong immune response, and the success of vaccination depends on the appropriate type of polymer in combination with the antigen.61,62 Similarly, researchers have developed lipid and lipid-based NPs as delivery platforms for mRNAs or siRNAs to enable the synthesis of key viral proteins for vaccination or to inactivate critical viral target genes, respectively.39 The field of therapeutic mRNAs to support vaccination has gained momentum, as well,63 at least in part due to nanotechnology-enabled strategies for cargo delivery. Moreover, the versatility of nanoplatforms as antigen-presenting systems offers great opportunities. Considering the possibility of random viral mutations that may alter the antigen shape, functionalizing nanomaterials with a wide number of molecules at the same time to target the virus, or motifs that are specific for pathogens, will increase the efficiency of vaccines and their ability to prevent viral infection.\nSeveral companies are working on mRNA vaccines encoding SARS-CoV-2 proteins such as the spike protein, encapsulated in nanoliposomes with specific physicochemical properties that are potentially akin to those documented for immunization against certain tumor antigens.64 The design of such nanocarriers, which will need to escape recognition by scavenger cells and to be nontoxic and nonimmunogenic, is a challenge that will require substantial time prior to clinical availability.\nOn March 16, 2020, Moderna, through a partnership with the Vaccine Research Center at the U.S. National Institutes of Health, enrolled the first participants into a Phase I clinical trial testing an mRNA vaccine (mRNA-1273) encapsulated in lipid NPs—a record time of just 63 days following sequence selection (NCT04283461).65 The enrollment of the first cohort of participants (18 to 55-year-old healthy subjects) concluded on April 16, 2020.66 CureVac and BioNTech (in partnership with Pfizer) are currently working on similar vaccines; Pfizer/BioNTech, in particular, have recently started the recruitment in Phase I/II trials (NCT04368728, NCT04380701). A DNA plasmid vaccine by Inovio Pharmaceuticals (INO-4800) has showed promising results in mice and guinea pigs according to a recent article published in Nature Communications(67) and has entered Phase I testing in humans (NCT04336410).\nAnother candidate COVID-19 vaccine for Phase I clinical trial is from the University of Oxford and AstraZeneca (NCT04324606).68 Around 1110 people will take part in the trial, which started recruitment at the end of April 2020. The vaccine is based on a chimpanzee adenovirus vaccine vector (ChAdOx1) and the SARS-CoV-2 spike protein. Chimpanzee adenoviral vectors against different pathogens have been already tested in thousands of subjects with demonstrated safety. To date, ChAdOx1 has been administered to six rhesus macaques exposed to high doses of SARS-CoV-2. The vaccine was unable to prevent infections, although it reduced the severity of the disease: no signs of virus replication were observed in the lungs, with significantly lower levels of respiratory disease and no lung damage compared to control animals, according to a recent article deposited in bioRxiv.69 Another adenoviral vector vaccine developed by CanSino Biological Inc. and Beijing Institute of Biotechnology using a genetically engineered replication-defective adenovirus type 5 vector to express the SARS-CoV-2 spike protein (Ad5-nCoV) is currently being tested in Phase I/II trials (NCT04398147, NCT04341389, NCT0431312).\nAlthough vaccines based on novel DNA and mRNA technologies might be promising, there are no such kinds of vaccines on the market, and it is unknown whether they can be effective in humans. Moderna, for instance, has generated preliminary safety data on different mRNA-based vaccines targeting other respiratory viruses, but their more advanced program (on a cytomegalovirus vaccine) is still in Phase II clinical testing. However, on May 18, Moderna announced that mRNA-1273 elicited antibody titers above the levels observed in convalescent individuals (and therefore considered potentially protective) in all eight initial participants across the 25 and 100 μg dose cohorts of the Phase I trial (NCT04283461).70 The company, after having received a fast-track approval from the FDA, is now moving on Phase II trials.\nConversely, strong evidence exists regarding the efficacy of protein-based vaccines such as recombinant-protein, viral-vector, attenuated, or inactivated vaccines across different infectious diseases, with licensed vaccines already existing for all of these platforms.27 All of the aforementioned approaches are currently being explored in the context of SARS-CoV-2.27 An inactivated vaccine developed by the China National Pharmaceutical Group (Sinopharm), in collaboration with the Wuhan Institute of Biological Products, is currently tested in a Phase I/II trial (ChiCTR2000031809), and a second inactivated vaccine (in collaboration with the Beijing Institute of Biological Products) has been currently approved for clinical testing (ChiCTR2000032459). A third aluminum salt (alum) adjuvanted inactivated vaccine, developed by Beijing-based Sinovac Biotech’s, was able to provide partial or complete protection in rhesus macaques, according to a recent article published in Science,71 and is currently being tested in Phase I/II trials (NCT04383574, NCT04352608).\nLive-attenuated vaccines are intrinsically immunogenic (e.g., due to the presence of viral DNA), but extensive safety tests are required due to the rare possibility of reversion to a pathogenic form able to cause infection. Vaccine candidates based in this application have previously been designed for SARS-CoV with high stability.72,73 Recombinant-protein vaccines and inactivated vaccines are safer but might require adjuvants to increase their immunogenicity. In the context of SARS-CoV-2, adjuvants are important for two reasons. First, adjuvants might increase the efficacy of the vaccine, especially in subjects with impaired immunological function, such as the elderly, or in subjects with comorbidities resulting in immune dysfunctions; in these patient cohorts, SARS-CoV-2 has a high lethality rate. Second, adjuvants can reduce the amount of vaccine protein(s) required per dose, which could facilitate scaling-up vaccine production in a reduced time frame.\nBeyond alum, which is in fact a nanoscale material74 that was developed in the 1920s for the tetanus and diphtheria toxoids,75 approval for a new adjuvant did not occur until 1997, with the introduction of the oil-in-water emulsion of squalene oil and polysorbate 80 and sorbitan trioleate surfactants (MF59) in the seasonal influenza vaccine for the elderly.76 The use of MF59 was further expanded to pandemic and avian influenza vaccines.77 Other adjuvants in licensed vaccines have been approved since 2000, as well: (a) AS03 (used for pandemic and avian influenza vaccines), similar to MF59, but including α-tocopherol as an additional immune stimulant; (b) AF 03 (used for pandemic influenza vaccines), an alternative squalene emulsion containing polyoxyethylene, cetostearyl ether, mannitol, and orbitan oleate;78 (c) AS 01 (used for herpes zoster vaccine), a liposome-based vaccine adjuvant system containing two immunostimulants, 3-O-desacyl-4′-monophosphoryl lipid A (MPL, a Toll-like receptor 4 agonist) and saponin QS-21, which activates the ACT-NLRP3 inflammasome pathway;79 and (d) AS04 (used for hepatitis B and human papilloma virus vaccines), which is a combination of MPL and aluminum hydroxide.76,80\nIn this context, the concept of “nanoimmunity by design” relies on the rational design of distinct physicochemical properties and specific functionalization of nanomaterials intended for fine-tuning their potential effects on the immune system.81 Nanomaterials have emerged as promising tools for immune modulation, either stimulating or suppressing the immune response. In fighting SARS-CoV-2, these properties may find applications for both prevention and therapy and in the context of vaccine development.\nMounting evidence indicates that nanomaterials such as graphene,82 nanodiamonds,83 carbon nanotubes,84 and polystyrene particles85 bear an intrinsic capacity to activate the immune system, depending on their functionalization.86 For instance, graphene oxide functionalized with amino groups (GO-NH2) induces activation of STAT1/IRF1 interferon signaling in monocytes and T cells, resulting in the production of T cell chemoattractants, and macrophage 1 (M1) 1/T-helper 1 (Th1) polarization of the immune response, with negligible toxicity.82 Remarkably, the ability of licensed adjuvants such as AS01 and AS03 to enhance adaptive immunity has been linked to their capacity to boost STAT1/IRF1 interferon signaling.87 In addition, recent SARS-CoV and MERS-CoV studies suggest that the development of a Th1-type response is central for controlling infection, which also may be true for SARS-CoV-2.88\nSeveral groups and consortia have been screening and characterizing nanomaterials according to their immunomodulatory properties and absence of cytotoxicity.56,57,82−84,86 The development of an adjuvant for clinical use is a lengthy process that requires extensive Phase III randomized trials in large and diverse cohorts of subjects, and the process generally requires several years.76 Pharmaceutical giants such as GlaxoSmithKline (GSK), which owns the ASs mentioned above and other adjuvant platforms, are engaged in several partnerships to embed their adjuvant systems with SARS-CoV-2-protein-based vaccines. A full-length recombinant SARS-CoV-2 glycoprotein nanoparticle vaccine adjuvanted with the saponin-based Matrix M developed by Novavax is in Phase I clinical testing (NCT04368988).89\nAlthough it is unlikely that novel adjuvants would be used in the context of the current pandemic, the SARS-CoV-2 pandemic offers an opportunity to reflect on the potential of nanotechnology for vaccine adjuvant development. In this context, it is critical to stream coherent pipelines covering in vitro and in vivo experiments specifically to select candidate materials that might be tested for clinical implementation as vaccine adjuvants.90\nIn this view, the status of nanomaterial-based vaccine adjuvants has been reviewed.91,92 In particular, immunomodulatory effects induced on the innate immune signaling have been demonstrated.91 For example, nanomaterials such as GO can elicit an inflammasome sensor (NLRP3)-dependent expression of IL-1β in macrophages.93 Notably, alum, the most commonly used adjuvant in human vaccines, induces the release of this cytokine in macrophages through the same NRLP-induced mechanism. These results suggest that nanomaterials such as GO and alum may be useful for medical applications.94\nConsidering that new vaccine development typically requires years to be approved and applied to the general population, there is also an urgent need to study how to improve treatment approaches. According to the first Phase III clinical studies, the antiviral drug Remdesivir, which was recently approved by the FDA for COVID-19 treatment in the United States, seems to be a promising treatment for adults diagnosed with COVID-19. Nano-based strategies have already been applied to enhance the effectiveness of Remdesivir in the context of other emerging viral infections (Nipah virus),95 suggesting the suitability of nanotechnology to assist with similar strategies for the treatment of COVID-19 as well as other possible pandemics in the future.96\n\nCan Nanotechnology Help to Control the Cytokine Storm?\nOne of the main features of COVID-19 is the triggering of a cytokine storm in the body, also known as cytokine release syndrome (CRS), which results from an excessive immune response and leads to the severe deterioration of patient health.25,97,98 This inflammatory storm is one of the major causes of the acute respiratory distress syndrome (ARDS) that is often associated with multiple-organ failure, representing the leading causes of death in critical patients.98 In particular, the role of interleukin (IL)-6 has been highlighted in patients requiring assisted ventilation. Ongoing clinical trials are testing drugs that block the receptor of IL-6 (Tocilizumab, an anti-IL-6 receptor antibody, and Sarilumab) or IL-6 itself (Siltuximab).\nWhereas a well-regulated cytokine response that is rapidly triggered by the host’s innate immunity can serve to prevent and to counteract an infection, an excessive, unbalanced, prolonged immune response can seriously harm the body. Therefore, therapeutic strategies aimed at effectively suppressing the cytokine storm are under investigation. Nanomaterials have been exploited to adjust the immune response to an optimized level, and such proprieties might be explored to inhibit cytokine releases.99 Nanosystems can enhance the specificity/efficiency of immunosuppressant delivery to target immune cells, with consequent reductions in drug dose, drug distribution to nontarget tissues and organs, and possible side effects. In addition, specific nanotools can be designed to evade the immune system and to enhance the solubility of poorly soluble immunosuppressant agents; the potential of finely tuning their surface charge opens possibilities for encapsulation strategies and offers accommodation for a high drug load. All of these mechanisms may also occur simultaneously, enhancing the activity of immunosuppressive agents.\nConcerning the role of macrophages in COVID-19, the presence of ACE2-expressing CD68+CD169+ macrophages containing SARS-CoV-2 nucleoprotein antigen and showing an increased release of IL-6 was observed in infected spleen and lymph nodes.100 Notably, immunohistochemical and immunofluorescence analyses of lymph nodes and spleen tissue from autopsy samples of patients who died from COVID-19 revealed lymphocytic apoptosis. The tissues infected by SARS-CoV-2 also showed an upregulated expression of Fas, suggesting a role for CD169+ macrophages in viral spreading, aberrant inflammation, and activation-induced lymphocyte apoptosis. Moreover, histological examinations of biopsy samples of patients who died from COVID-19 revealed an increased alveolar exudate due to the extended neutrophil and monocyte infiltrate in lung capillaries with fibrin deposition, probably leading to difficulties in gas exchange. Through nanomedicine, we envision therapeutic approaches aimed at targeting specific immune subpopulations to avoid these complications, and different nanomaterials have already been explored for their specific impact on different immune cell subpopulations.82,83,86 Octadecylamine-functionalized and dexamethasone-adsorbed nanodiamond promotes anti-inflammatory and proregenerative behavior in human macrophages in vitro.101 A low dose of this functionalized nanodiamond also reduced macrophage infiltration and expression of proinflammatory mediators iNOS and tumor necrosis factor (TNF)-α in mice. Overall, these results suggest that nanodiamond particles may be useful as an inherently immunomodulatory platform.\nFinally, the indiscriminate systemic suppression of the immune system may increase the susceptibility to sepsis due to underlying secondary bacterial infections that can exacerbate critical conditions in patients, causing death.102 Risk factors for mortality include acquired bacterial infections by opportunistic organisms and primary pathogens. Using nanocarriers to achieve targeted delivery of immunosuppressive drugs, or the specific and controlled inhibition of only a specific immune cell subpopulation, can play a critical role in limiting such complications.\nThe fast and complete removal of pro-inflammatory cytokines from the bloodstream has been shown to increase survival significantly in patients with early stage sepsis. Adsorption of cytokines from serum by extracorporeal perfusion through porous carbons is one of the most promising ways for their selective, quick, and complete removal from blood circulation. Hierarchical carbon materials with tuned porosity have shown effective adsorption of many cytokines, including IL-6 and even the largest ones, such as TNF-α.103 Based on their demonstrated outstanding performance in removing sepsis-associated pro-inflammatory cytokines from blood, mesoporous carbon adsorbents with hierarchical structure, tuned pore size, and surface chemistry, as well as graphene with open and accessible surfaces104 have significant potential to quickly remove inflammatory cytokines associated with CRS and to prevent mortality arising from an uncontrolled inflammatory cascade, thereby providing enough time for the defense mechanisms of the human body to fight the virus.\n\nPhotodynamic Inactivation of SARS-CoV-2\nIn addition to drug- and vaccine-based antiviral strategies, photodynamic therapy (PDT) stands as a unique approach to inactivate SARS-CoV-2. Using a light-based method, PDT attacks target cells via the excitation of photosensitive agents, called photosensitizers (PSs), with radiation characterized by a wavelength corresponding to its absorption spectrum to generate reactive oxygen species (ROS) in the presence of oxygen, which ultimately results in cell death. Photodynamic therapy is primarily used for the clinical treatment of various oncological disorders.105 It was not until the 1970s that PDT was first used clinically against viruses,106 exploiting ROS production to damage virus proteins, nucleic acids, and—if present—lipids.107 Even though there are research efforts for PDT-based virotherapies against different viruses, including herpes simplex virus, human papilloma virus, and human immunodeficiency virus,106−109 clinical use of PDT is limited due to hydrophobicity of PSs, poor target specificity, and limited tissue penetration ability.\nMost PSs are hydrophobic and aggregate in aqueous solutions, affecting their photochemical and photobiological properties.106,110 For this reason, Lim et al. have proposed a promising approach for photodynamic inactivation of viruses with NPs, developing sodium yttrium fluoride (NaYF4) upconversion NPs (UCNs) with zinc phthalocyanine PSs grafted onto their surfaces. Unlike most PSs, these UCNs are coated with polyethylenimine (PEI), which render them hydrophilic and easier to manipulate. These UCNs showed antiviral activity against Dengue virus serotype 2 and adenovirus type 5, which were used as models of enveloped and non-enveloped viruses, respectively.110 MXenes111,112 are a large family of 2D transition metal carbides, nitrides,113 and carbonitrides114 that exhibit unique electronic, optical, and catalytic properties. They have the general formula Mn+1XnTx, where M is an early transition metal (Ti, Zr, V, Mo, etc.), X is C and/or N, Tx represents the surface functional groups (=O, −OH, −F, −Cl), and n = 1–4.115,116 Some examples include Ti3C2Tx, V2CTx, and Nb2CTx, with over 30 stoichiometric compositions already synthesized with more than 100 predicted. Biocompatible MXenes, such as Ti3C2Tx, are hydrophilic and are among the most efficient light-to-heat transforming materials.117 The plasmon resonance extinction maxima of Ti3C2Tx is at 780 nm, enabling the use of near-infrared (IR) light for PDT. Several other MXenes have absorption maxima in the IR range and have shown outstanding performance in PDT and theranostic applications.118\nFullerene and graphene are also good candidates for virus inactivation by PDT and have proved to be effective against Semliki Forest virus (SFV), vesicular stomatitis virus (VSV), HSV-1, HIV-1, mosquito iridovirus (MIV), and influenza A virus (IAV), as well as the phage MS2.107 In addition, several 2D nanomaterials, including graphene-based materials, MXenes, black phosphorus, graphitic carbon nitride, tungsten disulfide, and molybdenum disulfide, have been reported to improve the efficacy of PDT considerably for cancer treatment.56,57 Therefore, determining if such nanomaterial-based PDT protocols could be exploited to inactivate SARS-CoV-2 is of great interest.\n\nBiomimetic Engineering of Nanodelivery Systems: Artificial Viruses in the Making\nIn an effort to engineer the next generation of nanoscale vectors, scientists have moved from using inorganic components aimed at obtaining inert structures to utilizing biological building blocks that are able to convey additional functionalities to the resulting construct. To cope with the complexity of the body and to evade the multiple layers of defense that tissues and organs have, it is critical to rely on the ability of certain materials to interact with, rather than to eschew, the biology of our body. Every NP system conceived to date faces one common fate: whether injected, inhaled, ingested, or absorbed through our epithelia, all will at some point come into contact with the mixture of fluids and organic compounds that comprise the body. Under such conditions, every material reacts in a unique way according to the conditions they individually face (i.e., the tissue or body region they are in), their composition (i.e., organic or inorganic), and their physical properties (i.e., size, shape, surface charge).119 Inorganic NPs can function as globular protein mimics because of their similar size, charge, shape, and surface features that can be chemically functionalized to resemble proteins. These similarities can be used in biotechnology to control virus pathways or cell receptor interactions with NPs.120\nAmong the many attempts, the legendary accomplishments, and the epic failures, we could list thousands of different NPs, differing from one another thanks to the creative endeavors of their designers. Despite their remarkable differences and researchers’ endeavors to make them one of a kind, they all aim to achieve one goal: to deliver in a specific way one particular form of payload, while remaining as unnoticed as possible by the body’s defense mechanisms. For everyone who has made an effort to create their own version of such a silver bullet, it has come to mind that nature in its incredible variety had already invented a few ingenious solutions to this problem. In the world of nano-based drug delivery, one entity dominates as the quintessential example of precision, efficiency, and stealth: the virus. Not by chance, these two worlds share many features that span from the physical laws that govern their assembly and stability to the chemical similarities in their overall composition.121\nHistorically, the field of nanomedicine and the global endeavor to generate NPs for drug delivery arose from one of the greatest struggles in medicine: gene therapy. At the core of that approach was the idea that viruses could be used as Trojan horses to deliver the correct sequence of the gene into the cells that harbored the mutated copy. In order to identify the virus that offered the best delivery service, a cadre of viral strains and species were tested, adapted, and engineered to fit the aims and hit the targets. It took decades of trial and error, of progressive adjustments, and a few tragic mistakes to identify the viruses that could provide the ideal backbone to develop viral vectors able to ferry genetic cargo into the target cell.122 In the midst of that global challenge, nanotechnology offered a safer and more controllable alternative: to generate bespoke structures that could replace viral vectors and do the same job, delivering a payload from the point of injection to the site of action.\nFast forward a few decades, and the promises made by both worlds seem to have finally become reality, with a number of active clinical trials, some clinical success stories, and a few commercial products in the market space. Interestingly, the two worlds seem to have maintained a safe distance so as to avoid any collision or dangerous proximity. Exchange of ideas between the fields of gene therapy and nanomedicine is limited, and the potential for disciplinary growth through knowledge exchange has remained elusive to scientists in both fields.5 Many scientists have suggested that nanotechnologists could find inspiration in the mechanisms devised by viruses to elude immune surveillance, to overcome biological barriers, and to deliver their genetic payload with high specificity. Similarly, gene therapists would find high-tech solutions to their scalability and safety issues in looking at the new generations of biomimetic particles being generated.\nThe principles of biomimicry and bioinspiration have been used to design and to engineer drug-delivery technologies that reproduce or recapitulate biological materials, for what pertains to not only their structure and chemistry but also, more importantly, their functions. In drug delivery, surface recognition and nanoscale interactions between materials and biological entities are key to the success of the delivery strategy, and the use of biological building blocks such as membrane proteins has been proposed as a way to convey targeting and shielding moieties simultaneously.123 One can only hope that the recent events and global attention that viruses are capturing in the scientific world will spur a renewed interest in finding ways to adapt viral features and mechanisms of action to the world of NPs. Increased focus in this field would be useful to create virus-like NPs able to circulate in the blood system, overcoming the endothelial barrier, and to deliver their therapeutic payload with high efficiency.124\n\nInterference with Cellular Uptake, Immobilization, and Inactivation of the Virus Outside of the Host Cell\nNanomaterials can be synthesized with a high specific surface area of a few hundred square meters per gram. Therefore, dependent on the surface properties, nanomaterials efficiently adsorb biomolecules and form a so-called biomolecular corona. This passive, nontargeted adsorption might be utilized to bind viruses, provided that the selected nanomaterial is relatively biocompatible. Viral surface proteins are often modified by sugar moieties or encompass positively charged amino acid patches that bind to lectins or glycosaminoglycans (GAGs) of heparan sulfate (HS), respectively.32 Robust interactions of virus particles with these host receptors is ensured by multivalent binding, which is likely why single-molecule inhibitors often are not capable of efficiently perturbing this key event but multivalent NPs are superior to block binding of different viruses to the host cell.125\nGold NPs capped with mercaptoethanesulfonate are effective inhibitors of HSV type 1 infection as they mimic cell-surface-receptor heparan sulfate and, therefore, competitively bind to the virus. Interestingly, polyvalent sulfated Au NPs inhibit virus binding to the host cell dependent on their size. Nanoparticles of diameters equal to and larger than the virus diameter (in this case, the stomatitis virus) more efficiently inhibit the binding to cells than smaller particles. Most likely, larger NPs efficiently cross-link virions, whereas smaller NPs simply decorate the viral surface.126 Papp et al. found that gold NPs decorated with SA effectively inhibited the binding of influenza virus to the target cells. In this case, viral recognition via its surface protein hemagglutinin of SA on the host cell membrane was a prerequisite for cellular entry.127 More recently, Cagno et al. reported antiviral NPs (Au and iron oxide core) with long and flexible linkers mimicking HS that strongly bind and inactivate viruses such as respiratory syncytial virus in vitro and in vivo in a lung infection model and even led to irreversible viral deformation.53 Hence, there is ample evidence that biocompatible, functionalized NPs can act as broad-spectrum antivirals. Notably, the receptor-binding domain of the spike S1 protein of SARS-CoV-2 binds not only to ACE2 but also to heparin128 and, thus, might be targeted by similar approaches as outlined above.\nOne of the most recent strategies to inhibit viral uptake is based on administration of recombinant ACE2 to inhibit binding competitively via the spike S1 protein.21 Knowing that multivalency is key to block virus–host interactions reliably, researchers have speculated that a nanostructured carrier could not only improve delivery and cargo stability but also might dramatically enhance binding strength.129\n\nSpeeding up the Nanomedicine-Based Approaches for COVID-19 by In Silico Analysis\nCurrently, repurposing drug molecules is a key strategy for identifying approved or investigational drugs outside the scope of the original medical indication that can be used to fight COVID-19.130,131 There are various advantages to this strategy, including already-established safety profiles, fast transition to clinical studies, and less investment needed, compared to the process of developing an entirely new drug.132,133 Therefore, these advantages have the potential to result in less risky and more rapid returns on investment in the development of repurposed drugs, benefits that are particularly important during pandemics. In addition to rapid diagnosis, making recommendations to physicians about rapid treatment methods can save the lives of many people. Combining in silico tools such as molecular docking, molecular dynamics, and computational chemistry with large drug databases provides a great advantage in selecting “possible candidates” from among thousands of pharmaceutically active substances (Figure 3). In silico analyses should be supported by the literature, expert opinions (pharmacologists and clinicians), and, when possible, preclinical and clinical findings. After selecting the best candidates following in silico analyses, detailed in vitro and in vivo experimentation should be undertaken prior to clinical studies. Recently, various drug-repurposing studies have been published to assist in the global COVID-19 pandemic response.134−136 Such studies aim to identify whether already-approved drugs have the capacity to interact with viral proteins or receptors on host cells.\nFigure 3 Schematic of how in silico analysis can be used to select candidate drug molecules for clinical studies. MD, molecular dynamics. In nanomedicine, computational models have recently garnered attention; such work may help to identify how nanomaterials interact with biological systems and to determine how the efficacy of these nanotherapeutics could be improved. Computational models indicate how NPs are taken up by healthy cells or tumor cells, enabling better predictions regarding the pharmacokinetic and pharmacodynamic properties of these materials.134 For example, Lunnoo et al. used a coarse-grained molecular dynamics (MD) simulation to observe the internalization pathways of various Au nanostructures (nanospheres, nanocages, nanorods, nanoplates, and nanohexapods) into an idealized mammalian plasma membrane.137 Other studies have simulated how different NPs can target tumor cells and deliver drugs.138,139 Therefore, in silico approaches that are currently used for drug repurposing, including molecular docking, molecular dynamics, and computational chemistry, constitute valuable tools to aid preclinical and clinical studies of nanomaterials directed for disease treatment. Considering the urgent need for nanomedicine against the current pandemic, in silico analyses may be especially useful in guiding the rational design of new NP formulations required to fight SARS-CoV-2."}
LitCovid-PD-UBERON
{"project":"LitCovid-PD-UBERON","denotations":[{"id":"T15","span":{"begin":95,"end":112},"obj":"Body_part"},{"id":"T16","span":{"begin":129,"end":133},"obj":"Body_part"},{"id":"T17","span":{"begin":153,"end":159},"obj":"Body_part"},{"id":"T18","span":{"begin":195,"end":201},"obj":"Body_part"},{"id":"T19","span":{"begin":393,"end":397},"obj":"Body_part"},{"id":"T20","span":{"begin":430,"end":435},"obj":"Body_part"},{"id":"T21","span":{"begin":526,"end":530},"obj":"Body_part"},{"id":"T22","span":{"begin":836,"end":840},"obj":"Body_part"},{"id":"T23","span":{"begin":857,"end":861},"obj":"Body_part"},{"id":"T24","span":{"begin":975,"end":979},"obj":"Body_part"},{"id":"T25","span":{"begin":1062,"end":1067},"obj":"Body_part"},{"id":"T26","span":{"begin":5037,"end":5050},"obj":"Body_part"},{"id":"T27","span":{"begin":9625,"end":9629},"obj":"Body_part"},{"id":"T28","span":{"begin":14350,"end":14363},"obj":"Body_part"},{"id":"T29","span":{"begin":14803,"end":14816},"obj":"Body_part"},{"id":"T30","span":{"begin":18546,"end":18551},"obj":"Body_part"},{"id":"T31","span":{"begin":19592,"end":19598},"obj":"Body_part"},{"id":"T32","span":{"begin":19688,"end":19701},"obj":"Body_part"},{"id":"T33","span":{"begin":20245,"end":20251},"obj":"Body_part"},{"id":"T34","span":{"begin":20256,"end":20267},"obj":"Body_part"},{"id":"T35","span":{"begin":20256,"end":20261},"obj":"Body_part"},{"id":"T36","span":{"begin":20336,"end":20347},"obj":"Body_part"},{"id":"T37","span":{"begin":20336,"end":20341},"obj":"Body_part"},{"id":"T38","span":{"begin":20352,"end":20358},"obj":"Body_part"},{"id":"T39","span":{"begin":20359,"end":20365},"obj":"Body_part"},{"id":"T40","span":{"begin":20784,"end":20791},"obj":"Body_part"},{"id":"T41","span":{"begin":20850,"end":20854},"obj":"Body_part"},{"id":"T42","span":{"begin":21714,"end":21727},"obj":"Body_part"},{"id":"T43","span":{"begin":22423,"end":22428},"obj":"Body_part"},{"id":"T44","span":{"begin":22568,"end":22573},"obj":"Body_part"},{"id":"T45","span":{"begin":22862,"end":22867},"obj":"Body_part"},{"id":"T46","span":{"begin":22933,"end":22937},"obj":"Body_part"},{"id":"T47","span":{"begin":24356,"end":24362},"obj":"Body_part"},{"id":"T48","span":{"begin":27079,"end":27085},"obj":"Body_part"},{"id":"T49","span":{"begin":27335,"end":27344},"obj":"Body_part"},{"id":"T50","span":{"begin":27567,"end":27571},"obj":"Body_part"},{"id":"T51","span":{"begin":27583,"end":27589},"obj":"Body_part"},{"id":"T52","span":{"begin":31928,"end":31933},"obj":"Body_part"},{"id":"T53","span":{"begin":34125,"end":34129},"obj":"Body_part"}],"attributes":[{"id":"A15","pred":"uberon_id","subj":"T15","obj":"http://purl.obolibrary.org/obo/UBERON_0000065"},{"id":"A16","pred":"uberon_id","subj":"T16","obj":"http://purl.obolibrary.org/obo/UBERON_0002048"},{"id":"A17","pred":"uberon_id","subj":"T17","obj":"http://purl.obolibrary.org/obo/UBERON_0000062"},{"id":"A18","pred":"uberon_id","subj":"T18","obj":"http://purl.obolibrary.org/obo/UBERON_0002113"},{"id":"A19","pred":"uberon_id","subj":"T19","obj":"http://purl.obolibrary.org/obo/UBERON_0002048"},{"id":"A20","pred":"uberon_id","subj":"T20","obj":"http://purl.obolibrary.org/obo/UBERON_0000062"},{"id":"A21","pred":"uberon_id","subj":"T21","obj":"http://purl.obolibrary.org/obo/UBERON_0002048"},{"id":"A22","pred":"uberon_id","subj":"T22","obj":"http://purl.obolibrary.org/obo/UBERON_0002048"},{"id":"A23","pred":"uberon_id","subj":"T23","obj":"http://purl.obolibrary.org/obo/UBERON_0002048"},{"id":"A24","pred":"uberon_id","subj":"T24","obj":"http://purl.obolibrary.org/obo/UBERON_0002048"},{"id":"A25","pred":"uberon_id","subj":"T25","obj":"http://purl.obolibrary.org/obo/UBERON_0002542"},{"id":"A26","pred":"uberon_id","subj":"T26","obj":"http://purl.obolibrary.org/obo/UBERON_0002405"},{"id":"A27","pred":"uberon_id","subj":"T27","obj":"http://purl.obolibrary.org/obo/UBERON_0002048"},{"id":"A28","pred":"uberon_id","subj":"T28","obj":"http://purl.obolibrary.org/obo/UBERON_0002405"},{"id":"A29","pred":"uberon_id","subj":"T29","obj":"http://purl.obolibrary.org/obo/UBERON_0002405"},{"id":"A30","pred":"uberon_id","subj":"T30","obj":"http://purl.obolibrary.org/obo/UBERON_0000062"},{"id":"A31","pred":"uberon_id","subj":"T31","obj":"http://purl.obolibrary.org/obo/UBERON_0000062"},{"id":"A32","pred":"uberon_id","subj":"T32","obj":"http://purl.obolibrary.org/obo/UBERON_0002405"},{"id":"A33","pred":"uberon_id","subj":"T33","obj":"http://purl.obolibrary.org/obo/UBERON_0002106"},{"id":"A34","pred":"uberon_id","subj":"T34","obj":"http://purl.obolibrary.org/obo/UBERON_0000029"},{"id":"A35","pred":"uberon_id","subj":"T35","obj":"http://purl.obolibrary.org/obo/UBERON_0002391"},{"id":"A36","pred":"uberon_id","subj":"T36","obj":"http://purl.obolibrary.org/obo/UBERON_0000029"},{"id":"A37","pred":"uberon_id","subj":"T37","obj":"http://purl.obolibrary.org/obo/UBERON_0002391"},{"id":"A38","pred":"uberon_id","subj":"T38","obj":"http://purl.obolibrary.org/obo/UBERON_0002106"},{"id":"A39","pred":"uberon_id","subj":"T39","obj":"http://purl.obolibrary.org/obo/UBERON_0000479"},{"id":"A40","pred":"uberon_id","subj":"T40","obj":"http://purl.obolibrary.org/obo/UBERON_0007780"},{"id":"A41","pred":"uberon_id","subj":"T41","obj":"http://purl.obolibrary.org/obo/UBERON_0002048"},{"id":"A42","pred":"uberon_id","subj":"T42","obj":"http://purl.obolibrary.org/obo/UBERON_0002405"},{"id":"A43","pred":"uberon_id","subj":"T43","obj":"http://purl.obolibrary.org/obo/UBERON_0001977"},{"id":"A44","pred":"uberon_id","subj":"T44","obj":"http://purl.obolibrary.org/obo/UBERON_0000178"},{"id":"A45","pred":"uberon_id","subj":"T45","obj":"http://purl.obolibrary.org/obo/UBERON_0000178"},{"id":"A46","pred":"uberon_id","subj":"T46","obj":"http://purl.obolibrary.org/obo/UBERON_0008915"},{"id":"A47","pred":"uberon_id","subj":"T47","obj":"http://purl.obolibrary.org/obo/UBERON_0000479"},{"id":"A48","pred":"uberon_id","subj":"T48","obj":"http://purl.obolibrary.org/obo/UBERON_0000062"},{"id":"A49","pred":"uberon_id","subj":"T49","obj":"http://purl.obolibrary.org/obo/UBERON_0000483"},{"id":"A50","pred":"uberon_id","subj":"T50","obj":"http://purl.obolibrary.org/obo/UBERON_0001456"},{"id":"A51","pred":"uberon_id","subj":"T51","obj":"http://purl.obolibrary.org/obo/UBERON_0000479"},{"id":"A52","pred":"uberon_id","subj":"T52","obj":"http://purl.obolibrary.org/obo/UBERON_0000178"},{"id":"A53","pred":"uberon_id","subj":"T53","obj":"http://purl.obolibrary.org/obo/UBERON_0002048"}],"text":"Nanotechnology Tools to Inactivate SARS-CoV-2 in Patients\nThe main target of SARS-CoV-2 is the respiratory tract (upper airways, lung),36 although other organs might also be infected (e.g., gut, kidney) and vasculature21 also appears to be a prime target. The expression of ACE2 probably determines uptake by different tissues.37\nIn addition to discussing immune-based approaches, because the lung is the most critically affected organ, we will center our discussion on the various options to inactivate the virus in the deep lung and to target the essential host cells for drug delivery. The virus reaches the alveoli and enters alveolar epithelial type II cells (AECII), due to the relatively high abundance of ACE2 and a permissive cellular milieu. These cells serve as a reservoir of the virus, which finally spreads throughout the lung, leading to the lung function impairment seen in severe cases. Airborne nanomaterials are optimally suited to penetrate into the deep lung due to the physicochemical properties of such aerosols, existing on the same size scale particles that penetrate most readily to the deep airways. Hence, nanomedicine is already actively pursuing ideas to deliver drugs, therapeutic proteins, and mRNAs by exploiting nanodevices for pulmonary delivery.38,39\nMoreover, the rapid emergence of SARS-CoV-2 has exposed one of the main weaknesses in the current medical landscape: the lack of broad-spectrum antiviral drugs. At present, there are only a handful of approved antivirals, and they are mostly virus-specific. Hence, when a new virus emerges, little can be done pharmacologically to slow down its spread. Some research efforts have been focused on the development of broad-spectrum drugs, which could potentially offer some efficacy against future emerging viruses (and maybe SARS-CoV-2). The various approaches developed over the years are mainly based on the creation of entry inhibitors.40,41 A highly conserved part in viruses is the attachment ligand (VAL). In most known respiratory viruses,42 the VAL targets either heparan sulfate proteoglycans (HSPG)43 or sialic acids (SA).44 Both HSPG and SA mimics have shown in vitro ability to bind to viruses, blocking their interaction with cell membranes, and often in a broad-spectrum way.45−47\nIn the context of nanomedicine, many nanomaterials have been developed, ranging from polymers48 to dendrimers,49 oligomers, NPs,50 liposomes,51 and small molecules.52 However, successful clinical translation has been hindered by the fact that, upon dilution, these compounds lose efficacy as the virus-compound complex dissociates leaving viruses free to restart their replication cycle. Recently, it has been shown that this limitation can be overcome by synthesizing NPs that, after binding, are able to inhibit viral infectivity irreversibly by permanently damaging the virion, refueling the hope for a true, broad-spectrum antiviral drug.53 Because the focus is also on the development of a drug specific to SARS-CoV-2, a good entry inhibitor could be based on blocking the S spike protein interaction with the cellular ACE2 receptor.19,21−23 Regardless of the specific approach, it is imperative that novel, effective antivirals be based on compounds that exhibit very low or negligible toxicity profiles, as patients will most likely need to receive those drugs for extended periods of time and will already be weakened. For these reasons, when designing antiviral drugs, clearance mechanisms have to be kept in mind. An example of this process is the recent redesign of broad-spectrum antiviral NPs into equally effective modified cyclodextrins.54 Moreover, nanotechnology may offer nanotheranostic approaches to fill the existing gap between diagnostics and therapy.55−57 The simultaneous management of both diagnostics and therapy for those suffering from COVID-19 or in future pandemics, as for many other diseases, is an additional potential strategy to take into consideration in which nanomaterials have proven to be effective tools. The advantages of the capabilities of nanotechnology and nanomaterials for combined therapeutics and diagnostics has been widely explored in cancer research; however, there have been considerable efforts in the past few years to extend the scope of this approach to other areas including infectious diseases.58\n\nNanomaterial-Based Vaccine Development and Immunomodulation\nFollowing the publication of the genetic sequence of SARS-CoV-2 on January 11, 2020, intense research efforts have been devoted to developing a vaccine against COVID-19. With unprecedented speed, this extraordinary scientific mobilization led the first vaccine candidate to enter the Phase I human clinical trial on March 16, 2020, and other novel candidates are rapidly following.59 Up to May 22, 2020, there are 10 COVID-19 candidate vaccines in clinical evaluations and 114 in preclinical development.60\nConcerning vaccine and immunization research, nanomaterials can assist in multiple ways to boost the upregulation required by the immune system and to direct the immune response specifically against antigens. Immune-targeted nanotherapeutics can be developed through their rational manufacture at the nanoscale level by designing nanomaterials that are able to amplify host’s immune response, for instance as adjuvants in the context of vaccination.\nThe development of a vaccine will rely either on the direct administration of viral antigens (e.g., in the form of recombinant proteins, vectored vaccines, or whole inactivated or attenuated virus) or RNA- or DNA-encoding viral antigens.27 Candidate antigens for immunization are surface proteins such as the immunogenic spike protein (S1), which is already targeted by antibodies of convalescent patients.27 Because the S1 protein is also essential for cellular uptake, many researchers are using this protein as the primary target for a vaccine.\nThere are many issues related to the delivery of a drug, protein, or RNA into the patient as the cargo is often degraded, not bioavailable, or is swiftly cleared. Nanotechnology provides multiple solutions to these challenges though, as nanocarriers can overcome some of these limitations.\nBiocompatible polymeric-, lipid-based, or inorganic NPs can be tuned with respect to their physicochemical properties to encapsulate cargo proteins with high loading efficiency, improving protein delivery and pharmacokinetics over conventional approaches.38 Intranasal delivery of polymer-encapsulated antigen triggers a strong immune response, and the success of vaccination depends on the appropriate type of polymer in combination with the antigen.61,62 Similarly, researchers have developed lipid and lipid-based NPs as delivery platforms for mRNAs or siRNAs to enable the synthesis of key viral proteins for vaccination or to inactivate critical viral target genes, respectively.39 The field of therapeutic mRNAs to support vaccination has gained momentum, as well,63 at least in part due to nanotechnology-enabled strategies for cargo delivery. Moreover, the versatility of nanoplatforms as antigen-presenting systems offers great opportunities. Considering the possibility of random viral mutations that may alter the antigen shape, functionalizing nanomaterials with a wide number of molecules at the same time to target the virus, or motifs that are specific for pathogens, will increase the efficiency of vaccines and their ability to prevent viral infection.\nSeveral companies are working on mRNA vaccines encoding SARS-CoV-2 proteins such as the spike protein, encapsulated in nanoliposomes with specific physicochemical properties that are potentially akin to those documented for immunization against certain tumor antigens.64 The design of such nanocarriers, which will need to escape recognition by scavenger cells and to be nontoxic and nonimmunogenic, is a challenge that will require substantial time prior to clinical availability.\nOn March 16, 2020, Moderna, through a partnership with the Vaccine Research Center at the U.S. National Institutes of Health, enrolled the first participants into a Phase I clinical trial testing an mRNA vaccine (mRNA-1273) encapsulated in lipid NPs—a record time of just 63 days following sequence selection (NCT04283461).65 The enrollment of the first cohort of participants (18 to 55-year-old healthy subjects) concluded on April 16, 2020.66 CureVac and BioNTech (in partnership with Pfizer) are currently working on similar vaccines; Pfizer/BioNTech, in particular, have recently started the recruitment in Phase I/II trials (NCT04368728, NCT04380701). A DNA plasmid vaccine by Inovio Pharmaceuticals (INO-4800) has showed promising results in mice and guinea pigs according to a recent article published in Nature Communications(67) and has entered Phase I testing in humans (NCT04336410).\nAnother candidate COVID-19 vaccine for Phase I clinical trial is from the University of Oxford and AstraZeneca (NCT04324606).68 Around 1110 people will take part in the trial, which started recruitment at the end of April 2020. The vaccine is based on a chimpanzee adenovirus vaccine vector (ChAdOx1) and the SARS-CoV-2 spike protein. Chimpanzee adenoviral vectors against different pathogens have been already tested in thousands of subjects with demonstrated safety. To date, ChAdOx1 has been administered to six rhesus macaques exposed to high doses of SARS-CoV-2. The vaccine was unable to prevent infections, although it reduced the severity of the disease: no signs of virus replication were observed in the lungs, with significantly lower levels of respiratory disease and no lung damage compared to control animals, according to a recent article deposited in bioRxiv.69 Another adenoviral vector vaccine developed by CanSino Biological Inc. and Beijing Institute of Biotechnology using a genetically engineered replication-defective adenovirus type 5 vector to express the SARS-CoV-2 spike protein (Ad5-nCoV) is currently being tested in Phase I/II trials (NCT04398147, NCT04341389, NCT0431312).\nAlthough vaccines based on novel DNA and mRNA technologies might be promising, there are no such kinds of vaccines on the market, and it is unknown whether they can be effective in humans. Moderna, for instance, has generated preliminary safety data on different mRNA-based vaccines targeting other respiratory viruses, but their more advanced program (on a cytomegalovirus vaccine) is still in Phase II clinical testing. However, on May 18, Moderna announced that mRNA-1273 elicited antibody titers above the levels observed in convalescent individuals (and therefore considered potentially protective) in all eight initial participants across the 25 and 100 μg dose cohorts of the Phase I trial (NCT04283461).70 The company, after having received a fast-track approval from the FDA, is now moving on Phase II trials.\nConversely, strong evidence exists regarding the efficacy of protein-based vaccines such as recombinant-protein, viral-vector, attenuated, or inactivated vaccines across different infectious diseases, with licensed vaccines already existing for all of these platforms.27 All of the aforementioned approaches are currently being explored in the context of SARS-CoV-2.27 An inactivated vaccine developed by the China National Pharmaceutical Group (Sinopharm), in collaboration with the Wuhan Institute of Biological Products, is currently tested in a Phase I/II trial (ChiCTR2000031809), and a second inactivated vaccine (in collaboration with the Beijing Institute of Biological Products) has been currently approved for clinical testing (ChiCTR2000032459). A third aluminum salt (alum) adjuvanted inactivated vaccine, developed by Beijing-based Sinovac Biotech’s, was able to provide partial or complete protection in rhesus macaques, according to a recent article published in Science,71 and is currently being tested in Phase I/II trials (NCT04383574, NCT04352608).\nLive-attenuated vaccines are intrinsically immunogenic (e.g., due to the presence of viral DNA), but extensive safety tests are required due to the rare possibility of reversion to a pathogenic form able to cause infection. Vaccine candidates based in this application have previously been designed for SARS-CoV with high stability.72,73 Recombinant-protein vaccines and inactivated vaccines are safer but might require adjuvants to increase their immunogenicity. In the context of SARS-CoV-2, adjuvants are important for two reasons. First, adjuvants might increase the efficacy of the vaccine, especially in subjects with impaired immunological function, such as the elderly, or in subjects with comorbidities resulting in immune dysfunctions; in these patient cohorts, SARS-CoV-2 has a high lethality rate. Second, adjuvants can reduce the amount of vaccine protein(s) required per dose, which could facilitate scaling-up vaccine production in a reduced time frame.\nBeyond alum, which is in fact a nanoscale material74 that was developed in the 1920s for the tetanus and diphtheria toxoids,75 approval for a new adjuvant did not occur until 1997, with the introduction of the oil-in-water emulsion of squalene oil and polysorbate 80 and sorbitan trioleate surfactants (MF59) in the seasonal influenza vaccine for the elderly.76 The use of MF59 was further expanded to pandemic and avian influenza vaccines.77 Other adjuvants in licensed vaccines have been approved since 2000, as well: (a) AS03 (used for pandemic and avian influenza vaccines), similar to MF59, but including α-tocopherol as an additional immune stimulant; (b) AF 03 (used for pandemic influenza vaccines), an alternative squalene emulsion containing polyoxyethylene, cetostearyl ether, mannitol, and orbitan oleate;78 (c) AS 01 (used for herpes zoster vaccine), a liposome-based vaccine adjuvant system containing two immunostimulants, 3-O-desacyl-4′-monophosphoryl lipid A (MPL, a Toll-like receptor 4 agonist) and saponin QS-21, which activates the ACT-NLRP3 inflammasome pathway;79 and (d) AS04 (used for hepatitis B and human papilloma virus vaccines), which is a combination of MPL and aluminum hydroxide.76,80\nIn this context, the concept of “nanoimmunity by design” relies on the rational design of distinct physicochemical properties and specific functionalization of nanomaterials intended for fine-tuning their potential effects on the immune system.81 Nanomaterials have emerged as promising tools for immune modulation, either stimulating or suppressing the immune response. In fighting SARS-CoV-2, these properties may find applications for both prevention and therapy and in the context of vaccine development.\nMounting evidence indicates that nanomaterials such as graphene,82 nanodiamonds,83 carbon nanotubes,84 and polystyrene particles85 bear an intrinsic capacity to activate the immune system, depending on their functionalization.86 For instance, graphene oxide functionalized with amino groups (GO-NH2) induces activation of STAT1/IRF1 interferon signaling in monocytes and T cells, resulting in the production of T cell chemoattractants, and macrophage 1 (M1) 1/T-helper 1 (Th1) polarization of the immune response, with negligible toxicity.82 Remarkably, the ability of licensed adjuvants such as AS01 and AS03 to enhance adaptive immunity has been linked to their capacity to boost STAT1/IRF1 interferon signaling.87 In addition, recent SARS-CoV and MERS-CoV studies suggest that the development of a Th1-type response is central for controlling infection, which also may be true for SARS-CoV-2.88\nSeveral groups and consortia have been screening and characterizing nanomaterials according to their immunomodulatory properties and absence of cytotoxicity.56,57,82−84,86 The development of an adjuvant for clinical use is a lengthy process that requires extensive Phase III randomized trials in large and diverse cohorts of subjects, and the process generally requires several years.76 Pharmaceutical giants such as GlaxoSmithKline (GSK), which owns the ASs mentioned above and other adjuvant platforms, are engaged in several partnerships to embed their adjuvant systems with SARS-CoV-2-protein-based vaccines. A full-length recombinant SARS-CoV-2 glycoprotein nanoparticle vaccine adjuvanted with the saponin-based Matrix M developed by Novavax is in Phase I clinical testing (NCT04368988).89\nAlthough it is unlikely that novel adjuvants would be used in the context of the current pandemic, the SARS-CoV-2 pandemic offers an opportunity to reflect on the potential of nanotechnology for vaccine adjuvant development. In this context, it is critical to stream coherent pipelines covering in vitro and in vivo experiments specifically to select candidate materials that might be tested for clinical implementation as vaccine adjuvants.90\nIn this view, the status of nanomaterial-based vaccine adjuvants has been reviewed.91,92 In particular, immunomodulatory effects induced on the innate immune signaling have been demonstrated.91 For example, nanomaterials such as GO can elicit an inflammasome sensor (NLRP3)-dependent expression of IL-1β in macrophages.93 Notably, alum, the most commonly used adjuvant in human vaccines, induces the release of this cytokine in macrophages through the same NRLP-induced mechanism. These results suggest that nanomaterials such as GO and alum may be useful for medical applications.94\nConsidering that new vaccine development typically requires years to be approved and applied to the general population, there is also an urgent need to study how to improve treatment approaches. According to the first Phase III clinical studies, the antiviral drug Remdesivir, which was recently approved by the FDA for COVID-19 treatment in the United States, seems to be a promising treatment for adults diagnosed with COVID-19. Nano-based strategies have already been applied to enhance the effectiveness of Remdesivir in the context of other emerging viral infections (Nipah virus),95 suggesting the suitability of nanotechnology to assist with similar strategies for the treatment of COVID-19 as well as other possible pandemics in the future.96\n\nCan Nanotechnology Help to Control the Cytokine Storm?\nOne of the main features of COVID-19 is the triggering of a cytokine storm in the body, also known as cytokine release syndrome (CRS), which results from an excessive immune response and leads to the severe deterioration of patient health.25,97,98 This inflammatory storm is one of the major causes of the acute respiratory distress syndrome (ARDS) that is often associated with multiple-organ failure, representing the leading causes of death in critical patients.98 In particular, the role of interleukin (IL)-6 has been highlighted in patients requiring assisted ventilation. Ongoing clinical trials are testing drugs that block the receptor of IL-6 (Tocilizumab, an anti-IL-6 receptor antibody, and Sarilumab) or IL-6 itself (Siltuximab).\nWhereas a well-regulated cytokine response that is rapidly triggered by the host’s innate immunity can serve to prevent and to counteract an infection, an excessive, unbalanced, prolonged immune response can seriously harm the body. Therefore, therapeutic strategies aimed at effectively suppressing the cytokine storm are under investigation. Nanomaterials have been exploited to adjust the immune response to an optimized level, and such proprieties might be explored to inhibit cytokine releases.99 Nanosystems can enhance the specificity/efficiency of immunosuppressant delivery to target immune cells, with consequent reductions in drug dose, drug distribution to nontarget tissues and organs, and possible side effects. In addition, specific nanotools can be designed to evade the immune system and to enhance the solubility of poorly soluble immunosuppressant agents; the potential of finely tuning their surface charge opens possibilities for encapsulation strategies and offers accommodation for a high drug load. All of these mechanisms may also occur simultaneously, enhancing the activity of immunosuppressive agents.\nConcerning the role of macrophages in COVID-19, the presence of ACE2-expressing CD68+CD169+ macrophages containing SARS-CoV-2 nucleoprotein antigen and showing an increased release of IL-6 was observed in infected spleen and lymph nodes.100 Notably, immunohistochemical and immunofluorescence analyses of lymph nodes and spleen tissue from autopsy samples of patients who died from COVID-19 revealed lymphocytic apoptosis. The tissues infected by SARS-CoV-2 also showed an upregulated expression of Fas, suggesting a role for CD169+ macrophages in viral spreading, aberrant inflammation, and activation-induced lymphocyte apoptosis. Moreover, histological examinations of biopsy samples of patients who died from COVID-19 revealed an increased alveolar exudate due to the extended neutrophil and monocyte infiltrate in lung capillaries with fibrin deposition, probably leading to difficulties in gas exchange. Through nanomedicine, we envision therapeutic approaches aimed at targeting specific immune subpopulations to avoid these complications, and different nanomaterials have already been explored for their specific impact on different immune cell subpopulations.82,83,86 Octadecylamine-functionalized and dexamethasone-adsorbed nanodiamond promotes anti-inflammatory and proregenerative behavior in human macrophages in vitro.101 A low dose of this functionalized nanodiamond also reduced macrophage infiltration and expression of proinflammatory mediators iNOS and tumor necrosis factor (TNF)-α in mice. Overall, these results suggest that nanodiamond particles may be useful as an inherently immunomodulatory platform.\nFinally, the indiscriminate systemic suppression of the immune system may increase the susceptibility to sepsis due to underlying secondary bacterial infections that can exacerbate critical conditions in patients, causing death.102 Risk factors for mortality include acquired bacterial infections by opportunistic organisms and primary pathogens. Using nanocarriers to achieve targeted delivery of immunosuppressive drugs, or the specific and controlled inhibition of only a specific immune cell subpopulation, can play a critical role in limiting such complications.\nThe fast and complete removal of pro-inflammatory cytokines from the bloodstream has been shown to increase survival significantly in patients with early stage sepsis. Adsorption of cytokines from serum by extracorporeal perfusion through porous carbons is one of the most promising ways for their selective, quick, and complete removal from blood circulation. Hierarchical carbon materials with tuned porosity have shown effective adsorption of many cytokines, including IL-6 and even the largest ones, such as TNF-α.103 Based on their demonstrated outstanding performance in removing sepsis-associated pro-inflammatory cytokines from blood, mesoporous carbon adsorbents with hierarchical structure, tuned pore size, and surface chemistry, as well as graphene with open and accessible surfaces104 have significant potential to quickly remove inflammatory cytokines associated with CRS and to prevent mortality arising from an uncontrolled inflammatory cascade, thereby providing enough time for the defense mechanisms of the human body to fight the virus.\n\nPhotodynamic Inactivation of SARS-CoV-2\nIn addition to drug- and vaccine-based antiviral strategies, photodynamic therapy (PDT) stands as a unique approach to inactivate SARS-CoV-2. Using a light-based method, PDT attacks target cells via the excitation of photosensitive agents, called photosensitizers (PSs), with radiation characterized by a wavelength corresponding to its absorption spectrum to generate reactive oxygen species (ROS) in the presence of oxygen, which ultimately results in cell death. Photodynamic therapy is primarily used for the clinical treatment of various oncological disorders.105 It was not until the 1970s that PDT was first used clinically against viruses,106 exploiting ROS production to damage virus proteins, nucleic acids, and—if present—lipids.107 Even though there are research efforts for PDT-based virotherapies against different viruses, including herpes simplex virus, human papilloma virus, and human immunodeficiency virus,106−109 clinical use of PDT is limited due to hydrophobicity of PSs, poor target specificity, and limited tissue penetration ability.\nMost PSs are hydrophobic and aggregate in aqueous solutions, affecting their photochemical and photobiological properties.106,110 For this reason, Lim et al. have proposed a promising approach for photodynamic inactivation of viruses with NPs, developing sodium yttrium fluoride (NaYF4) upconversion NPs (UCNs) with zinc phthalocyanine PSs grafted onto their surfaces. Unlike most PSs, these UCNs are coated with polyethylenimine (PEI), which render them hydrophilic and easier to manipulate. These UCNs showed antiviral activity against Dengue virus serotype 2 and adenovirus type 5, which were used as models of enveloped and non-enveloped viruses, respectively.110 MXenes111,112 are a large family of 2D transition metal carbides, nitrides,113 and carbonitrides114 that exhibit unique electronic, optical, and catalytic properties. They have the general formula Mn+1XnTx, where M is an early transition metal (Ti, Zr, V, Mo, etc.), X is C and/or N, Tx represents the surface functional groups (=O, −OH, −F, −Cl), and n = 1–4.115,116 Some examples include Ti3C2Tx, V2CTx, and Nb2CTx, with over 30 stoichiometric compositions already synthesized with more than 100 predicted. Biocompatible MXenes, such as Ti3C2Tx, are hydrophilic and are among the most efficient light-to-heat transforming materials.117 The plasmon resonance extinction maxima of Ti3C2Tx is at 780 nm, enabling the use of near-infrared (IR) light for PDT. Several other MXenes have absorption maxima in the IR range and have shown outstanding performance in PDT and theranostic applications.118\nFullerene and graphene are also good candidates for virus inactivation by PDT and have proved to be effective against Semliki Forest virus (SFV), vesicular stomatitis virus (VSV), HSV-1, HIV-1, mosquito iridovirus (MIV), and influenza A virus (IAV), as well as the phage MS2.107 In addition, several 2D nanomaterials, including graphene-based materials, MXenes, black phosphorus, graphitic carbon nitride, tungsten disulfide, and molybdenum disulfide, have been reported to improve the efficacy of PDT considerably for cancer treatment.56,57 Therefore, determining if such nanomaterial-based PDT protocols could be exploited to inactivate SARS-CoV-2 is of great interest.\n\nBiomimetic Engineering of Nanodelivery Systems: Artificial Viruses in the Making\nIn an effort to engineer the next generation of nanoscale vectors, scientists have moved from using inorganic components aimed at obtaining inert structures to utilizing biological building blocks that are able to convey additional functionalities to the resulting construct. To cope with the complexity of the body and to evade the multiple layers of defense that tissues and organs have, it is critical to rely on the ability of certain materials to interact with, rather than to eschew, the biology of our body. Every NP system conceived to date faces one common fate: whether injected, inhaled, ingested, or absorbed through our epithelia, all will at some point come into contact with the mixture of fluids and organic compounds that comprise the body. Under such conditions, every material reacts in a unique way according to the conditions they individually face (i.e., the tissue or body region they are in), their composition (i.e., organic or inorganic), and their physical properties (i.e., size, shape, surface charge).119 Inorganic NPs can function as globular protein mimics because of their similar size, charge, shape, and surface features that can be chemically functionalized to resemble proteins. These similarities can be used in biotechnology to control virus pathways or cell receptor interactions with NPs.120\nAmong the many attempts, the legendary accomplishments, and the epic failures, we could list thousands of different NPs, differing from one another thanks to the creative endeavors of their designers. Despite their remarkable differences and researchers’ endeavors to make them one of a kind, they all aim to achieve one goal: to deliver in a specific way one particular form of payload, while remaining as unnoticed as possible by the body’s defense mechanisms. For everyone who has made an effort to create their own version of such a silver bullet, it has come to mind that nature in its incredible variety had already invented a few ingenious solutions to this problem. In the world of nano-based drug delivery, one entity dominates as the quintessential example of precision, efficiency, and stealth: the virus. Not by chance, these two worlds share many features that span from the physical laws that govern their assembly and stability to the chemical similarities in their overall composition.121\nHistorically, the field of nanomedicine and the global endeavor to generate NPs for drug delivery arose from one of the greatest struggles in medicine: gene therapy. At the core of that approach was the idea that viruses could be used as Trojan horses to deliver the correct sequence of the gene into the cells that harbored the mutated copy. In order to identify the virus that offered the best delivery service, a cadre of viral strains and species were tested, adapted, and engineered to fit the aims and hit the targets. It took decades of trial and error, of progressive adjustments, and a few tragic mistakes to identify the viruses that could provide the ideal backbone to develop viral vectors able to ferry genetic cargo into the target cell.122 In the midst of that global challenge, nanotechnology offered a safer and more controllable alternative: to generate bespoke structures that could replace viral vectors and do the same job, delivering a payload from the point of injection to the site of action.\nFast forward a few decades, and the promises made by both worlds seem to have finally become reality, with a number of active clinical trials, some clinical success stories, and a few commercial products in the market space. Interestingly, the two worlds seem to have maintained a safe distance so as to avoid any collision or dangerous proximity. Exchange of ideas between the fields of gene therapy and nanomedicine is limited, and the potential for disciplinary growth through knowledge exchange has remained elusive to scientists in both fields.5 Many scientists have suggested that nanotechnologists could find inspiration in the mechanisms devised by viruses to elude immune surveillance, to overcome biological barriers, and to deliver their genetic payload with high specificity. Similarly, gene therapists would find high-tech solutions to their scalability and safety issues in looking at the new generations of biomimetic particles being generated.\nThe principles of biomimicry and bioinspiration have been used to design and to engineer drug-delivery technologies that reproduce or recapitulate biological materials, for what pertains to not only their structure and chemistry but also, more importantly, their functions. In drug delivery, surface recognition and nanoscale interactions between materials and biological entities are key to the success of the delivery strategy, and the use of biological building blocks such as membrane proteins has been proposed as a way to convey targeting and shielding moieties simultaneously.123 One can only hope that the recent events and global attention that viruses are capturing in the scientific world will spur a renewed interest in finding ways to adapt viral features and mechanisms of action to the world of NPs. Increased focus in this field would be useful to create virus-like NPs able to circulate in the blood system, overcoming the endothelial barrier, and to deliver their therapeutic payload with high efficiency.124\n\nInterference with Cellular Uptake, Immobilization, and Inactivation of the Virus Outside of the Host Cell\nNanomaterials can be synthesized with a high specific surface area of a few hundred square meters per gram. Therefore, dependent on the surface properties, nanomaterials efficiently adsorb biomolecules and form a so-called biomolecular corona. This passive, nontargeted adsorption might be utilized to bind viruses, provided that the selected nanomaterial is relatively biocompatible. Viral surface proteins are often modified by sugar moieties or encompass positively charged amino acid patches that bind to lectins or glycosaminoglycans (GAGs) of heparan sulfate (HS), respectively.32 Robust interactions of virus particles with these host receptors is ensured by multivalent binding, which is likely why single-molecule inhibitors often are not capable of efficiently perturbing this key event but multivalent NPs are superior to block binding of different viruses to the host cell.125\nGold NPs capped with mercaptoethanesulfonate are effective inhibitors of HSV type 1 infection as they mimic cell-surface-receptor heparan sulfate and, therefore, competitively bind to the virus. Interestingly, polyvalent sulfated Au NPs inhibit virus binding to the host cell dependent on their size. Nanoparticles of diameters equal to and larger than the virus diameter (in this case, the stomatitis virus) more efficiently inhibit the binding to cells than smaller particles. Most likely, larger NPs efficiently cross-link virions, whereas smaller NPs simply decorate the viral surface.126 Papp et al. found that gold NPs decorated with SA effectively inhibited the binding of influenza virus to the target cells. In this case, viral recognition via its surface protein hemagglutinin of SA on the host cell membrane was a prerequisite for cellular entry.127 More recently, Cagno et al. reported antiviral NPs (Au and iron oxide core) with long and flexible linkers mimicking HS that strongly bind and inactivate viruses such as respiratory syncytial virus in vitro and in vivo in a lung infection model and even led to irreversible viral deformation.53 Hence, there is ample evidence that biocompatible, functionalized NPs can act as broad-spectrum antivirals. Notably, the receptor-binding domain of the spike S1 protein of SARS-CoV-2 binds not only to ACE2 but also to heparin128 and, thus, might be targeted by similar approaches as outlined above.\nOne of the most recent strategies to inhibit viral uptake is based on administration of recombinant ACE2 to inhibit binding competitively via the spike S1 protein.21 Knowing that multivalency is key to block virus–host interactions reliably, researchers have speculated that a nanostructured carrier could not only improve delivery and cargo stability but also might dramatically enhance binding strength.129\n\nSpeeding up the Nanomedicine-Based Approaches for COVID-19 by In Silico Analysis\nCurrently, repurposing drug molecules is a key strategy for identifying approved or investigational drugs outside the scope of the original medical indication that can be used to fight COVID-19.130,131 There are various advantages to this strategy, including already-established safety profiles, fast transition to clinical studies, and less investment needed, compared to the process of developing an entirely new drug.132,133 Therefore, these advantages have the potential to result in less risky and more rapid returns on investment in the development of repurposed drugs, benefits that are particularly important during pandemics. In addition to rapid diagnosis, making recommendations to physicians about rapid treatment methods can save the lives of many people. Combining in silico tools such as molecular docking, molecular dynamics, and computational chemistry with large drug databases provides a great advantage in selecting “possible candidates” from among thousands of pharmaceutically active substances (Figure 3). In silico analyses should be supported by the literature, expert opinions (pharmacologists and clinicians), and, when possible, preclinical and clinical findings. After selecting the best candidates following in silico analyses, detailed in vitro and in vivo experimentation should be undertaken prior to clinical studies. Recently, various drug-repurposing studies have been published to assist in the global COVID-19 pandemic response.134−136 Such studies aim to identify whether already-approved drugs have the capacity to interact with viral proteins or receptors on host cells.\nFigure 3 Schematic of how in silico analysis can be used to select candidate drug molecules for clinical studies. MD, molecular dynamics. In nanomedicine, computational models have recently garnered attention; such work may help to identify how nanomaterials interact with biological systems and to determine how the efficacy of these nanotherapeutics could be improved. Computational models indicate how NPs are taken up by healthy cells or tumor cells, enabling better predictions regarding the pharmacokinetic and pharmacodynamic properties of these materials.134 For example, Lunnoo et al. used a coarse-grained molecular dynamics (MD) simulation to observe the internalization pathways of various Au nanostructures (nanospheres, nanocages, nanorods, nanoplates, and nanohexapods) into an idealized mammalian plasma membrane.137 Other studies have simulated how different NPs can target tumor cells and deliver drugs.138,139 Therefore, in silico approaches that are currently used for drug repurposing, including molecular docking, molecular dynamics, and computational chemistry, constitute valuable tools to aid preclinical and clinical studies of nanomaterials directed for disease treatment. Considering the urgent need for nanomedicine against the current pandemic, in silico analyses may be especially useful in guiding the rational design of new NP formulations required to fight SARS-CoV-2."}
LitCovid-PD-MONDO
{"project":"LitCovid-PD-MONDO","denotations":[{"id":"T57","span":{"begin":35,"end":43},"obj":"Disease"},{"id":"T58","span":{"begin":77,"end":85},"obj":"Disease"},{"id":"T59","span":{"begin":1320,"end":1328},"obj":"Disease"},{"id":"T60","span":{"begin":1811,"end":1819},"obj":"Disease"},{"id":"T61","span":{"begin":2993,"end":3001},"obj":"Disease"},{"id":"T62","span":{"begin":3846,"end":3854},"obj":"Disease"},{"id":"T63","span":{"begin":4169,"end":4175},"obj":"Disease"},{"id":"T64","span":{"begin":4316,"end":4326},"obj":"Disease"},{"id":"T65","span":{"begin":4453,"end":4461},"obj":"Disease"},{"id":"T66","span":{"begin":4560,"end":4568},"obj":"Disease"},{"id":"T67","span":{"begin":4817,"end":4825},"obj":"Disease"},{"id":"T68","span":{"begin":7448,"end":7463},"obj":"Disease"},{"id":"T69","span":{"begin":7454,"end":7463},"obj":"Disease"},{"id":"T70","span":{"begin":7521,"end":7529},"obj":"Disease"},{"id":"T71","span":{"begin":7718,"end":7723},"obj":"Disease"},{"id":"T72","span":{"begin":8860,"end":8868},"obj":"Disease"},{"id":"T73","span":{"begin":9151,"end":9159},"obj":"Disease"},{"id":"T74","span":{"begin":9398,"end":9406},"obj":"Disease"},{"id":"T75","span":{"begin":9444,"end":9454},"obj":"Disease"},{"id":"T76","span":{"begin":9598,"end":9617},"obj":"Disease"},{"id":"T77","span":{"begin":9923,"end":9931},"obj":"Disease"},{"id":"T78","span":{"begin":9949,"end":9952},"obj":"Disease"},{"id":"T79","span":{"begin":11045,"end":11055},"obj":"Disease"},{"id":"T80","span":{"begin":11220,"end":11228},"obj":"Disease"},{"id":"T81","span":{"begin":12146,"end":12155},"obj":"Disease"},{"id":"T82","span":{"begin":12236,"end":12244},"obj":"Disease"},{"id":"T83","span":{"begin":12415,"end":12423},"obj":"Disease"},{"id":"T84","span":{"begin":12658,"end":12677},"obj":"Disease"},{"id":"T85","span":{"begin":12705,"end":12713},"obj":"Disease"},{"id":"T86","span":{"begin":12995,"end":13002},"obj":"Disease"},{"id":"T87","span":{"begin":13007,"end":13017},"obj":"Disease"},{"id":"T88","span":{"begin":13227,"end":13236},"obj":"Disease"},{"id":"T89","span":{"begin":13317,"end":13332},"obj":"Disease"},{"id":"T90","span":{"begin":13323,"end":13332},"obj":"Disease"},{"id":"T91","span":{"begin":13454,"end":13469},"obj":"Disease"},{"id":"T92","span":{"begin":13460,"end":13469},"obj":"Disease"},{"id":"T93","span":{"begin":13589,"end":13598},"obj":"Disease"},{"id":"T94","span":{"begin":13742,"end":13755},"obj":"Disease"},{"id":"T95","span":{"begin":14012,"end":14023},"obj":"Disease"},{"id":"T96","span":{"begin":14012,"end":14021},"obj":"Disease"},{"id":"T97","span":{"begin":14034,"end":14043},"obj":"Disease"},{"id":"T98","span":{"begin":14503,"end":14511},"obj":"Disease"},{"id":"T99","span":{"begin":15366,"end":15374},"obj":"Disease"},{"id":"T100","span":{"begin":15475,"end":15484},"obj":"Disease"},{"id":"T101","span":{"begin":15513,"end":15521},"obj":"Disease"},{"id":"T102","span":{"begin":16105,"end":16113},"obj":"Disease"},{"id":"T103","span":{"begin":16166,"end":16174},"obj":"Disease"},{"id":"T104","span":{"begin":16426,"end":16434},"obj":"Disease"},{"id":"T105","span":{"begin":17671,"end":17679},"obj":"Disease"},{"id":"T106","span":{"begin":17772,"end":17780},"obj":"Disease"},{"id":"T107","span":{"begin":17906,"end":17922},"obj":"Disease"},{"id":"T108","span":{"begin":18040,"end":18048},"obj":"Disease"},{"id":"T109","span":{"begin":18186,"end":18194},"obj":"Disease"},{"id":"T110","span":{"begin":18287,"end":18290},"obj":"Disease"},{"id":"T112","span":{"begin":18464,"end":18499},"obj":"Disease"},{"id":"T113","span":{"begin":18470,"end":18499},"obj":"Disease"},{"id":"T114","span":{"begin":18501,"end":18505},"obj":"Disease"},{"id":"T115","span":{"begin":18537,"end":18559},"obj":"Disease"},{"id":"T116","span":{"begin":19042,"end":19051},"obj":"Disease"},{"id":"T117","span":{"begin":20069,"end":20077},"obj":"Disease"},{"id":"T118","span":{"begin":20146,"end":20154},"obj":"Disease"},{"id":"T119","span":{"begin":20413,"end":20421},"obj":"Disease"},{"id":"T120","span":{"begin":20478,"end":20486},"obj":"Disease"},{"id":"T121","span":{"begin":20605,"end":20617},"obj":"Disease"},{"id":"T122","span":{"begin":20744,"end":20752},"obj":"Disease"},{"id":"T123","span":{"begin":21503,"end":21508},"obj":"Disease"},{"id":"T124","span":{"begin":21798,"end":21818},"obj":"Disease"},{"id":"T125","span":{"begin":21934,"end":21954},"obj":"Disease"},{"id":"T126","span":{"begin":23108,"end":23111},"obj":"Disease"},{"id":"T128","span":{"begin":23313,"end":23321},"obj":"Disease"},{"id":"T129","span":{"begin":23454,"end":23462},"obj":"Disease"},{"id":"T130","span":{"begin":23541,"end":23555},"obj":"Disease"},{"id":"T131","span":{"begin":24172,"end":24186},"obj":"Disease"},{"id":"T132","span":{"begin":24200,"end":24209},"obj":"Disease"},{"id":"T133","span":{"begin":24227,"end":24243},"obj":"Disease"},{"id":"T134","span":{"begin":24922,"end":24928},"obj":"Disease"},{"id":"T135","span":{"begin":26094,"end":26114},"obj":"Disease"},{"id":"T136","span":{"begin":26104,"end":26114},"obj":"Disease"},{"id":"T137","span":{"begin":26173,"end":26182},"obj":"Disease"},{"id":"T138","span":{"begin":26467,"end":26473},"obj":"Disease"},{"id":"T139","span":{"begin":26587,"end":26595},"obj":"Disease"},{"id":"T140","span":{"begin":33124,"end":33133},"obj":"Disease"},{"id":"T141","span":{"begin":33431,"end":33441},"obj":"Disease"},{"id":"T142","span":{"begin":33720,"end":33729},"obj":"Disease"},{"id":"T143","span":{"begin":34130,"end":34139},"obj":"Disease"},{"id":"T144","span":{"begin":34368,"end":34376},"obj":"Disease"},{"id":"T145","span":{"begin":34955,"end":34963},"obj":"Disease"},{"id":"T146","span":{"begin":36425,"end":36433},"obj":"Disease"},{"id":"T147","span":{"begin":37041,"end":37046},"obj":"Disease"},{"id":"T148","span":{"begin":37490,"end":37495},"obj":"Disease"},{"id":"T149","span":{"begin":37990,"end":37998},"obj":"Disease"}],"attributes":[{"id":"A57","pred":"mondo_id","subj":"T57","obj":"http://purl.obolibrary.org/obo/MONDO_0005091"},{"id":"A58","pred":"mondo_id","subj":"T58","obj":"http://purl.obolibrary.org/obo/MONDO_0005091"},{"id":"A59","pred":"mondo_id","subj":"T59","obj":"http://purl.obolibrary.org/obo/MONDO_0005091"},{"id":"A60","pred":"mondo_id","subj":"T60","obj":"http://purl.obolibrary.org/obo/MONDO_0005091"},{"id":"A61","pred":"mondo_id","subj":"T61","obj":"http://purl.obolibrary.org/obo/MONDO_0005091"},{"id":"A62","pred":"mondo_id","subj":"T62","obj":"http://purl.obolibrary.org/obo/MONDO_0100096"},{"id":"A63","pred":"mondo_id","subj":"T63","obj":"http://purl.obolibrary.org/obo/MONDO_0004992"},{"id":"A64","pred":"mondo_id","subj":"T64","obj":"http://purl.obolibrary.org/obo/MONDO_0005550"},{"id":"A65","pred":"mondo_id","subj":"T65","obj":"http://purl.obolibrary.org/obo/MONDO_0005091"},{"id":"A66","pred":"mondo_id","subj":"T66","obj":"http://purl.obolibrary.org/obo/MONDO_0100096"},{"id":"A67","pred":"mondo_id","subj":"T67","obj":"http://purl.obolibrary.org/obo/MONDO_0100096"},{"id":"A68","pred":"mondo_id","subj":"T68","obj":"http://purl.obolibrary.org/obo/MONDO_0005108"},{"id":"A69","pred":"mondo_id","subj":"T69","obj":"http://purl.obolibrary.org/obo/MONDO_0005550"},{"id":"A70","pred":"mondo_id","subj":"T70","obj":"http://purl.obolibrary.org/obo/MONDO_0005091"},{"id":"A71","pred":"mondo_id","subj":"T71","obj":"http://purl.obolibrary.org/obo/MONDO_0005070"},{"id":"A72","pred":"mondo_id","subj":"T72","obj":"http://purl.obolibrary.org/obo/MONDO_0100096"},{"id":"A73","pred":"mondo_id","subj":"T73","obj":"http://purl.obolibrary.org/obo/MONDO_0005091"},{"id":"A74","pred":"mondo_id","subj":"T74","obj":"http://purl.obolibrary.org/obo/MONDO_0005091"},{"id":"A75","pred":"mondo_id","subj":"T75","obj":"http://purl.obolibrary.org/obo/MONDO_0005550"},{"id":"A76","pred":"mondo_id","subj":"T76","obj":"http://purl.obolibrary.org/obo/MONDO_0005087"},{"id":"A77","pred":"mondo_id","subj":"T77","obj":"http://purl.obolibrary.org/obo/MONDO_0005091"},{"id":"A78","pred":"mondo_id","subj":"T78","obj":"http://purl.obolibrary.org/obo/MONDO_0011194"},{"id":"A79","pred":"mondo_id","subj":"T79","obj":"http://purl.obolibrary.org/obo/MONDO_0005550"},{"id":"A80","pred":"mondo_id","subj":"T80","obj":"http://purl.obolibrary.org/obo/MONDO_0005091"},{"id":"A81","pred":"mondo_id","subj":"T81","obj":"http://purl.obolibrary.org/obo/MONDO_0005550"},{"id":"A82","pred":"mondo_id","subj":"T82","obj":"http://purl.obolibrary.org/obo/MONDO_0005091"},{"id":"A83","pred":"mondo_id","subj":"T83","obj":"http://purl.obolibrary.org/obo/MONDO_0005091"},{"id":"A84","pred":"mondo_id","subj":"T84","obj":"http://purl.obolibrary.org/obo/MONDO_0005046"},{"id":"A85","pred":"mondo_id","subj":"T85","obj":"http://purl.obolibrary.org/obo/MONDO_0005091"},{"id":"A86","pred":"mondo_id","subj":"T86","obj":"http://purl.obolibrary.org/obo/MONDO_0005526"},{"id":"A87","pred":"mondo_id","subj":"T87","obj":"http://purl.obolibrary.org/obo/MONDO_0005504"},{"id":"A88","pred":"mondo_id","subj":"T88","obj":"http://purl.obolibrary.org/obo/MONDO_0005812"},{"id":"A89","pred":"mondo_id","subj":"T89","obj":"http://purl.obolibrary.org/obo/MONDO_0018695"},{"id":"A90","pred":"mondo_id","subj":"T90","obj":"http://purl.obolibrary.org/obo/MONDO_0005812"},{"id":"A91","pred":"mondo_id","subj":"T91","obj":"http://purl.obolibrary.org/obo/MONDO_0018695"},{"id":"A92","pred":"mondo_id","subj":"T92","obj":"http://purl.obolibrary.org/obo/MONDO_0005812"},{"id":"A93","pred":"mondo_id","subj":"T93","obj":"http://purl.obolibrary.org/obo/MONDO_0005812"},{"id":"A94","pred":"mondo_id","subj":"T94","obj":"http://purl.obolibrary.org/obo/MONDO_0005609"},{"id":"A95","pred":"mondo_id","subj":"T95","obj":"http://purl.obolibrary.org/obo/MONDO_0005344"},{"id":"A96","pred":"mondo_id","subj":"T96","obj":"http://purl.obolibrary.org/obo/MONDO_0002251"},{"id":"A97","pred":"mondo_id","subj":"T97","obj":"http://purl.obolibrary.org/obo/MONDO_0002363"},{"id":"A98","pred":"mondo_id","subj":"T98","obj":"http://purl.obolibrary.org/obo/MONDO_0005091"},{"id":"A99","pred":"mondo_id","subj":"T99","obj":"http://purl.obolibrary.org/obo/MONDO_0005091"},{"id":"A100","pred":"mondo_id","subj":"T100","obj":"http://purl.obolibrary.org/obo/MONDO_0005550"},{"id":"A101","pred":"mondo_id","subj":"T101","obj":"http://purl.obolibrary.org/obo/MONDO_0005091"},{"id":"A102","pred":"mondo_id","subj":"T102","obj":"http://purl.obolibrary.org/obo/MONDO_0005091"},{"id":"A103","pred":"mondo_id","subj":"T103","obj":"http://purl.obolibrary.org/obo/MONDO_0005091"},{"id":"A104","pred":"mondo_id","subj":"T104","obj":"http://purl.obolibrary.org/obo/MONDO_0005091"},{"id":"A105","pred":"mondo_id","subj":"T105","obj":"http://purl.obolibrary.org/obo/MONDO_0100096"},{"id":"A106","pred":"mondo_id","subj":"T106","obj":"http://purl.obolibrary.org/obo/MONDO_0100096"},{"id":"A107","pred":"mondo_id","subj":"T107","obj":"http://purl.obolibrary.org/obo/MONDO_0005108"},{"id":"A108","pred":"mondo_id","subj":"T108","obj":"http://purl.obolibrary.org/obo/MONDO_0100096"},{"id":"A109","pred":"mondo_id","subj":"T109","obj":"http://purl.obolibrary.org/obo/MONDO_0100096"},{"id":"A110","pred":"mondo_id","subj":"T110","obj":"http://purl.obolibrary.org/obo/MONDO_0007399"},{"id":"A111","pred":"mondo_id","subj":"T110","obj":"http://purl.obolibrary.org/obo/MONDO_0017361"},{"id":"A112","pred":"mondo_id","subj":"T112","obj":"http://purl.obolibrary.org/obo/MONDO_0006502"},{"id":"A113","pred":"mondo_id","subj":"T113","obj":"http://purl.obolibrary.org/obo/MONDO_0009971"},{"id":"A114","pred":"mondo_id","subj":"T114","obj":"http://purl.obolibrary.org/obo/MONDO_0006502"},{"id":"A115","pred":"mondo_id","subj":"T115","obj":"http://purl.obolibrary.org/obo/MONDO_0043726"},{"id":"A116","pred":"mondo_id","subj":"T116","obj":"http://purl.obolibrary.org/obo/MONDO_0005550"},{"id":"A117","pred":"mondo_id","subj":"T117","obj":"http://purl.obolibrary.org/obo/MONDO_0100096"},{"id":"A118","pred":"mondo_id","subj":"T118","obj":"http://purl.obolibrary.org/obo/MONDO_0005091"},{"id":"A119","pred":"mondo_id","subj":"T119","obj":"http://purl.obolibrary.org/obo/MONDO_0100096"},{"id":"A120","pred":"mondo_id","subj":"T120","obj":"http://purl.obolibrary.org/obo/MONDO_0005091"},{"id":"A121","pred":"mondo_id","subj":"T121","obj":"http://purl.obolibrary.org/obo/MONDO_0021166"},{"id":"A122","pred":"mondo_id","subj":"T122","obj":"http://purl.obolibrary.org/obo/MONDO_0100096"},{"id":"A123","pred":"mondo_id","subj":"T123","obj":"http://purl.obolibrary.org/obo/MONDO_0005070"},{"id":"A124","pred":"mondo_id","subj":"T124","obj":"http://purl.obolibrary.org/obo/MONDO_0005113"},{"id":"A125","pred":"mondo_id","subj":"T125","obj":"http://purl.obolibrary.org/obo/MONDO_0005113"},{"id":"A126","pred":"mondo_id","subj":"T126","obj":"http://purl.obolibrary.org/obo/MONDO_0007399"},{"id":"A127","pred":"mondo_id","subj":"T126","obj":"http://purl.obolibrary.org/obo/MONDO_0017361"},{"id":"A128","pred":"mondo_id","subj":"T128","obj":"http://purl.obolibrary.org/obo/MONDO_0005091"},{"id":"A129","pred":"mondo_id","subj":"T129","obj":"http://purl.obolibrary.org/obo/MONDO_0005091"},{"id":"A130","pred":"mondo_id","subj":"T130","obj":"http://purl.obolibrary.org/obo/MONDO_0006598"},{"id":"A131","pred":"mondo_id","subj":"T131","obj":"http://purl.obolibrary.org/obo/MONDO_0004609"},{"id":"A132","pred":"mondo_id","subj":"T132","obj":"http://purl.obolibrary.org/obo/MONDO_0002363"},{"id":"A133","pred":"mondo_id","subj":"T133","obj":"http://purl.obolibrary.org/obo/MONDO_0021094"},{"id":"A134","pred":"mondo_id","subj":"T134","obj":"http://purl.obolibrary.org/obo/MONDO_0005502"},{"id":"A135","pred":"mondo_id","subj":"T135","obj":"http://purl.obolibrary.org/obo/MONDO_0025028"},{"id":"A136","pred":"mondo_id","subj":"T136","obj":"http://purl.obolibrary.org/obo/MONDO_0004842"},{"id":"A137","pred":"mondo_id","subj":"T137","obj":"http://purl.obolibrary.org/obo/MONDO_0005812"},{"id":"A138","pred":"mondo_id","subj":"T138","obj":"http://purl.obolibrary.org/obo/MONDO_0004992"},{"id":"A139","pred":"mondo_id","subj":"T139","obj":"http://purl.obolibrary.org/obo/MONDO_0005091"},{"id":"A140","pred":"mondo_id","subj":"T140","obj":"http://purl.obolibrary.org/obo/MONDO_0005550"},{"id":"A141","pred":"mondo_id","subj":"T141","obj":"http://purl.obolibrary.org/obo/MONDO_0004842"},{"id":"A142","pred":"mondo_id","subj":"T142","obj":"http://purl.obolibrary.org/obo/MONDO_0005812"},{"id":"A143","pred":"mondo_id","subj":"T143","obj":"http://purl.obolibrary.org/obo/MONDO_0005550"},{"id":"A144","pred":"mondo_id","subj":"T144","obj":"http://purl.obolibrary.org/obo/MONDO_0005091"},{"id":"A145","pred":"mondo_id","subj":"T145","obj":"http://purl.obolibrary.org/obo/MONDO_0100096"},{"id":"A146","pred":"mondo_id","subj":"T146","obj":"http://purl.obolibrary.org/obo/MONDO_0100096"},{"id":"A147","pred":"mondo_id","subj":"T147","obj":"http://purl.obolibrary.org/obo/MONDO_0005070"},{"id":"A148","pred":"mondo_id","subj":"T148","obj":"http://purl.obolibrary.org/obo/MONDO_0005070"},{"id":"A149","pred":"mondo_id","subj":"T149","obj":"http://purl.obolibrary.org/obo/MONDO_0005091"}],"text":"Nanotechnology Tools to Inactivate SARS-CoV-2 in Patients\nThe main target of SARS-CoV-2 is the respiratory tract (upper airways, lung),36 although other organs might also be infected (e.g., gut, kidney) and vasculature21 also appears to be a prime target. The expression of ACE2 probably determines uptake by different tissues.37\nIn addition to discussing immune-based approaches, because the lung is the most critically affected organ, we will center our discussion on the various options to inactivate the virus in the deep lung and to target the essential host cells for drug delivery. The virus reaches the alveoli and enters alveolar epithelial type II cells (AECII), due to the relatively high abundance of ACE2 and a permissive cellular milieu. These cells serve as a reservoir of the virus, which finally spreads throughout the lung, leading to the lung function impairment seen in severe cases. Airborne nanomaterials are optimally suited to penetrate into the deep lung due to the physicochemical properties of such aerosols, existing on the same size scale particles that penetrate most readily to the deep airways. Hence, nanomedicine is already actively pursuing ideas to deliver drugs, therapeutic proteins, and mRNAs by exploiting nanodevices for pulmonary delivery.38,39\nMoreover, the rapid emergence of SARS-CoV-2 has exposed one of the main weaknesses in the current medical landscape: the lack of broad-spectrum antiviral drugs. At present, there are only a handful of approved antivirals, and they are mostly virus-specific. Hence, when a new virus emerges, little can be done pharmacologically to slow down its spread. Some research efforts have been focused on the development of broad-spectrum drugs, which could potentially offer some efficacy against future emerging viruses (and maybe SARS-CoV-2). The various approaches developed over the years are mainly based on the creation of entry inhibitors.40,41 A highly conserved part in viruses is the attachment ligand (VAL). In most known respiratory viruses,42 the VAL targets either heparan sulfate proteoglycans (HSPG)43 or sialic acids (SA).44 Both HSPG and SA mimics have shown in vitro ability to bind to viruses, blocking their interaction with cell membranes, and often in a broad-spectrum way.45−47\nIn the context of nanomedicine, many nanomaterials have been developed, ranging from polymers48 to dendrimers,49 oligomers, NPs,50 liposomes,51 and small molecules.52 However, successful clinical translation has been hindered by the fact that, upon dilution, these compounds lose efficacy as the virus-compound complex dissociates leaving viruses free to restart their replication cycle. Recently, it has been shown that this limitation can be overcome by synthesizing NPs that, after binding, are able to inhibit viral infectivity irreversibly by permanently damaging the virion, refueling the hope for a true, broad-spectrum antiviral drug.53 Because the focus is also on the development of a drug specific to SARS-CoV-2, a good entry inhibitor could be based on blocking the S spike protein interaction with the cellular ACE2 receptor.19,21−23 Regardless of the specific approach, it is imperative that novel, effective antivirals be based on compounds that exhibit very low or negligible toxicity profiles, as patients will most likely need to receive those drugs for extended periods of time and will already be weakened. For these reasons, when designing antiviral drugs, clearance mechanisms have to be kept in mind. An example of this process is the recent redesign of broad-spectrum antiviral NPs into equally effective modified cyclodextrins.54 Moreover, nanotechnology may offer nanotheranostic approaches to fill the existing gap between diagnostics and therapy.55−57 The simultaneous management of both diagnostics and therapy for those suffering from COVID-19 or in future pandemics, as for many other diseases, is an additional potential strategy to take into consideration in which nanomaterials have proven to be effective tools. The advantages of the capabilities of nanotechnology and nanomaterials for combined therapeutics and diagnostics has been widely explored in cancer research; however, there have been considerable efforts in the past few years to extend the scope of this approach to other areas including infectious diseases.58\n\nNanomaterial-Based Vaccine Development and Immunomodulation\nFollowing the publication of the genetic sequence of SARS-CoV-2 on January 11, 2020, intense research efforts have been devoted to developing a vaccine against COVID-19. With unprecedented speed, this extraordinary scientific mobilization led the first vaccine candidate to enter the Phase I human clinical trial on March 16, 2020, and other novel candidates are rapidly following.59 Up to May 22, 2020, there are 10 COVID-19 candidate vaccines in clinical evaluations and 114 in preclinical development.60\nConcerning vaccine and immunization research, nanomaterials can assist in multiple ways to boost the upregulation required by the immune system and to direct the immune response specifically against antigens. Immune-targeted nanotherapeutics can be developed through their rational manufacture at the nanoscale level by designing nanomaterials that are able to amplify host’s immune response, for instance as adjuvants in the context of vaccination.\nThe development of a vaccine will rely either on the direct administration of viral antigens (e.g., in the form of recombinant proteins, vectored vaccines, or whole inactivated or attenuated virus) or RNA- or DNA-encoding viral antigens.27 Candidate antigens for immunization are surface proteins such as the immunogenic spike protein (S1), which is already targeted by antibodies of convalescent patients.27 Because the S1 protein is also essential for cellular uptake, many researchers are using this protein as the primary target for a vaccine.\nThere are many issues related to the delivery of a drug, protein, or RNA into the patient as the cargo is often degraded, not bioavailable, or is swiftly cleared. Nanotechnology provides multiple solutions to these challenges though, as nanocarriers can overcome some of these limitations.\nBiocompatible polymeric-, lipid-based, or inorganic NPs can be tuned with respect to their physicochemical properties to encapsulate cargo proteins with high loading efficiency, improving protein delivery and pharmacokinetics over conventional approaches.38 Intranasal delivery of polymer-encapsulated antigen triggers a strong immune response, and the success of vaccination depends on the appropriate type of polymer in combination with the antigen.61,62 Similarly, researchers have developed lipid and lipid-based NPs as delivery platforms for mRNAs or siRNAs to enable the synthesis of key viral proteins for vaccination or to inactivate critical viral target genes, respectively.39 The field of therapeutic mRNAs to support vaccination has gained momentum, as well,63 at least in part due to nanotechnology-enabled strategies for cargo delivery. Moreover, the versatility of nanoplatforms as antigen-presenting systems offers great opportunities. Considering the possibility of random viral mutations that may alter the antigen shape, functionalizing nanomaterials with a wide number of molecules at the same time to target the virus, or motifs that are specific for pathogens, will increase the efficiency of vaccines and their ability to prevent viral infection.\nSeveral companies are working on mRNA vaccines encoding SARS-CoV-2 proteins such as the spike protein, encapsulated in nanoliposomes with specific physicochemical properties that are potentially akin to those documented for immunization against certain tumor antigens.64 The design of such nanocarriers, which will need to escape recognition by scavenger cells and to be nontoxic and nonimmunogenic, is a challenge that will require substantial time prior to clinical availability.\nOn March 16, 2020, Moderna, through a partnership with the Vaccine Research Center at the U.S. National Institutes of Health, enrolled the first participants into a Phase I clinical trial testing an mRNA vaccine (mRNA-1273) encapsulated in lipid NPs—a record time of just 63 days following sequence selection (NCT04283461).65 The enrollment of the first cohort of participants (18 to 55-year-old healthy subjects) concluded on April 16, 2020.66 CureVac and BioNTech (in partnership with Pfizer) are currently working on similar vaccines; Pfizer/BioNTech, in particular, have recently started the recruitment in Phase I/II trials (NCT04368728, NCT04380701). A DNA plasmid vaccine by Inovio Pharmaceuticals (INO-4800) has showed promising results in mice and guinea pigs according to a recent article published in Nature Communications(67) and has entered Phase I testing in humans (NCT04336410).\nAnother candidate COVID-19 vaccine for Phase I clinical trial is from the University of Oxford and AstraZeneca (NCT04324606).68 Around 1110 people will take part in the trial, which started recruitment at the end of April 2020. The vaccine is based on a chimpanzee adenovirus vaccine vector (ChAdOx1) and the SARS-CoV-2 spike protein. Chimpanzee adenoviral vectors against different pathogens have been already tested in thousands of subjects with demonstrated safety. To date, ChAdOx1 has been administered to six rhesus macaques exposed to high doses of SARS-CoV-2. The vaccine was unable to prevent infections, although it reduced the severity of the disease: no signs of virus replication were observed in the lungs, with significantly lower levels of respiratory disease and no lung damage compared to control animals, according to a recent article deposited in bioRxiv.69 Another adenoviral vector vaccine developed by CanSino Biological Inc. and Beijing Institute of Biotechnology using a genetically engineered replication-defective adenovirus type 5 vector to express the SARS-CoV-2 spike protein (Ad5-nCoV) is currently being tested in Phase I/II trials (NCT04398147, NCT04341389, NCT0431312).\nAlthough vaccines based on novel DNA and mRNA technologies might be promising, there are no such kinds of vaccines on the market, and it is unknown whether they can be effective in humans. Moderna, for instance, has generated preliminary safety data on different mRNA-based vaccines targeting other respiratory viruses, but their more advanced program (on a cytomegalovirus vaccine) is still in Phase II clinical testing. However, on May 18, Moderna announced that mRNA-1273 elicited antibody titers above the levels observed in convalescent individuals (and therefore considered potentially protective) in all eight initial participants across the 25 and 100 μg dose cohorts of the Phase I trial (NCT04283461).70 The company, after having received a fast-track approval from the FDA, is now moving on Phase II trials.\nConversely, strong evidence exists regarding the efficacy of protein-based vaccines such as recombinant-protein, viral-vector, attenuated, or inactivated vaccines across different infectious diseases, with licensed vaccines already existing for all of these platforms.27 All of the aforementioned approaches are currently being explored in the context of SARS-CoV-2.27 An inactivated vaccine developed by the China National Pharmaceutical Group (Sinopharm), in collaboration with the Wuhan Institute of Biological Products, is currently tested in a Phase I/II trial (ChiCTR2000031809), and a second inactivated vaccine (in collaboration with the Beijing Institute of Biological Products) has been currently approved for clinical testing (ChiCTR2000032459). A third aluminum salt (alum) adjuvanted inactivated vaccine, developed by Beijing-based Sinovac Biotech’s, was able to provide partial or complete protection in rhesus macaques, according to a recent article published in Science,71 and is currently being tested in Phase I/II trials (NCT04383574, NCT04352608).\nLive-attenuated vaccines are intrinsically immunogenic (e.g., due to the presence of viral DNA), but extensive safety tests are required due to the rare possibility of reversion to a pathogenic form able to cause infection. Vaccine candidates based in this application have previously been designed for SARS-CoV with high stability.72,73 Recombinant-protein vaccines and inactivated vaccines are safer but might require adjuvants to increase their immunogenicity. In the context of SARS-CoV-2, adjuvants are important for two reasons. First, adjuvants might increase the efficacy of the vaccine, especially in subjects with impaired immunological function, such as the elderly, or in subjects with comorbidities resulting in immune dysfunctions; in these patient cohorts, SARS-CoV-2 has a high lethality rate. Second, adjuvants can reduce the amount of vaccine protein(s) required per dose, which could facilitate scaling-up vaccine production in a reduced time frame.\nBeyond alum, which is in fact a nanoscale material74 that was developed in the 1920s for the tetanus and diphtheria toxoids,75 approval for a new adjuvant did not occur until 1997, with the introduction of the oil-in-water emulsion of squalene oil and polysorbate 80 and sorbitan trioleate surfactants (MF59) in the seasonal influenza vaccine for the elderly.76 The use of MF59 was further expanded to pandemic and avian influenza vaccines.77 Other adjuvants in licensed vaccines have been approved since 2000, as well: (a) AS03 (used for pandemic and avian influenza vaccines), similar to MF59, but including α-tocopherol as an additional immune stimulant; (b) AF 03 (used for pandemic influenza vaccines), an alternative squalene emulsion containing polyoxyethylene, cetostearyl ether, mannitol, and orbitan oleate;78 (c) AS 01 (used for herpes zoster vaccine), a liposome-based vaccine adjuvant system containing two immunostimulants, 3-O-desacyl-4′-monophosphoryl lipid A (MPL, a Toll-like receptor 4 agonist) and saponin QS-21, which activates the ACT-NLRP3 inflammasome pathway;79 and (d) AS04 (used for hepatitis B and human papilloma virus vaccines), which is a combination of MPL and aluminum hydroxide.76,80\nIn this context, the concept of “nanoimmunity by design” relies on the rational design of distinct physicochemical properties and specific functionalization of nanomaterials intended for fine-tuning their potential effects on the immune system.81 Nanomaterials have emerged as promising tools for immune modulation, either stimulating or suppressing the immune response. In fighting SARS-CoV-2, these properties may find applications for both prevention and therapy and in the context of vaccine development.\nMounting evidence indicates that nanomaterials such as graphene,82 nanodiamonds,83 carbon nanotubes,84 and polystyrene particles85 bear an intrinsic capacity to activate the immune system, depending on their functionalization.86 For instance, graphene oxide functionalized with amino groups (GO-NH2) induces activation of STAT1/IRF1 interferon signaling in monocytes and T cells, resulting in the production of T cell chemoattractants, and macrophage 1 (M1) 1/T-helper 1 (Th1) polarization of the immune response, with negligible toxicity.82 Remarkably, the ability of licensed adjuvants such as AS01 and AS03 to enhance adaptive immunity has been linked to their capacity to boost STAT1/IRF1 interferon signaling.87 In addition, recent SARS-CoV and MERS-CoV studies suggest that the development of a Th1-type response is central for controlling infection, which also may be true for SARS-CoV-2.88\nSeveral groups and consortia have been screening and characterizing nanomaterials according to their immunomodulatory properties and absence of cytotoxicity.56,57,82−84,86 The development of an adjuvant for clinical use is a lengthy process that requires extensive Phase III randomized trials in large and diverse cohorts of subjects, and the process generally requires several years.76 Pharmaceutical giants such as GlaxoSmithKline (GSK), which owns the ASs mentioned above and other adjuvant platforms, are engaged in several partnerships to embed their adjuvant systems with SARS-CoV-2-protein-based vaccines. A full-length recombinant SARS-CoV-2 glycoprotein nanoparticle vaccine adjuvanted with the saponin-based Matrix M developed by Novavax is in Phase I clinical testing (NCT04368988).89\nAlthough it is unlikely that novel adjuvants would be used in the context of the current pandemic, the SARS-CoV-2 pandemic offers an opportunity to reflect on the potential of nanotechnology for vaccine adjuvant development. In this context, it is critical to stream coherent pipelines covering in vitro and in vivo experiments specifically to select candidate materials that might be tested for clinical implementation as vaccine adjuvants.90\nIn this view, the status of nanomaterial-based vaccine adjuvants has been reviewed.91,92 In particular, immunomodulatory effects induced on the innate immune signaling have been demonstrated.91 For example, nanomaterials such as GO can elicit an inflammasome sensor (NLRP3)-dependent expression of IL-1β in macrophages.93 Notably, alum, the most commonly used adjuvant in human vaccines, induces the release of this cytokine in macrophages through the same NRLP-induced mechanism. These results suggest that nanomaterials such as GO and alum may be useful for medical applications.94\nConsidering that new vaccine development typically requires years to be approved and applied to the general population, there is also an urgent need to study how to improve treatment approaches. According to the first Phase III clinical studies, the antiviral drug Remdesivir, which was recently approved by the FDA for COVID-19 treatment in the United States, seems to be a promising treatment for adults diagnosed with COVID-19. Nano-based strategies have already been applied to enhance the effectiveness of Remdesivir in the context of other emerging viral infections (Nipah virus),95 suggesting the suitability of nanotechnology to assist with similar strategies for the treatment of COVID-19 as well as other possible pandemics in the future.96\n\nCan Nanotechnology Help to Control the Cytokine Storm?\nOne of the main features of COVID-19 is the triggering of a cytokine storm in the body, also known as cytokine release syndrome (CRS), which results from an excessive immune response and leads to the severe deterioration of patient health.25,97,98 This inflammatory storm is one of the major causes of the acute respiratory distress syndrome (ARDS) that is often associated with multiple-organ failure, representing the leading causes of death in critical patients.98 In particular, the role of interleukin (IL)-6 has been highlighted in patients requiring assisted ventilation. Ongoing clinical trials are testing drugs that block the receptor of IL-6 (Tocilizumab, an anti-IL-6 receptor antibody, and Sarilumab) or IL-6 itself (Siltuximab).\nWhereas a well-regulated cytokine response that is rapidly triggered by the host’s innate immunity can serve to prevent and to counteract an infection, an excessive, unbalanced, prolonged immune response can seriously harm the body. Therefore, therapeutic strategies aimed at effectively suppressing the cytokine storm are under investigation. Nanomaterials have been exploited to adjust the immune response to an optimized level, and such proprieties might be explored to inhibit cytokine releases.99 Nanosystems can enhance the specificity/efficiency of immunosuppressant delivery to target immune cells, with consequent reductions in drug dose, drug distribution to nontarget tissues and organs, and possible side effects. In addition, specific nanotools can be designed to evade the immune system and to enhance the solubility of poorly soluble immunosuppressant agents; the potential of finely tuning their surface charge opens possibilities for encapsulation strategies and offers accommodation for a high drug load. All of these mechanisms may also occur simultaneously, enhancing the activity of immunosuppressive agents.\nConcerning the role of macrophages in COVID-19, the presence of ACE2-expressing CD68+CD169+ macrophages containing SARS-CoV-2 nucleoprotein antigen and showing an increased release of IL-6 was observed in infected spleen and lymph nodes.100 Notably, immunohistochemical and immunofluorescence analyses of lymph nodes and spleen tissue from autopsy samples of patients who died from COVID-19 revealed lymphocytic apoptosis. The tissues infected by SARS-CoV-2 also showed an upregulated expression of Fas, suggesting a role for CD169+ macrophages in viral spreading, aberrant inflammation, and activation-induced lymphocyte apoptosis. Moreover, histological examinations of biopsy samples of patients who died from COVID-19 revealed an increased alveolar exudate due to the extended neutrophil and monocyte infiltrate in lung capillaries with fibrin deposition, probably leading to difficulties in gas exchange. Through nanomedicine, we envision therapeutic approaches aimed at targeting specific immune subpopulations to avoid these complications, and different nanomaterials have already been explored for their specific impact on different immune cell subpopulations.82,83,86 Octadecylamine-functionalized and dexamethasone-adsorbed nanodiamond promotes anti-inflammatory and proregenerative behavior in human macrophages in vitro.101 A low dose of this functionalized nanodiamond also reduced macrophage infiltration and expression of proinflammatory mediators iNOS and tumor necrosis factor (TNF)-α in mice. Overall, these results suggest that nanodiamond particles may be useful as an inherently immunomodulatory platform.\nFinally, the indiscriminate systemic suppression of the immune system may increase the susceptibility to sepsis due to underlying secondary bacterial infections that can exacerbate critical conditions in patients, causing death.102 Risk factors for mortality include acquired bacterial infections by opportunistic organisms and primary pathogens. Using nanocarriers to achieve targeted delivery of immunosuppressive drugs, or the specific and controlled inhibition of only a specific immune cell subpopulation, can play a critical role in limiting such complications.\nThe fast and complete removal of pro-inflammatory cytokines from the bloodstream has been shown to increase survival significantly in patients with early stage sepsis. Adsorption of cytokines from serum by extracorporeal perfusion through porous carbons is one of the most promising ways for their selective, quick, and complete removal from blood circulation. Hierarchical carbon materials with tuned porosity have shown effective adsorption of many cytokines, including IL-6 and even the largest ones, such as TNF-α.103 Based on their demonstrated outstanding performance in removing sepsis-associated pro-inflammatory cytokines from blood, mesoporous carbon adsorbents with hierarchical structure, tuned pore size, and surface chemistry, as well as graphene with open and accessible surfaces104 have significant potential to quickly remove inflammatory cytokines associated with CRS and to prevent mortality arising from an uncontrolled inflammatory cascade, thereby providing enough time for the defense mechanisms of the human body to fight the virus.\n\nPhotodynamic Inactivation of SARS-CoV-2\nIn addition to drug- and vaccine-based antiviral strategies, photodynamic therapy (PDT) stands as a unique approach to inactivate SARS-CoV-2. Using a light-based method, PDT attacks target cells via the excitation of photosensitive agents, called photosensitizers (PSs), with radiation characterized by a wavelength corresponding to its absorption spectrum to generate reactive oxygen species (ROS) in the presence of oxygen, which ultimately results in cell death. Photodynamic therapy is primarily used for the clinical treatment of various oncological disorders.105 It was not until the 1970s that PDT was first used clinically against viruses,106 exploiting ROS production to damage virus proteins, nucleic acids, and—if present—lipids.107 Even though there are research efforts for PDT-based virotherapies against different viruses, including herpes simplex virus, human papilloma virus, and human immunodeficiency virus,106−109 clinical use of PDT is limited due to hydrophobicity of PSs, poor target specificity, and limited tissue penetration ability.\nMost PSs are hydrophobic and aggregate in aqueous solutions, affecting their photochemical and photobiological properties.106,110 For this reason, Lim et al. have proposed a promising approach for photodynamic inactivation of viruses with NPs, developing sodium yttrium fluoride (NaYF4) upconversion NPs (UCNs) with zinc phthalocyanine PSs grafted onto their surfaces. Unlike most PSs, these UCNs are coated with polyethylenimine (PEI), which render them hydrophilic and easier to manipulate. These UCNs showed antiviral activity against Dengue virus serotype 2 and adenovirus type 5, which were used as models of enveloped and non-enveloped viruses, respectively.110 MXenes111,112 are a large family of 2D transition metal carbides, nitrides,113 and carbonitrides114 that exhibit unique electronic, optical, and catalytic properties. They have the general formula Mn+1XnTx, where M is an early transition metal (Ti, Zr, V, Mo, etc.), X is C and/or N, Tx represents the surface functional groups (=O, −OH, −F, −Cl), and n = 1–4.115,116 Some examples include Ti3C2Tx, V2CTx, and Nb2CTx, with over 30 stoichiometric compositions already synthesized with more than 100 predicted. Biocompatible MXenes, such as Ti3C2Tx, are hydrophilic and are among the most efficient light-to-heat transforming materials.117 The plasmon resonance extinction maxima of Ti3C2Tx is at 780 nm, enabling the use of near-infrared (IR) light for PDT. Several other MXenes have absorption maxima in the IR range and have shown outstanding performance in PDT and theranostic applications.118\nFullerene and graphene are also good candidates for virus inactivation by PDT and have proved to be effective against Semliki Forest virus (SFV), vesicular stomatitis virus (VSV), HSV-1, HIV-1, mosquito iridovirus (MIV), and influenza A virus (IAV), as well as the phage MS2.107 In addition, several 2D nanomaterials, including graphene-based materials, MXenes, black phosphorus, graphitic carbon nitride, tungsten disulfide, and molybdenum disulfide, have been reported to improve the efficacy of PDT considerably for cancer treatment.56,57 Therefore, determining if such nanomaterial-based PDT protocols could be exploited to inactivate SARS-CoV-2 is of great interest.\n\nBiomimetic Engineering of Nanodelivery Systems: Artificial Viruses in the Making\nIn an effort to engineer the next generation of nanoscale vectors, scientists have moved from using inorganic components aimed at obtaining inert structures to utilizing biological building blocks that are able to convey additional functionalities to the resulting construct. To cope with the complexity of the body and to evade the multiple layers of defense that tissues and organs have, it is critical to rely on the ability of certain materials to interact with, rather than to eschew, the biology of our body. Every NP system conceived to date faces one common fate: whether injected, inhaled, ingested, or absorbed through our epithelia, all will at some point come into contact with the mixture of fluids and organic compounds that comprise the body. Under such conditions, every material reacts in a unique way according to the conditions they individually face (i.e., the tissue or body region they are in), their composition (i.e., organic or inorganic), and their physical properties (i.e., size, shape, surface charge).119 Inorganic NPs can function as globular protein mimics because of their similar size, charge, shape, and surface features that can be chemically functionalized to resemble proteins. These similarities can be used in biotechnology to control virus pathways or cell receptor interactions with NPs.120\nAmong the many attempts, the legendary accomplishments, and the epic failures, we could list thousands of different NPs, differing from one another thanks to the creative endeavors of their designers. Despite their remarkable differences and researchers’ endeavors to make them one of a kind, they all aim to achieve one goal: to deliver in a specific way one particular form of payload, while remaining as unnoticed as possible by the body’s defense mechanisms. For everyone who has made an effort to create their own version of such a silver bullet, it has come to mind that nature in its incredible variety had already invented a few ingenious solutions to this problem. In the world of nano-based drug delivery, one entity dominates as the quintessential example of precision, efficiency, and stealth: the virus. Not by chance, these two worlds share many features that span from the physical laws that govern their assembly and stability to the chemical similarities in their overall composition.121\nHistorically, the field of nanomedicine and the global endeavor to generate NPs for drug delivery arose from one of the greatest struggles in medicine: gene therapy. At the core of that approach was the idea that viruses could be used as Trojan horses to deliver the correct sequence of the gene into the cells that harbored the mutated copy. In order to identify the virus that offered the best delivery service, a cadre of viral strains and species were tested, adapted, and engineered to fit the aims and hit the targets. It took decades of trial and error, of progressive adjustments, and a few tragic mistakes to identify the viruses that could provide the ideal backbone to develop viral vectors able to ferry genetic cargo into the target cell.122 In the midst of that global challenge, nanotechnology offered a safer and more controllable alternative: to generate bespoke structures that could replace viral vectors and do the same job, delivering a payload from the point of injection to the site of action.\nFast forward a few decades, and the promises made by both worlds seem to have finally become reality, with a number of active clinical trials, some clinical success stories, and a few commercial products in the market space. Interestingly, the two worlds seem to have maintained a safe distance so as to avoid any collision or dangerous proximity. Exchange of ideas between the fields of gene therapy and nanomedicine is limited, and the potential for disciplinary growth through knowledge exchange has remained elusive to scientists in both fields.5 Many scientists have suggested that nanotechnologists could find inspiration in the mechanisms devised by viruses to elude immune surveillance, to overcome biological barriers, and to deliver their genetic payload with high specificity. Similarly, gene therapists would find high-tech solutions to their scalability and safety issues in looking at the new generations of biomimetic particles being generated.\nThe principles of biomimicry and bioinspiration have been used to design and to engineer drug-delivery technologies that reproduce or recapitulate biological materials, for what pertains to not only their structure and chemistry but also, more importantly, their functions. In drug delivery, surface recognition and nanoscale interactions between materials and biological entities are key to the success of the delivery strategy, and the use of biological building blocks such as membrane proteins has been proposed as a way to convey targeting and shielding moieties simultaneously.123 One can only hope that the recent events and global attention that viruses are capturing in the scientific world will spur a renewed interest in finding ways to adapt viral features and mechanisms of action to the world of NPs. Increased focus in this field would be useful to create virus-like NPs able to circulate in the blood system, overcoming the endothelial barrier, and to deliver their therapeutic payload with high efficiency.124\n\nInterference with Cellular Uptake, Immobilization, and Inactivation of the Virus Outside of the Host Cell\nNanomaterials can be synthesized with a high specific surface area of a few hundred square meters per gram. Therefore, dependent on the surface properties, nanomaterials efficiently adsorb biomolecules and form a so-called biomolecular corona. This passive, nontargeted adsorption might be utilized to bind viruses, provided that the selected nanomaterial is relatively biocompatible. Viral surface proteins are often modified by sugar moieties or encompass positively charged amino acid patches that bind to lectins or glycosaminoglycans (GAGs) of heparan sulfate (HS), respectively.32 Robust interactions of virus particles with these host receptors is ensured by multivalent binding, which is likely why single-molecule inhibitors often are not capable of efficiently perturbing this key event but multivalent NPs are superior to block binding of different viruses to the host cell.125\nGold NPs capped with mercaptoethanesulfonate are effective inhibitors of HSV type 1 infection as they mimic cell-surface-receptor heparan sulfate and, therefore, competitively bind to the virus. Interestingly, polyvalent sulfated Au NPs inhibit virus binding to the host cell dependent on their size. Nanoparticles of diameters equal to and larger than the virus diameter (in this case, the stomatitis virus) more efficiently inhibit the binding to cells than smaller particles. Most likely, larger NPs efficiently cross-link virions, whereas smaller NPs simply decorate the viral surface.126 Papp et al. found that gold NPs decorated with SA effectively inhibited the binding of influenza virus to the target cells. In this case, viral recognition via its surface protein hemagglutinin of SA on the host cell membrane was a prerequisite for cellular entry.127 More recently, Cagno et al. reported antiviral NPs (Au and iron oxide core) with long and flexible linkers mimicking HS that strongly bind and inactivate viruses such as respiratory syncytial virus in vitro and in vivo in a lung infection model and even led to irreversible viral deformation.53 Hence, there is ample evidence that biocompatible, functionalized NPs can act as broad-spectrum antivirals. Notably, the receptor-binding domain of the spike S1 protein of SARS-CoV-2 binds not only to ACE2 but also to heparin128 and, thus, might be targeted by similar approaches as outlined above.\nOne of the most recent strategies to inhibit viral uptake is based on administration of recombinant ACE2 to inhibit binding competitively via the spike S1 protein.21 Knowing that multivalency is key to block virus–host interactions reliably, researchers have speculated that a nanostructured carrier could not only improve delivery and cargo stability but also might dramatically enhance binding strength.129\n\nSpeeding up the Nanomedicine-Based Approaches for COVID-19 by In Silico Analysis\nCurrently, repurposing drug molecules is a key strategy for identifying approved or investigational drugs outside the scope of the original medical indication that can be used to fight COVID-19.130,131 There are various advantages to this strategy, including already-established safety profiles, fast transition to clinical studies, and less investment needed, compared to the process of developing an entirely new drug.132,133 Therefore, these advantages have the potential to result in less risky and more rapid returns on investment in the development of repurposed drugs, benefits that are particularly important during pandemics. In addition to rapid diagnosis, making recommendations to physicians about rapid treatment methods can save the lives of many people. Combining in silico tools such as molecular docking, molecular dynamics, and computational chemistry with large drug databases provides a great advantage in selecting “possible candidates” from among thousands of pharmaceutically active substances (Figure 3). In silico analyses should be supported by the literature, expert opinions (pharmacologists and clinicians), and, when possible, preclinical and clinical findings. After selecting the best candidates following in silico analyses, detailed in vitro and in vivo experimentation should be undertaken prior to clinical studies. Recently, various drug-repurposing studies have been published to assist in the global COVID-19 pandemic response.134−136 Such studies aim to identify whether already-approved drugs have the capacity to interact with viral proteins or receptors on host cells.\nFigure 3 Schematic of how in silico analysis can be used to select candidate drug molecules for clinical studies. MD, molecular dynamics. In nanomedicine, computational models have recently garnered attention; such work may help to identify how nanomaterials interact with biological systems and to determine how the efficacy of these nanotherapeutics could be improved. Computational models indicate how NPs are taken up by healthy cells or tumor cells, enabling better predictions regarding the pharmacokinetic and pharmacodynamic properties of these materials.134 For example, Lunnoo et al. used a coarse-grained molecular dynamics (MD) simulation to observe the internalization pathways of various Au nanostructures (nanospheres, nanocages, nanorods, nanoplates, and nanohexapods) into an idealized mammalian plasma membrane.137 Other studies have simulated how different NPs can target tumor cells and deliver drugs.138,139 Therefore, in silico approaches that are currently used for drug repurposing, including molecular docking, molecular dynamics, and computational chemistry, constitute valuable tools to aid preclinical and clinical studies of nanomaterials directed for disease treatment. Considering the urgent need for nanomedicine against the current pandemic, in silico analyses may be especially useful in guiding the rational design of new NP formulations required to fight SARS-CoV-2."}
LitCovid-PD-CLO
{"project":"LitCovid-PD-CLO","denotations":[{"id":"T106","span":{"begin":120,"end":127},"obj":"http://purl.obolibrary.org/obo/UBERON_0001005"},{"id":"T107","span":{"begin":129,"end":133},"obj":"http://purl.obolibrary.org/obo/UBERON_0002048"},{"id":"T108","span":{"begin":129,"end":133},"obj":"http://www.ebi.ac.uk/efo/EFO_0000934"},{"id":"T109","span":{"begin":135,"end":137},"obj":"http://purl.obolibrary.org/obo/CLO_0001313"},{"id":"T110","span":{"begin":153,"end":159},"obj":"http://purl.obolibrary.org/obo/UBERON_0003103"},{"id":"T111","span":{"begin":190,"end":193},"obj":"http://purl.obolibrary.org/obo/UBERON_0001007"},{"id":"T112","span":{"begin":190,"end":193},"obj":"http://purl.obolibrary.org/obo/UBERON_0001555"},{"id":"T113","span":{"begin":190,"end":193},"obj":"http://www.ebi.ac.uk/efo/EFO_0000834"},{"id":"T114","span":{"begin":195,"end":201},"obj":"http://purl.obolibrary.org/obo/UBERON_0002113"},{"id":"T115","span":{"begin":195,"end":201},"obj":"http://www.ebi.ac.uk/efo/EFO_0000927"},{"id":"T116","span":{"begin":195,"end":201},"obj":"http://www.ebi.ac.uk/efo/EFO_0000929"},{"id":"T117","span":{"begin":240,"end":241},"obj":"http://purl.obolibrary.org/obo/CLO_0001020"},{"id":"T118","span":{"begin":393,"end":397},"obj":"http://purl.obolibrary.org/obo/UBERON_0002048"},{"id":"T119","span":{"begin":393,"end":397},"obj":"http://www.ebi.ac.uk/efo/EFO_0000934"},{"id":"T120","span":{"begin":430,"end":435},"obj":"http://purl.obolibrary.org/obo/UBERON_0003103"},{"id":"T121","span":{"begin":508,"end":513},"obj":"http://purl.obolibrary.org/obo/NCBITaxon_10239"},{"id":"T122","span":{"begin":526,"end":530},"obj":"http://purl.obolibrary.org/obo/UBERON_0002048"},{"id":"T123","span":{"begin":526,"end":530},"obj":"http://www.ebi.ac.uk/efo/EFO_0000934"},{"id":"T124","span":{"begin":564,"end":569},"obj":"http://purl.obolibrary.org/obo/GO_0005623"},{"id":"T125","span":{"begin":593,"end":598},"obj":"http://purl.obolibrary.org/obo/NCBITaxon_10239"},{"id":"T126","span":{"begin":639,"end":649},"obj":"http://purl.obolibrary.org/obo/CL_0000066"},{"id":"T127","span":{"begin":658,"end":663},"obj":"http://purl.obolibrary.org/obo/GO_0005623"},{"id":"T128","span":{"begin":722,"end":723},"obj":"http://purl.obolibrary.org/obo/CLO_0001020"},{"id":"T129","span":{"begin":758,"end":763},"obj":"http://purl.obolibrary.org/obo/GO_0005623"},{"id":"T130","span":{"begin":773,"end":774},"obj":"http://purl.obolibrary.org/obo/CLO_0001020"},{"id":"T131","span":{"begin":792,"end":797},"obj":"http://purl.obolibrary.org/obo/NCBITaxon_10239"},{"id":"T132","span":{"begin":836,"end":840},"obj":"http://purl.obolibrary.org/obo/UBERON_0002048"},{"id":"T133","span":{"begin":836,"end":840},"obj":"http://www.ebi.ac.uk/efo/EFO_0000934"},{"id":"T134","span":{"begin":857,"end":861},"obj":"http://purl.obolibrary.org/obo/UBERON_0002048"},{"id":"T135","span":{"begin":857,"end":861},"obj":"http://www.ebi.ac.uk/efo/EFO_0000934"},{"id":"T136","span":{"begin":975,"end":979},"obj":"http://purl.obolibrary.org/obo/UBERON_0002048"},{"id":"T137","span":{"begin":975,"end":979},"obj":"http://www.ebi.ac.uk/efo/EFO_0000934"},{"id":"T138","span":{"begin":1118,"end":1125},"obj":"http://purl.obolibrary.org/obo/UBERON_0001005"},{"id":"T139","span":{"begin":1158,"end":1166},"obj":"http://purl.obolibrary.org/obo/CLO_0001658"},{"id":"T140","span":{"begin":1331,"end":1334},"obj":"http://purl.obolibrary.org/obo/CLO_0051582"},{"id":"T141","span":{"begin":1475,"end":1476},"obj":"http://purl.obolibrary.org/obo/CLO_0001020"},{"id":"T142","span":{"begin":1529,"end":1534},"obj":"http://purl.obolibrary.org/obo/NCBITaxon_10239"},{"id":"T143","span":{"begin":1557,"end":1558},"obj":"http://purl.obolibrary.org/obo/CLO_0001020"},{"id":"T144","span":{"begin":1563,"end":1568},"obj":"http://purl.obolibrary.org/obo/NCBITaxon_10239"},{"id":"T145","span":{"begin":1672,"end":1679},"obj":"http://purl.obolibrary.org/obo/CLO_0009985"},{"id":"T146","span":{"begin":1792,"end":1799},"obj":"http://purl.obolibrary.org/obo/NCBITaxon_10239"},{"id":"T147","span":{"begin":1931,"end":1932},"obj":"http://purl.obolibrary.org/obo/CLO_0001020"},{"id":"T148","span":{"begin":1958,"end":1965},"obj":"http://purl.obolibrary.org/obo/NCBITaxon_10239"},{"id":"T149","span":{"begin":1992,"end":1995},"obj":"http://purl.obolibrary.org/obo/CLO_0037067"},{"id":"T150","span":{"begin":2024,"end":2031},"obj":"http://purl.obolibrary.org/obo/NCBITaxon_10239"},{"id":"T151","span":{"begin":2039,"end":2042},"obj":"http://purl.obolibrary.org/obo/CLO_0037067"},{"id":"T152","span":{"begin":2058,"end":2087},"obj":"http://purl.obolibrary.org/obo/PR_000001307"},{"id":"T153","span":{"begin":2089,"end":2093},"obj":"http://purl.obolibrary.org/obo/PR_000001307"},{"id":"T154","span":{"begin":2126,"end":2130},"obj":"http://purl.obolibrary.org/obo/PR_000001307"},{"id":"T155","span":{"begin":2184,"end":2191},"obj":"http://purl.obolibrary.org/obo/NCBITaxon_10239"},{"id":"T156","span":{"begin":2225,"end":2229},"obj":"http://purl.obolibrary.org/obo/GO_0005623"},{"id":"T157","span":{"begin":2230,"end":2239},"obj":"http://purl.obolibrary.org/obo/UBERON_0000158"},{"id":"T158","span":{"begin":2254,"end":2255},"obj":"http://purl.obolibrary.org/obo/CLO_0001020"},{"id":"T159","span":{"begin":2275,"end":2277},"obj":"http://purl.obolibrary.org/obo/CLO_0053799"},{"id":"T160","span":{"begin":2445,"end":2447},"obj":"http://purl.obolibrary.org/obo/CLO_0001407"},{"id":"T161","span":{"begin":2489,"end":2492},"obj":"http://purl.obolibrary.org/obo/CLO_0051582"},{"id":"T162","span":{"begin":2577,"end":2582},"obj":"http://purl.obolibrary.org/obo/NCBITaxon_10239"},{"id":"T163","span":{"begin":2620,"end":2627},"obj":"http://purl.obolibrary.org/obo/NCBITaxon_10239"},{"id":"T164","span":{"begin":2682,"end":2685},"obj":"http://purl.obolibrary.org/obo/CLO_0051582"},{"id":"T165","span":{"begin":2885,"end":2886},"obj":"http://purl.obolibrary.org/obo/CLO_0001020"},{"id":"T166","span":{"begin":2938,"end":2943},"obj":"http://purl.obolibrary.org/obo/CLO_0009985"},{"id":"T167","span":{"begin":2974,"end":2975},"obj":"http://purl.obolibrary.org/obo/CLO_0001020"},{"id":"T168","span":{"begin":3002,"end":3006},"obj":"http://purl.obolibrary.org/obo/CLO_0001236"},{"id":"T169","span":{"begin":4141,"end":4144},"obj":"http://purl.obolibrary.org/obo/CLO_0051582"},{"id":"T170","span":{"begin":4475,"end":4477},"obj":"http://purl.obolibrary.org/obo/CLO_0053733"},{"id":"T171","span":{"begin":4542,"end":4543},"obj":"http://purl.obolibrary.org/obo/CLO_0001020"},{"id":"T172","span":{"begin":4692,"end":4697},"obj":"http://purl.obolibrary.org/obo/NCBITaxon_9606"},{"id":"T173","span":{"begin":4794,"end":4796},"obj":"http://purl.obolibrary.org/obo/CLO_0050507"},{"id":"T174","span":{"begin":4873,"end":4876},"obj":"http://purl.obolibrary.org/obo/CLO_0053001"},{"id":"T175","span":{"begin":5037,"end":5050},"obj":"http://purl.obolibrary.org/obo/UBERON_0002405"},{"id":"T176","span":{"begin":5376,"end":5377},"obj":"http://purl.obolibrary.org/obo/CLO_0001020"},{"id":"T177","span":{"begin":5548,"end":5553},"obj":"http://purl.obolibrary.org/obo/NCBITaxon_10239"},{"id":"T178","span":{"begin":5594,"end":5596},"obj":"http://purl.obolibrary.org/obo/CLO_0050509"},{"id":"T179","span":{"begin":5693,"end":5695},"obj":"http://purl.obolibrary.org/obo/CLO_0050050"},{"id":"T180","span":{"begin":5763,"end":5765},"obj":"http://purl.obolibrary.org/obo/CLO_0050509"},{"id":"T181","span":{"begin":5778,"end":5780},"obj":"http://purl.obolibrary.org/obo/CLO_0050050"},{"id":"T182","span":{"begin":5894,"end":5895},"obj":"http://purl.obolibrary.org/obo/CLO_0001020"},{"id":"T183","span":{"begin":5954,"end":5955},"obj":"http://purl.obolibrary.org/obo/CLO_0001020"},{"id":"T184","span":{"begin":6514,"end":6515},"obj":"http://purl.obolibrary.org/obo/CLO_0001020"},{"id":"T185","span":{"begin":6859,"end":6864},"obj":"http://purl.obolibrary.org/obo/OGG_0000000002"},{"id":"T186","span":{"begin":6886,"end":6891},"obj":"http://purl.obolibrary.org/obo/UBERON_0007688"},{"id":"T187","span":{"begin":6936,"end":6939},"obj":"http://purl.obolibrary.org/obo/CLO_0051582"},{"id":"T188","span":{"begin":7270,"end":7271},"obj":"http://purl.obolibrary.org/obo/CLO_0001020"},{"id":"T189","span":{"begin":7328,"end":7333},"obj":"http://purl.obolibrary.org/obo/NCBITaxon_10239"},{"id":"T190","span":{"begin":7820,"end":7825},"obj":"http://purl.obolibrary.org/obo/GO_0005623"},{"id":"T191","span":{"begin":7868,"end":7869},"obj":"http://purl.obolibrary.org/obo/CLO_0001020"},{"id":"T192","span":{"begin":7983,"end":7984},"obj":"http://purl.obolibrary.org/obo/CLO_0001020"},{"id":"T193","span":{"begin":8110,"end":8111},"obj":"http://purl.obolibrary.org/obo/CLO_0001020"},{"id":"T194","span":{"begin":8135,"end":8142},"obj":"http://purl.obolibrary.org/obo/UBERON_0000473"},{"id":"T195","span":{"begin":8197,"end":8198},"obj":"http://purl.obolibrary.org/obo/CLO_0001020"},{"id":"T196","span":{"begin":8325,"end":8327},"obj":"http://purl.obolibrary.org/obo/CLO_0050510"},{"id":"T197","span":{"begin":8604,"end":8605},"obj":"http://purl.obolibrary.org/obo/CLO_0001020"},{"id":"T198","span":{"begin":8663,"end":8666},"obj":"http://purl.obolibrary.org/obo/CLO_0051582"},{"id":"T199","span":{"begin":8704,"end":8715},"obj":"http://purl.obolibrary.org/obo/NCBITaxon_10141"},{"id":"T200","span":{"begin":8729,"end":8730},"obj":"http://purl.obolibrary.org/obo/CLO_0001020"},{"id":"T201","span":{"begin":8789,"end":8792},"obj":"http://purl.obolibrary.org/obo/CLO_0051582"},{"id":"T202","span":{"begin":8809,"end":8816},"obj":"http://purl.obolibrary.org/obo/UBERON_0000473"},{"id":"T203","span":{"begin":8820,"end":8826},"obj":"http://purl.obolibrary.org/obo/NCBITaxon_9606"},{"id":"T204","span":{"begin":9094,"end":9095},"obj":"http://purl.obolibrary.org/obo/CLO_0001020"},{"id":"T205","span":{"begin":9096,"end":9106},"obj":"http://purl.obolibrary.org/obo/NCBITaxon_9598"},{"id":"T206","span":{"begin":9177,"end":9187},"obj":"http://purl.obolibrary.org/obo/NCBITaxon_9598"},{"id":"T207","span":{"begin":9253,"end":9259},"obj":"http://purl.obolibrary.org/obo/UBERON_0000473"},{"id":"T208","span":{"begin":9328,"end":9331},"obj":"http://purl.obolibrary.org/obo/CLO_0051582"},{"id":"T209","span":{"begin":9357,"end":9372},"obj":"http://purl.obolibrary.org/obo/NCBITaxon_9544"},{"id":"T210","span":{"begin":9517,"end":9522},"obj":"http://purl.obolibrary.org/obo/NCBITaxon_10239"},{"id":"T211","span":{"begin":9556,"end":9561},"obj":"http://www.ebi.ac.uk/efo/EFO_0000934"},{"id":"T212","span":{"begin":9625,"end":9629},"obj":"http://purl.obolibrary.org/obo/UBERON_0002048"},{"id":"T213","span":{"begin":9625,"end":9629},"obj":"http://www.ebi.ac.uk/efo/EFO_0000934"},{"id":"T214","span":{"begin":9657,"end":9664},"obj":"http://purl.obolibrary.org/obo/NCBITaxon_33208"},{"id":"T215","span":{"begin":9679,"end":9680},"obj":"http://purl.obolibrary.org/obo/CLO_0001020"},{"id":"T216","span":{"begin":9836,"end":9837},"obj":"http://purl.obolibrary.org/obo/CLO_0001020"},{"id":"T217","span":{"begin":9978,"end":9984},"obj":"http://purl.obolibrary.org/obo/UBERON_0000473"},{"id":"T218","span":{"begin":10227,"end":10233},"obj":"http://purl.obolibrary.org/obo/NCBITaxon_9606"},{"id":"T219","span":{"begin":10258,"end":10261},"obj":"http://purl.obolibrary.org/obo/CLO_0051582"},{"id":"T220","span":{"begin":10357,"end":10364},"obj":"http://purl.obolibrary.org/obo/NCBITaxon_10239"},{"id":"T221","span":{"begin":10402,"end":10403},"obj":"http://purl.obolibrary.org/obo/CLO_0001020"},{"id":"T222","span":{"begin":10459,"end":10466},"obj":"http://purl.obolibrary.org/obo/UBERON_0000473"},{"id":"T223","span":{"begin":10484,"end":10486},"obj":"http://purl.obolibrary.org/obo/CLO_0050510"},{"id":"T224","span":{"begin":10795,"end":10796},"obj":"http://purl.obolibrary.org/obo/CLO_0001020"},{"id":"T225","span":{"begin":11133,"end":11135},"obj":"http://purl.obolibrary.org/obo/CLO_0050509"},{"id":"T226","span":{"begin":11402,"end":11408},"obj":"http://purl.obolibrary.org/obo/UBERON_0000473"},{"id":"T227","span":{"begin":11412,"end":11413},"obj":"http://purl.obolibrary.org/obo/CLO_0001020"},{"id":"T228","span":{"begin":11455,"end":11456},"obj":"http://purl.obolibrary.org/obo/CLO_0001020"},{"id":"T229","span":{"begin":11553,"end":11556},"obj":"http://purl.obolibrary.org/obo/CLO_0051582"},{"id":"T230","span":{"begin":11594,"end":11601},"obj":"http://purl.obolibrary.org/obo/UBERON_0000473"},{"id":"T231","span":{"begin":11622,"end":11623},"obj":"http://purl.obolibrary.org/obo/CLO_0001020"},{"id":"T232","span":{"begin":11783,"end":11798},"obj":"http://purl.obolibrary.org/obo/NCBITaxon_9544"},{"id":"T233","span":{"begin":11813,"end":11814},"obj":"http://purl.obolibrary.org/obo/CLO_0001020"},{"id":"T234","span":{"begin":11851,"end":11853},"obj":"http://purl.obolibrary.org/obo/CLO_0054055"},{"id":"T235","span":{"begin":11877,"end":11883},"obj":"http://purl.obolibrary.org/obo/UBERON_0000473"},{"id":"T236","span":{"begin":12051,"end":12056},"obj":"http://purl.obolibrary.org/obo/UBERON_0000473"},{"id":"T237","span":{"begin":12114,"end":12115},"obj":"http://purl.obolibrary.org/obo/CLO_0001020"},{"id":"T238","span":{"begin":12716,"end":12719},"obj":"http://purl.obolibrary.org/obo/CLO_0051582"},{"id":"T239","span":{"begin":12720,"end":12721},"obj":"http://purl.obolibrary.org/obo/CLO_0001020"},{"id":"T240","span":{"begin":12880,"end":12881},"obj":"http://purl.obolibrary.org/obo/CLO_0001020"},{"id":"T241","span":{"begin":12932,"end":12933},"obj":"http://purl.obolibrary.org/obo/CLO_0001020"},{"id":"T242","span":{"begin":13042,"end":13043},"obj":"http://purl.obolibrary.org/obo/CLO_0001020"},{"id":"T243","span":{"begin":13423,"end":13424},"obj":"http://purl.obolibrary.org/obo/CLO_0001020"},{"id":"T244","span":{"begin":13561,"end":13562},"obj":"http://purl.obolibrary.org/obo/CLO_0001021"},{"id":"T245","span":{"begin":13766,"end":13767},"obj":"http://purl.obolibrary.org/obo/CLO_0001020"},{"id":"T246","span":{"begin":13876,"end":13877},"obj":"http://purl.obolibrary.org/obo/CLO_0001020"},{"id":"T247","span":{"begin":13884,"end":13885},"obj":"http://purl.obolibrary.org/obo/CLO_0001020"},{"id":"T248","span":{"begin":13941,"end":13950},"obj":"http://purl.obolibrary.org/obo/CLO_0001658"},{"id":"T249","span":{"begin":14022,"end":14023},"obj":"http://purl.obolibrary.org/obo/CLO_0001021"},{"id":"T250","span":{"begin":14028,"end":14033},"obj":"http://purl.obolibrary.org/obo/NCBITaxon_9606"},{"id":"T251","span":{"begin":14044,"end":14049},"obj":"http://purl.obolibrary.org/obo/NCBITaxon_10239"},{"id":"T252","span":{"begin":14070,"end":14071},"obj":"http://purl.obolibrary.org/obo/CLO_0001020"},{"id":"T253","span":{"begin":14350,"end":14363},"obj":"http://purl.obolibrary.org/obo/UBERON_0002405"},{"id":"T254","span":{"begin":14790,"end":14798},"obj":"http://purl.obolibrary.org/obo/CLO_0001658"},{"id":"T255","span":{"begin":14803,"end":14816},"obj":"http://purl.obolibrary.org/obo/UBERON_0002405"},{"id":"T256","span":{"begin":14937,"end":14947},"obj":"http://purl.obolibrary.org/obo/CLO_0001658"},{"id":"T257","span":{"begin":14973,"end":14982},"obj":"http://purl.obolibrary.org/obo/SO_0000418"},{"id":"T258","span":{"begin":14986,"end":14995},"obj":"http://purl.obolibrary.org/obo/CL_0000576"},{"id":"T259","span":{"begin":15000,"end":15007},"obj":"http://purl.obolibrary.org/obo/CL_0000084"},{"id":"T260","span":{"begin":15040,"end":15046},"obj":"http://purl.obolibrary.org/obo/CL_0000084"},{"id":"T261","span":{"begin":15083,"end":15085},"obj":"http://purl.obolibrary.org/obo/CLO_0007448"},{"id":"T262","span":{"begin":15083,"end":15085},"obj":"http://purl.obolibrary.org/obo/CLO_0050175"},{"id":"T263","span":{"begin":15268,"end":15271},"obj":"http://purl.obolibrary.org/obo/CLO_0051582"},{"id":"T264","span":{"begin":15333,"end":15342},"obj":"http://purl.obolibrary.org/obo/SO_0000418"},{"id":"T265","span":{"begin":15428,"end":15429},"obj":"http://purl.obolibrary.org/obo/CLO_0001020"},{"id":"T266","span":{"begin":15750,"end":15751},"obj":"http://purl.obolibrary.org/obo/CLO_0001020"},{"id":"T267","span":{"begin":15982,"end":15985},"obj":"http://purl.obolibrary.org/obo/NCBITaxon_9793"},{"id":"T268","span":{"begin":16140,"end":16141},"obj":"http://purl.obolibrary.org/obo/CLO_0001020"},{"id":"T269","span":{"begin":16298,"end":16305},"obj":"http://purl.obolibrary.org/obo/UBERON_0000473"},{"id":"T270","span":{"begin":16708,"end":16714},"obj":"http://purl.obolibrary.org/obo/UBERON_0000473"},{"id":"T271","span":{"begin":16832,"end":16835},"obj":"http://purl.obolibrary.org/obo/CLO_0051582"},{"id":"T272","span":{"begin":16925,"end":16934},"obj":"http://purl.obolibrary.org/obo/SO_0000418"},{"id":"T273","span":{"begin":17139,"end":17144},"obj":"http://purl.obolibrary.org/obo/NCBITaxon_9606"},{"id":"T274","span":{"begin":17348,"end":17350},"obj":"http://purl.obolibrary.org/obo/CLO_0001527"},{"id":"T275","span":{"begin":17724,"end":17725},"obj":"http://purl.obolibrary.org/obo/CLO_0001020"},{"id":"T276","span":{"begin":17930,"end":17935},"obj":"http://purl.obolibrary.org/obo/NCBITaxon_10239"},{"id":"T277","span":{"begin":18216,"end":18217},"obj":"http://purl.obolibrary.org/obo/CLO_0001020"},{"id":"T278","span":{"begin":18546,"end":18551},"obj":"http://purl.obolibrary.org/obo/UBERON_0003103"},{"id":"T279","span":{"begin":18672,"end":18675},"obj":"http://purl.obolibrary.org/obo/CLO_0051582"},{"id":"T280","span":{"begin":18765,"end":18772},"obj":"http://purl.obolibrary.org/obo/UBERON_0000473"},{"id":"T281","span":{"begin":18909,"end":18910},"obj":"http://purl.obolibrary.org/obo/CLO_0001020"},{"id":"T282","span":{"begin":19501,"end":19506},"obj":"http://purl.obolibrary.org/obo/GO_0005623"},{"id":"T283","span":{"begin":19592,"end":19598},"obj":"http://purl.obolibrary.org/obo/UBERON_0003103"},{"id":"T284","span":{"begin":19688,"end":19701},"obj":"http://purl.obolibrary.org/obo/UBERON_0002405"},{"id":"T285","span":{"begin":19906,"end":19907},"obj":"http://purl.obolibrary.org/obo/CLO_0001020"},{"id":"T286","span":{"begin":19993,"end":20001},"obj":"http://purl.obolibrary.org/obo/CLO_0001658"},{"id":"T287","span":{"begin":20111,"end":20115},"obj":"http://purl.obolibrary.org/obo/PR_000002064"},{"id":"T288","span":{"begin":20245,"end":20251},"obj":"http://purl.obolibrary.org/obo/UBERON_0002106"},{"id":"T289","span":{"begin":20256,"end":20267},"obj":"http://purl.obolibrary.org/obo/UBERON_0000029"},{"id":"T290","span":{"begin":20336,"end":20347},"obj":"http://purl.obolibrary.org/obo/UBERON_0000029"},{"id":"T291","span":{"begin":20352,"end":20358},"obj":"http://purl.obolibrary.org/obo/UBERON_0002106"},{"id":"T292","span":{"begin":20546,"end":20547},"obj":"http://purl.obolibrary.org/obo/CLO_0001020"},{"id":"T293","span":{"begin":20623,"end":20633},"obj":"http://purl.obolibrary.org/obo/CLO_0001658"},{"id":"T294","span":{"begin":20827,"end":20835},"obj":"http://purl.obolibrary.org/obo/CL_0000576"},{"id":"T295","span":{"begin":20850,"end":20854},"obj":"http://purl.obolibrary.org/obo/UBERON_0002048"},{"id":"T296","span":{"begin":20850,"end":20854},"obj":"http://www.ebi.ac.uk/efo/EFO_0000934"},{"id":"T297","span":{"begin":21179,"end":21183},"obj":"http://purl.obolibrary.org/obo/GO_0005623"},{"id":"T298","span":{"begin":21336,"end":21341},"obj":"http://purl.obolibrary.org/obo/NCBITaxon_9606"},{"id":"T299","span":{"begin":21367,"end":21368},"obj":"http://purl.obolibrary.org/obo/CLO_0001020"},{"id":"T300","span":{"begin":21714,"end":21727},"obj":"http://purl.obolibrary.org/obo/UBERON_0002405"},{"id":"T301","span":{"begin":21886,"end":21889},"obj":"http://purl.obolibrary.org/obo/CLO_0054060"},{"id":"T302","span":{"begin":21972,"end":21981},"obj":"http://purl.obolibrary.org/obo/OBI_0100026"},{"id":"T303","span":{"begin":21972,"end":21981},"obj":"http://purl.obolibrary.org/obo/UBERON_0000468"},{"id":"T304","span":{"begin":22131,"end":22132},"obj":"http://purl.obolibrary.org/obo/CLO_0001020"},{"id":"T305","span":{"begin":22149,"end":22153},"obj":"http://purl.obolibrary.org/obo/GO_0005623"},{"id":"T306","span":{"begin":22178,"end":22179},"obj":"http://purl.obolibrary.org/obo/CLO_0001020"},{"id":"T307","span":{"begin":22307,"end":22310},"obj":"http://purl.obolibrary.org/obo/CLO_0051582"},{"id":"T308","span":{"begin":22568,"end":22573},"obj":"http://purl.obolibrary.org/obo/UBERON_0000178"},{"id":"T309","span":{"begin":22568,"end":22573},"obj":"http://www.ebi.ac.uk/efo/EFO_0000296"},{"id":"T310","span":{"begin":22862,"end":22867},"obj":"http://purl.obolibrary.org/obo/UBERON_0000178"},{"id":"T311","span":{"begin":22862,"end":22867},"obj":"http://www.ebi.ac.uk/efo/EFO_0000296"},{"id":"T312","span":{"begin":23252,"end":23257},"obj":"http://purl.obolibrary.org/obo/NCBITaxon_9606"},{"id":"T313","span":{"begin":23276,"end":23281},"obj":"http://purl.obolibrary.org/obo/NCBITaxon_10239"},{"id":"T314","span":{"begin":23422,"end":23423},"obj":"http://purl.obolibrary.org/obo/CLO_0001020"},{"id":"T315","span":{"begin":23472,"end":23473},"obj":"http://purl.obolibrary.org/obo/CLO_0001020"},{"id":"T316","span":{"begin":23513,"end":23518},"obj":"http://purl.obolibrary.org/obo/GO_0005623"},{"id":"T317","span":{"begin":23627,"end":23628},"obj":"http://purl.obolibrary.org/obo/CLO_0001020"},{"id":"T318","span":{"begin":23778,"end":23782},"obj":"http://purl.obolibrary.org/obo/GO_0005623"},{"id":"T319","span":{"begin":23963,"end":23970},"obj":"http://purl.obolibrary.org/obo/NCBITaxon_10239"},{"id":"T320","span":{"begin":24011,"end":24016},"obj":"http://purl.obolibrary.org/obo/NCBITaxon_10239"},{"id":"T321","span":{"begin":24153,"end":24160},"obj":"http://purl.obolibrary.org/obo/NCBITaxon_10239"},{"id":"T322","span":{"begin":24187,"end":24192},"obj":"http://purl.obolibrary.org/obo/NCBITaxon_10239"},{"id":"T323","span":{"begin":24194,"end":24199},"obj":"http://purl.obolibrary.org/obo/NCBITaxon_9606"},{"id":"T324","span":{"begin":24210,"end":24215},"obj":"http://purl.obolibrary.org/obo/NCBITaxon_10239"},{"id":"T325","span":{"begin":24221,"end":24226},"obj":"http://purl.obolibrary.org/obo/NCBITaxon_9606"},{"id":"T326","span":{"begin":24244,"end":24249},"obj":"http://purl.obolibrary.org/obo/NCBITaxon_10239"},{"id":"T327","span":{"begin":24556,"end":24557},"obj":"http://purl.obolibrary.org/obo/CLO_0001020"},{"id":"T328","span":{"begin":24610,"end":24617},"obj":"http://purl.obolibrary.org/obo/NCBITaxon_10239"},{"id":"T329","span":{"begin":24905,"end":24913},"obj":"http://purl.obolibrary.org/obo/CLO_0001658"},{"id":"T330","span":{"begin":24929,"end":24934},"obj":"http://purl.obolibrary.org/obo/NCBITaxon_10239"},{"id":"T331","span":{"begin":25026,"end":25033},"obj":"http://purl.obolibrary.org/obo/NCBITaxon_10239"},{"id":"T332","span":{"begin":25070,"end":25071},"obj":"http://purl.obolibrary.org/obo/CLO_0001020"},{"id":"T333","span":{"begin":25308,"end":25310},"obj":"http://purl.obolibrary.org/obo/CLO_0007815"},{"id":"T334","span":{"begin":25395,"end":25397},"obj":"http://purl.obolibrary.org/obo/CLO_0052906"},{"id":"T335","span":{"begin":26000,"end":26005},"obj":"http://purl.obolibrary.org/obo/NCBITaxon_10239"},{"id":"T336","span":{"begin":26081,"end":26086},"obj":"http://purl.obolibrary.org/obo/NCBITaxon_10239"},{"id":"T337","span":{"begin":26115,"end":26120},"obj":"http://purl.obolibrary.org/obo/NCBITaxon_10239"},{"id":"T338","span":{"begin":26142,"end":26150},"obj":"http://purl.obolibrary.org/obo/NCBITaxon_7157"},{"id":"T339","span":{"begin":26183,"end":26184},"obj":"http://purl.obolibrary.org/obo/CLO_0001020"},{"id":"T340","span":{"begin":26185,"end":26190},"obj":"http://purl.obolibrary.org/obo/NCBITaxon_10239"},{"id":"T341","span":{"begin":26680,"end":26687},"obj":"http://purl.obolibrary.org/obo/NCBITaxon_10239"},{"id":"T342","span":{"begin":27079,"end":27085},"obj":"http://purl.obolibrary.org/obo/UBERON_0003103"},{"id":"T343","span":{"begin":27223,"end":27225},"obj":"http://purl.obolibrary.org/obo/CLO_0008192"},{"id":"T344","span":{"begin":27251,"end":27256},"obj":"http://purl.obolibrary.org/obo/UBERON_0001456"},{"id":"T345","span":{"begin":27418,"end":27425},"obj":"http://purl.obolibrary.org/obo/OBI_0100026"},{"id":"T346","span":{"begin":27418,"end":27425},"obj":"http://purl.obolibrary.org/obo/UBERON_0000468"},{"id":"T347","span":{"begin":27508,"end":27509},"obj":"http://purl.obolibrary.org/obo/CLO_0001020"},{"id":"T348","span":{"begin":27567,"end":27571},"obj":"http://purl.obolibrary.org/obo/UBERON_0001456"},{"id":"T349","span":{"begin":27644,"end":27651},"obj":"http://purl.obolibrary.org/obo/OBI_0100026"},{"id":"T350","span":{"begin":27644,"end":27651},"obj":"http://purl.obolibrary.org/obo/UBERON_0000468"},{"id":"T351","span":{"begin":27977,"end":27982},"obj":"http://purl.obolibrary.org/obo/NCBITaxon_10239"},{"id":"T352","span":{"begin":27995,"end":27999},"obj":"http://purl.obolibrary.org/obo/GO_0005623"},{"id":"T353","span":{"begin":28320,"end":28321},"obj":"http://purl.obolibrary.org/obo/CLO_0001020"},{"id":"T354","span":{"begin":28337,"end":28340},"obj":"http://purl.obolibrary.org/obo/PR_000001343"},{"id":"T355","span":{"begin":28376,"end":28377},"obj":"http://purl.obolibrary.org/obo/CLO_0001020"},{"id":"T356","span":{"begin":28515,"end":28518},"obj":"http://purl.obolibrary.org/obo/CLO_0051582"},{"id":"T357","span":{"begin":28570,"end":28571},"obj":"http://purl.obolibrary.org/obo/CLO_0001020"},{"id":"T358","span":{"begin":28590,"end":28593},"obj":"http://purl.obolibrary.org/obo/CLO_0051582"},{"id":"T359","span":{"begin":28666,"end":28667},"obj":"http://purl.obolibrary.org/obo/CLO_0001020"},{"id":"T360","span":{"begin":28845,"end":28850},"obj":"http://purl.obolibrary.org/obo/NCBITaxon_10239"},{"id":"T361","span":{"begin":29036,"end":29039},"obj":"http://purl.obolibrary.org/obo/CLO_0001053"},{"id":"T362","span":{"begin":29058,"end":29063},"obj":"http://purl.obolibrary.org/obo/UBERON_0007688"},{"id":"T363","span":{"begin":29192,"end":29196},"obj":"http://purl.obolibrary.org/obo/OGG_0000000002"},{"id":"T364","span":{"begin":29253,"end":29260},"obj":"http://purl.obolibrary.org/obo/NCBITaxon_10239"},{"id":"T365","span":{"begin":29285,"end":29291},"obj":"http://purl.obolibrary.org/obo/NCBITaxon_9796"},{"id":"T366","span":{"begin":29331,"end":29335},"obj":"http://purl.obolibrary.org/obo/OGG_0000000002"},{"id":"T367","span":{"begin":29345,"end":29350},"obj":"http://purl.obolibrary.org/obo/GO_0005623"},{"id":"T368","span":{"begin":29408,"end":29413},"obj":"http://purl.obolibrary.org/obo/NCBITaxon_10239"},{"id":"T369","span":{"begin":29454,"end":29455},"obj":"http://purl.obolibrary.org/obo/CLO_0001020"},{"id":"T370","span":{"begin":29496,"end":29502},"obj":"http://purl.obolibrary.org/obo/UBERON_0000473"},{"id":"T371","span":{"begin":29633,"end":29634},"obj":"http://purl.obolibrary.org/obo/CLO_0001020"},{"id":"T372","span":{"begin":29671,"end":29678},"obj":"http://purl.obolibrary.org/obo/NCBITaxon_10239"},{"id":"T373","span":{"begin":29786,"end":29790},"obj":"http://purl.obolibrary.org/obo/GO_0005623"},{"id":"T374","span":{"begin":29857,"end":29858},"obj":"http://purl.obolibrary.org/obo/CLO_0001020"},{"id":"T375","span":{"begin":29996,"end":29997},"obj":"http://purl.obolibrary.org/obo/CLO_0001020"},{"id":"T376","span":{"begin":30070,"end":30071},"obj":"http://purl.obolibrary.org/obo/CLO_0001020"},{"id":"T377","span":{"begin":30164,"end":30165},"obj":"http://purl.obolibrary.org/obo/CLO_0001020"},{"id":"T378","span":{"begin":30176,"end":30182},"obj":"http://purl.obolibrary.org/obo/CLO_0001658"},{"id":"T379","span":{"begin":30235,"end":30236},"obj":"http://purl.obolibrary.org/obo/CLO_0001020"},{"id":"T380","span":{"begin":30336,"end":30337},"obj":"http://purl.obolibrary.org/obo/CLO_0001020"},{"id":"T381","span":{"begin":30435,"end":30441},"obj":"http://purl.obolibrary.org/obo/UBERON_0007688"},{"id":"T382","span":{"begin":30445,"end":30449},"obj":"http://purl.obolibrary.org/obo/OGG_0000000002"},{"id":"T383","span":{"begin":30556,"end":30559},"obj":"http://purl.obolibrary.org/obo/CLO_0051582"},{"id":"T384","span":{"begin":30599,"end":30605},"obj":"http://purl.obolibrary.org/obo/UBERON_0007688"},{"id":"T385","span":{"begin":30714,"end":30721},"obj":"http://purl.obolibrary.org/obo/NCBITaxon_10239"},{"id":"T386","span":{"begin":30856,"end":30860},"obj":"http://purl.obolibrary.org/obo/OGG_0000000002"},{"id":"T387","span":{"begin":31497,"end":31505},"obj":"http://purl.obolibrary.org/obo/UBERON_0000158"},{"id":"T388","span":{"begin":31515,"end":31518},"obj":"http://purl.obolibrary.org/obo/CLO_0051582"},{"id":"T389","span":{"begin":31536,"end":31537},"obj":"http://purl.obolibrary.org/obo/CLO_0001020"},{"id":"T390","span":{"begin":31671,"end":31678},"obj":"http://purl.obolibrary.org/obo/NCBITaxon_10239"},{"id":"T391","span":{"begin":31727,"end":31728},"obj":"http://purl.obolibrary.org/obo/CLO_0001020"},{"id":"T392","span":{"begin":31842,"end":31847},"obj":"http://purl.obolibrary.org/obo/CLO_0009985"},{"id":"T393","span":{"begin":31856,"end":31861},"obj":"http://purl.obolibrary.org/obo/UBERON_0007688"},{"id":"T394","span":{"begin":31888,"end":31893},"obj":"http://purl.obolibrary.org/obo/NCBITaxon_10239"},{"id":"T395","span":{"begin":31928,"end":31933},"obj":"http://purl.obolibrary.org/obo/UBERON_0000178"},{"id":"T396","span":{"begin":31928,"end":31933},"obj":"http://www.ebi.ac.uk/efo/EFO_0000296"},{"id":"T397","span":{"begin":32120,"end":32125},"obj":"http://purl.obolibrary.org/obo/NCBITaxon_10239"},{"id":"T398","span":{"begin":32146,"end":32150},"obj":"http://purl.obolibrary.org/obo/GO_0005623"},{"id":"T399","span":{"begin":32189,"end":32190},"obj":"http://purl.obolibrary.org/obo/CLO_0001020"},{"id":"T400","span":{"begin":32221,"end":32222},"obj":"http://purl.obolibrary.org/obo/CLO_0001020"},{"id":"T401","span":{"begin":32362,"end":32363},"obj":"http://purl.obolibrary.org/obo/CLO_0001020"},{"id":"T402","span":{"begin":32458,"end":32465},"obj":"http://purl.obolibrary.org/obo/NCBITaxon_10239"},{"id":"T403","span":{"begin":32761,"end":32766},"obj":"http://purl.obolibrary.org/obo/NCBITaxon_10239"},{"id":"T404","span":{"begin":33011,"end":33018},"obj":"http://purl.obolibrary.org/obo/NCBITaxon_10239"},{"id":"T405","span":{"begin":33031,"end":33035},"obj":"http://purl.obolibrary.org/obo/GO_0005623"},{"id":"T406","span":{"begin":33148,"end":33152},"obj":"http://purl.obolibrary.org/obo/GO_0005623"},{"id":"T407","span":{"begin":33228,"end":33233},"obj":"http://purl.obolibrary.org/obo/NCBITaxon_10239"},{"id":"T408","span":{"begin":33285,"end":33290},"obj":"http://purl.obolibrary.org/obo/NCBITaxon_10239"},{"id":"T409","span":{"begin":33311,"end":33315},"obj":"http://purl.obolibrary.org/obo/GO_0005623"},{"id":"T410","span":{"begin":33397,"end":33402},"obj":"http://purl.obolibrary.org/obo/NCBITaxon_10239"},{"id":"T411","span":{"begin":33442,"end":33447},"obj":"http://purl.obolibrary.org/obo/NCBITaxon_10239"},{"id":"T412","span":{"begin":33489,"end":33494},"obj":"http://purl.obolibrary.org/obo/GO_0005623"},{"id":"T413","span":{"begin":33730,"end":33735},"obj":"http://purl.obolibrary.org/obo/NCBITaxon_10239"},{"id":"T414","span":{"begin":33750,"end":33755},"obj":"http://purl.obolibrary.org/obo/GO_0005623"},{"id":"T415","span":{"begin":33845,"end":33849},"obj":"http://purl.obolibrary.org/obo/GO_0005623"},{"id":"T416","span":{"begin":33850,"end":33858},"obj":"http://purl.obolibrary.org/obo/UBERON_0000158"},{"id":"T417","span":{"begin":33863,"end":33864},"obj":"http://purl.obolibrary.org/obo/CLO_0001020"},{"id":"T418","span":{"begin":34055,"end":34062},"obj":"http://purl.obolibrary.org/obo/NCBITaxon_10239"},{"id":"T419","span":{"begin":34093,"end":34098},"obj":"http://purl.obolibrary.org/obo/NCBITaxon_10239"},{"id":"T420","span":{"begin":34123,"end":34124},"obj":"http://purl.obolibrary.org/obo/CLO_0001020"},{"id":"T421","span":{"begin":34125,"end":34129},"obj":"http://purl.obolibrary.org/obo/UBERON_0002048"},{"id":"T422","span":{"begin":34125,"end":34129},"obj":"http://www.ebi.ac.uk/efo/EFO_0000934"},{"id":"T423","span":{"begin":34354,"end":34356},"obj":"http://purl.obolibrary.org/obo/CLO_0050050"},{"id":"T424","span":{"begin":34647,"end":34649},"obj":"http://purl.obolibrary.org/obo/CLO_0050050"},{"id":"T425","span":{"begin":34703,"end":34708},"obj":"http://purl.obolibrary.org/obo/NCBITaxon_10239"},{"id":"T426","span":{"begin":34770,"end":34771},"obj":"http://purl.obolibrary.org/obo/CLO_0001020"},{"id":"T427","span":{"begin":35027,"end":35028},"obj":"http://purl.obolibrary.org/obo/CLO_0001020"},{"id":"T428","span":{"begin":35891,"end":35892},"obj":"http://purl.obolibrary.org/obo/CLO_0001020"},{"id":"T429","span":{"begin":35985,"end":35991},"obj":"http://purl.obolibrary.org/obo/CLO_0001658"},{"id":"T430","span":{"begin":36473,"end":36476},"obj":"http://purl.obolibrary.org/obo/PR_000001343"},{"id":"T431","span":{"begin":36591,"end":36596},"obj":"http://purl.obolibrary.org/obo/GO_0005623"},{"id":"T432","span":{"begin":36713,"end":36715},"obj":"http://purl.obolibrary.org/obo/CLO_0007622"},{"id":"T433","span":{"begin":37032,"end":37037},"obj":"http://purl.obolibrary.org/obo/GO_0005623"},{"id":"T434","span":{"begin":37047,"end":37052},"obj":"http://purl.obolibrary.org/obo/GO_0005623"},{"id":"T435","span":{"begin":37198,"end":37199},"obj":"http://purl.obolibrary.org/obo/CLO_0001020"},{"id":"T436","span":{"begin":37235,"end":37237},"obj":"http://purl.obolibrary.org/obo/CLO_0007622"},{"id":"T437","span":{"begin":37412,"end":37418},"obj":"http://purl.obolibrary.org/obo/UBERON_0001969"},{"id":"T438","span":{"begin":37419,"end":37427},"obj":"http://purl.obolibrary.org/obo/UBERON_0000158"},{"id":"T439","span":{"begin":37496,"end":37501},"obj":"http://purl.obolibrary.org/obo/GO_0005623"},{"id":"T440","span":{"begin":37956,"end":37958},"obj":"http://purl.obolibrary.org/obo/CLO_0008192"}],"text":"Nanotechnology Tools to Inactivate SARS-CoV-2 in Patients\nThe main target of SARS-CoV-2 is the respiratory tract (upper airways, lung),36 although other organs might also be infected (e.g., gut, kidney) and vasculature21 also appears to be a prime target. The expression of ACE2 probably determines uptake by different tissues.37\nIn addition to discussing immune-based approaches, because the lung is the most critically affected organ, we will center our discussion on the various options to inactivate the virus in the deep lung and to target the essential host cells for drug delivery. The virus reaches the alveoli and enters alveolar epithelial type II cells (AECII), due to the relatively high abundance of ACE2 and a permissive cellular milieu. These cells serve as a reservoir of the virus, which finally spreads throughout the lung, leading to the lung function impairment seen in severe cases. Airborne nanomaterials are optimally suited to penetrate into the deep lung due to the physicochemical properties of such aerosols, existing on the same size scale particles that penetrate most readily to the deep airways. Hence, nanomedicine is already actively pursuing ideas to deliver drugs, therapeutic proteins, and mRNAs by exploiting nanodevices for pulmonary delivery.38,39\nMoreover, the rapid emergence of SARS-CoV-2 has exposed one of the main weaknesses in the current medical landscape: the lack of broad-spectrum antiviral drugs. At present, there are only a handful of approved antivirals, and they are mostly virus-specific. Hence, when a new virus emerges, little can be done pharmacologically to slow down its spread. Some research efforts have been focused on the development of broad-spectrum drugs, which could potentially offer some efficacy against future emerging viruses (and maybe SARS-CoV-2). The various approaches developed over the years are mainly based on the creation of entry inhibitors.40,41 A highly conserved part in viruses is the attachment ligand (VAL). In most known respiratory viruses,42 the VAL targets either heparan sulfate proteoglycans (HSPG)43 or sialic acids (SA).44 Both HSPG and SA mimics have shown in vitro ability to bind to viruses, blocking their interaction with cell membranes, and often in a broad-spectrum way.45−47\nIn the context of nanomedicine, many nanomaterials have been developed, ranging from polymers48 to dendrimers,49 oligomers, NPs,50 liposomes,51 and small molecules.52 However, successful clinical translation has been hindered by the fact that, upon dilution, these compounds lose efficacy as the virus-compound complex dissociates leaving viruses free to restart their replication cycle. Recently, it has been shown that this limitation can be overcome by synthesizing NPs that, after binding, are able to inhibit viral infectivity irreversibly by permanently damaging the virion, refueling the hope for a true, broad-spectrum antiviral drug.53 Because the focus is also on the development of a drug specific to SARS-CoV-2, a good entry inhibitor could be based on blocking the S spike protein interaction with the cellular ACE2 receptor.19,21−23 Regardless of the specific approach, it is imperative that novel, effective antivirals be based on compounds that exhibit very low or negligible toxicity profiles, as patients will most likely need to receive those drugs for extended periods of time and will already be weakened. For these reasons, when designing antiviral drugs, clearance mechanisms have to be kept in mind. An example of this process is the recent redesign of broad-spectrum antiviral NPs into equally effective modified cyclodextrins.54 Moreover, nanotechnology may offer nanotheranostic approaches to fill the existing gap between diagnostics and therapy.55−57 The simultaneous management of both diagnostics and therapy for those suffering from COVID-19 or in future pandemics, as for many other diseases, is an additional potential strategy to take into consideration in which nanomaterials have proven to be effective tools. The advantages of the capabilities of nanotechnology and nanomaterials for combined therapeutics and diagnostics has been widely explored in cancer research; however, there have been considerable efforts in the past few years to extend the scope of this approach to other areas including infectious diseases.58\n\nNanomaterial-Based Vaccine Development and Immunomodulation\nFollowing the publication of the genetic sequence of SARS-CoV-2 on January 11, 2020, intense research efforts have been devoted to developing a vaccine against COVID-19. With unprecedented speed, this extraordinary scientific mobilization led the first vaccine candidate to enter the Phase I human clinical trial on March 16, 2020, and other novel candidates are rapidly following.59 Up to May 22, 2020, there are 10 COVID-19 candidate vaccines in clinical evaluations and 114 in preclinical development.60\nConcerning vaccine and immunization research, nanomaterials can assist in multiple ways to boost the upregulation required by the immune system and to direct the immune response specifically against antigens. Immune-targeted nanotherapeutics can be developed through their rational manufacture at the nanoscale level by designing nanomaterials that are able to amplify host’s immune response, for instance as adjuvants in the context of vaccination.\nThe development of a vaccine will rely either on the direct administration of viral antigens (e.g., in the form of recombinant proteins, vectored vaccines, or whole inactivated or attenuated virus) or RNA- or DNA-encoding viral antigens.27 Candidate antigens for immunization are surface proteins such as the immunogenic spike protein (S1), which is already targeted by antibodies of convalescent patients.27 Because the S1 protein is also essential for cellular uptake, many researchers are using this protein as the primary target for a vaccine.\nThere are many issues related to the delivery of a drug, protein, or RNA into the patient as the cargo is often degraded, not bioavailable, or is swiftly cleared. Nanotechnology provides multiple solutions to these challenges though, as nanocarriers can overcome some of these limitations.\nBiocompatible polymeric-, lipid-based, or inorganic NPs can be tuned with respect to their physicochemical properties to encapsulate cargo proteins with high loading efficiency, improving protein delivery and pharmacokinetics over conventional approaches.38 Intranasal delivery of polymer-encapsulated antigen triggers a strong immune response, and the success of vaccination depends on the appropriate type of polymer in combination with the antigen.61,62 Similarly, researchers have developed lipid and lipid-based NPs as delivery platforms for mRNAs or siRNAs to enable the synthesis of key viral proteins for vaccination or to inactivate critical viral target genes, respectively.39 The field of therapeutic mRNAs to support vaccination has gained momentum, as well,63 at least in part due to nanotechnology-enabled strategies for cargo delivery. Moreover, the versatility of nanoplatforms as antigen-presenting systems offers great opportunities. Considering the possibility of random viral mutations that may alter the antigen shape, functionalizing nanomaterials with a wide number of molecules at the same time to target the virus, or motifs that are specific for pathogens, will increase the efficiency of vaccines and their ability to prevent viral infection.\nSeveral companies are working on mRNA vaccines encoding SARS-CoV-2 proteins such as the spike protein, encapsulated in nanoliposomes with specific physicochemical properties that are potentially akin to those documented for immunization against certain tumor antigens.64 The design of such nanocarriers, which will need to escape recognition by scavenger cells and to be nontoxic and nonimmunogenic, is a challenge that will require substantial time prior to clinical availability.\nOn March 16, 2020, Moderna, through a partnership with the Vaccine Research Center at the U.S. National Institutes of Health, enrolled the first participants into a Phase I clinical trial testing an mRNA vaccine (mRNA-1273) encapsulated in lipid NPs—a record time of just 63 days following sequence selection (NCT04283461).65 The enrollment of the first cohort of participants (18 to 55-year-old healthy subjects) concluded on April 16, 2020.66 CureVac and BioNTech (in partnership with Pfizer) are currently working on similar vaccines; Pfizer/BioNTech, in particular, have recently started the recruitment in Phase I/II trials (NCT04368728, NCT04380701). A DNA plasmid vaccine by Inovio Pharmaceuticals (INO-4800) has showed promising results in mice and guinea pigs according to a recent article published in Nature Communications(67) and has entered Phase I testing in humans (NCT04336410).\nAnother candidate COVID-19 vaccine for Phase I clinical trial is from the University of Oxford and AstraZeneca (NCT04324606).68 Around 1110 people will take part in the trial, which started recruitment at the end of April 2020. The vaccine is based on a chimpanzee adenovirus vaccine vector (ChAdOx1) and the SARS-CoV-2 spike protein. Chimpanzee adenoviral vectors against different pathogens have been already tested in thousands of subjects with demonstrated safety. To date, ChAdOx1 has been administered to six rhesus macaques exposed to high doses of SARS-CoV-2. The vaccine was unable to prevent infections, although it reduced the severity of the disease: no signs of virus replication were observed in the lungs, with significantly lower levels of respiratory disease and no lung damage compared to control animals, according to a recent article deposited in bioRxiv.69 Another adenoviral vector vaccine developed by CanSino Biological Inc. and Beijing Institute of Biotechnology using a genetically engineered replication-defective adenovirus type 5 vector to express the SARS-CoV-2 spike protein (Ad5-nCoV) is currently being tested in Phase I/II trials (NCT04398147, NCT04341389, NCT0431312).\nAlthough vaccines based on novel DNA and mRNA technologies might be promising, there are no such kinds of vaccines on the market, and it is unknown whether they can be effective in humans. Moderna, for instance, has generated preliminary safety data on different mRNA-based vaccines targeting other respiratory viruses, but their more advanced program (on a cytomegalovirus vaccine) is still in Phase II clinical testing. However, on May 18, Moderna announced that mRNA-1273 elicited antibody titers above the levels observed in convalescent individuals (and therefore considered potentially protective) in all eight initial participants across the 25 and 100 μg dose cohorts of the Phase I trial (NCT04283461).70 The company, after having received a fast-track approval from the FDA, is now moving on Phase II trials.\nConversely, strong evidence exists regarding the efficacy of protein-based vaccines such as recombinant-protein, viral-vector, attenuated, or inactivated vaccines across different infectious diseases, with licensed vaccines already existing for all of these platforms.27 All of the aforementioned approaches are currently being explored in the context of SARS-CoV-2.27 An inactivated vaccine developed by the China National Pharmaceutical Group (Sinopharm), in collaboration with the Wuhan Institute of Biological Products, is currently tested in a Phase I/II trial (ChiCTR2000031809), and a second inactivated vaccine (in collaboration with the Beijing Institute of Biological Products) has been currently approved for clinical testing (ChiCTR2000032459). A third aluminum salt (alum) adjuvanted inactivated vaccine, developed by Beijing-based Sinovac Biotech’s, was able to provide partial or complete protection in rhesus macaques, according to a recent article published in Science,71 and is currently being tested in Phase I/II trials (NCT04383574, NCT04352608).\nLive-attenuated vaccines are intrinsically immunogenic (e.g., due to the presence of viral DNA), but extensive safety tests are required due to the rare possibility of reversion to a pathogenic form able to cause infection. Vaccine candidates based in this application have previously been designed for SARS-CoV with high stability.72,73 Recombinant-protein vaccines and inactivated vaccines are safer but might require adjuvants to increase their immunogenicity. In the context of SARS-CoV-2, adjuvants are important for two reasons. First, adjuvants might increase the efficacy of the vaccine, especially in subjects with impaired immunological function, such as the elderly, or in subjects with comorbidities resulting in immune dysfunctions; in these patient cohorts, SARS-CoV-2 has a high lethality rate. Second, adjuvants can reduce the amount of vaccine protein(s) required per dose, which could facilitate scaling-up vaccine production in a reduced time frame.\nBeyond alum, which is in fact a nanoscale material74 that was developed in the 1920s for the tetanus and diphtheria toxoids,75 approval for a new adjuvant did not occur until 1997, with the introduction of the oil-in-water emulsion of squalene oil and polysorbate 80 and sorbitan trioleate surfactants (MF59) in the seasonal influenza vaccine for the elderly.76 The use of MF59 was further expanded to pandemic and avian influenza vaccines.77 Other adjuvants in licensed vaccines have been approved since 2000, as well: (a) AS03 (used for pandemic and avian influenza vaccines), similar to MF59, but including α-tocopherol as an additional immune stimulant; (b) AF 03 (used for pandemic influenza vaccines), an alternative squalene emulsion containing polyoxyethylene, cetostearyl ether, mannitol, and orbitan oleate;78 (c) AS 01 (used for herpes zoster vaccine), a liposome-based vaccine adjuvant system containing two immunostimulants, 3-O-desacyl-4′-monophosphoryl lipid A (MPL, a Toll-like receptor 4 agonist) and saponin QS-21, which activates the ACT-NLRP3 inflammasome pathway;79 and (d) AS04 (used for hepatitis B and human papilloma virus vaccines), which is a combination of MPL and aluminum hydroxide.76,80\nIn this context, the concept of “nanoimmunity by design” relies on the rational design of distinct physicochemical properties and specific functionalization of nanomaterials intended for fine-tuning their potential effects on the immune system.81 Nanomaterials have emerged as promising tools for immune modulation, either stimulating or suppressing the immune response. In fighting SARS-CoV-2, these properties may find applications for both prevention and therapy and in the context of vaccine development.\nMounting evidence indicates that nanomaterials such as graphene,82 nanodiamonds,83 carbon nanotubes,84 and polystyrene particles85 bear an intrinsic capacity to activate the immune system, depending on their functionalization.86 For instance, graphene oxide functionalized with amino groups (GO-NH2) induces activation of STAT1/IRF1 interferon signaling in monocytes and T cells, resulting in the production of T cell chemoattractants, and macrophage 1 (M1) 1/T-helper 1 (Th1) polarization of the immune response, with negligible toxicity.82 Remarkably, the ability of licensed adjuvants such as AS01 and AS03 to enhance adaptive immunity has been linked to their capacity to boost STAT1/IRF1 interferon signaling.87 In addition, recent SARS-CoV and MERS-CoV studies suggest that the development of a Th1-type response is central for controlling infection, which also may be true for SARS-CoV-2.88\nSeveral groups and consortia have been screening and characterizing nanomaterials according to their immunomodulatory properties and absence of cytotoxicity.56,57,82−84,86 The development of an adjuvant for clinical use is a lengthy process that requires extensive Phase III randomized trials in large and diverse cohorts of subjects, and the process generally requires several years.76 Pharmaceutical giants such as GlaxoSmithKline (GSK), which owns the ASs mentioned above and other adjuvant platforms, are engaged in several partnerships to embed their adjuvant systems with SARS-CoV-2-protein-based vaccines. A full-length recombinant SARS-CoV-2 glycoprotein nanoparticle vaccine adjuvanted with the saponin-based Matrix M developed by Novavax is in Phase I clinical testing (NCT04368988).89\nAlthough it is unlikely that novel adjuvants would be used in the context of the current pandemic, the SARS-CoV-2 pandemic offers an opportunity to reflect on the potential of nanotechnology for vaccine adjuvant development. In this context, it is critical to stream coherent pipelines covering in vitro and in vivo experiments specifically to select candidate materials that might be tested for clinical implementation as vaccine adjuvants.90\nIn this view, the status of nanomaterial-based vaccine adjuvants has been reviewed.91,92 In particular, immunomodulatory effects induced on the innate immune signaling have been demonstrated.91 For example, nanomaterials such as GO can elicit an inflammasome sensor (NLRP3)-dependent expression of IL-1β in macrophages.93 Notably, alum, the most commonly used adjuvant in human vaccines, induces the release of this cytokine in macrophages through the same NRLP-induced mechanism. These results suggest that nanomaterials such as GO and alum may be useful for medical applications.94\nConsidering that new vaccine development typically requires years to be approved and applied to the general population, there is also an urgent need to study how to improve treatment approaches. According to the first Phase III clinical studies, the antiviral drug Remdesivir, which was recently approved by the FDA for COVID-19 treatment in the United States, seems to be a promising treatment for adults diagnosed with COVID-19. Nano-based strategies have already been applied to enhance the effectiveness of Remdesivir in the context of other emerging viral infections (Nipah virus),95 suggesting the suitability of nanotechnology to assist with similar strategies for the treatment of COVID-19 as well as other possible pandemics in the future.96\n\nCan Nanotechnology Help to Control the Cytokine Storm?\nOne of the main features of COVID-19 is the triggering of a cytokine storm in the body, also known as cytokine release syndrome (CRS), which results from an excessive immune response and leads to the severe deterioration of patient health.25,97,98 This inflammatory storm is one of the major causes of the acute respiratory distress syndrome (ARDS) that is often associated with multiple-organ failure, representing the leading causes of death in critical patients.98 In particular, the role of interleukin (IL)-6 has been highlighted in patients requiring assisted ventilation. Ongoing clinical trials are testing drugs that block the receptor of IL-6 (Tocilizumab, an anti-IL-6 receptor antibody, and Sarilumab) or IL-6 itself (Siltuximab).\nWhereas a well-regulated cytokine response that is rapidly triggered by the host’s innate immunity can serve to prevent and to counteract an infection, an excessive, unbalanced, prolonged immune response can seriously harm the body. Therefore, therapeutic strategies aimed at effectively suppressing the cytokine storm are under investigation. Nanomaterials have been exploited to adjust the immune response to an optimized level, and such proprieties might be explored to inhibit cytokine releases.99 Nanosystems can enhance the specificity/efficiency of immunosuppressant delivery to target immune cells, with consequent reductions in drug dose, drug distribution to nontarget tissues and organs, and possible side effects. In addition, specific nanotools can be designed to evade the immune system and to enhance the solubility of poorly soluble immunosuppressant agents; the potential of finely tuning their surface charge opens possibilities for encapsulation strategies and offers accommodation for a high drug load. All of these mechanisms may also occur simultaneously, enhancing the activity of immunosuppressive agents.\nConcerning the role of macrophages in COVID-19, the presence of ACE2-expressing CD68+CD169+ macrophages containing SARS-CoV-2 nucleoprotein antigen and showing an increased release of IL-6 was observed in infected spleen and lymph nodes.100 Notably, immunohistochemical and immunofluorescence analyses of lymph nodes and spleen tissue from autopsy samples of patients who died from COVID-19 revealed lymphocytic apoptosis. The tissues infected by SARS-CoV-2 also showed an upregulated expression of Fas, suggesting a role for CD169+ macrophages in viral spreading, aberrant inflammation, and activation-induced lymphocyte apoptosis. Moreover, histological examinations of biopsy samples of patients who died from COVID-19 revealed an increased alveolar exudate due to the extended neutrophil and monocyte infiltrate in lung capillaries with fibrin deposition, probably leading to difficulties in gas exchange. Through nanomedicine, we envision therapeutic approaches aimed at targeting specific immune subpopulations to avoid these complications, and different nanomaterials have already been explored for their specific impact on different immune cell subpopulations.82,83,86 Octadecylamine-functionalized and dexamethasone-adsorbed nanodiamond promotes anti-inflammatory and proregenerative behavior in human macrophages in vitro.101 A low dose of this functionalized nanodiamond also reduced macrophage infiltration and expression of proinflammatory mediators iNOS and tumor necrosis factor (TNF)-α in mice. Overall, these results suggest that nanodiamond particles may be useful as an inherently immunomodulatory platform.\nFinally, the indiscriminate systemic suppression of the immune system may increase the susceptibility to sepsis due to underlying secondary bacterial infections that can exacerbate critical conditions in patients, causing death.102 Risk factors for mortality include acquired bacterial infections by opportunistic organisms and primary pathogens. Using nanocarriers to achieve targeted delivery of immunosuppressive drugs, or the specific and controlled inhibition of only a specific immune cell subpopulation, can play a critical role in limiting such complications.\nThe fast and complete removal of pro-inflammatory cytokines from the bloodstream has been shown to increase survival significantly in patients with early stage sepsis. Adsorption of cytokines from serum by extracorporeal perfusion through porous carbons is one of the most promising ways for their selective, quick, and complete removal from blood circulation. Hierarchical carbon materials with tuned porosity have shown effective adsorption of many cytokines, including IL-6 and even the largest ones, such as TNF-α.103 Based on their demonstrated outstanding performance in removing sepsis-associated pro-inflammatory cytokines from blood, mesoporous carbon adsorbents with hierarchical structure, tuned pore size, and surface chemistry, as well as graphene with open and accessible surfaces104 have significant potential to quickly remove inflammatory cytokines associated with CRS and to prevent mortality arising from an uncontrolled inflammatory cascade, thereby providing enough time for the defense mechanisms of the human body to fight the virus.\n\nPhotodynamic Inactivation of SARS-CoV-2\nIn addition to drug- and vaccine-based antiviral strategies, photodynamic therapy (PDT) stands as a unique approach to inactivate SARS-CoV-2. Using a light-based method, PDT attacks target cells via the excitation of photosensitive agents, called photosensitizers (PSs), with radiation characterized by a wavelength corresponding to its absorption spectrum to generate reactive oxygen species (ROS) in the presence of oxygen, which ultimately results in cell death. Photodynamic therapy is primarily used for the clinical treatment of various oncological disorders.105 It was not until the 1970s that PDT was first used clinically against viruses,106 exploiting ROS production to damage virus proteins, nucleic acids, and—if present—lipids.107 Even though there are research efforts for PDT-based virotherapies against different viruses, including herpes simplex virus, human papilloma virus, and human immunodeficiency virus,106−109 clinical use of PDT is limited due to hydrophobicity of PSs, poor target specificity, and limited tissue penetration ability.\nMost PSs are hydrophobic and aggregate in aqueous solutions, affecting their photochemical and photobiological properties.106,110 For this reason, Lim et al. have proposed a promising approach for photodynamic inactivation of viruses with NPs, developing sodium yttrium fluoride (NaYF4) upconversion NPs (UCNs) with zinc phthalocyanine PSs grafted onto their surfaces. Unlike most PSs, these UCNs are coated with polyethylenimine (PEI), which render them hydrophilic and easier to manipulate. These UCNs showed antiviral activity against Dengue virus serotype 2 and adenovirus type 5, which were used as models of enveloped and non-enveloped viruses, respectively.110 MXenes111,112 are a large family of 2D transition metal carbides, nitrides,113 and carbonitrides114 that exhibit unique electronic, optical, and catalytic properties. They have the general formula Mn+1XnTx, where M is an early transition metal (Ti, Zr, V, Mo, etc.), X is C and/or N, Tx represents the surface functional groups (=O, −OH, −F, −Cl), and n = 1–4.115,116 Some examples include Ti3C2Tx, V2CTx, and Nb2CTx, with over 30 stoichiometric compositions already synthesized with more than 100 predicted. Biocompatible MXenes, such as Ti3C2Tx, are hydrophilic and are among the most efficient light-to-heat transforming materials.117 The plasmon resonance extinction maxima of Ti3C2Tx is at 780 nm, enabling the use of near-infrared (IR) light for PDT. Several other MXenes have absorption maxima in the IR range and have shown outstanding performance in PDT and theranostic applications.118\nFullerene and graphene are also good candidates for virus inactivation by PDT and have proved to be effective against Semliki Forest virus (SFV), vesicular stomatitis virus (VSV), HSV-1, HIV-1, mosquito iridovirus (MIV), and influenza A virus (IAV), as well as the phage MS2.107 In addition, several 2D nanomaterials, including graphene-based materials, MXenes, black phosphorus, graphitic carbon nitride, tungsten disulfide, and molybdenum disulfide, have been reported to improve the efficacy of PDT considerably for cancer treatment.56,57 Therefore, determining if such nanomaterial-based PDT protocols could be exploited to inactivate SARS-CoV-2 is of great interest.\n\nBiomimetic Engineering of Nanodelivery Systems: Artificial Viruses in the Making\nIn an effort to engineer the next generation of nanoscale vectors, scientists have moved from using inorganic components aimed at obtaining inert structures to utilizing biological building blocks that are able to convey additional functionalities to the resulting construct. To cope with the complexity of the body and to evade the multiple layers of defense that tissues and organs have, it is critical to rely on the ability of certain materials to interact with, rather than to eschew, the biology of our body. Every NP system conceived to date faces one common fate: whether injected, inhaled, ingested, or absorbed through our epithelia, all will at some point come into contact with the mixture of fluids and organic compounds that comprise the body. Under such conditions, every material reacts in a unique way according to the conditions they individually face (i.e., the tissue or body region they are in), their composition (i.e., organic or inorganic), and their physical properties (i.e., size, shape, surface charge).119 Inorganic NPs can function as globular protein mimics because of their similar size, charge, shape, and surface features that can be chemically functionalized to resemble proteins. These similarities can be used in biotechnology to control virus pathways or cell receptor interactions with NPs.120\nAmong the many attempts, the legendary accomplishments, and the epic failures, we could list thousands of different NPs, differing from one another thanks to the creative endeavors of their designers. Despite their remarkable differences and researchers’ endeavors to make them one of a kind, they all aim to achieve one goal: to deliver in a specific way one particular form of payload, while remaining as unnoticed as possible by the body’s defense mechanisms. For everyone who has made an effort to create their own version of such a silver bullet, it has come to mind that nature in its incredible variety had already invented a few ingenious solutions to this problem. In the world of nano-based drug delivery, one entity dominates as the quintessential example of precision, efficiency, and stealth: the virus. Not by chance, these two worlds share many features that span from the physical laws that govern their assembly and stability to the chemical similarities in their overall composition.121\nHistorically, the field of nanomedicine and the global endeavor to generate NPs for drug delivery arose from one of the greatest struggles in medicine: gene therapy. At the core of that approach was the idea that viruses could be used as Trojan horses to deliver the correct sequence of the gene into the cells that harbored the mutated copy. In order to identify the virus that offered the best delivery service, a cadre of viral strains and species were tested, adapted, and engineered to fit the aims and hit the targets. It took decades of trial and error, of progressive adjustments, and a few tragic mistakes to identify the viruses that could provide the ideal backbone to develop viral vectors able to ferry genetic cargo into the target cell.122 In the midst of that global challenge, nanotechnology offered a safer and more controllable alternative: to generate bespoke structures that could replace viral vectors and do the same job, delivering a payload from the point of injection to the site of action.\nFast forward a few decades, and the promises made by both worlds seem to have finally become reality, with a number of active clinical trials, some clinical success stories, and a few commercial products in the market space. Interestingly, the two worlds seem to have maintained a safe distance so as to avoid any collision or dangerous proximity. Exchange of ideas between the fields of gene therapy and nanomedicine is limited, and the potential for disciplinary growth through knowledge exchange has remained elusive to scientists in both fields.5 Many scientists have suggested that nanotechnologists could find inspiration in the mechanisms devised by viruses to elude immune surveillance, to overcome biological barriers, and to deliver their genetic payload with high specificity. Similarly, gene therapists would find high-tech solutions to their scalability and safety issues in looking at the new generations of biomimetic particles being generated.\nThe principles of biomimicry and bioinspiration have been used to design and to engineer drug-delivery technologies that reproduce or recapitulate biological materials, for what pertains to not only their structure and chemistry but also, more importantly, their functions. In drug delivery, surface recognition and nanoscale interactions between materials and biological entities are key to the success of the delivery strategy, and the use of biological building blocks such as membrane proteins has been proposed as a way to convey targeting and shielding moieties simultaneously.123 One can only hope that the recent events and global attention that viruses are capturing in the scientific world will spur a renewed interest in finding ways to adapt viral features and mechanisms of action to the world of NPs. Increased focus in this field would be useful to create virus-like NPs able to circulate in the blood system, overcoming the endothelial barrier, and to deliver their therapeutic payload with high efficiency.124\n\nInterference with Cellular Uptake, Immobilization, and Inactivation of the Virus Outside of the Host Cell\nNanomaterials can be synthesized with a high specific surface area of a few hundred square meters per gram. Therefore, dependent on the surface properties, nanomaterials efficiently adsorb biomolecules and form a so-called biomolecular corona. This passive, nontargeted adsorption might be utilized to bind viruses, provided that the selected nanomaterial is relatively biocompatible. Viral surface proteins are often modified by sugar moieties or encompass positively charged amino acid patches that bind to lectins or glycosaminoglycans (GAGs) of heparan sulfate (HS), respectively.32 Robust interactions of virus particles with these host receptors is ensured by multivalent binding, which is likely why single-molecule inhibitors often are not capable of efficiently perturbing this key event but multivalent NPs are superior to block binding of different viruses to the host cell.125\nGold NPs capped with mercaptoethanesulfonate are effective inhibitors of HSV type 1 infection as they mimic cell-surface-receptor heparan sulfate and, therefore, competitively bind to the virus. Interestingly, polyvalent sulfated Au NPs inhibit virus binding to the host cell dependent on their size. Nanoparticles of diameters equal to and larger than the virus diameter (in this case, the stomatitis virus) more efficiently inhibit the binding to cells than smaller particles. Most likely, larger NPs efficiently cross-link virions, whereas smaller NPs simply decorate the viral surface.126 Papp et al. found that gold NPs decorated with SA effectively inhibited the binding of influenza virus to the target cells. In this case, viral recognition via its surface protein hemagglutinin of SA on the host cell membrane was a prerequisite for cellular entry.127 More recently, Cagno et al. reported antiviral NPs (Au and iron oxide core) with long and flexible linkers mimicking HS that strongly bind and inactivate viruses such as respiratory syncytial virus in vitro and in vivo in a lung infection model and even led to irreversible viral deformation.53 Hence, there is ample evidence that biocompatible, functionalized NPs can act as broad-spectrum antivirals. Notably, the receptor-binding domain of the spike S1 protein of SARS-CoV-2 binds not only to ACE2 but also to heparin128 and, thus, might be targeted by similar approaches as outlined above.\nOne of the most recent strategies to inhibit viral uptake is based on administration of recombinant ACE2 to inhibit binding competitively via the spike S1 protein.21 Knowing that multivalency is key to block virus–host interactions reliably, researchers have speculated that a nanostructured carrier could not only improve delivery and cargo stability but also might dramatically enhance binding strength.129\n\nSpeeding up the Nanomedicine-Based Approaches for COVID-19 by In Silico Analysis\nCurrently, repurposing drug molecules is a key strategy for identifying approved or investigational drugs outside the scope of the original medical indication that can be used to fight COVID-19.130,131 There are various advantages to this strategy, including already-established safety profiles, fast transition to clinical studies, and less investment needed, compared to the process of developing an entirely new drug.132,133 Therefore, these advantages have the potential to result in less risky and more rapid returns on investment in the development of repurposed drugs, benefits that are particularly important during pandemics. In addition to rapid diagnosis, making recommendations to physicians about rapid treatment methods can save the lives of many people. Combining in silico tools such as molecular docking, molecular dynamics, and computational chemistry with large drug databases provides a great advantage in selecting “possible candidates” from among thousands of pharmaceutically active substances (Figure 3). In silico analyses should be supported by the literature, expert opinions (pharmacologists and clinicians), and, when possible, preclinical and clinical findings. After selecting the best candidates following in silico analyses, detailed in vitro and in vivo experimentation should be undertaken prior to clinical studies. Recently, various drug-repurposing studies have been published to assist in the global COVID-19 pandemic response.134−136 Such studies aim to identify whether already-approved drugs have the capacity to interact with viral proteins or receptors on host cells.\nFigure 3 Schematic of how in silico analysis can be used to select candidate drug molecules for clinical studies. MD, molecular dynamics. In nanomedicine, computational models have recently garnered attention; such work may help to identify how nanomaterials interact with biological systems and to determine how the efficacy of these nanotherapeutics could be improved. Computational models indicate how NPs are taken up by healthy cells or tumor cells, enabling better predictions regarding the pharmacokinetic and pharmacodynamic properties of these materials.134 For example, Lunnoo et al. used a coarse-grained molecular dynamics (MD) simulation to observe the internalization pathways of various Au nanostructures (nanospheres, nanocages, nanorods, nanoplates, and nanohexapods) into an idealized mammalian plasma membrane.137 Other studies have simulated how different NPs can target tumor cells and deliver drugs.138,139 Therefore, in silico approaches that are currently used for drug repurposing, including molecular docking, molecular dynamics, and computational chemistry, constitute valuable tools to aid preclinical and clinical studies of nanomaterials directed for disease treatment. Considering the urgent need for nanomedicine against the current pandemic, in silico analyses may be especially useful in guiding the rational design of new NP formulations required to fight SARS-CoV-2."}
LitCovid-PD-CHEBI
{"project":"LitCovid-PD-CHEBI","denotations":[{"id":"T50","span":{"begin":574,"end":578},"obj":"Chemical"},{"id":"T51","span":{"begin":655,"end":657},"obj":"Chemical"},{"id":"T52","span":{"begin":1193,"end":1198},"obj":"Chemical"},{"id":"T53","span":{"begin":1212,"end":1220},"obj":"Chemical"},{"id":"T54","span":{"begin":1431,"end":1446},"obj":"Chemical"},{"id":"T55","span":{"begin":1431,"end":1440},"obj":"Chemical"},{"id":"T56","span":{"begin":1441,"end":1446},"obj":"Chemical"},{"id":"T57","span":{"begin":1497,"end":1507},"obj":"Chemical"},{"id":"T58","span":{"begin":1717,"end":1722},"obj":"Chemical"},{"id":"T59","span":{"begin":1914,"end":1924},"obj":"Chemical"},{"id":"T60","span":{"begin":1984,"end":1990},"obj":"Chemical"},{"id":"T61","span":{"begin":2058,"end":2073},"obj":"Chemical"},{"id":"T62","span":{"begin":2058,"end":2065},"obj":"Chemical"},{"id":"T63","span":{"begin":2066,"end":2073},"obj":"Chemical"},{"id":"T64","span":{"begin":2074,"end":2087},"obj":"Chemical"},{"id":"T65","span":{"begin":2100,"end":2112},"obj":"Chemical"},{"id":"T66","span":{"begin":2107,"end":2112},"obj":"Chemical"},{"id":"T67","span":{"begin":2114,"end":2116},"obj":"Chemical"},{"id":"T72","span":{"begin":2135,"end":2137},"obj":"Chemical"},{"id":"T77","span":{"begin":2394,"end":2403},"obj":"Chemical"},{"id":"T78","span":{"begin":2405,"end":2408},"obj":"Chemical"},{"id":"T79","span":{"begin":2435,"end":2444},"obj":"Chemical"},{"id":"T80","span":{"begin":2750,"end":2753},"obj":"Chemical"},{"id":"T81","span":{"begin":2908,"end":2922},"obj":"Chemical"},{"id":"T82","span":{"begin":2908,"end":2917},"obj":"Chemical"},{"id":"T83","span":{"begin":2918,"end":2922},"obj":"Chemical"},{"id":"T84","span":{"begin":2976,"end":2980},"obj":"Chemical"},{"id":"T85","span":{"begin":3018,"end":3027},"obj":"Chemical"},{"id":"T86","span":{"begin":3067,"end":3074},"obj":"Chemical"},{"id":"T87","span":{"begin":3204,"end":3214},"obj":"Chemical"},{"id":"T88","span":{"begin":3343,"end":3348},"obj":"Chemical"},{"id":"T89","span":{"begin":3442,"end":3457},"obj":"Chemical"},{"id":"T90","span":{"begin":3442,"end":3451},"obj":"Chemical"},{"id":"T91","span":{"begin":3452,"end":3457},"obj":"Chemical"},{"id":"T92","span":{"begin":3573,"end":3582},"obj":"Chemical"},{"id":"T93","span":{"begin":3583,"end":3586},"obj":"Chemical"},{"id":"T94","span":{"begin":3619,"end":3632},"obj":"Chemical"},{"id":"T95","span":{"begin":5106,"end":5114},"obj":"Chemical"},{"id":"T96","span":{"begin":5316,"end":5325},"obj":"Chemical"},{"id":"T97","span":{"begin":5441,"end":5449},"obj":"Chemical"},{"id":"T98","span":{"begin":5484,"end":5492},"obj":"Chemical"},{"id":"T99","span":{"begin":5566,"end":5569},"obj":"Chemical"},{"id":"T100","span":{"begin":5585,"end":5593},"obj":"Chemical"},{"id":"T101","span":{"begin":5607,"end":5615},"obj":"Chemical"},{"id":"T102","span":{"begin":5645,"end":5653},"obj":"Chemical"},{"id":"T103","span":{"begin":5684,"end":5691},"obj":"Chemical"},{"id":"T104","span":{"begin":5781,"end":5788},"obj":"Chemical"},{"id":"T105","span":{"begin":5860,"end":5867},"obj":"Chemical"},{"id":"T106","span":{"begin":5956,"end":5960},"obj":"Chemical"},{"id":"T107","span":{"begin":5962,"end":5969},"obj":"Chemical"},{"id":"T108","span":{"begin":6221,"end":6226},"obj":"Chemical"},{"id":"T109","span":{"begin":6247,"end":6250},"obj":"Chemical"},{"id":"T110","span":{"begin":6334,"end":6342},"obj":"Chemical"},{"id":"T111","span":{"begin":6383,"end":6390},"obj":"Chemical"},{"id":"T112","span":{"begin":6476,"end":6483},"obj":"Chemical"},{"id":"T114","span":{"begin":6497,"end":6504},"obj":"Chemical"},{"id":"T115","span":{"begin":6606,"end":6613},"obj":"Chemical"},{"id":"T117","span":{"begin":6638,"end":6645},"obj":"Chemical"},{"id":"T118","span":{"begin":6690,"end":6695},"obj":"Chemical"},{"id":"T119","span":{"begin":6700,"end":6705},"obj":"Chemical"},{"id":"T120","span":{"begin":6712,"end":6715},"obj":"Chemical"},{"id":"T121","span":{"begin":6795,"end":6803},"obj":"Chemical"},{"id":"T122","span":{"begin":7092,"end":7099},"obj":"Chemical"},{"id":"T123","span":{"begin":7220,"end":7227},"obj":"Chemical"},{"id":"T124","span":{"begin":7287,"end":7296},"obj":"Chemical"},{"id":"T125","span":{"begin":7532,"end":7540},"obj":"Chemical"},{"id":"T126","span":{"begin":7559,"end":7566},"obj":"Chemical"},{"id":"T127","span":{"begin":7724,"end":7732},"obj":"Chemical"},{"id":"T128","span":{"begin":8187,"end":8192},"obj":"Chemical"},{"id":"T129","span":{"begin":8193,"end":8196},"obj":"Chemical"},{"id":"T130","span":{"begin":8566,"end":8568},"obj":"Chemical"},{"id":"T131","span":{"begin":8606,"end":8609},"obj":"Chemical"},{"id":"T132","span":{"begin":9168,"end":9175},"obj":"Chemical"},{"id":"T133","span":{"begin":9940,"end":9947},"obj":"Chemical"},{"id":"T134","span":{"begin":9996,"end":9998},"obj":"Chemical"},{"id":"T135","span":{"begin":10079,"end":10082},"obj":"Chemical"},{"id":"T136","span":{"begin":10447,"end":10449},"obj":"Chemical"},{"id":"T137","span":{"begin":10854,"end":10856},"obj":"Chemical"},{"id":"T138","span":{"begin":10926,"end":10933},"obj":"Chemical"},{"id":"T139","span":{"begin":10969,"end":10976},"obj":"Chemical"},{"id":"T140","span":{"begin":11422,"end":11424},"obj":"Chemical"},{"id":"T141","span":{"begin":11630,"end":11638},"obj":"Chemical"},{"id":"T142","span":{"begin":11639,"end":11643},"obj":"Chemical"},{"id":"T144","span":{"begin":11645,"end":11649},"obj":"Chemical"},{"id":"T145","span":{"begin":11895,"end":11897},"obj":"Chemical"},{"id":"T146","span":{"begin":12024,"end":12027},"obj":"Chemical"},{"id":"T147","span":{"begin":12190,"end":12201},"obj":"Chemical"},{"id":"T148","span":{"begin":12283,"end":12290},"obj":"Chemical"},{"id":"T149","span":{"begin":12353,"end":12362},"obj":"Chemical"},{"id":"T150","span":{"begin":12427,"end":12436},"obj":"Chemical"},{"id":"T151","span":{"begin":12475,"end":12484},"obj":"Chemical"},{"id":"T152","span":{"begin":12751,"end":12760},"obj":"Chemical"},{"id":"T153","span":{"begin":12794,"end":12801},"obj":"Chemical"},{"id":"T154","span":{"begin":12909,"end":12913},"obj":"Chemical"},{"id":"T155","span":{"begin":13048,"end":13056},"obj":"Chemical"},{"id":"T156","span":{"begin":13119,"end":13124},"obj":"Chemical"},{"id":"T157","span":{"begin":13137,"end":13145},"obj":"Chemical"},{"id":"T158","span":{"begin":13154,"end":13168},"obj":"Chemical"},{"id":"T159","span":{"begin":13154,"end":13165},"obj":"Chemical"},{"id":"T163","span":{"begin":13192,"end":13203},"obj":"Chemical"},{"id":"T164","span":{"begin":13351,"end":13360},"obj":"Chemical"},{"id":"T165","span":{"begin":13514,"end":13524},"obj":"Chemical"},{"id":"T166","span":{"begin":13564,"end":13566},"obj":"Chemical"},{"id":"T167","span":{"begin":13625,"end":13633},"obj":"Chemical"},{"id":"T168","span":{"begin":13654,"end":13669},"obj":"Chemical"},{"id":"T169","span":{"begin":13683,"end":13688},"obj":"Chemical"},{"id":"T170","span":{"begin":13690,"end":13698},"obj":"Chemical"},{"id":"T172","span":{"begin":13712,"end":13718},"obj":"Chemical"},{"id":"T173","span":{"begin":13791,"end":13799},"obj":"Chemical"},{"id":"T174","span":{"begin":13870,"end":13877},"obj":"Chemical"},{"id":"T177","span":{"begin":13870,"end":13875},"obj":"Chemical"},{"id":"T178","span":{"begin":13907,"end":13914},"obj":"Chemical"},{"id":"T179","span":{"begin":13920,"end":13927},"obj":"Chemical"},{"id":"T180","span":{"begin":14095,"end":14103},"obj":"Chemical"},{"id":"T181","span":{"begin":14104,"end":14113},"obj":"Chemical"},{"id":"T182","span":{"begin":14684,"end":14692},"obj":"Chemical"},{"id":"T183","span":{"begin":14712,"end":14728},"obj":"Chemical"},{"id":"T184","span":{"begin":14712,"end":14718},"obj":"Chemical"},{"id":"T186","span":{"begin":14736,"end":14747},"obj":"Chemical"},{"id":"T187","span":{"begin":14872,"end":14886},"obj":"Chemical"},{"id":"T188","span":{"begin":14872,"end":14880},"obj":"Chemical"},{"id":"T189","span":{"begin":14881,"end":14886},"obj":"Chemical"},{"id":"T191","span":{"begin":14907,"end":14919},"obj":"Chemical"},{"id":"T192","span":{"begin":14907,"end":14912},"obj":"Chemical"},{"id":"T193","span":{"begin":14962,"end":14972},"obj":"Chemical"},{"id":"T194","span":{"begin":15083,"end":15085},"obj":"Chemical"},{"id":"T198","span":{"begin":15207,"end":15216},"obj":"Chemical"},{"id":"T199","span":{"begin":15322,"end":15332},"obj":"Chemical"},{"id":"T200","span":{"begin":15721,"end":15729},"obj":"Chemical"},{"id":"T201","span":{"begin":16012,"end":16020},"obj":"Chemical"},{"id":"T202","span":{"begin":16083,"end":16091},"obj":"Chemical"},{"id":"T203","span":{"begin":16116,"end":16123},"obj":"Chemical"},{"id":"T204","span":{"begin":16177,"end":16189},"obj":"Chemical"},{"id":"T205","span":{"begin":16190,"end":16202},"obj":"Chemical"},{"id":"T206","span":{"begin":16231,"end":16238},"obj":"Chemical"},{"id":"T207","span":{"begin":16358,"end":16367},"obj":"Chemical"},{"id":"T208","span":{"begin":16526,"end":16534},"obj":"Chemical"},{"id":"T209","span":{"begin":16754,"end":16763},"obj":"Chemical"},{"id":"T210","span":{"begin":16822,"end":16831},"obj":"Chemical"},{"id":"T211","span":{"begin":17065,"end":17067},"obj":"Chemical"},{"id":"T213","span":{"begin":17098,"end":17102},"obj":"Chemical"},{"id":"T214","span":{"begin":17127,"end":17135},"obj":"Chemical"},{"id":"T215","span":{"begin":17304,"end":17308},"obj":"Chemical"},{"id":"T216","span":{"begin":17601,"end":17615},"obj":"Chemical"},{"id":"T217","span":{"begin":17601,"end":17610},"obj":"Chemical"},{"id":"T218","span":{"begin":17611,"end":17615},"obj":"Chemical"},{"id":"T219","span":{"begin":18666,"end":18668},"obj":"Chemical"},{"id":"T221","span":{"begin":18773,"end":18778},"obj":"Chemical"},{"id":"T222","span":{"begin":18806,"end":18808},"obj":"Chemical"},{"id":"T224","span":{"begin":18833,"end":18835},"obj":"Chemical"},{"id":"T226","span":{"begin":18875,"end":18877},"obj":"Chemical"},{"id":"T228","span":{"begin":19457,"end":19474},"obj":"Chemical"},{"id":"T229","span":{"begin":19538,"end":19542},"obj":"Chemical"},{"id":"T230","span":{"begin":19549,"end":19553},"obj":"Chemical"},{"id":"T231","span":{"begin":19750,"end":19767},"obj":"Chemical"},{"id":"T232","span":{"begin":19913,"end":19917},"obj":"Chemical"},{"id":"T233","span":{"begin":20005,"end":20029},"obj":"Chemical"},{"id":"T234","span":{"begin":20171,"end":20178},"obj":"Chemical"},{"id":"T235","span":{"begin":20215,"end":20217},"obj":"Chemical"},{"id":"T237","span":{"begin":21208,"end":21222},"obj":"Chemical"},{"id":"T238","span":{"begin":21242,"end":21255},"obj":"Chemical"},{"id":"T239","span":{"begin":22074,"end":22079},"obj":"Chemical"},{"id":"T240","span":{"begin":22600,"end":22606},"obj":"Chemical"},{"id":"T242","span":{"begin":22698,"end":22700},"obj":"Chemical"},{"id":"T244","span":{"begin":22880,"end":22886},"obj":"Chemical"},{"id":"T246","span":{"begin":22978,"end":22986},"obj":"Chemical"},{"id":"T247","span":{"begin":23339,"end":23343},"obj":"Chemical"},{"id":"T248","span":{"begin":23363,"end":23372},"obj":"Chemical"},{"id":"T249","span":{"begin":23407,"end":23410},"obj":"Chemical"},{"id":"T251","span":{"begin":23494,"end":23497},"obj":"Chemical"},{"id":"T253","span":{"begin":23693,"end":23716},"obj":"Chemical"},{"id":"T254","span":{"begin":23702,"end":23708},"obj":"Chemical"},{"id":"T255","span":{"begin":23718,"end":23721},"obj":"Chemical"},{"id":"T256","span":{"begin":23742,"end":23748},"obj":"Chemical"},{"id":"T257","span":{"begin":23925,"end":23928},"obj":"Chemical"},{"id":"T259","span":{"begin":23986,"end":23989},"obj":"Chemical"},{"id":"T260","span":{"begin":24017,"end":24025},"obj":"Chemical"},{"id":"T261","span":{"begin":24027,"end":24040},"obj":"Chemical"},{"id":"T262","span":{"begin":24035,"end":24040},"obj":"Chemical"},{"id":"T263","span":{"begin":24057,"end":24063},"obj":"Chemical"},{"id":"T264","span":{"begin":24111,"end":24114},"obj":"Chemical"},{"id":"T266","span":{"begin":24274,"end":24277},"obj":"Chemical"},{"id":"T268","span":{"begin":24623,"end":24626},"obj":"Chemical"},{"id":"T269","span":{"begin":24639,"end":24645},"obj":"Chemical"},{"id":"T270","span":{"begin":24646,"end":24653},"obj":"Chemical"},{"id":"T271","span":{"begin":24654,"end":24662},"obj":"Chemical"},{"id":"T272","span":{"begin":24684,"end":24687},"obj":"Chemical"},{"id":"T273","span":{"begin":24700,"end":24719},"obj":"Chemical"},{"id":"T274","span":{"begin":24700,"end":24704},"obj":"Chemical"},{"id":"T276","span":{"begin":24705,"end":24719},"obj":"Chemical"},{"id":"T277","span":{"begin":24815,"end":24818},"obj":"Chemical"},{"id":"T279","span":{"begin":24895,"end":24904},"obj":"Chemical"},{"id":"T280","span":{"begin":25091,"end":25107},"obj":"Chemical"},{"id":"T281","span":{"begin":25118,"end":25126},"obj":"Chemical"},{"id":"T282","span":{"begin":25249,"end":25251},"obj":"Chemical"},{"id":"T283","span":{"begin":25279,"end":25295},"obj":"Chemical"},{"id":"T284","span":{"begin":25297,"end":25299},"obj":"Chemical"},{"id":"T285","span":{"begin":25301,"end":25303},"obj":"Chemical"},{"id":"T286","span":{"begin":25308,"end":25310},"obj":"Chemical"},{"id":"T287","span":{"begin":25395,"end":25397},"obj":"Chemical"},{"id":"T288","span":{"begin":25790,"end":25792},"obj":"Chemical"},{"id":"T289","span":{"begin":25804,"end":25807},"obj":"Chemical"},{"id":"T291","span":{"begin":25860,"end":25862},"obj":"Chemical"},{"id":"T292","span":{"begin":25911,"end":25914},"obj":"Chemical"},{"id":"T294","span":{"begin":25962,"end":25970},"obj":"Chemical"},{"id":"T295","span":{"begin":26022,"end":26025},"obj":"Chemical"},{"id":"T297","span":{"begin":26276,"end":26284},"obj":"Chemical"},{"id":"T298","span":{"begin":26316,"end":26326},"obj":"Chemical"},{"id":"T300","span":{"begin":26338,"end":26352},"obj":"Chemical"},{"id":"T301","span":{"begin":26338,"end":26344},"obj":"Chemical"},{"id":"T303","span":{"begin":26345,"end":26352},"obj":"Chemical"},{"id":"T305","span":{"begin":26354,"end":26372},"obj":"Chemical"},{"id":"T306","span":{"begin":26354,"end":26362},"obj":"Chemical"},{"id":"T307","span":{"begin":26363,"end":26372},"obj":"Chemical"},{"id":"T308","span":{"begin":26378,"end":26398},"obj":"Chemical"},{"id":"T309","span":{"begin":26378,"end":26388},"obj":"Chemical"},{"id":"T310","span":{"begin":26389,"end":26398},"obj":"Chemical"},{"id":"T311","span":{"begin":26446,"end":26449},"obj":"Chemical"},{"id":"T313","span":{"begin":26540,"end":26543},"obj":"Chemical"},{"id":"T315","span":{"begin":27223,"end":27225},"obj":"Chemical"},{"id":"T318","span":{"begin":27396,"end":27403},"obj":"Chemical"},{"id":"T319","span":{"begin":27418,"end":27435},"obj":"Chemical"},{"id":"T321","span":{"begin":27747,"end":27750},"obj":"Chemical"},{"id":"T322","span":{"begin":27776,"end":27783},"obj":"Chemical"},{"id":"T323","span":{"begin":27908,"end":27916},"obj":"Chemical"},{"id":"T324","span":{"begin":28027,"end":28030},"obj":"Chemical"},{"id":"T325","span":{"begin":28151,"end":28154},"obj":"Chemical"},{"id":"T326","span":{"begin":28572,"end":28578},"obj":"Chemical"},{"id":"T328","span":{"begin":28736,"end":28740},"obj":"Chemical"},{"id":"T329","span":{"begin":29116,"end":29119},"obj":"Chemical"},{"id":"T330","span":{"begin":29124,"end":29128},"obj":"Chemical"},{"id":"T331","span":{"begin":29182,"end":29190},"obj":"Chemical"},{"id":"T332","span":{"begin":31106,"end":31110},"obj":"Chemical"},{"id":"T333","span":{"begin":31294,"end":31298},"obj":"Chemical"},{"id":"T334","span":{"begin":31506,"end":31514},"obj":"Chemical"},{"id":"T335","span":{"begin":31827,"end":31830},"obj":"Chemical"},{"id":"T336","span":{"begin":31899,"end":31902},"obj":"Chemical"},{"id":"T337","span":{"begin":32387,"end":32393},"obj":"Chemical"},{"id":"T338","span":{"begin":32550,"end":32558},"obj":"Chemical"},{"id":"T339","span":{"begin":32628,"end":32638},"obj":"Chemical"},{"id":"T340","span":{"begin":32628,"end":32633},"obj":"Chemical"},{"id":"T341","span":{"begin":32634,"end":32638},"obj":"Chemical"},{"id":"T342","span":{"begin":32671,"end":32689},"obj":"Chemical"},{"id":"T343","span":{"begin":32691,"end":32695},"obj":"Chemical"},{"id":"T344","span":{"begin":32700,"end":32715},"obj":"Chemical"},{"id":"T345","span":{"begin":32700,"end":32707},"obj":"Chemical"},{"id":"T346","span":{"begin":32708,"end":32715},"obj":"Chemical"},{"id":"T347","span":{"begin":32717,"end":32719},"obj":"Chemical"},{"id":"T349","span":{"begin":32865,"end":32873},"obj":"Chemical"},{"id":"T350","span":{"begin":32874,"end":32884},"obj":"Chemical"},{"id":"T351","span":{"begin":32964,"end":32967},"obj":"Chemical"},{"id":"T352","span":{"begin":33040,"end":33044},"obj":"Chemical"},{"id":"T353","span":{"begin":33045,"end":33048},"obj":"Chemical"},{"id":"T354","span":{"begin":33099,"end":33109},"obj":"Chemical"},{"id":"T355","span":{"begin":33170,"end":33185},"obj":"Chemical"},{"id":"T356","span":{"begin":33170,"end":33177},"obj":"Chemical"},{"id":"T357","span":{"begin":33178,"end":33185},"obj":"Chemical"},{"id":"T358","span":{"begin":33270,"end":33272},"obj":"Chemical"},{"id":"T359","span":{"begin":33273,"end":33276},"obj":"Chemical"},{"id":"T360","span":{"begin":33539,"end":33542},"obj":"Chemical"},{"id":"T361","span":{"begin":33591,"end":33594},"obj":"Chemical"},{"id":"T362","span":{"begin":33656,"end":33660},"obj":"Chemical"},{"id":"T364","span":{"begin":33661,"end":33664},"obj":"Chemical"},{"id":"T365","span":{"begin":33680,"end":33682},"obj":"Chemical"},{"id":"T370","span":{"begin":33805,"end":33812},"obj":"Chemical"},{"id":"T371","span":{"begin":33830,"end":33832},"obj":"Chemical"},{"id":"T376","span":{"begin":33938,"end":33947},"obj":"Chemical"},{"id":"T377","span":{"begin":33948,"end":33951},"obj":"Chemical"},{"id":"T378","span":{"begin":33953,"end":33955},"obj":"Chemical"},{"id":"T379","span":{"begin":33960,"end":33970},"obj":"Chemical"},{"id":"T381","span":{"begin":33960,"end":33964},"obj":"Chemical"},{"id":"T382","span":{"begin":33965,"end":33970},"obj":"Chemical"},{"id":"T384","span":{"begin":34018,"end":34020},"obj":"Chemical"},{"id":"T386","span":{"begin":34262,"end":34265},"obj":"Chemical"},{"id":"T387","span":{"begin":34292,"end":34302},"obj":"Chemical"},{"id":"T388","span":{"begin":34357,"end":34364},"obj":"Chemical"},{"id":"T389","span":{"begin":34650,"end":34657},"obj":"Chemical"},{"id":"T390","span":{"begin":34787,"end":34794},"obj":"Chemical"},{"id":"T391","span":{"begin":35009,"end":35013},"obj":"Chemical"},{"id":"T392","span":{"begin":35014,"end":35023},"obj":"Chemical"},{"id":"T393","span":{"begin":35086,"end":35091},"obj":"Chemical"},{"id":"T394","span":{"begin":35401,"end":35405},"obj":"Chemical"},{"id":"T395","span":{"begin":35555,"end":35560},"obj":"Chemical"},{"id":"T396","span":{"begin":35867,"end":35871},"obj":"Chemical"},{"id":"T397","span":{"begin":36356,"end":36360},"obj":"Chemical"},{"id":"T398","span":{"begin":36514,"end":36519},"obj":"Chemical"},{"id":"T399","span":{"begin":36561,"end":36569},"obj":"Chemical"},{"id":"T400","span":{"begin":36676,"end":36680},"obj":"Chemical"},{"id":"T401","span":{"begin":36681,"end":36690},"obj":"Chemical"},{"id":"T402","span":{"begin":36713,"end":36715},"obj":"Chemical"},{"id":"T403","span":{"begin":37004,"end":37007},"obj":"Chemical"},{"id":"T404","span":{"begin":37235,"end":37237},"obj":"Chemical"},{"id":"T405","span":{"begin":37301,"end":37303},"obj":"Chemical"},{"id":"T406","span":{"begin":37333,"end":37342},"obj":"Chemical"},{"id":"T407","span":{"begin":37344,"end":37352},"obj":"Chemical"},{"id":"T408","span":{"begin":37475,"end":37478},"obj":"Chemical"},{"id":"T409","span":{"begin":37514,"end":37519},"obj":"Chemical"},{"id":"T410","span":{"begin":37588,"end":37592},"obj":"Chemical"},{"id":"T411","span":{"begin":37956,"end":37958},"obj":"Chemical"}],"attributes":[{"id":"A50","pred":"chebi_id","subj":"T50","obj":"http://purl.obolibrary.org/obo/CHEBI_23888"},{"id":"A51","pred":"chebi_id","subj":"T51","obj":"http://purl.obolibrary.org/obo/CHEBI_74067"},{"id":"A52","pred":"chebi_id","subj":"T52","obj":"http://purl.obolibrary.org/obo/CHEBI_23888"},{"id":"A53","pred":"chebi_id","subj":"T53","obj":"http://purl.obolibrary.org/obo/CHEBI_36080"},{"id":"A54","pred":"chebi_id","subj":"T54","obj":"http://purl.obolibrary.org/obo/CHEBI_36044"},{"id":"A55","pred":"chebi_id","subj":"T55","obj":"http://purl.obolibrary.org/obo/CHEBI_22587"},{"id":"A56","pred":"chebi_id","subj":"T56","obj":"http://purl.obolibrary.org/obo/CHEBI_23888"},{"id":"A57","pred":"chebi_id","subj":"T57","obj":"http://purl.obolibrary.org/obo/CHEBI_22587"},{"id":"A58","pred":"chebi_id","subj":"T58","obj":"http://purl.obolibrary.org/obo/CHEBI_23888"},{"id":"A59","pred":"chebi_id","subj":"T59","obj":"http://purl.obolibrary.org/obo/CHEBI_35222"},{"id":"A60","pred":"chebi_id","subj":"T60","obj":"http://purl.obolibrary.org/obo/CHEBI_52214"},{"id":"A61","pred":"chebi_id","subj":"T61","obj":"http://purl.obolibrary.org/obo/CHEBI_28815"},{"id":"A62","pred":"chebi_id","subj":"T62","obj":"http://purl.obolibrary.org/obo/CHEBI_24500"},{"id":"A63","pred":"chebi_id","subj":"T63","obj":"http://purl.obolibrary.org/obo/CHEBI_16189"},{"id":"A64","pred":"chebi_id","subj":"T64","obj":"http://purl.obolibrary.org/obo/CHEBI_37396"},{"id":"A65","pred":"chebi_id","subj":"T65","obj":"http://purl.obolibrary.org/obo/CHEBI_26667"},{"id":"A66","pred":"chebi_id","subj":"T66","obj":"http://purl.obolibrary.org/obo/CHEBI_37527"},{"id":"A67","pred":"chebi_id","subj":"T67","obj":"http://purl.obolibrary.org/obo/CHEBI_35962"},{"id":"A68","pred":"chebi_id","subj":"T67","obj":"http://purl.obolibrary.org/obo/CHEBI_38358"},{"id":"A69","pred":"chebi_id","subj":"T67","obj":"http://purl.obolibrary.org/obo/CHEBI_45373"},{"id":"A70","pred":"chebi_id","subj":"T67","obj":"http://purl.obolibrary.org/obo/CHEBI_74801"},{"id":"A71","pred":"chebi_id","subj":"T67","obj":"http://purl.obolibrary.org/obo/CHEBI_26667"},{"id":"A72","pred":"chebi_id","subj":"T72","obj":"http://purl.obolibrary.org/obo/CHEBI_35962"},{"id":"A73","pred":"chebi_id","subj":"T72","obj":"http://purl.obolibrary.org/obo/CHEBI_38358"},{"id":"A74","pred":"chebi_id","subj":"T72","obj":"http://purl.obolibrary.org/obo/CHEBI_45373"},{"id":"A75","pred":"chebi_id","subj":"T72","obj":"http://purl.obolibrary.org/obo/CHEBI_74801"},{"id":"A76","pred":"chebi_id","subj":"T72","obj":"http://purl.obolibrary.org/obo/CHEBI_26667"},{"id":"A77","pred":"chebi_id","subj":"T77","obj":"http://purl.obolibrary.org/obo/CHEBI_132554"},{"id":"A78","pred":"chebi_id","subj":"T78","obj":"http://purl.obolibrary.org/obo/CHEBI_50803"},{"id":"A79","pred":"chebi_id","subj":"T79","obj":"http://purl.obolibrary.org/obo/CHEBI_25367"},{"id":"A80","pred":"chebi_id","subj":"T80","obj":"http://purl.obolibrary.org/obo/CHEBI_50803"},{"id":"A81","pred":"chebi_id","subj":"T81","obj":"http://purl.obolibrary.org/obo/CHEBI_36044"},{"id":"A82","pred":"chebi_id","subj":"T82","obj":"http://purl.obolibrary.org/obo/CHEBI_22587"},{"id":"A83","pred":"chebi_id","subj":"T83","obj":"http://purl.obolibrary.org/obo/CHEBI_23888"},{"id":"A84","pred":"chebi_id","subj":"T84","obj":"http://purl.obolibrary.org/obo/CHEBI_23888"},{"id":"A85","pred":"chebi_id","subj":"T85","obj":"http://purl.obolibrary.org/obo/CHEBI_35222"},{"id":"A86","pred":"chebi_id","subj":"T86","obj":"http://purl.obolibrary.org/obo/CHEBI_36080"},{"id":"A87","pred":"chebi_id","subj":"T87","obj":"http://purl.obolibrary.org/obo/CHEBI_22587"},{"id":"A88","pred":"chebi_id","subj":"T88","obj":"http://purl.obolibrary.org/obo/CHEBI_23888"},{"id":"A89","pred":"chebi_id","subj":"T89","obj":"http://purl.obolibrary.org/obo/CHEBI_36044"},{"id":"A90","pred":"chebi_id","subj":"T90","obj":"http://purl.obolibrary.org/obo/CHEBI_22587"},{"id":"A91","pred":"chebi_id","subj":"T91","obj":"http://purl.obolibrary.org/obo/CHEBI_23888"},{"id":"A92","pred":"chebi_id","subj":"T92","obj":"http://purl.obolibrary.org/obo/CHEBI_22587"},{"id":"A93","pred":"chebi_id","subj":"T93","obj":"http://purl.obolibrary.org/obo/CHEBI_50803"},{"id":"A94","pred":"chebi_id","subj":"T94","obj":"http://purl.obolibrary.org/obo/CHEBI_23456"},{"id":"A95","pred":"chebi_id","subj":"T95","obj":"http://purl.obolibrary.org/obo/CHEBI_59132"},{"id":"A96","pred":"chebi_id","subj":"T96","obj":"http://purl.obolibrary.org/obo/CHEBI_60809"},{"id":"A97","pred":"chebi_id","subj":"T97","obj":"http://purl.obolibrary.org/obo/CHEBI_59132"},{"id":"A98","pred":"chebi_id","subj":"T98","obj":"http://purl.obolibrary.org/obo/CHEBI_36080"},{"id":"A99","pred":"chebi_id","subj":"T99","obj":"http://purl.obolibrary.org/obo/CHEBI_16991"},{"id":"A100","pred":"chebi_id","subj":"T100","obj":"http://purl.obolibrary.org/obo/CHEBI_59132"},{"id":"A101","pred":"chebi_id","subj":"T101","obj":"http://purl.obolibrary.org/obo/CHEBI_59132"},{"id":"A102","pred":"chebi_id","subj":"T102","obj":"http://purl.obolibrary.org/obo/CHEBI_36080"},{"id":"A103","pred":"chebi_id","subj":"T103","obj":"http://purl.obolibrary.org/obo/CHEBI_36080"},{"id":"A104","pred":"chebi_id","subj":"T104","obj":"http://purl.obolibrary.org/obo/CHEBI_36080"},{"id":"A105","pred":"chebi_id","subj":"T105","obj":"http://purl.obolibrary.org/obo/CHEBI_36080"},{"id":"A106","pred":"chebi_id","subj":"T106","obj":"http://purl.obolibrary.org/obo/CHEBI_23888"},{"id":"A107","pred":"chebi_id","subj":"T107","obj":"http://purl.obolibrary.org/obo/CHEBI_36080"},{"id":"A108","pred":"chebi_id","subj":"T108","obj":"http://purl.obolibrary.org/obo/CHEBI_18059"},{"id":"A109","pred":"chebi_id","subj":"T109","obj":"http://purl.obolibrary.org/obo/CHEBI_50803"},{"id":"A110","pred":"chebi_id","subj":"T110","obj":"http://purl.obolibrary.org/obo/CHEBI_36080"},{"id":"A111","pred":"chebi_id","subj":"T111","obj":"http://purl.obolibrary.org/obo/CHEBI_36080"},{"id":"A112","pred":"chebi_id","subj":"T112","obj":"http://purl.obolibrary.org/obo/CHEBI_33839"},{"id":"A113","pred":"chebi_id","subj":"T112","obj":"http://purl.obolibrary.org/obo/CHEBI_60027"},{"id":"A114","pred":"chebi_id","subj":"T114","obj":"http://purl.obolibrary.org/obo/CHEBI_59132"},{"id":"A115","pred":"chebi_id","subj":"T115","obj":"http://purl.obolibrary.org/obo/CHEBI_33839"},{"id":"A116","pred":"chebi_id","subj":"T115","obj":"http://purl.obolibrary.org/obo/CHEBI_60027"},{"id":"A117","pred":"chebi_id","subj":"T117","obj":"http://purl.obolibrary.org/obo/CHEBI_59132"},{"id":"A118","pred":"chebi_id","subj":"T118","obj":"http://purl.obolibrary.org/obo/CHEBI_18059"},{"id":"A119","pred":"chebi_id","subj":"T119","obj":"http://purl.obolibrary.org/obo/CHEBI_18059"},{"id":"A120","pred":"chebi_id","subj":"T120","obj":"http://purl.obolibrary.org/obo/CHEBI_50803"},{"id":"A121","pred":"chebi_id","subj":"T121","obj":"http://purl.obolibrary.org/obo/CHEBI_36080"},{"id":"A122","pred":"chebi_id","subj":"T122","obj":"http://purl.obolibrary.org/obo/CHEBI_59132"},{"id":"A123","pred":"chebi_id","subj":"T123","obj":"http://purl.obolibrary.org/obo/CHEBI_59132"},{"id":"A124","pred":"chebi_id","subj":"T124","obj":"http://purl.obolibrary.org/obo/CHEBI_25367"},{"id":"A125","pred":"chebi_id","subj":"T125","obj":"http://purl.obolibrary.org/obo/CHEBI_36080"},{"id":"A126","pred":"chebi_id","subj":"T126","obj":"http://purl.obolibrary.org/obo/CHEBI_36080"},{"id":"A127","pred":"chebi_id","subj":"T127","obj":"http://purl.obolibrary.org/obo/CHEBI_59132"},{"id":"A128","pred":"chebi_id","subj":"T128","obj":"http://purl.obolibrary.org/obo/CHEBI_18059"},{"id":"A129","pred":"chebi_id","subj":"T129","obj":"http://purl.obolibrary.org/obo/CHEBI_50803"},{"id":"A130","pred":"chebi_id","subj":"T130","obj":"http://purl.obolibrary.org/obo/CHEBI_74067"},{"id":"A131","pred":"chebi_id","subj":"T131","obj":"http://purl.obolibrary.org/obo/CHEBI_16991"},{"id":"A132","pred":"chebi_id","subj":"T132","obj":"http://purl.obolibrary.org/obo/CHEBI_36080"},{"id":"A133","pred":"chebi_id","subj":"T133","obj":"http://purl.obolibrary.org/obo/CHEBI_36080"},{"id":"A134","pred":"chebi_id","subj":"T134","obj":"http://purl.obolibrary.org/obo/CHEBI_74067"},{"id":"A135","pred":"chebi_id","subj":"T135","obj":"http://purl.obolibrary.org/obo/CHEBI_16991"},{"id":"A136","pred":"chebi_id","subj":"T136","obj":"http://purl.obolibrary.org/obo/CHEBI_74067"},{"id":"A137","pred":"chebi_id","subj":"T137","obj":"http://purl.obolibrary.org/obo/CHEBI_74067"},{"id":"A138","pred":"chebi_id","subj":"T138","obj":"http://purl.obolibrary.org/obo/CHEBI_36080"},{"id":"A139","pred":"chebi_id","subj":"T139","obj":"http://purl.obolibrary.org/obo/CHEBI_36080"},{"id":"A140","pred":"chebi_id","subj":"T140","obj":"http://purl.obolibrary.org/obo/CHEBI_74067"},{"id":"A141","pred":"chebi_id","subj":"T141","obj":"http://purl.obolibrary.org/obo/CHEBI_28984"},{"id":"A142","pred":"chebi_id","subj":"T142","obj":"http://purl.obolibrary.org/obo/CHEBI_24866"},{"id":"A143","pred":"chebi_id","subj":"T142","obj":"http://purl.obolibrary.org/obo/CHEBI_26710"},{"id":"A144","pred":"chebi_id","subj":"T144","obj":"http://purl.obolibrary.org/obo/CHEBI_74768"},{"id":"A145","pred":"chebi_id","subj":"T145","obj":"http://purl.obolibrary.org/obo/CHEBI_74067"},{"id":"A146","pred":"chebi_id","subj":"T146","obj":"http://purl.obolibrary.org/obo/CHEBI_16991"},{"id":"A147","pred":"chebi_id","subj":"T147","obj":"http://purl.obolibrary.org/obo/CHEBI_33232"},{"id":"A148","pred":"chebi_id","subj":"T148","obj":"http://purl.obolibrary.org/obo/CHEBI_36080"},{"id":"A149","pred":"chebi_id","subj":"T149","obj":"http://purl.obolibrary.org/obo/CHEBI_60809"},{"id":"A150","pred":"chebi_id","subj":"T150","obj":"http://purl.obolibrary.org/obo/CHEBI_60809"},{"id":"A151","pred":"chebi_id","subj":"T151","obj":"http://purl.obolibrary.org/obo/CHEBI_60809"},{"id":"A152","pred":"chebi_id","subj":"T152","obj":"http://purl.obolibrary.org/obo/CHEBI_60809"},{"id":"A153","pred":"chebi_id","subj":"T153","obj":"http://purl.obolibrary.org/obo/CHEBI_36080"},{"id":"A154","pred":"chebi_id","subj":"T154","obj":"http://purl.obolibrary.org/obo/CHEBI_74768"},{"id":"A155","pred":"chebi_id","subj":"T155","obj":"http://purl.obolibrary.org/obo/CHEBI_60809"},{"id":"A156","pred":"chebi_id","subj":"T156","obj":"http://purl.obolibrary.org/obo/CHEBI_15377"},{"id":"A157","pred":"chebi_id","subj":"T157","obj":"http://purl.obolibrary.org/obo/CHEBI_15440"},{"id":"A158","pred":"chebi_id","subj":"T158","obj":"http://purl.obolibrary.org/obo/CHEBI_53426"},{"id":"A159","pred":"chebi_id","subj":"T159","obj":"http://purl.obolibrary.org/obo/CHEBI_53422"},{"id":"A160","pred":"chebi_id","subj":"T159","obj":"http://purl.obolibrary.org/obo/CHEBI_53423"},{"id":"A161","pred":"chebi_id","subj":"T159","obj":"http://purl.obolibrary.org/obo/CHEBI_53424"},{"id":"A162","pred":"chebi_id","subj":"T159","obj":"http://purl.obolibrary.org/obo/CHEBI_53425"},{"id":"A163","pred":"chebi_id","subj":"T163","obj":"http://purl.obolibrary.org/obo/CHEBI_35195"},{"id":"A164","pred":"chebi_id","subj":"T164","obj":"http://purl.obolibrary.org/obo/CHEBI_60809"},{"id":"A165","pred":"chebi_id","subj":"T165","obj":"http://purl.obolibrary.org/obo/CHEBI_27013"},{"id":"A166","pred":"chebi_id","subj":"T166","obj":"http://purl.obolibrary.org/obo/CHEBI_73807"},{"id":"A167","pred":"chebi_id","subj":"T167","obj":"http://purl.obolibrary.org/obo/CHEBI_15440"},{"id":"A168","pred":"chebi_id","subj":"T168","obj":"http://purl.obolibrary.org/obo/CHEBI_46793"},{"id":"A169","pred":"chebi_id","subj":"T169","obj":"http://purl.obolibrary.org/obo/CHEBI_25698"},{"id":"A170","pred":"chebi_id","subj":"T170","obj":"http://purl.obolibrary.org/obo/CHEBI_16899"},{"id":"A171","pred":"chebi_id","subj":"T170","obj":"http://purl.obolibrary.org/obo/CHEBI_29864"},{"id":"A172","pred":"chebi_id","subj":"T172","obj":"http://purl.obolibrary.org/obo/CHEBI_30823"},{"id":"A173","pred":"chebi_id","subj":"T173","obj":"http://purl.obolibrary.org/obo/CHEBI_60809"},{"id":"A174","pred":"chebi_id","subj":"T174","obj":"http://purl.obolibrary.org/obo/CHEBI_25051"},{"id":"A175","pred":"chebi_id","subj":"T174","obj":"http://purl.obolibrary.org/obo/CHEBI_47040"},{"id":"A176","pred":"chebi_id","subj":"T174","obj":"http://purl.obolibrary.org/obo/CHEBI_58712"},{"id":"A177","pred":"chebi_id","subj":"T177","obj":"http://purl.obolibrary.org/obo/CHEBI_18059"},{"id":"A178","pred":"chebi_id","subj":"T178","obj":"http://purl.obolibrary.org/obo/CHEBI_48705"},{"id":"A179","pred":"chebi_id","subj":"T179","obj":"http://purl.obolibrary.org/obo/CHEBI_26605"},{"id":"A180","pred":"chebi_id","subj":"T180","obj":"http://purl.obolibrary.org/obo/CHEBI_28984"},{"id":"A181","pred":"chebi_id","subj":"T181","obj":"http://purl.obolibrary.org/obo/CHEBI_16234"},{"id":"A182","pred":"chebi_id","subj":"T182","obj":"http://purl.obolibrary.org/obo/CHEBI_36973"},{"id":"A183","pred":"chebi_id","subj":"T183","obj":"http://purl.obolibrary.org/obo/CHEBI_50594"},{"id":"A184","pred":"chebi_id","subj":"T184","obj":"http://purl.obolibrary.org/obo/CHEBI_27594"},{"id":"A185","pred":"chebi_id","subj":"T184","obj":"http://purl.obolibrary.org/obo/CHEBI_33415"},{"id":"A186","pred":"chebi_id","subj":"T186","obj":"http://purl.obolibrary.org/obo/CHEBI_53276"},{"id":"A187","pred":"chebi_id","subj":"T187","obj":"http://purl.obolibrary.org/obo/CHEBI_132889"},{"id":"A188","pred":"chebi_id","subj":"T188","obj":"http://purl.obolibrary.org/obo/CHEBI_36973"},{"id":"A189","pred":"chebi_id","subj":"T189","obj":"http://purl.obolibrary.org/obo/CHEBI_25741"},{"id":"A190","pred":"chebi_id","subj":"T189","obj":"http://purl.obolibrary.org/obo/CHEBI_29356"},{"id":"A191","pred":"chebi_id","subj":"T191","obj":"http://purl.obolibrary.org/obo/CHEBI_51142"},{"id":"A192","pred":"chebi_id","subj":"T192","obj":"http://purl.obolibrary.org/obo/CHEBI_46882"},{"id":"A193","pred":"chebi_id","subj":"T193","obj":"http://purl.obolibrary.org/obo/CHEBI_52999"},{"id":"A194","pred":"chebi_id","subj":"T194","obj":"http://purl.obolibrary.org/obo/CHEBI_51079"},{"id":"A195","pred":"chebi_id","subj":"T194","obj":"http://purl.obolibrary.org/obo/CHEBI_139019"},{"id":"A196","pred":"chebi_id","subj":"T194","obj":"http://purl.obolibrary.org/obo/CHEBI_140152"},{"id":"A197","pred":"chebi_id","subj":"T194","obj":"http://purl.obolibrary.org/obo/CHEBI_34826"},{"id":"A198","pred":"chebi_id","subj":"T198","obj":"http://purl.obolibrary.org/obo/CHEBI_60809"},{"id":"A199","pred":"chebi_id","subj":"T199","obj":"http://purl.obolibrary.org/obo/CHEBI_52999"},{"id":"A200","pred":"chebi_id","subj":"T200","obj":"http://purl.obolibrary.org/obo/CHEBI_60809"},{"id":"A201","pred":"chebi_id","subj":"T201","obj":"http://purl.obolibrary.org/obo/CHEBI_60809"},{"id":"A202","pred":"chebi_id","subj":"T202","obj":"http://purl.obolibrary.org/obo/CHEBI_60809"},{"id":"A203","pred":"chebi_id","subj":"T203","obj":"http://purl.obolibrary.org/obo/CHEBI_36080"},{"id":"A204","pred":"chebi_id","subj":"T204","obj":"http://purl.obolibrary.org/obo/CHEBI_17089"},{"id":"A205","pred":"chebi_id","subj":"T205","obj":"http://purl.obolibrary.org/obo/CHEBI_50803"},{"id":"A206","pred":"chebi_id","subj":"T206","obj":"http://purl.obolibrary.org/obo/CHEBI_26605"},{"id":"A207","pred":"chebi_id","subj":"T207","obj":"http://purl.obolibrary.org/obo/CHEBI_60809"},{"id":"A208","pred":"chebi_id","subj":"T208","obj":"http://purl.obolibrary.org/obo/CHEBI_60809"},{"id":"A209","pred":"chebi_id","subj":"T209","obj":"http://purl.obolibrary.org/obo/CHEBI_60809"},{"id":"A210","pred":"chebi_id","subj":"T210","obj":"http://purl.obolibrary.org/obo/CHEBI_60809"},{"id":"A211","pred":"chebi_id","subj":"T211","obj":"http://purl.obolibrary.org/obo/CHEBI_63895"},{"id":"A212","pred":"chebi_id","subj":"T211","obj":"http://purl.obolibrary.org/obo/CHEBI_74072"},{"id":"A213","pred":"chebi_id","subj":"T213","obj":"http://purl.obolibrary.org/obo/CHEBI_74768"},{"id":"A214","pred":"chebi_id","subj":"T214","obj":"http://purl.obolibrary.org/obo/CHEBI_60809"},{"id":"A215","pred":"chebi_id","subj":"T215","obj":"http://purl.obolibrary.org/obo/CHEBI_74768"},{"id":"A216","pred":"chebi_id","subj":"T216","obj":"http://purl.obolibrary.org/obo/CHEBI_36044"},{"id":"A217","pred":"chebi_id","subj":"T217","obj":"http://purl.obolibrary.org/obo/CHEBI_22587"},{"id":"A218","pred":"chebi_id","subj":"T218","obj":"http://purl.obolibrary.org/obo/CHEBI_23888"},{"id":"A219","pred":"chebi_id","subj":"T219","obj":"http://purl.obolibrary.org/obo/CHEBI_63895"},{"id":"A220","pred":"chebi_id","subj":"T219","obj":"http://purl.obolibrary.org/obo/CHEBI_74072"},{"id":"A221","pred":"chebi_id","subj":"T221","obj":"http://purl.obolibrary.org/obo/CHEBI_23888"},{"id":"A222","pred":"chebi_id","subj":"T222","obj":"http://purl.obolibrary.org/obo/CHEBI_63895"},{"id":"A223","pred":"chebi_id","subj":"T222","obj":"http://purl.obolibrary.org/obo/CHEBI_74072"},{"id":"A224","pred":"chebi_id","subj":"T224","obj":"http://purl.obolibrary.org/obo/CHEBI_63895"},{"id":"A225","pred":"chebi_id","subj":"T224","obj":"http://purl.obolibrary.org/obo/CHEBI_74072"},{"id":"A226","pred":"chebi_id","subj":"T226","obj":"http://purl.obolibrary.org/obo/CHEBI_63895"},{"id":"A227","pred":"chebi_id","subj":"T226","obj":"http://purl.obolibrary.org/obo/CHEBI_74072"},{"id":"A228","pred":"chebi_id","subj":"T228","obj":"http://purl.obolibrary.org/obo/CHEBI_35705"},{"id":"A229","pred":"chebi_id","subj":"T229","obj":"http://purl.obolibrary.org/obo/CHEBI_23888"},{"id":"A230","pred":"chebi_id","subj":"T230","obj":"http://purl.obolibrary.org/obo/CHEBI_23888"},{"id":"A231","pred":"chebi_id","subj":"T231","obj":"http://purl.obolibrary.org/obo/CHEBI_35705"},{"id":"A232","pred":"chebi_id","subj":"T232","obj":"http://purl.obolibrary.org/obo/CHEBI_23888"},{"id":"A233","pred":"chebi_id","subj":"T233","obj":"http://purl.obolibrary.org/obo/CHEBI_35705"},{"id":"A234","pred":"chebi_id","subj":"T234","obj":"http://purl.obolibrary.org/obo/CHEBI_59132"},{"id":"A235","pred":"chebi_id","subj":"T235","obj":"http://purl.obolibrary.org/obo/CHEBI_63895"},{"id":"A236","pred":"chebi_id","subj":"T235","obj":"http://purl.obolibrary.org/obo/CHEBI_74072"},{"id":"A237","pred":"chebi_id","subj":"T237","obj":"http://purl.obolibrary.org/obo/CHEBI_63866"},{"id":"A238","pred":"chebi_id","subj":"T238","obj":"http://purl.obolibrary.org/obo/CHEBI_41879"},{"id":"A239","pred":"chebi_id","subj":"T239","obj":"http://purl.obolibrary.org/obo/CHEBI_23888"},{"id":"A240","pred":"chebi_id","subj":"T240","obj":"http://purl.obolibrary.org/obo/CHEBI_27594"},{"id":"A241","pred":"chebi_id","subj":"T240","obj":"http://purl.obolibrary.org/obo/CHEBI_33415"},{"id":"A242","pred":"chebi_id","subj":"T242","obj":"http://purl.obolibrary.org/obo/CHEBI_63895"},{"id":"A243","pred":"chebi_id","subj":"T242","obj":"http://purl.obolibrary.org/obo/CHEBI_74072"},{"id":"A244","pred":"chebi_id","subj":"T244","obj":"http://purl.obolibrary.org/obo/CHEBI_27594"},{"id":"A245","pred":"chebi_id","subj":"T244","obj":"http://purl.obolibrary.org/obo/CHEBI_33415"},{"id":"A246","pred":"chebi_id","subj":"T246","obj":"http://purl.obolibrary.org/obo/CHEBI_36973"},{"id":"A247","pred":"chebi_id","subj":"T247","obj":"http://purl.obolibrary.org/obo/CHEBI_23888"},{"id":"A248","pred":"chebi_id","subj":"T248","obj":"http://purl.obolibrary.org/obo/CHEBI_22587"},{"id":"A249","pred":"chebi_id","subj":"T249","obj":"http://purl.obolibrary.org/obo/CHEBI_53228"},{"id":"A250","pred":"chebi_id","subj":"T249","obj":"http://purl.obolibrary.org/obo/CHEBI_60622"},{"id":"A251","pred":"chebi_id","subj":"T251","obj":"http://purl.obolibrary.org/obo/CHEBI_53228"},{"id":"A252","pred":"chebi_id","subj":"T251","obj":"http://purl.obolibrary.org/obo/CHEBI_60622"},{"id":"A253","pred":"chebi_id","subj":"T253","obj":"http://purl.obolibrary.org/obo/CHEBI_26523"},{"id":"A254","pred":"chebi_id","subj":"T254","obj":"http://purl.obolibrary.org/obo/CHEBI_25805"},{"id":"A255","pred":"chebi_id","subj":"T255","obj":"http://purl.obolibrary.org/obo/CHEBI_26523"},{"id":"A256","pred":"chebi_id","subj":"T256","obj":"http://purl.obolibrary.org/obo/CHEBI_25805"},{"id":"A257","pred":"chebi_id","subj":"T257","obj":"http://purl.obolibrary.org/obo/CHEBI_53228"},{"id":"A258","pred":"chebi_id","subj":"T257","obj":"http://purl.obolibrary.org/obo/CHEBI_60622"},{"id":"A259","pred":"chebi_id","subj":"T259","obj":"http://purl.obolibrary.org/obo/CHEBI_26523"},{"id":"A260","pred":"chebi_id","subj":"T260","obj":"http://purl.obolibrary.org/obo/CHEBI_36080"},{"id":"A261","pred":"chebi_id","subj":"T261","obj":"http://purl.obolibrary.org/obo/CHEBI_33696"},{"id":"A262","pred":"chebi_id","subj":"T262","obj":"http://purl.obolibrary.org/obo/CHEBI_37527"},{"id":"A263","pred":"chebi_id","subj":"T263","obj":"http://purl.obolibrary.org/obo/CHEBI_18059"},{"id":"A264","pred":"chebi_id","subj":"T264","obj":"http://purl.obolibrary.org/obo/CHEBI_53228"},{"id":"A265","pred":"chebi_id","subj":"T264","obj":"http://purl.obolibrary.org/obo/CHEBI_60622"},{"id":"A266","pred":"chebi_id","subj":"T266","obj":"http://purl.obolibrary.org/obo/CHEBI_53228"},{"id":"A267","pred":"chebi_id","subj":"T266","obj":"http://purl.obolibrary.org/obo/CHEBI_60622"},{"id":"A268","pred":"chebi_id","subj":"T268","obj":"http://purl.obolibrary.org/obo/CHEBI_50803"},{"id":"A269","pred":"chebi_id","subj":"T269","obj":"http://purl.obolibrary.org/obo/CHEBI_26708"},{"id":"A270","pred":"chebi_id","subj":"T270","obj":"http://purl.obolibrary.org/obo/CHEBI_33331"},{"id":"A271","pred":"chebi_id","subj":"T271","obj":"http://purl.obolibrary.org/obo/CHEBI_17051"},{"id":"A272","pred":"chebi_id","subj":"T272","obj":"http://purl.obolibrary.org/obo/CHEBI_50803"},{"id":"A273","pred":"chebi_id","subj":"T273","obj":"http://purl.obolibrary.org/obo/CHEBI_51218"},{"id":"A274","pred":"chebi_id","subj":"T274","obj":"http://purl.obolibrary.org/obo/CHEBI_27363"},{"id":"A275","pred":"chebi_id","subj":"T274","obj":"http://purl.obolibrary.org/obo/CHEBI_30185"},{"id":"A276","pred":"chebi_id","subj":"T276","obj":"http://purl.obolibrary.org/obo/CHEBI_34921"},{"id":"A277","pred":"chebi_id","subj":"T277","obj":"http://purl.obolibrary.org/obo/CHEBI_144613"},{"id":"A278","pred":"chebi_id","subj":"T277","obj":"http://purl.obolibrary.org/obo/CHEBI_53231"},{"id":"A279","pred":"chebi_id","subj":"T279","obj":"http://purl.obolibrary.org/obo/CHEBI_22587"},{"id":"A280","pred":"chebi_id","subj":"T280","obj":"http://purl.obolibrary.org/obo/CHEBI_27081"},{"id":"A281","pred":"chebi_id","subj":"T281","obj":"http://purl.obolibrary.org/obo/CHEBI_50882"},{"id":"A282","pred":"chebi_id","subj":"T282","obj":"http://purl.obolibrary.org/obo/CHEBI_18291"},{"id":"A283","pred":"chebi_id","subj":"T283","obj":"http://purl.obolibrary.org/obo/CHEBI_27081"},{"id":"A284","pred":"chebi_id","subj":"T284","obj":"http://purl.obolibrary.org/obo/CHEBI_33341"},{"id":"A285","pred":"chebi_id","subj":"T285","obj":"http://purl.obolibrary.org/obo/CHEBI_33342"},{"id":"A286","pred":"chebi_id","subj":"T286","obj":"http://purl.obolibrary.org/obo/CHEBI_28685"},{"id":"A287","pred":"chebi_id","subj":"T287","obj":"http://purl.obolibrary.org/obo/CHEBI_23116"},{"id":"A288","pred":"chebi_id","subj":"T288","obj":"http://purl.obolibrary.org/obo/CHEBI_74061"},{"id":"A289","pred":"chebi_id","subj":"T289","obj":"http://purl.obolibrary.org/obo/CHEBI_53228"},{"id":"A290","pred":"chebi_id","subj":"T289","obj":"http://purl.obolibrary.org/obo/CHEBI_60622"},{"id":"A291","pred":"chebi_id","subj":"T291","obj":"http://purl.obolibrary.org/obo/CHEBI_74061"},{"id":"A292","pred":"chebi_id","subj":"T292","obj":"http://purl.obolibrary.org/obo/CHEBI_53228"},{"id":"A293","pred":"chebi_id","subj":"T292","obj":"http://purl.obolibrary.org/obo/CHEBI_60622"},{"id":"A294","pred":"chebi_id","subj":"T294","obj":"http://purl.obolibrary.org/obo/CHEBI_36973"},{"id":"A295","pred":"chebi_id","subj":"T295","obj":"http://purl.obolibrary.org/obo/CHEBI_53228"},{"id":"A296","pred":"chebi_id","subj":"T295","obj":"http://purl.obolibrary.org/obo/CHEBI_60622"},{"id":"A297","pred":"chebi_id","subj":"T297","obj":"http://purl.obolibrary.org/obo/CHEBI_36973"},{"id":"A298","pred":"chebi_id","subj":"T298","obj":"http://purl.obolibrary.org/obo/CHEBI_28659"},{"id":"A299","pred":"chebi_id","subj":"T298","obj":"http://purl.obolibrary.org/obo/CHEBI_35895"},{"id":"A300","pred":"chebi_id","subj":"T300","obj":"http://purl.obolibrary.org/obo/CHEBI_29308"},{"id":"A301","pred":"chebi_id","subj":"T301","obj":"http://purl.obolibrary.org/obo/CHEBI_27594"},{"id":"A302","pred":"chebi_id","subj":"T301","obj":"http://purl.obolibrary.org/obo/CHEBI_33415"},{"id":"A303","pred":"chebi_id","subj":"T303","obj":"http://purl.obolibrary.org/obo/CHEBI_29240"},{"id":"A304","pred":"chebi_id","subj":"T303","obj":"http://purl.obolibrary.org/obo/CHEBI_50882"},{"id":"A305","pred":"chebi_id","subj":"T305","obj":"http://purl.obolibrary.org/obo/CHEBI_30521"},{"id":"A306","pred":"chebi_id","subj":"T306","obj":"http://purl.obolibrary.org/obo/CHEBI_27998"},{"id":"A307","pred":"chebi_id","subj":"T307","obj":"http://purl.obolibrary.org/obo/CHEBI_48343"},{"id":"A308","pred":"chebi_id","subj":"T308","obj":"http://purl.obolibrary.org/obo/CHEBI_30704"},{"id":"A309","pred":"chebi_id","subj":"T309","obj":"http://purl.obolibrary.org/obo/CHEBI_28685"},{"id":"A310","pred":"chebi_id","subj":"T310","obj":"http://purl.obolibrary.org/obo/CHEBI_48343"},{"id":"A311","pred":"chebi_id","subj":"T311","obj":"http://purl.obolibrary.org/obo/CHEBI_53228"},{"id":"A312","pred":"chebi_id","subj":"T311","obj":"http://purl.obolibrary.org/obo/CHEBI_60622"},{"id":"A313","pred":"chebi_id","subj":"T313","obj":"http://purl.obolibrary.org/obo/CHEBI_53228"},{"id":"A314","pred":"chebi_id","subj":"T313","obj":"http://purl.obolibrary.org/obo/CHEBI_60622"},{"id":"A315","pred":"chebi_id","subj":"T315","obj":"http://purl.obolibrary.org/obo/CHEBI_50803"},{"id":"A316","pred":"chebi_id","subj":"T315","obj":"http://purl.obolibrary.org/obo/CHEBI_53793"},{"id":"A317","pred":"chebi_id","subj":"T315","obj":"http://purl.obolibrary.org/obo/CHEBI_73425"},{"id":"A318","pred":"chebi_id","subj":"T318","obj":"http://purl.obolibrary.org/obo/CHEBI_60004"},{"id":"A319","pred":"chebi_id","subj":"T319","obj":"http://purl.obolibrary.org/obo/CHEBI_50860"},{"id":"A320","pred":"chebi_id","subj":"T319","obj":"http://purl.obolibrary.org/obo/CHEBI_72695"},{"id":"A321","pred":"chebi_id","subj":"T321","obj":"http://purl.obolibrary.org/obo/CHEBI_50803"},{"id":"A322","pred":"chebi_id","subj":"T322","obj":"http://purl.obolibrary.org/obo/CHEBI_36080"},{"id":"A323","pred":"chebi_id","subj":"T323","obj":"http://purl.obolibrary.org/obo/CHEBI_36080"},{"id":"A324","pred":"chebi_id","subj":"T324","obj":"http://purl.obolibrary.org/obo/CHEBI_50803"},{"id":"A325","pred":"chebi_id","subj":"T325","obj":"http://purl.obolibrary.org/obo/CHEBI_50803"},{"id":"A326","pred":"chebi_id","subj":"T326","obj":"http://purl.obolibrary.org/obo/CHEBI_30512"},{"id":"A327","pred":"chebi_id","subj":"T326","obj":"http://purl.obolibrary.org/obo/CHEBI_9141"},{"id":"A328","pred":"chebi_id","subj":"T328","obj":"http://purl.obolibrary.org/obo/CHEBI_23888"},{"id":"A329","pred":"chebi_id","subj":"T329","obj":"http://purl.obolibrary.org/obo/CHEBI_50803"},{"id":"A330","pred":"chebi_id","subj":"T330","obj":"http://purl.obolibrary.org/obo/CHEBI_23888"},{"id":"A331","pred":"chebi_id","subj":"T331","obj":"http://purl.obolibrary.org/obo/CHEBI_23888"},{"id":"A332","pred":"chebi_id","subj":"T332","obj":"http://purl.obolibrary.org/obo/CHEBI_23888"},{"id":"A333","pred":"chebi_id","subj":"T333","obj":"http://purl.obolibrary.org/obo/CHEBI_23888"},{"id":"A334","pred":"chebi_id","subj":"T334","obj":"http://purl.obolibrary.org/obo/CHEBI_36080"},{"id":"A335","pred":"chebi_id","subj":"T335","obj":"http://purl.obolibrary.org/obo/CHEBI_50803"},{"id":"A336","pred":"chebi_id","subj":"T336","obj":"http://purl.obolibrary.org/obo/CHEBI_50803"},{"id":"A337","pred":"chebi_id","subj":"T337","obj":"http://purl.obolibrary.org/obo/CHEBI_37409"},{"id":"A338","pred":"chebi_id","subj":"T338","obj":"http://purl.obolibrary.org/obo/CHEBI_36080"},{"id":"A339","pred":"chebi_id","subj":"T339","obj":"http://purl.obolibrary.org/obo/CHEBI_33709"},{"id":"A340","pred":"chebi_id","subj":"T340","obj":"http://purl.obolibrary.org/obo/CHEBI_46882"},{"id":"A341","pred":"chebi_id","subj":"T341","obj":"http://purl.obolibrary.org/obo/CHEBI_37527"},{"id":"A342","pred":"chebi_id","subj":"T342","obj":"http://purl.obolibrary.org/obo/CHEBI_18085"},{"id":"A343","pred":"chebi_id","subj":"T343","obj":"http://purl.obolibrary.org/obo/CHEBI_18085"},{"id":"A344","pred":"chebi_id","subj":"T344","obj":"http://purl.obolibrary.org/obo/CHEBI_28815"},{"id":"A345","pred":"chebi_id","subj":"T345","obj":"http://purl.obolibrary.org/obo/CHEBI_24500"},{"id":"A346","pred":"chebi_id","subj":"T346","obj":"http://purl.obolibrary.org/obo/CHEBI_16189"},{"id":"A347","pred":"chebi_id","subj":"T347","obj":"http://purl.obolibrary.org/obo/CHEBI_74056"},{"id":"A348","pred":"chebi_id","subj":"T347","obj":"http://purl.obolibrary.org/obo/CHEBI_28815"},{"id":"A349","pred":"chebi_id","subj":"T349","obj":"http://purl.obolibrary.org/obo/CHEBI_25367"},{"id":"A350","pred":"chebi_id","subj":"T350","obj":"http://purl.obolibrary.org/obo/CHEBI_35222"},{"id":"A351","pred":"chebi_id","subj":"T351","obj":"http://purl.obolibrary.org/obo/CHEBI_50803"},{"id":"A352","pred":"chebi_id","subj":"T352","obj":"http://purl.obolibrary.org/obo/CHEBI_29287"},{"id":"A353","pred":"chebi_id","subj":"T353","obj":"http://purl.obolibrary.org/obo/CHEBI_50803"},{"id":"A354","pred":"chebi_id","subj":"T354","obj":"http://purl.obolibrary.org/obo/CHEBI_35222"},{"id":"A355","pred":"chebi_id","subj":"T355","obj":"http://purl.obolibrary.org/obo/CHEBI_28815"},{"id":"A356","pred":"chebi_id","subj":"T356","obj":"http://purl.obolibrary.org/obo/CHEBI_24500"},{"id":"A357","pred":"chebi_id","subj":"T357","obj":"http://purl.obolibrary.org/obo/CHEBI_16189"},{"id":"A358","pred":"chebi_id","subj":"T358","obj":"http://purl.obolibrary.org/obo/CHEBI_29287"},{"id":"A359","pred":"chebi_id","subj":"T359","obj":"http://purl.obolibrary.org/obo/CHEBI_50803"},{"id":"A360","pred":"chebi_id","subj":"T360","obj":"http://purl.obolibrary.org/obo/CHEBI_50803"},{"id":"A361","pred":"chebi_id","subj":"T361","obj":"http://purl.obolibrary.org/obo/CHEBI_50803"},{"id":"A362","pred":"chebi_id","subj":"T362","obj":"http://purl.obolibrary.org/obo/CHEBI_29287"},{"id":"A363","pred":"chebi_id","subj":"T362","obj":"http://purl.obolibrary.org/obo/CHEBI_30050"},{"id":"A364","pred":"chebi_id","subj":"T364","obj":"http://purl.obolibrary.org/obo/CHEBI_50803"},{"id":"A365","pred":"chebi_id","subj":"T365","obj":"http://purl.obolibrary.org/obo/CHEBI_35962"},{"id":"A366","pred":"chebi_id","subj":"T365","obj":"http://purl.obolibrary.org/obo/CHEBI_38358"},{"id":"A367","pred":"chebi_id","subj":"T365","obj":"http://purl.obolibrary.org/obo/CHEBI_45373"},{"id":"A368","pred":"chebi_id","subj":"T365","obj":"http://purl.obolibrary.org/obo/CHEBI_74801"},{"id":"A369","pred":"chebi_id","subj":"T365","obj":"http://purl.obolibrary.org/obo/CHEBI_26667"},{"id":"A370","pred":"chebi_id","subj":"T370","obj":"http://purl.obolibrary.org/obo/CHEBI_36080"},{"id":"A371","pred":"chebi_id","subj":"T371","obj":"http://purl.obolibrary.org/obo/CHEBI_35962"},{"id":"A372","pred":"chebi_id","subj":"T371","obj":"http://purl.obolibrary.org/obo/CHEBI_38358"},{"id":"A373","pred":"chebi_id","subj":"T371","obj":"http://purl.obolibrary.org/obo/CHEBI_45373"},{"id":"A374","pred":"chebi_id","subj":"T371","obj":"http://purl.obolibrary.org/obo/CHEBI_74801"},{"id":"A375","pred":"chebi_id","subj":"T371","obj":"http://purl.obolibrary.org/obo/CHEBI_26667"},{"id":"A376","pred":"chebi_id","subj":"T376","obj":"http://purl.obolibrary.org/obo/CHEBI_22587"},{"id":"A377","pred":"chebi_id","subj":"T377","obj":"http://purl.obolibrary.org/obo/CHEBI_50803"},{"id":"A378","pred":"chebi_id","subj":"T378","obj":"http://purl.obolibrary.org/obo/CHEBI_29287"},{"id":"A379","pred":"chebi_id","subj":"T379","obj":"http://purl.obolibrary.org/obo/CHEBI_50816"},{"id":"A380","pred":"chebi_id","subj":"T379","obj":"http://purl.obolibrary.org/obo/CHEBI_50820"},{"id":"A381","pred":"chebi_id","subj":"T381","obj":"http://purl.obolibrary.org/obo/CHEBI_18248"},{"id":"A382","pred":"chebi_id","subj":"T382","obj":"http://purl.obolibrary.org/obo/CHEBI_25741"},{"id":"A383","pred":"chebi_id","subj":"T382","obj":"http://purl.obolibrary.org/obo/CHEBI_29356"},{"id":"A384","pred":"chebi_id","subj":"T384","obj":"http://purl.obolibrary.org/obo/CHEBI_74056"},{"id":"A385","pred":"chebi_id","subj":"T384","obj":"http://purl.obolibrary.org/obo/CHEBI_28815"},{"id":"A386","pred":"chebi_id","subj":"T386","obj":"http://purl.obolibrary.org/obo/CHEBI_50803"},{"id":"A387","pred":"chebi_id","subj":"T387","obj":"http://purl.obolibrary.org/obo/CHEBI_22587"},{"id":"A388","pred":"chebi_id","subj":"T388","obj":"http://purl.obolibrary.org/obo/CHEBI_36080"},{"id":"A389","pred":"chebi_id","subj":"T389","obj":"http://purl.obolibrary.org/obo/CHEBI_36080"},{"id":"A390","pred":"chebi_id","subj":"T390","obj":"http://purl.obolibrary.org/obo/CHEBI_78059"},{"id":"A391","pred":"chebi_id","subj":"T391","obj":"http://purl.obolibrary.org/obo/CHEBI_23888"},{"id":"A392","pred":"chebi_id","subj":"T392","obj":"http://purl.obolibrary.org/obo/CHEBI_25367"},{"id":"A393","pred":"chebi_id","subj":"T393","obj":"http://purl.obolibrary.org/obo/CHEBI_23888"},{"id":"A394","pred":"chebi_id","subj":"T394","obj":"http://purl.obolibrary.org/obo/CHEBI_23888"},{"id":"A395","pred":"chebi_id","subj":"T395","obj":"http://purl.obolibrary.org/obo/CHEBI_23888"},{"id":"A396","pred":"chebi_id","subj":"T396","obj":"http://purl.obolibrary.org/obo/CHEBI_23888"},{"id":"A397","pred":"chebi_id","subj":"T397","obj":"http://purl.obolibrary.org/obo/CHEBI_23888"},{"id":"A398","pred":"chebi_id","subj":"T398","obj":"http://purl.obolibrary.org/obo/CHEBI_23888"},{"id":"A399","pred":"chebi_id","subj":"T399","obj":"http://purl.obolibrary.org/obo/CHEBI_36080"},{"id":"A400","pred":"chebi_id","subj":"T400","obj":"http://purl.obolibrary.org/obo/CHEBI_23888"},{"id":"A401","pred":"chebi_id","subj":"T401","obj":"http://purl.obolibrary.org/obo/CHEBI_25367"},{"id":"A402","pred":"chebi_id","subj":"T402","obj":"http://purl.obolibrary.org/obo/CHEBI_74699"},{"id":"A403","pred":"chebi_id","subj":"T403","obj":"http://purl.obolibrary.org/obo/CHEBI_50803"},{"id":"A404","pred":"chebi_id","subj":"T404","obj":"http://purl.obolibrary.org/obo/CHEBI_74699"},{"id":"A405","pred":"chebi_id","subj":"T405","obj":"http://purl.obolibrary.org/obo/CHEBI_29287"},{"id":"A406","pred":"chebi_id","subj":"T406","obj":"http://purl.obolibrary.org/obo/CHEBI_51117"},{"id":"A407","pred":"chebi_id","subj":"T407","obj":"http://purl.obolibrary.org/obo/CHEBI_50805"},{"id":"A408","pred":"chebi_id","subj":"T408","obj":"http://purl.obolibrary.org/obo/CHEBI_50803"},{"id":"A409","pred":"chebi_id","subj":"T409","obj":"http://purl.obolibrary.org/obo/CHEBI_23888"},{"id":"A410","pred":"chebi_id","subj":"T410","obj":"http://purl.obolibrary.org/obo/CHEBI_23888"},{"id":"A411","pred":"chebi_id","subj":"T411","obj":"http://purl.obolibrary.org/obo/CHEBI_50803"},{"id":"A412","pred":"chebi_id","subj":"T411","obj":"http://purl.obolibrary.org/obo/CHEBI_53793"},{"id":"A413","pred":"chebi_id","subj":"T411","obj":"http://purl.obolibrary.org/obo/CHEBI_73425"}],"text":"Nanotechnology Tools to Inactivate SARS-CoV-2 in Patients\nThe main target of SARS-CoV-2 is the respiratory tract (upper airways, lung),36 although other organs might also be infected (e.g., gut, kidney) and vasculature21 also appears to be a prime target. The expression of ACE2 probably determines uptake by different tissues.37\nIn addition to discussing immune-based approaches, because the lung is the most critically affected organ, we will center our discussion on the various options to inactivate the virus in the deep lung and to target the essential host cells for drug delivery. The virus reaches the alveoli and enters alveolar epithelial type II cells (AECII), due to the relatively high abundance of ACE2 and a permissive cellular milieu. These cells serve as a reservoir of the virus, which finally spreads throughout the lung, leading to the lung function impairment seen in severe cases. Airborne nanomaterials are optimally suited to penetrate into the deep lung due to the physicochemical properties of such aerosols, existing on the same size scale particles that penetrate most readily to the deep airways. Hence, nanomedicine is already actively pursuing ideas to deliver drugs, therapeutic proteins, and mRNAs by exploiting nanodevices for pulmonary delivery.38,39\nMoreover, the rapid emergence of SARS-CoV-2 has exposed one of the main weaknesses in the current medical landscape: the lack of broad-spectrum antiviral drugs. At present, there are only a handful of approved antivirals, and they are mostly virus-specific. Hence, when a new virus emerges, little can be done pharmacologically to slow down its spread. Some research efforts have been focused on the development of broad-spectrum drugs, which could potentially offer some efficacy against future emerging viruses (and maybe SARS-CoV-2). The various approaches developed over the years are mainly based on the creation of entry inhibitors.40,41 A highly conserved part in viruses is the attachment ligand (VAL). In most known respiratory viruses,42 the VAL targets either heparan sulfate proteoglycans (HSPG)43 or sialic acids (SA).44 Both HSPG and SA mimics have shown in vitro ability to bind to viruses, blocking their interaction with cell membranes, and often in a broad-spectrum way.45−47\nIn the context of nanomedicine, many nanomaterials have been developed, ranging from polymers48 to dendrimers,49 oligomers, NPs,50 liposomes,51 and small molecules.52 However, successful clinical translation has been hindered by the fact that, upon dilution, these compounds lose efficacy as the virus-compound complex dissociates leaving viruses free to restart their replication cycle. Recently, it has been shown that this limitation can be overcome by synthesizing NPs that, after binding, are able to inhibit viral infectivity irreversibly by permanently damaging the virion, refueling the hope for a true, broad-spectrum antiviral drug.53 Because the focus is also on the development of a drug specific to SARS-CoV-2, a good entry inhibitor could be based on blocking the S spike protein interaction with the cellular ACE2 receptor.19,21−23 Regardless of the specific approach, it is imperative that novel, effective antivirals be based on compounds that exhibit very low or negligible toxicity profiles, as patients will most likely need to receive those drugs for extended periods of time and will already be weakened. For these reasons, when designing antiviral drugs, clearance mechanisms have to be kept in mind. An example of this process is the recent redesign of broad-spectrum antiviral NPs into equally effective modified cyclodextrins.54 Moreover, nanotechnology may offer nanotheranostic approaches to fill the existing gap between diagnostics and therapy.55−57 The simultaneous management of both diagnostics and therapy for those suffering from COVID-19 or in future pandemics, as for many other diseases, is an additional potential strategy to take into consideration in which nanomaterials have proven to be effective tools. The advantages of the capabilities of nanotechnology and nanomaterials for combined therapeutics and diagnostics has been widely explored in cancer research; however, there have been considerable efforts in the past few years to extend the scope of this approach to other areas including infectious diseases.58\n\nNanomaterial-Based Vaccine Development and Immunomodulation\nFollowing the publication of the genetic sequence of SARS-CoV-2 on January 11, 2020, intense research efforts have been devoted to developing a vaccine against COVID-19. With unprecedented speed, this extraordinary scientific mobilization led the first vaccine candidate to enter the Phase I human clinical trial on March 16, 2020, and other novel candidates are rapidly following.59 Up to May 22, 2020, there are 10 COVID-19 candidate vaccines in clinical evaluations and 114 in preclinical development.60\nConcerning vaccine and immunization research, nanomaterials can assist in multiple ways to boost the upregulation required by the immune system and to direct the immune response specifically against antigens. Immune-targeted nanotherapeutics can be developed through their rational manufacture at the nanoscale level by designing nanomaterials that are able to amplify host’s immune response, for instance as adjuvants in the context of vaccination.\nThe development of a vaccine will rely either on the direct administration of viral antigens (e.g., in the form of recombinant proteins, vectored vaccines, or whole inactivated or attenuated virus) or RNA- or DNA-encoding viral antigens.27 Candidate antigens for immunization are surface proteins such as the immunogenic spike protein (S1), which is already targeted by antibodies of convalescent patients.27 Because the S1 protein is also essential for cellular uptake, many researchers are using this protein as the primary target for a vaccine.\nThere are many issues related to the delivery of a drug, protein, or RNA into the patient as the cargo is often degraded, not bioavailable, or is swiftly cleared. Nanotechnology provides multiple solutions to these challenges though, as nanocarriers can overcome some of these limitations.\nBiocompatible polymeric-, lipid-based, or inorganic NPs can be tuned with respect to their physicochemical properties to encapsulate cargo proteins with high loading efficiency, improving protein delivery and pharmacokinetics over conventional approaches.38 Intranasal delivery of polymer-encapsulated antigen triggers a strong immune response, and the success of vaccination depends on the appropriate type of polymer in combination with the antigen.61,62 Similarly, researchers have developed lipid and lipid-based NPs as delivery platforms for mRNAs or siRNAs to enable the synthesis of key viral proteins for vaccination or to inactivate critical viral target genes, respectively.39 The field of therapeutic mRNAs to support vaccination has gained momentum, as well,63 at least in part due to nanotechnology-enabled strategies for cargo delivery. Moreover, the versatility of nanoplatforms as antigen-presenting systems offers great opportunities. Considering the possibility of random viral mutations that may alter the antigen shape, functionalizing nanomaterials with a wide number of molecules at the same time to target the virus, or motifs that are specific for pathogens, will increase the efficiency of vaccines and their ability to prevent viral infection.\nSeveral companies are working on mRNA vaccines encoding SARS-CoV-2 proteins such as the spike protein, encapsulated in nanoliposomes with specific physicochemical properties that are potentially akin to those documented for immunization against certain tumor antigens.64 The design of such nanocarriers, which will need to escape recognition by scavenger cells and to be nontoxic and nonimmunogenic, is a challenge that will require substantial time prior to clinical availability.\nOn March 16, 2020, Moderna, through a partnership with the Vaccine Research Center at the U.S. National Institutes of Health, enrolled the first participants into a Phase I clinical trial testing an mRNA vaccine (mRNA-1273) encapsulated in lipid NPs—a record time of just 63 days following sequence selection (NCT04283461).65 The enrollment of the first cohort of participants (18 to 55-year-old healthy subjects) concluded on April 16, 2020.66 CureVac and BioNTech (in partnership with Pfizer) are currently working on similar vaccines; Pfizer/BioNTech, in particular, have recently started the recruitment in Phase I/II trials (NCT04368728, NCT04380701). A DNA plasmid vaccine by Inovio Pharmaceuticals (INO-4800) has showed promising results in mice and guinea pigs according to a recent article published in Nature Communications(67) and has entered Phase I testing in humans (NCT04336410).\nAnother candidate COVID-19 vaccine for Phase I clinical trial is from the University of Oxford and AstraZeneca (NCT04324606).68 Around 1110 people will take part in the trial, which started recruitment at the end of April 2020. The vaccine is based on a chimpanzee adenovirus vaccine vector (ChAdOx1) and the SARS-CoV-2 spike protein. Chimpanzee adenoviral vectors against different pathogens have been already tested in thousands of subjects with demonstrated safety. To date, ChAdOx1 has been administered to six rhesus macaques exposed to high doses of SARS-CoV-2. The vaccine was unable to prevent infections, although it reduced the severity of the disease: no signs of virus replication were observed in the lungs, with significantly lower levels of respiratory disease and no lung damage compared to control animals, according to a recent article deposited in bioRxiv.69 Another adenoviral vector vaccine developed by CanSino Biological Inc. and Beijing Institute of Biotechnology using a genetically engineered replication-defective adenovirus type 5 vector to express the SARS-CoV-2 spike protein (Ad5-nCoV) is currently being tested in Phase I/II trials (NCT04398147, NCT04341389, NCT0431312).\nAlthough vaccines based on novel DNA and mRNA technologies might be promising, there are no such kinds of vaccines on the market, and it is unknown whether they can be effective in humans. Moderna, for instance, has generated preliminary safety data on different mRNA-based vaccines targeting other respiratory viruses, but their more advanced program (on a cytomegalovirus vaccine) is still in Phase II clinical testing. However, on May 18, Moderna announced that mRNA-1273 elicited antibody titers above the levels observed in convalescent individuals (and therefore considered potentially protective) in all eight initial participants across the 25 and 100 μg dose cohorts of the Phase I trial (NCT04283461).70 The company, after having received a fast-track approval from the FDA, is now moving on Phase II trials.\nConversely, strong evidence exists regarding the efficacy of protein-based vaccines such as recombinant-protein, viral-vector, attenuated, or inactivated vaccines across different infectious diseases, with licensed vaccines already existing for all of these platforms.27 All of the aforementioned approaches are currently being explored in the context of SARS-CoV-2.27 An inactivated vaccine developed by the China National Pharmaceutical Group (Sinopharm), in collaboration with the Wuhan Institute of Biological Products, is currently tested in a Phase I/II trial (ChiCTR2000031809), and a second inactivated vaccine (in collaboration with the Beijing Institute of Biological Products) has been currently approved for clinical testing (ChiCTR2000032459). A third aluminum salt (alum) adjuvanted inactivated vaccine, developed by Beijing-based Sinovac Biotech’s, was able to provide partial or complete protection in rhesus macaques, according to a recent article published in Science,71 and is currently being tested in Phase I/II trials (NCT04383574, NCT04352608).\nLive-attenuated vaccines are intrinsically immunogenic (e.g., due to the presence of viral DNA), but extensive safety tests are required due to the rare possibility of reversion to a pathogenic form able to cause infection. Vaccine candidates based in this application have previously been designed for SARS-CoV with high stability.72,73 Recombinant-protein vaccines and inactivated vaccines are safer but might require adjuvants to increase their immunogenicity. In the context of SARS-CoV-2, adjuvants are important for two reasons. First, adjuvants might increase the efficacy of the vaccine, especially in subjects with impaired immunological function, such as the elderly, or in subjects with comorbidities resulting in immune dysfunctions; in these patient cohorts, SARS-CoV-2 has a high lethality rate. Second, adjuvants can reduce the amount of vaccine protein(s) required per dose, which could facilitate scaling-up vaccine production in a reduced time frame.\nBeyond alum, which is in fact a nanoscale material74 that was developed in the 1920s for the tetanus and diphtheria toxoids,75 approval for a new adjuvant did not occur until 1997, with the introduction of the oil-in-water emulsion of squalene oil and polysorbate 80 and sorbitan trioleate surfactants (MF59) in the seasonal influenza vaccine for the elderly.76 The use of MF59 was further expanded to pandemic and avian influenza vaccines.77 Other adjuvants in licensed vaccines have been approved since 2000, as well: (a) AS03 (used for pandemic and avian influenza vaccines), similar to MF59, but including α-tocopherol as an additional immune stimulant; (b) AF 03 (used for pandemic influenza vaccines), an alternative squalene emulsion containing polyoxyethylene, cetostearyl ether, mannitol, and orbitan oleate;78 (c) AS 01 (used for herpes zoster vaccine), a liposome-based vaccine adjuvant system containing two immunostimulants, 3-O-desacyl-4′-monophosphoryl lipid A (MPL, a Toll-like receptor 4 agonist) and saponin QS-21, which activates the ACT-NLRP3 inflammasome pathway;79 and (d) AS04 (used for hepatitis B and human papilloma virus vaccines), which is a combination of MPL and aluminum hydroxide.76,80\nIn this context, the concept of “nanoimmunity by design” relies on the rational design of distinct physicochemical properties and specific functionalization of nanomaterials intended for fine-tuning their potential effects on the immune system.81 Nanomaterials have emerged as promising tools for immune modulation, either stimulating or suppressing the immune response. In fighting SARS-CoV-2, these properties may find applications for both prevention and therapy and in the context of vaccine development.\nMounting evidence indicates that nanomaterials such as graphene,82 nanodiamonds,83 carbon nanotubes,84 and polystyrene particles85 bear an intrinsic capacity to activate the immune system, depending on their functionalization.86 For instance, graphene oxide functionalized with amino groups (GO-NH2) induces activation of STAT1/IRF1 interferon signaling in monocytes and T cells, resulting in the production of T cell chemoattractants, and macrophage 1 (M1) 1/T-helper 1 (Th1) polarization of the immune response, with negligible toxicity.82 Remarkably, the ability of licensed adjuvants such as AS01 and AS03 to enhance adaptive immunity has been linked to their capacity to boost STAT1/IRF1 interferon signaling.87 In addition, recent SARS-CoV and MERS-CoV studies suggest that the development of a Th1-type response is central for controlling infection, which also may be true for SARS-CoV-2.88\nSeveral groups and consortia have been screening and characterizing nanomaterials according to their immunomodulatory properties and absence of cytotoxicity.56,57,82−84,86 The development of an adjuvant for clinical use is a lengthy process that requires extensive Phase III randomized trials in large and diverse cohorts of subjects, and the process generally requires several years.76 Pharmaceutical giants such as GlaxoSmithKline (GSK), which owns the ASs mentioned above and other adjuvant platforms, are engaged in several partnerships to embed their adjuvant systems with SARS-CoV-2-protein-based vaccines. A full-length recombinant SARS-CoV-2 glycoprotein nanoparticle vaccine adjuvanted with the saponin-based Matrix M developed by Novavax is in Phase I clinical testing (NCT04368988).89\nAlthough it is unlikely that novel adjuvants would be used in the context of the current pandemic, the SARS-CoV-2 pandemic offers an opportunity to reflect on the potential of nanotechnology for vaccine adjuvant development. In this context, it is critical to stream coherent pipelines covering in vitro and in vivo experiments specifically to select candidate materials that might be tested for clinical implementation as vaccine adjuvants.90\nIn this view, the status of nanomaterial-based vaccine adjuvants has been reviewed.91,92 In particular, immunomodulatory effects induced on the innate immune signaling have been demonstrated.91 For example, nanomaterials such as GO can elicit an inflammasome sensor (NLRP3)-dependent expression of IL-1β in macrophages.93 Notably, alum, the most commonly used adjuvant in human vaccines, induces the release of this cytokine in macrophages through the same NRLP-induced mechanism. These results suggest that nanomaterials such as GO and alum may be useful for medical applications.94\nConsidering that new vaccine development typically requires years to be approved and applied to the general population, there is also an urgent need to study how to improve treatment approaches. According to the first Phase III clinical studies, the antiviral drug Remdesivir, which was recently approved by the FDA for COVID-19 treatment in the United States, seems to be a promising treatment for adults diagnosed with COVID-19. Nano-based strategies have already been applied to enhance the effectiveness of Remdesivir in the context of other emerging viral infections (Nipah virus),95 suggesting the suitability of nanotechnology to assist with similar strategies for the treatment of COVID-19 as well as other possible pandemics in the future.96\n\nCan Nanotechnology Help to Control the Cytokine Storm?\nOne of the main features of COVID-19 is the triggering of a cytokine storm in the body, also known as cytokine release syndrome (CRS), which results from an excessive immune response and leads to the severe deterioration of patient health.25,97,98 This inflammatory storm is one of the major causes of the acute respiratory distress syndrome (ARDS) that is often associated with multiple-organ failure, representing the leading causes of death in critical patients.98 In particular, the role of interleukin (IL)-6 has been highlighted in patients requiring assisted ventilation. Ongoing clinical trials are testing drugs that block the receptor of IL-6 (Tocilizumab, an anti-IL-6 receptor antibody, and Sarilumab) or IL-6 itself (Siltuximab).\nWhereas a well-regulated cytokine response that is rapidly triggered by the host’s innate immunity can serve to prevent and to counteract an infection, an excessive, unbalanced, prolonged immune response can seriously harm the body. Therefore, therapeutic strategies aimed at effectively suppressing the cytokine storm are under investigation. Nanomaterials have been exploited to adjust the immune response to an optimized level, and such proprieties might be explored to inhibit cytokine releases.99 Nanosystems can enhance the specificity/efficiency of immunosuppressant delivery to target immune cells, with consequent reductions in drug dose, drug distribution to nontarget tissues and organs, and possible side effects. In addition, specific nanotools can be designed to evade the immune system and to enhance the solubility of poorly soluble immunosuppressant agents; the potential of finely tuning their surface charge opens possibilities for encapsulation strategies and offers accommodation for a high drug load. All of these mechanisms may also occur simultaneously, enhancing the activity of immunosuppressive agents.\nConcerning the role of macrophages in COVID-19, the presence of ACE2-expressing CD68+CD169+ macrophages containing SARS-CoV-2 nucleoprotein antigen and showing an increased release of IL-6 was observed in infected spleen and lymph nodes.100 Notably, immunohistochemical and immunofluorescence analyses of lymph nodes and spleen tissue from autopsy samples of patients who died from COVID-19 revealed lymphocytic apoptosis. The tissues infected by SARS-CoV-2 also showed an upregulated expression of Fas, suggesting a role for CD169+ macrophages in viral spreading, aberrant inflammation, and activation-induced lymphocyte apoptosis. Moreover, histological examinations of biopsy samples of patients who died from COVID-19 revealed an increased alveolar exudate due to the extended neutrophil and monocyte infiltrate in lung capillaries with fibrin deposition, probably leading to difficulties in gas exchange. Through nanomedicine, we envision therapeutic approaches aimed at targeting specific immune subpopulations to avoid these complications, and different nanomaterials have already been explored for their specific impact on different immune cell subpopulations.82,83,86 Octadecylamine-functionalized and dexamethasone-adsorbed nanodiamond promotes anti-inflammatory and proregenerative behavior in human macrophages in vitro.101 A low dose of this functionalized nanodiamond also reduced macrophage infiltration and expression of proinflammatory mediators iNOS and tumor necrosis factor (TNF)-α in mice. Overall, these results suggest that nanodiamond particles may be useful as an inherently immunomodulatory platform.\nFinally, the indiscriminate systemic suppression of the immune system may increase the susceptibility to sepsis due to underlying secondary bacterial infections that can exacerbate critical conditions in patients, causing death.102 Risk factors for mortality include acquired bacterial infections by opportunistic organisms and primary pathogens. Using nanocarriers to achieve targeted delivery of immunosuppressive drugs, or the specific and controlled inhibition of only a specific immune cell subpopulation, can play a critical role in limiting such complications.\nThe fast and complete removal of pro-inflammatory cytokines from the bloodstream has been shown to increase survival significantly in patients with early stage sepsis. Adsorption of cytokines from serum by extracorporeal perfusion through porous carbons is one of the most promising ways for their selective, quick, and complete removal from blood circulation. Hierarchical carbon materials with tuned porosity have shown effective adsorption of many cytokines, including IL-6 and even the largest ones, such as TNF-α.103 Based on their demonstrated outstanding performance in removing sepsis-associated pro-inflammatory cytokines from blood, mesoporous carbon adsorbents with hierarchical structure, tuned pore size, and surface chemistry, as well as graphene with open and accessible surfaces104 have significant potential to quickly remove inflammatory cytokines associated with CRS and to prevent mortality arising from an uncontrolled inflammatory cascade, thereby providing enough time for the defense mechanisms of the human body to fight the virus.\n\nPhotodynamic Inactivation of SARS-CoV-2\nIn addition to drug- and vaccine-based antiviral strategies, photodynamic therapy (PDT) stands as a unique approach to inactivate SARS-CoV-2. Using a light-based method, PDT attacks target cells via the excitation of photosensitive agents, called photosensitizers (PSs), with radiation characterized by a wavelength corresponding to its absorption spectrum to generate reactive oxygen species (ROS) in the presence of oxygen, which ultimately results in cell death. Photodynamic therapy is primarily used for the clinical treatment of various oncological disorders.105 It was not until the 1970s that PDT was first used clinically against viruses,106 exploiting ROS production to damage virus proteins, nucleic acids, and—if present—lipids.107 Even though there are research efforts for PDT-based virotherapies against different viruses, including herpes simplex virus, human papilloma virus, and human immunodeficiency virus,106−109 clinical use of PDT is limited due to hydrophobicity of PSs, poor target specificity, and limited tissue penetration ability.\nMost PSs are hydrophobic and aggregate in aqueous solutions, affecting their photochemical and photobiological properties.106,110 For this reason, Lim et al. have proposed a promising approach for photodynamic inactivation of viruses with NPs, developing sodium yttrium fluoride (NaYF4) upconversion NPs (UCNs) with zinc phthalocyanine PSs grafted onto their surfaces. Unlike most PSs, these UCNs are coated with polyethylenimine (PEI), which render them hydrophilic and easier to manipulate. These UCNs showed antiviral activity against Dengue virus serotype 2 and adenovirus type 5, which were used as models of enveloped and non-enveloped viruses, respectively.110 MXenes111,112 are a large family of 2D transition metal carbides, nitrides,113 and carbonitrides114 that exhibit unique electronic, optical, and catalytic properties. They have the general formula Mn+1XnTx, where M is an early transition metal (Ti, Zr, V, Mo, etc.), X is C and/or N, Tx represents the surface functional groups (=O, −OH, −F, −Cl), and n = 1–4.115,116 Some examples include Ti3C2Tx, V2CTx, and Nb2CTx, with over 30 stoichiometric compositions already synthesized with more than 100 predicted. Biocompatible MXenes, such as Ti3C2Tx, are hydrophilic and are among the most efficient light-to-heat transforming materials.117 The plasmon resonance extinction maxima of Ti3C2Tx is at 780 nm, enabling the use of near-infrared (IR) light for PDT. Several other MXenes have absorption maxima in the IR range and have shown outstanding performance in PDT and theranostic applications.118\nFullerene and graphene are also good candidates for virus inactivation by PDT and have proved to be effective against Semliki Forest virus (SFV), vesicular stomatitis virus (VSV), HSV-1, HIV-1, mosquito iridovirus (MIV), and influenza A virus (IAV), as well as the phage MS2.107 In addition, several 2D nanomaterials, including graphene-based materials, MXenes, black phosphorus, graphitic carbon nitride, tungsten disulfide, and molybdenum disulfide, have been reported to improve the efficacy of PDT considerably for cancer treatment.56,57 Therefore, determining if such nanomaterial-based PDT protocols could be exploited to inactivate SARS-CoV-2 is of great interest.\n\nBiomimetic Engineering of Nanodelivery Systems: Artificial Viruses in the Making\nIn an effort to engineer the next generation of nanoscale vectors, scientists have moved from using inorganic components aimed at obtaining inert structures to utilizing biological building blocks that are able to convey additional functionalities to the resulting construct. To cope with the complexity of the body and to evade the multiple layers of defense that tissues and organs have, it is critical to rely on the ability of certain materials to interact with, rather than to eschew, the biology of our body. Every NP system conceived to date faces one common fate: whether injected, inhaled, ingested, or absorbed through our epithelia, all will at some point come into contact with the mixture of fluids and organic compounds that comprise the body. Under such conditions, every material reacts in a unique way according to the conditions they individually face (i.e., the tissue or body region they are in), their composition (i.e., organic or inorganic), and their physical properties (i.e., size, shape, surface charge).119 Inorganic NPs can function as globular protein mimics because of their similar size, charge, shape, and surface features that can be chemically functionalized to resemble proteins. These similarities can be used in biotechnology to control virus pathways or cell receptor interactions with NPs.120\nAmong the many attempts, the legendary accomplishments, and the epic failures, we could list thousands of different NPs, differing from one another thanks to the creative endeavors of their designers. Despite their remarkable differences and researchers’ endeavors to make them one of a kind, they all aim to achieve one goal: to deliver in a specific way one particular form of payload, while remaining as unnoticed as possible by the body’s defense mechanisms. For everyone who has made an effort to create their own version of such a silver bullet, it has come to mind that nature in its incredible variety had already invented a few ingenious solutions to this problem. In the world of nano-based drug delivery, one entity dominates as the quintessential example of precision, efficiency, and stealth: the virus. Not by chance, these two worlds share many features that span from the physical laws that govern their assembly and stability to the chemical similarities in their overall composition.121\nHistorically, the field of nanomedicine and the global endeavor to generate NPs for drug delivery arose from one of the greatest struggles in medicine: gene therapy. At the core of that approach was the idea that viruses could be used as Trojan horses to deliver the correct sequence of the gene into the cells that harbored the mutated copy. In order to identify the virus that offered the best delivery service, a cadre of viral strains and species were tested, adapted, and engineered to fit the aims and hit the targets. It took decades of trial and error, of progressive adjustments, and a few tragic mistakes to identify the viruses that could provide the ideal backbone to develop viral vectors able to ferry genetic cargo into the target cell.122 In the midst of that global challenge, nanotechnology offered a safer and more controllable alternative: to generate bespoke structures that could replace viral vectors and do the same job, delivering a payload from the point of injection to the site of action.\nFast forward a few decades, and the promises made by both worlds seem to have finally become reality, with a number of active clinical trials, some clinical success stories, and a few commercial products in the market space. Interestingly, the two worlds seem to have maintained a safe distance so as to avoid any collision or dangerous proximity. Exchange of ideas between the fields of gene therapy and nanomedicine is limited, and the potential for disciplinary growth through knowledge exchange has remained elusive to scientists in both fields.5 Many scientists have suggested that nanotechnologists could find inspiration in the mechanisms devised by viruses to elude immune surveillance, to overcome biological barriers, and to deliver their genetic payload with high specificity. Similarly, gene therapists would find high-tech solutions to their scalability and safety issues in looking at the new generations of biomimetic particles being generated.\nThe principles of biomimicry and bioinspiration have been used to design and to engineer drug-delivery technologies that reproduce or recapitulate biological materials, for what pertains to not only their structure and chemistry but also, more importantly, their functions. In drug delivery, surface recognition and nanoscale interactions between materials and biological entities are key to the success of the delivery strategy, and the use of biological building blocks such as membrane proteins has been proposed as a way to convey targeting and shielding moieties simultaneously.123 One can only hope that the recent events and global attention that viruses are capturing in the scientific world will spur a renewed interest in finding ways to adapt viral features and mechanisms of action to the world of NPs. Increased focus in this field would be useful to create virus-like NPs able to circulate in the blood system, overcoming the endothelial barrier, and to deliver their therapeutic payload with high efficiency.124\n\nInterference with Cellular Uptake, Immobilization, and Inactivation of the Virus Outside of the Host Cell\nNanomaterials can be synthesized with a high specific surface area of a few hundred square meters per gram. Therefore, dependent on the surface properties, nanomaterials efficiently adsorb biomolecules and form a so-called biomolecular corona. This passive, nontargeted adsorption might be utilized to bind viruses, provided that the selected nanomaterial is relatively biocompatible. Viral surface proteins are often modified by sugar moieties or encompass positively charged amino acid patches that bind to lectins or glycosaminoglycans (GAGs) of heparan sulfate (HS), respectively.32 Robust interactions of virus particles with these host receptors is ensured by multivalent binding, which is likely why single-molecule inhibitors often are not capable of efficiently perturbing this key event but multivalent NPs are superior to block binding of different viruses to the host cell.125\nGold NPs capped with mercaptoethanesulfonate are effective inhibitors of HSV type 1 infection as they mimic cell-surface-receptor heparan sulfate and, therefore, competitively bind to the virus. Interestingly, polyvalent sulfated Au NPs inhibit virus binding to the host cell dependent on their size. Nanoparticles of diameters equal to and larger than the virus diameter (in this case, the stomatitis virus) more efficiently inhibit the binding to cells than smaller particles. Most likely, larger NPs efficiently cross-link virions, whereas smaller NPs simply decorate the viral surface.126 Papp et al. found that gold NPs decorated with SA effectively inhibited the binding of influenza virus to the target cells. In this case, viral recognition via its surface protein hemagglutinin of SA on the host cell membrane was a prerequisite for cellular entry.127 More recently, Cagno et al. reported antiviral NPs (Au and iron oxide core) with long and flexible linkers mimicking HS that strongly bind and inactivate viruses such as respiratory syncytial virus in vitro and in vivo in a lung infection model and even led to irreversible viral deformation.53 Hence, there is ample evidence that biocompatible, functionalized NPs can act as broad-spectrum antivirals. Notably, the receptor-binding domain of the spike S1 protein of SARS-CoV-2 binds not only to ACE2 but also to heparin128 and, thus, might be targeted by similar approaches as outlined above.\nOne of the most recent strategies to inhibit viral uptake is based on administration of recombinant ACE2 to inhibit binding competitively via the spike S1 protein.21 Knowing that multivalency is key to block virus–host interactions reliably, researchers have speculated that a nanostructured carrier could not only improve delivery and cargo stability but also might dramatically enhance binding strength.129\n\nSpeeding up the Nanomedicine-Based Approaches for COVID-19 by In Silico Analysis\nCurrently, repurposing drug molecules is a key strategy for identifying approved or investigational drugs outside the scope of the original medical indication that can be used to fight COVID-19.130,131 There are various advantages to this strategy, including already-established safety profiles, fast transition to clinical studies, and less investment needed, compared to the process of developing an entirely new drug.132,133 Therefore, these advantages have the potential to result in less risky and more rapid returns on investment in the development of repurposed drugs, benefits that are particularly important during pandemics. In addition to rapid diagnosis, making recommendations to physicians about rapid treatment methods can save the lives of many people. Combining in silico tools such as molecular docking, molecular dynamics, and computational chemistry with large drug databases provides a great advantage in selecting “possible candidates” from among thousands of pharmaceutically active substances (Figure 3). In silico analyses should be supported by the literature, expert opinions (pharmacologists and clinicians), and, when possible, preclinical and clinical findings. After selecting the best candidates following in silico analyses, detailed in vitro and in vivo experimentation should be undertaken prior to clinical studies. Recently, various drug-repurposing studies have been published to assist in the global COVID-19 pandemic response.134−136 Such studies aim to identify whether already-approved drugs have the capacity to interact with viral proteins or receptors on host cells.\nFigure 3 Schematic of how in silico analysis can be used to select candidate drug molecules for clinical studies. MD, molecular dynamics. In nanomedicine, computational models have recently garnered attention; such work may help to identify how nanomaterials interact with biological systems and to determine how the efficacy of these nanotherapeutics could be improved. Computational models indicate how NPs are taken up by healthy cells or tumor cells, enabling better predictions regarding the pharmacokinetic and pharmacodynamic properties of these materials.134 For example, Lunnoo et al. used a coarse-grained molecular dynamics (MD) simulation to observe the internalization pathways of various Au nanostructures (nanospheres, nanocages, nanorods, nanoplates, and nanohexapods) into an idealized mammalian plasma membrane.137 Other studies have simulated how different NPs can target tumor cells and deliver drugs.138,139 Therefore, in silico approaches that are currently used for drug repurposing, including molecular docking, molecular dynamics, and computational chemistry, constitute valuable tools to aid preclinical and clinical studies of nanomaterials directed for disease treatment. Considering the urgent need for nanomedicine against the current pandemic, in silico analyses may be especially useful in guiding the rational design of new NP formulations required to fight SARS-CoV-2."}
LitCovid-PD-HP
{"project":"LitCovid-PD-HP","denotations":[{"id":"T19","span":{"begin":4169,"end":4175},"obj":"Phenotype"},{"id":"T20","span":{"begin":7718,"end":7723},"obj":"Phenotype"},{"id":"T21","span":{"begin":14012,"end":14021},"obj":"Phenotype"},{"id":"T22","span":{"begin":14034,"end":14043},"obj":"Phenotype"},{"id":"T23","span":{"begin":18142,"end":18156},"obj":"Phenotype"},{"id":"T24","span":{"begin":18218,"end":18232},"obj":"Phenotype"},{"id":"T25","span":{"begin":18260,"end":18285},"obj":"Phenotype"},{"id":"T26","span":{"begin":18470,"end":18490},"obj":"Phenotype"},{"id":"T27","span":{"begin":19205,"end":19219},"obj":"Phenotype"},{"id":"T28","span":{"begin":21503,"end":21508},"obj":"Phenotype"},{"id":"T29","span":{"begin":21763,"end":21769},"obj":"Phenotype"},{"id":"T30","span":{"begin":22386,"end":22392},"obj":"Phenotype"},{"id":"T31","span":{"begin":22812,"end":22818},"obj":"Phenotype"},{"id":"T32","span":{"begin":23541,"end":23555},"obj":"Phenotype"},{"id":"T33","span":{"begin":24200,"end":24209},"obj":"Phenotype"},{"id":"T34","span":{"begin":24227,"end":24243},"obj":"Phenotype"},{"id":"T35","span":{"begin":26104,"end":26114},"obj":"Phenotype"},{"id":"T36","span":{"begin":26467,"end":26473},"obj":"Phenotype"},{"id":"T37","span":{"begin":33431,"end":33441},"obj":"Phenotype"},{"id":"T38","span":{"begin":37041,"end":37046},"obj":"Phenotype"},{"id":"T39","span":{"begin":37490,"end":37495},"obj":"Phenotype"}],"attributes":[{"id":"A19","pred":"hp_id","subj":"T19","obj":"http://purl.obolibrary.org/obo/HP_0002664"},{"id":"A20","pred":"hp_id","subj":"T20","obj":"http://purl.obolibrary.org/obo/HP_0002664"},{"id":"A21","pred":"hp_id","subj":"T21","obj":"http://purl.obolibrary.org/obo/HP_0012115"},{"id":"A22","pred":"hp_id","subj":"T22","obj":"http://purl.obolibrary.org/obo/HP_0012740"},{"id":"A23","pred":"hp_id","subj":"T23","obj":"http://purl.obolibrary.org/obo/HP_0033041"},{"id":"A24","pred":"hp_id","subj":"T24","obj":"http://purl.obolibrary.org/obo/HP_0033041"},{"id":"A25","pred":"hp_id","subj":"T25","obj":"http://purl.obolibrary.org/obo/HP_0033041"},{"id":"A26","pred":"hp_id","subj":"T26","obj":"http://purl.obolibrary.org/obo/HP_0002098"},{"id":"A27","pred":"hp_id","subj":"T27","obj":"http://purl.obolibrary.org/obo/HP_0033041"},{"id":"A28","pred":"hp_id","subj":"T28","obj":"http://purl.obolibrary.org/obo/HP_0002664"},{"id":"A29","pred":"hp_id","subj":"T29","obj":"http://purl.obolibrary.org/obo/HP_0100806"},{"id":"A30","pred":"hp_id","subj":"T30","obj":"http://purl.obolibrary.org/obo/HP_0100806"},{"id":"A31","pred":"hp_id","subj":"T31","obj":"http://purl.obolibrary.org/obo/HP_0100806"},{"id":"A32","pred":"hp_id","subj":"T32","obj":"http://purl.obolibrary.org/obo/HP_0000992"},{"id":"A33","pred":"hp_id","subj":"T33","obj":"http://purl.obolibrary.org/obo/HP_0012740"},{"id":"A34","pred":"hp_id","subj":"T34","obj":"http://purl.obolibrary.org/obo/HP_0002721"},{"id":"A35","pred":"hp_id","subj":"T35","obj":"http://purl.obolibrary.org/obo/HP_0010280"},{"id":"A36","pred":"hp_id","subj":"T36","obj":"http://purl.obolibrary.org/obo/HP_0002664"},{"id":"A37","pred":"hp_id","subj":"T37","obj":"http://purl.obolibrary.org/obo/HP_0010280"},{"id":"A38","pred":"hp_id","subj":"T38","obj":"http://purl.obolibrary.org/obo/HP_0002664"},{"id":"A39","pred":"hp_id","subj":"T39","obj":"http://purl.obolibrary.org/obo/HP_0002664"}],"text":"Nanotechnology Tools to Inactivate SARS-CoV-2 in Patients\nThe main target of SARS-CoV-2 is the respiratory tract (upper airways, lung),36 although other organs might also be infected (e.g., gut, kidney) and vasculature21 also appears to be a prime target. The expression of ACE2 probably determines uptake by different tissues.37\nIn addition to discussing immune-based approaches, because the lung is the most critically affected organ, we will center our discussion on the various options to inactivate the virus in the deep lung and to target the essential host cells for drug delivery. The virus reaches the alveoli and enters alveolar epithelial type II cells (AECII), due to the relatively high abundance of ACE2 and a permissive cellular milieu. These cells serve as a reservoir of the virus, which finally spreads throughout the lung, leading to the lung function impairment seen in severe cases. Airborne nanomaterials are optimally suited to penetrate into the deep lung due to the physicochemical properties of such aerosols, existing on the same size scale particles that penetrate most readily to the deep airways. Hence, nanomedicine is already actively pursuing ideas to deliver drugs, therapeutic proteins, and mRNAs by exploiting nanodevices for pulmonary delivery.38,39\nMoreover, the rapid emergence of SARS-CoV-2 has exposed one of the main weaknesses in the current medical landscape: the lack of broad-spectrum antiviral drugs. At present, there are only a handful of approved antivirals, and they are mostly virus-specific. Hence, when a new virus emerges, little can be done pharmacologically to slow down its spread. Some research efforts have been focused on the development of broad-spectrum drugs, which could potentially offer some efficacy against future emerging viruses (and maybe SARS-CoV-2). The various approaches developed over the years are mainly based on the creation of entry inhibitors.40,41 A highly conserved part in viruses is the attachment ligand (VAL). In most known respiratory viruses,42 the VAL targets either heparan sulfate proteoglycans (HSPG)43 or sialic acids (SA).44 Both HSPG and SA mimics have shown in vitro ability to bind to viruses, blocking their interaction with cell membranes, and often in a broad-spectrum way.45−47\nIn the context of nanomedicine, many nanomaterials have been developed, ranging from polymers48 to dendrimers,49 oligomers, NPs,50 liposomes,51 and small molecules.52 However, successful clinical translation has been hindered by the fact that, upon dilution, these compounds lose efficacy as the virus-compound complex dissociates leaving viruses free to restart their replication cycle. Recently, it has been shown that this limitation can be overcome by synthesizing NPs that, after binding, are able to inhibit viral infectivity irreversibly by permanently damaging the virion, refueling the hope for a true, broad-spectrum antiviral drug.53 Because the focus is also on the development of a drug specific to SARS-CoV-2, a good entry inhibitor could be based on blocking the S spike protein interaction with the cellular ACE2 receptor.19,21−23 Regardless of the specific approach, it is imperative that novel, effective antivirals be based on compounds that exhibit very low or negligible toxicity profiles, as patients will most likely need to receive those drugs for extended periods of time and will already be weakened. For these reasons, when designing antiviral drugs, clearance mechanisms have to be kept in mind. An example of this process is the recent redesign of broad-spectrum antiviral NPs into equally effective modified cyclodextrins.54 Moreover, nanotechnology may offer nanotheranostic approaches to fill the existing gap between diagnostics and therapy.55−57 The simultaneous management of both diagnostics and therapy for those suffering from COVID-19 or in future pandemics, as for many other diseases, is an additional potential strategy to take into consideration in which nanomaterials have proven to be effective tools. The advantages of the capabilities of nanotechnology and nanomaterials for combined therapeutics and diagnostics has been widely explored in cancer research; however, there have been considerable efforts in the past few years to extend the scope of this approach to other areas including infectious diseases.58\n\nNanomaterial-Based Vaccine Development and Immunomodulation\nFollowing the publication of the genetic sequence of SARS-CoV-2 on January 11, 2020, intense research efforts have been devoted to developing a vaccine against COVID-19. With unprecedented speed, this extraordinary scientific mobilization led the first vaccine candidate to enter the Phase I human clinical trial on March 16, 2020, and other novel candidates are rapidly following.59 Up to May 22, 2020, there are 10 COVID-19 candidate vaccines in clinical evaluations and 114 in preclinical development.60\nConcerning vaccine and immunization research, nanomaterials can assist in multiple ways to boost the upregulation required by the immune system and to direct the immune response specifically against antigens. Immune-targeted nanotherapeutics can be developed through their rational manufacture at the nanoscale level by designing nanomaterials that are able to amplify host’s immune response, for instance as adjuvants in the context of vaccination.\nThe development of a vaccine will rely either on the direct administration of viral antigens (e.g., in the form of recombinant proteins, vectored vaccines, or whole inactivated or attenuated virus) or RNA- or DNA-encoding viral antigens.27 Candidate antigens for immunization are surface proteins such as the immunogenic spike protein (S1), which is already targeted by antibodies of convalescent patients.27 Because the S1 protein is also essential for cellular uptake, many researchers are using this protein as the primary target for a vaccine.\nThere are many issues related to the delivery of a drug, protein, or RNA into the patient as the cargo is often degraded, not bioavailable, or is swiftly cleared. Nanotechnology provides multiple solutions to these challenges though, as nanocarriers can overcome some of these limitations.\nBiocompatible polymeric-, lipid-based, or inorganic NPs can be tuned with respect to their physicochemical properties to encapsulate cargo proteins with high loading efficiency, improving protein delivery and pharmacokinetics over conventional approaches.38 Intranasal delivery of polymer-encapsulated antigen triggers a strong immune response, and the success of vaccination depends on the appropriate type of polymer in combination with the antigen.61,62 Similarly, researchers have developed lipid and lipid-based NPs as delivery platforms for mRNAs or siRNAs to enable the synthesis of key viral proteins for vaccination or to inactivate critical viral target genes, respectively.39 The field of therapeutic mRNAs to support vaccination has gained momentum, as well,63 at least in part due to nanotechnology-enabled strategies for cargo delivery. Moreover, the versatility of nanoplatforms as antigen-presenting systems offers great opportunities. Considering the possibility of random viral mutations that may alter the antigen shape, functionalizing nanomaterials with a wide number of molecules at the same time to target the virus, or motifs that are specific for pathogens, will increase the efficiency of vaccines and their ability to prevent viral infection.\nSeveral companies are working on mRNA vaccines encoding SARS-CoV-2 proteins such as the spike protein, encapsulated in nanoliposomes with specific physicochemical properties that are potentially akin to those documented for immunization against certain tumor antigens.64 The design of such nanocarriers, which will need to escape recognition by scavenger cells and to be nontoxic and nonimmunogenic, is a challenge that will require substantial time prior to clinical availability.\nOn March 16, 2020, Moderna, through a partnership with the Vaccine Research Center at the U.S. National Institutes of Health, enrolled the first participants into a Phase I clinical trial testing an mRNA vaccine (mRNA-1273) encapsulated in lipid NPs—a record time of just 63 days following sequence selection (NCT04283461).65 The enrollment of the first cohort of participants (18 to 55-year-old healthy subjects) concluded on April 16, 2020.66 CureVac and BioNTech (in partnership with Pfizer) are currently working on similar vaccines; Pfizer/BioNTech, in particular, have recently started the recruitment in Phase I/II trials (NCT04368728, NCT04380701). A DNA plasmid vaccine by Inovio Pharmaceuticals (INO-4800) has showed promising results in mice and guinea pigs according to a recent article published in Nature Communications(67) and has entered Phase I testing in humans (NCT04336410).\nAnother candidate COVID-19 vaccine for Phase I clinical trial is from the University of Oxford and AstraZeneca (NCT04324606).68 Around 1110 people will take part in the trial, which started recruitment at the end of April 2020. The vaccine is based on a chimpanzee adenovirus vaccine vector (ChAdOx1) and the SARS-CoV-2 spike protein. Chimpanzee adenoviral vectors against different pathogens have been already tested in thousands of subjects with demonstrated safety. To date, ChAdOx1 has been administered to six rhesus macaques exposed to high doses of SARS-CoV-2. The vaccine was unable to prevent infections, although it reduced the severity of the disease: no signs of virus replication were observed in the lungs, with significantly lower levels of respiratory disease and no lung damage compared to control animals, according to a recent article deposited in bioRxiv.69 Another adenoviral vector vaccine developed by CanSino Biological Inc. and Beijing Institute of Biotechnology using a genetically engineered replication-defective adenovirus type 5 vector to express the SARS-CoV-2 spike protein (Ad5-nCoV) is currently being tested in Phase I/II trials (NCT04398147, NCT04341389, NCT0431312).\nAlthough vaccines based on novel DNA and mRNA technologies might be promising, there are no such kinds of vaccines on the market, and it is unknown whether they can be effective in humans. Moderna, for instance, has generated preliminary safety data on different mRNA-based vaccines targeting other respiratory viruses, but their more advanced program (on a cytomegalovirus vaccine) is still in Phase II clinical testing. However, on May 18, Moderna announced that mRNA-1273 elicited antibody titers above the levels observed in convalescent individuals (and therefore considered potentially protective) in all eight initial participants across the 25 and 100 μg dose cohorts of the Phase I trial (NCT04283461).70 The company, after having received a fast-track approval from the FDA, is now moving on Phase II trials.\nConversely, strong evidence exists regarding the efficacy of protein-based vaccines such as recombinant-protein, viral-vector, attenuated, or inactivated vaccines across different infectious diseases, with licensed vaccines already existing for all of these platforms.27 All of the aforementioned approaches are currently being explored in the context of SARS-CoV-2.27 An inactivated vaccine developed by the China National Pharmaceutical Group (Sinopharm), in collaboration with the Wuhan Institute of Biological Products, is currently tested in a Phase I/II trial (ChiCTR2000031809), and a second inactivated vaccine (in collaboration with the Beijing Institute of Biological Products) has been currently approved for clinical testing (ChiCTR2000032459). A third aluminum salt (alum) adjuvanted inactivated vaccine, developed by Beijing-based Sinovac Biotech’s, was able to provide partial or complete protection in rhesus macaques, according to a recent article published in Science,71 and is currently being tested in Phase I/II trials (NCT04383574, NCT04352608).\nLive-attenuated vaccines are intrinsically immunogenic (e.g., due to the presence of viral DNA), but extensive safety tests are required due to the rare possibility of reversion to a pathogenic form able to cause infection. Vaccine candidates based in this application have previously been designed for SARS-CoV with high stability.72,73 Recombinant-protein vaccines and inactivated vaccines are safer but might require adjuvants to increase their immunogenicity. In the context of SARS-CoV-2, adjuvants are important for two reasons. First, adjuvants might increase the efficacy of the vaccine, especially in subjects with impaired immunological function, such as the elderly, or in subjects with comorbidities resulting in immune dysfunctions; in these patient cohorts, SARS-CoV-2 has a high lethality rate. Second, adjuvants can reduce the amount of vaccine protein(s) required per dose, which could facilitate scaling-up vaccine production in a reduced time frame.\nBeyond alum, which is in fact a nanoscale material74 that was developed in the 1920s for the tetanus and diphtheria toxoids,75 approval for a new adjuvant did not occur until 1997, with the introduction of the oil-in-water emulsion of squalene oil and polysorbate 80 and sorbitan trioleate surfactants (MF59) in the seasonal influenza vaccine for the elderly.76 The use of MF59 was further expanded to pandemic and avian influenza vaccines.77 Other adjuvants in licensed vaccines have been approved since 2000, as well: (a) AS03 (used for pandemic and avian influenza vaccines), similar to MF59, but including α-tocopherol as an additional immune stimulant; (b) AF 03 (used for pandemic influenza vaccines), an alternative squalene emulsion containing polyoxyethylene, cetostearyl ether, mannitol, and orbitan oleate;78 (c) AS 01 (used for herpes zoster vaccine), a liposome-based vaccine adjuvant system containing two immunostimulants, 3-O-desacyl-4′-monophosphoryl lipid A (MPL, a Toll-like receptor 4 agonist) and saponin QS-21, which activates the ACT-NLRP3 inflammasome pathway;79 and (d) AS04 (used for hepatitis B and human papilloma virus vaccines), which is a combination of MPL and aluminum hydroxide.76,80\nIn this context, the concept of “nanoimmunity by design” relies on the rational design of distinct physicochemical properties and specific functionalization of nanomaterials intended for fine-tuning their potential effects on the immune system.81 Nanomaterials have emerged as promising tools for immune modulation, either stimulating or suppressing the immune response. In fighting SARS-CoV-2, these properties may find applications for both prevention and therapy and in the context of vaccine development.\nMounting evidence indicates that nanomaterials such as graphene,82 nanodiamonds,83 carbon nanotubes,84 and polystyrene particles85 bear an intrinsic capacity to activate the immune system, depending on their functionalization.86 For instance, graphene oxide functionalized with amino groups (GO-NH2) induces activation of STAT1/IRF1 interferon signaling in monocytes and T cells, resulting in the production of T cell chemoattractants, and macrophage 1 (M1) 1/T-helper 1 (Th1) polarization of the immune response, with negligible toxicity.82 Remarkably, the ability of licensed adjuvants such as AS01 and AS03 to enhance adaptive immunity has been linked to their capacity to boost STAT1/IRF1 interferon signaling.87 In addition, recent SARS-CoV and MERS-CoV studies suggest that the development of a Th1-type response is central for controlling infection, which also may be true for SARS-CoV-2.88\nSeveral groups and consortia have been screening and characterizing nanomaterials according to their immunomodulatory properties and absence of cytotoxicity.56,57,82−84,86 The development of an adjuvant for clinical use is a lengthy process that requires extensive Phase III randomized trials in large and diverse cohorts of subjects, and the process generally requires several years.76 Pharmaceutical giants such as GlaxoSmithKline (GSK), which owns the ASs mentioned above and other adjuvant platforms, are engaged in several partnerships to embed their adjuvant systems with SARS-CoV-2-protein-based vaccines. A full-length recombinant SARS-CoV-2 glycoprotein nanoparticle vaccine adjuvanted with the saponin-based Matrix M developed by Novavax is in Phase I clinical testing (NCT04368988).89\nAlthough it is unlikely that novel adjuvants would be used in the context of the current pandemic, the SARS-CoV-2 pandemic offers an opportunity to reflect on the potential of nanotechnology for vaccine adjuvant development. In this context, it is critical to stream coherent pipelines covering in vitro and in vivo experiments specifically to select candidate materials that might be tested for clinical implementation as vaccine adjuvants.90\nIn this view, the status of nanomaterial-based vaccine adjuvants has been reviewed.91,92 In particular, immunomodulatory effects induced on the innate immune signaling have been demonstrated.91 For example, nanomaterials such as GO can elicit an inflammasome sensor (NLRP3)-dependent expression of IL-1β in macrophages.93 Notably, alum, the most commonly used adjuvant in human vaccines, induces the release of this cytokine in macrophages through the same NRLP-induced mechanism. These results suggest that nanomaterials such as GO and alum may be useful for medical applications.94\nConsidering that new vaccine development typically requires years to be approved and applied to the general population, there is also an urgent need to study how to improve treatment approaches. According to the first Phase III clinical studies, the antiviral drug Remdesivir, which was recently approved by the FDA for COVID-19 treatment in the United States, seems to be a promising treatment for adults diagnosed with COVID-19. Nano-based strategies have already been applied to enhance the effectiveness of Remdesivir in the context of other emerging viral infections (Nipah virus),95 suggesting the suitability of nanotechnology to assist with similar strategies for the treatment of COVID-19 as well as other possible pandemics in the future.96\n\nCan Nanotechnology Help to Control the Cytokine Storm?\nOne of the main features of COVID-19 is the triggering of a cytokine storm in the body, also known as cytokine release syndrome (CRS), which results from an excessive immune response and leads to the severe deterioration of patient health.25,97,98 This inflammatory storm is one of the major causes of the acute respiratory distress syndrome (ARDS) that is often associated with multiple-organ failure, representing the leading causes of death in critical patients.98 In particular, the role of interleukin (IL)-6 has been highlighted in patients requiring assisted ventilation. Ongoing clinical trials are testing drugs that block the receptor of IL-6 (Tocilizumab, an anti-IL-6 receptor antibody, and Sarilumab) or IL-6 itself (Siltuximab).\nWhereas a well-regulated cytokine response that is rapidly triggered by the host’s innate immunity can serve to prevent and to counteract an infection, an excessive, unbalanced, prolonged immune response can seriously harm the body. Therefore, therapeutic strategies aimed at effectively suppressing the cytokine storm are under investigation. Nanomaterials have been exploited to adjust the immune response to an optimized level, and such proprieties might be explored to inhibit cytokine releases.99 Nanosystems can enhance the specificity/efficiency of immunosuppressant delivery to target immune cells, with consequent reductions in drug dose, drug distribution to nontarget tissues and organs, and possible side effects. In addition, specific nanotools can be designed to evade the immune system and to enhance the solubility of poorly soluble immunosuppressant agents; the potential of finely tuning their surface charge opens possibilities for encapsulation strategies and offers accommodation for a high drug load. All of these mechanisms may also occur simultaneously, enhancing the activity of immunosuppressive agents.\nConcerning the role of macrophages in COVID-19, the presence of ACE2-expressing CD68+CD169+ macrophages containing SARS-CoV-2 nucleoprotein antigen and showing an increased release of IL-6 was observed in infected spleen and lymph nodes.100 Notably, immunohistochemical and immunofluorescence analyses of lymph nodes and spleen tissue from autopsy samples of patients who died from COVID-19 revealed lymphocytic apoptosis. The tissues infected by SARS-CoV-2 also showed an upregulated expression of Fas, suggesting a role for CD169+ macrophages in viral spreading, aberrant inflammation, and activation-induced lymphocyte apoptosis. Moreover, histological examinations of biopsy samples of patients who died from COVID-19 revealed an increased alveolar exudate due to the extended neutrophil and monocyte infiltrate in lung capillaries with fibrin deposition, probably leading to difficulties in gas exchange. Through nanomedicine, we envision therapeutic approaches aimed at targeting specific immune subpopulations to avoid these complications, and different nanomaterials have already been explored for their specific impact on different immune cell subpopulations.82,83,86 Octadecylamine-functionalized and dexamethasone-adsorbed nanodiamond promotes anti-inflammatory and proregenerative behavior in human macrophages in vitro.101 A low dose of this functionalized nanodiamond also reduced macrophage infiltration and expression of proinflammatory mediators iNOS and tumor necrosis factor (TNF)-α in mice. Overall, these results suggest that nanodiamond particles may be useful as an inherently immunomodulatory platform.\nFinally, the indiscriminate systemic suppression of the immune system may increase the susceptibility to sepsis due to underlying secondary bacterial infections that can exacerbate critical conditions in patients, causing death.102 Risk factors for mortality include acquired bacterial infections by opportunistic organisms and primary pathogens. Using nanocarriers to achieve targeted delivery of immunosuppressive drugs, or the specific and controlled inhibition of only a specific immune cell subpopulation, can play a critical role in limiting such complications.\nThe fast and complete removal of pro-inflammatory cytokines from the bloodstream has been shown to increase survival significantly in patients with early stage sepsis. Adsorption of cytokines from serum by extracorporeal perfusion through porous carbons is one of the most promising ways for their selective, quick, and complete removal from blood circulation. Hierarchical carbon materials with tuned porosity have shown effective adsorption of many cytokines, including IL-6 and even the largest ones, such as TNF-α.103 Based on their demonstrated outstanding performance in removing sepsis-associated pro-inflammatory cytokines from blood, mesoporous carbon adsorbents with hierarchical structure, tuned pore size, and surface chemistry, as well as graphene with open and accessible surfaces104 have significant potential to quickly remove inflammatory cytokines associated with CRS and to prevent mortality arising from an uncontrolled inflammatory cascade, thereby providing enough time for the defense mechanisms of the human body to fight the virus.\n\nPhotodynamic Inactivation of SARS-CoV-2\nIn addition to drug- and vaccine-based antiviral strategies, photodynamic therapy (PDT) stands as a unique approach to inactivate SARS-CoV-2. Using a light-based method, PDT attacks target cells via the excitation of photosensitive agents, called photosensitizers (PSs), with radiation characterized by a wavelength corresponding to its absorption spectrum to generate reactive oxygen species (ROS) in the presence of oxygen, which ultimately results in cell death. Photodynamic therapy is primarily used for the clinical treatment of various oncological disorders.105 It was not until the 1970s that PDT was first used clinically against viruses,106 exploiting ROS production to damage virus proteins, nucleic acids, and—if present—lipids.107 Even though there are research efforts for PDT-based virotherapies against different viruses, including herpes simplex virus, human papilloma virus, and human immunodeficiency virus,106−109 clinical use of PDT is limited due to hydrophobicity of PSs, poor target specificity, and limited tissue penetration ability.\nMost PSs are hydrophobic and aggregate in aqueous solutions, affecting their photochemical and photobiological properties.106,110 For this reason, Lim et al. have proposed a promising approach for photodynamic inactivation of viruses with NPs, developing sodium yttrium fluoride (NaYF4) upconversion NPs (UCNs) with zinc phthalocyanine PSs grafted onto their surfaces. Unlike most PSs, these UCNs are coated with polyethylenimine (PEI), which render them hydrophilic and easier to manipulate. These UCNs showed antiviral activity against Dengue virus serotype 2 and adenovirus type 5, which were used as models of enveloped and non-enveloped viruses, respectively.110 MXenes111,112 are a large family of 2D transition metal carbides, nitrides,113 and carbonitrides114 that exhibit unique electronic, optical, and catalytic properties. They have the general formula Mn+1XnTx, where M is an early transition metal (Ti, Zr, V, Mo, etc.), X is C and/or N, Tx represents the surface functional groups (=O, −OH, −F, −Cl), and n = 1–4.115,116 Some examples include Ti3C2Tx, V2CTx, and Nb2CTx, with over 30 stoichiometric compositions already synthesized with more than 100 predicted. Biocompatible MXenes, such as Ti3C2Tx, are hydrophilic and are among the most efficient light-to-heat transforming materials.117 The plasmon resonance extinction maxima of Ti3C2Tx is at 780 nm, enabling the use of near-infrared (IR) light for PDT. Several other MXenes have absorption maxima in the IR range and have shown outstanding performance in PDT and theranostic applications.118\nFullerene and graphene are also good candidates for virus inactivation by PDT and have proved to be effective against Semliki Forest virus (SFV), vesicular stomatitis virus (VSV), HSV-1, HIV-1, mosquito iridovirus (MIV), and influenza A virus (IAV), as well as the phage MS2.107 In addition, several 2D nanomaterials, including graphene-based materials, MXenes, black phosphorus, graphitic carbon nitride, tungsten disulfide, and molybdenum disulfide, have been reported to improve the efficacy of PDT considerably for cancer treatment.56,57 Therefore, determining if such nanomaterial-based PDT protocols could be exploited to inactivate SARS-CoV-2 is of great interest.\n\nBiomimetic Engineering of Nanodelivery Systems: Artificial Viruses in the Making\nIn an effort to engineer the next generation of nanoscale vectors, scientists have moved from using inorganic components aimed at obtaining inert structures to utilizing biological building blocks that are able to convey additional functionalities to the resulting construct. To cope with the complexity of the body and to evade the multiple layers of defense that tissues and organs have, it is critical to rely on the ability of certain materials to interact with, rather than to eschew, the biology of our body. Every NP system conceived to date faces one common fate: whether injected, inhaled, ingested, or absorbed through our epithelia, all will at some point come into contact with the mixture of fluids and organic compounds that comprise the body. Under such conditions, every material reacts in a unique way according to the conditions they individually face (i.e., the tissue or body region they are in), their composition (i.e., organic or inorganic), and their physical properties (i.e., size, shape, surface charge).119 Inorganic NPs can function as globular protein mimics because of their similar size, charge, shape, and surface features that can be chemically functionalized to resemble proteins. These similarities can be used in biotechnology to control virus pathways or cell receptor interactions with NPs.120\nAmong the many attempts, the legendary accomplishments, and the epic failures, we could list thousands of different NPs, differing from one another thanks to the creative endeavors of their designers. Despite their remarkable differences and researchers’ endeavors to make them one of a kind, they all aim to achieve one goal: to deliver in a specific way one particular form of payload, while remaining as unnoticed as possible by the body’s defense mechanisms. For everyone who has made an effort to create their own version of such a silver bullet, it has come to mind that nature in its incredible variety had already invented a few ingenious solutions to this problem. In the world of nano-based drug delivery, one entity dominates as the quintessential example of precision, efficiency, and stealth: the virus. Not by chance, these two worlds share many features that span from the physical laws that govern their assembly and stability to the chemical similarities in their overall composition.121\nHistorically, the field of nanomedicine and the global endeavor to generate NPs for drug delivery arose from one of the greatest struggles in medicine: gene therapy. At the core of that approach was the idea that viruses could be used as Trojan horses to deliver the correct sequence of the gene into the cells that harbored the mutated copy. In order to identify the virus that offered the best delivery service, a cadre of viral strains and species were tested, adapted, and engineered to fit the aims and hit the targets. It took decades of trial and error, of progressive adjustments, and a few tragic mistakes to identify the viruses that could provide the ideal backbone to develop viral vectors able to ferry genetic cargo into the target cell.122 In the midst of that global challenge, nanotechnology offered a safer and more controllable alternative: to generate bespoke structures that could replace viral vectors and do the same job, delivering a payload from the point of injection to the site of action.\nFast forward a few decades, and the promises made by both worlds seem to have finally become reality, with a number of active clinical trials, some clinical success stories, and a few commercial products in the market space. Interestingly, the two worlds seem to have maintained a safe distance so as to avoid any collision or dangerous proximity. Exchange of ideas between the fields of gene therapy and nanomedicine is limited, and the potential for disciplinary growth through knowledge exchange has remained elusive to scientists in both fields.5 Many scientists have suggested that nanotechnologists could find inspiration in the mechanisms devised by viruses to elude immune surveillance, to overcome biological barriers, and to deliver their genetic payload with high specificity. Similarly, gene therapists would find high-tech solutions to their scalability and safety issues in looking at the new generations of biomimetic particles being generated.\nThe principles of biomimicry and bioinspiration have been used to design and to engineer drug-delivery technologies that reproduce or recapitulate biological materials, for what pertains to not only their structure and chemistry but also, more importantly, their functions. In drug delivery, surface recognition and nanoscale interactions between materials and biological entities are key to the success of the delivery strategy, and the use of biological building blocks such as membrane proteins has been proposed as a way to convey targeting and shielding moieties simultaneously.123 One can only hope that the recent events and global attention that viruses are capturing in the scientific world will spur a renewed interest in finding ways to adapt viral features and mechanisms of action to the world of NPs. Increased focus in this field would be useful to create virus-like NPs able to circulate in the blood system, overcoming the endothelial barrier, and to deliver their therapeutic payload with high efficiency.124\n\nInterference with Cellular Uptake, Immobilization, and Inactivation of the Virus Outside of the Host Cell\nNanomaterials can be synthesized with a high specific surface area of a few hundred square meters per gram. Therefore, dependent on the surface properties, nanomaterials efficiently adsorb biomolecules and form a so-called biomolecular corona. This passive, nontargeted adsorption might be utilized to bind viruses, provided that the selected nanomaterial is relatively biocompatible. Viral surface proteins are often modified by sugar moieties or encompass positively charged amino acid patches that bind to lectins or glycosaminoglycans (GAGs) of heparan sulfate (HS), respectively.32 Robust interactions of virus particles with these host receptors is ensured by multivalent binding, which is likely why single-molecule inhibitors often are not capable of efficiently perturbing this key event but multivalent NPs are superior to block binding of different viruses to the host cell.125\nGold NPs capped with mercaptoethanesulfonate are effective inhibitors of HSV type 1 infection as they mimic cell-surface-receptor heparan sulfate and, therefore, competitively bind to the virus. Interestingly, polyvalent sulfated Au NPs inhibit virus binding to the host cell dependent on their size. Nanoparticles of diameters equal to and larger than the virus diameter (in this case, the stomatitis virus) more efficiently inhibit the binding to cells than smaller particles. Most likely, larger NPs efficiently cross-link virions, whereas smaller NPs simply decorate the viral surface.126 Papp et al. found that gold NPs decorated with SA effectively inhibited the binding of influenza virus to the target cells. In this case, viral recognition via its surface protein hemagglutinin of SA on the host cell membrane was a prerequisite for cellular entry.127 More recently, Cagno et al. reported antiviral NPs (Au and iron oxide core) with long and flexible linkers mimicking HS that strongly bind and inactivate viruses such as respiratory syncytial virus in vitro and in vivo in a lung infection model and even led to irreversible viral deformation.53 Hence, there is ample evidence that biocompatible, functionalized NPs can act as broad-spectrum antivirals. Notably, the receptor-binding domain of the spike S1 protein of SARS-CoV-2 binds not only to ACE2 but also to heparin128 and, thus, might be targeted by similar approaches as outlined above.\nOne of the most recent strategies to inhibit viral uptake is based on administration of recombinant ACE2 to inhibit binding competitively via the spike S1 protein.21 Knowing that multivalency is key to block virus–host interactions reliably, researchers have speculated that a nanostructured carrier could not only improve delivery and cargo stability but also might dramatically enhance binding strength.129\n\nSpeeding up the Nanomedicine-Based Approaches for COVID-19 by In Silico Analysis\nCurrently, repurposing drug molecules is a key strategy for identifying approved or investigational drugs outside the scope of the original medical indication that can be used to fight COVID-19.130,131 There are various advantages to this strategy, including already-established safety profiles, fast transition to clinical studies, and less investment needed, compared to the process of developing an entirely new drug.132,133 Therefore, these advantages have the potential to result in less risky and more rapid returns on investment in the development of repurposed drugs, benefits that are particularly important during pandemics. In addition to rapid diagnosis, making recommendations to physicians about rapid treatment methods can save the lives of many people. Combining in silico tools such as molecular docking, molecular dynamics, and computational chemistry with large drug databases provides a great advantage in selecting “possible candidates” from among thousands of pharmaceutically active substances (Figure 3). In silico analyses should be supported by the literature, expert opinions (pharmacologists and clinicians), and, when possible, preclinical and clinical findings. After selecting the best candidates following in silico analyses, detailed in vitro and in vivo experimentation should be undertaken prior to clinical studies. Recently, various drug-repurposing studies have been published to assist in the global COVID-19 pandemic response.134−136 Such studies aim to identify whether already-approved drugs have the capacity to interact with viral proteins or receptors on host cells.\nFigure 3 Schematic of how in silico analysis can be used to select candidate drug molecules for clinical studies. MD, molecular dynamics. In nanomedicine, computational models have recently garnered attention; such work may help to identify how nanomaterials interact with biological systems and to determine how the efficacy of these nanotherapeutics could be improved. Computational models indicate how NPs are taken up by healthy cells or tumor cells, enabling better predictions regarding the pharmacokinetic and pharmacodynamic properties of these materials.134 For example, Lunnoo et al. used a coarse-grained molecular dynamics (MD) simulation to observe the internalization pathways of various Au nanostructures (nanospheres, nanocages, nanorods, nanoplates, and nanohexapods) into an idealized mammalian plasma membrane.137 Other studies have simulated how different NPs can target tumor cells and deliver drugs.138,139 Therefore, in silico approaches that are currently used for drug repurposing, including molecular docking, molecular dynamics, and computational chemistry, constitute valuable tools to aid preclinical and clinical studies of nanomaterials directed for disease treatment. Considering the urgent need for nanomedicine against the current pandemic, in silico analyses may be especially useful in guiding the rational design of new NP formulations required to fight SARS-CoV-2."}
LitCovid-PD-GO-BP
{"project":"LitCovid-PD-GO-BP","denotations":[{"id":"T20","span":{"begin":299,"end":305},"obj":"http://purl.obolibrary.org/obo/GO_0098739"},{"id":"T21","span":{"begin":299,"end":305},"obj":"http://purl.obolibrary.org/obo/GO_0098657"},{"id":"T22","span":{"begin":2477,"end":2488},"obj":"http://purl.obolibrary.org/obo/GO_0006412"},{"id":"T23","span":{"begin":5069,"end":5084},"obj":"http://purl.obolibrary.org/obo/GO_0006955"},{"id":"T24","span":{"begin":5283,"end":5298},"obj":"http://purl.obolibrary.org/obo/GO_0006955"},{"id":"T25","span":{"begin":5820,"end":5826},"obj":"http://purl.obolibrary.org/obo/GO_0098739"},{"id":"T26","span":{"begin":5820,"end":5826},"obj":"http://purl.obolibrary.org/obo/GO_0098657"},{"id":"T27","span":{"begin":6523,"end":6538},"obj":"http://purl.obolibrary.org/obo/GO_0006955"},{"id":"T28","span":{"begin":6772,"end":6781},"obj":"http://purl.obolibrary.org/obo/GO_0009058"},{"id":"T29","span":{"begin":7448,"end":7463},"obj":"http://purl.obolibrary.org/obo/GO_0016032"},{"id":"T30","span":{"begin":14474,"end":14489},"obj":"http://purl.obolibrary.org/obo/GO_0006955"},{"id":"T31","span":{"begin":14973,"end":14982},"obj":"http://purl.obolibrary.org/obo/GO_0023052"},{"id":"T32","span":{"begin":15126,"end":15141},"obj":"http://purl.obolibrary.org/obo/GO_0006955"},{"id":"T33","span":{"begin":15333,"end":15342},"obj":"http://purl.obolibrary.org/obo/GO_0023052"},{"id":"T34","span":{"begin":16911,"end":16924},"obj":"http://purl.obolibrary.org/obo/GO_0045087"},{"id":"T35","span":{"begin":16925,"end":16934},"obj":"http://purl.obolibrary.org/obo/GO_0023052"},{"id":"T36","span":{"begin":17906,"end":17922},"obj":"http://purl.obolibrary.org/obo/GO_0016032"},{"id":"T37","span":{"begin":18325,"end":18340},"obj":"http://purl.obolibrary.org/obo/GO_0006955"},{"id":"T38","span":{"begin":18984,"end":18999},"obj":"http://purl.obolibrary.org/obo/GO_0045087"},{"id":"T39","span":{"begin":19089,"end":19104},"obj":"http://purl.obolibrary.org/obo/GO_0006955"},{"id":"T40","span":{"begin":19293,"end":19308},"obj":"http://purl.obolibrary.org/obo/GO_0006955"},{"id":"T41","span":{"begin":20431,"end":20452},"obj":"http://purl.obolibrary.org/obo/GO_0070227"},{"id":"T42","span":{"begin":20443,"end":20452},"obj":"http://purl.obolibrary.org/obo/GO_0097194"},{"id":"T43","span":{"begin":20443,"end":20452},"obj":"http://purl.obolibrary.org/obo/GO_0006915"},{"id":"T44","span":{"begin":20605,"end":20617},"obj":"http://purl.obolibrary.org/obo/GO_0006954"},{"id":"T45","span":{"begin":20642,"end":20662},"obj":"http://purl.obolibrary.org/obo/GO_0070227"},{"id":"T46","span":{"begin":20653,"end":20662},"obj":"http://purl.obolibrary.org/obo/GO_0097194"},{"id":"T47","span":{"begin":20653,"end":20662},"obj":"http://purl.obolibrary.org/obo/GO_0006915"},{"id":"T48","span":{"begin":20931,"end":20939},"obj":"http://purl.obolibrary.org/obo/GO_0015297"},{"id":"T49","span":{"begin":21324,"end":21332},"obj":"http://purl.obolibrary.org/obo/GO_0007610"},{"id":"T50","span":{"begin":21509,"end":21517},"obj":"http://purl.obolibrary.org/obo/GO_0070265"},{"id":"T51","span":{"begin":21509,"end":21517},"obj":"http://purl.obolibrary.org/obo/GO_0019835"},{"id":"T52","span":{"begin":21509,"end":21517},"obj":"http://purl.obolibrary.org/obo/GO_0008219"},{"id":"T53","span":{"begin":21509,"end":21517},"obj":"http://purl.obolibrary.org/obo/GO_0001906"},{"id":"T54","span":{"begin":22568,"end":22585},"obj":"http://purl.obolibrary.org/obo/GO_0008015"},{"id":"T55","span":{"begin":23778,"end":23788},"obj":"http://purl.obolibrary.org/obo/GO_0008219"},{"id":"T56","span":{"begin":30522,"end":30528},"obj":"http://purl.obolibrary.org/obo/GO_0040007"},{"id":"T57","span":{"begin":30547,"end":30555},"obj":"http://purl.obolibrary.org/obo/GO_0015297"},{"id":"T58","span":{"begin":32072,"end":32078},"obj":"http://purl.obolibrary.org/obo/GO_0098739"},{"id":"T59","span":{"begin":32072,"end":32078},"obj":"http://purl.obolibrary.org/obo/GO_0098657"},{"id":"T60","span":{"begin":34546,"end":34552},"obj":"http://purl.obolibrary.org/obo/GO_0098739"},{"id":"T61","span":{"begin":34546,"end":34552},"obj":"http://purl.obolibrary.org/obo/GO_0098657"},{"id":"T62","span":{"begin":34603,"end":34618},"obj":"http://purl.obolibrary.org/obo/GO_0051100"},{"id":"T63","span":{"begin":34703,"end":34726},"obj":"http://purl.obolibrary.org/obo/GO_0019048"}],"text":"Nanotechnology Tools to Inactivate SARS-CoV-2 in Patients\nThe main target of SARS-CoV-2 is the respiratory tract (upper airways, lung),36 although other organs might also be infected (e.g., gut, kidney) and vasculature21 also appears to be a prime target. The expression of ACE2 probably determines uptake by different tissues.37\nIn addition to discussing immune-based approaches, because the lung is the most critically affected organ, we will center our discussion on the various options to inactivate the virus in the deep lung and to target the essential host cells for drug delivery. The virus reaches the alveoli and enters alveolar epithelial type II cells (AECII), due to the relatively high abundance of ACE2 and a permissive cellular milieu. These cells serve as a reservoir of the virus, which finally spreads throughout the lung, leading to the lung function impairment seen in severe cases. Airborne nanomaterials are optimally suited to penetrate into the deep lung due to the physicochemical properties of such aerosols, existing on the same size scale particles that penetrate most readily to the deep airways. Hence, nanomedicine is already actively pursuing ideas to deliver drugs, therapeutic proteins, and mRNAs by exploiting nanodevices for pulmonary delivery.38,39\nMoreover, the rapid emergence of SARS-CoV-2 has exposed one of the main weaknesses in the current medical landscape: the lack of broad-spectrum antiviral drugs. At present, there are only a handful of approved antivirals, and they are mostly virus-specific. Hence, when a new virus emerges, little can be done pharmacologically to slow down its spread. Some research efforts have been focused on the development of broad-spectrum drugs, which could potentially offer some efficacy against future emerging viruses (and maybe SARS-CoV-2). The various approaches developed over the years are mainly based on the creation of entry inhibitors.40,41 A highly conserved part in viruses is the attachment ligand (VAL). In most known respiratory viruses,42 the VAL targets either heparan sulfate proteoglycans (HSPG)43 or sialic acids (SA).44 Both HSPG and SA mimics have shown in vitro ability to bind to viruses, blocking their interaction with cell membranes, and often in a broad-spectrum way.45−47\nIn the context of nanomedicine, many nanomaterials have been developed, ranging from polymers48 to dendrimers,49 oligomers, NPs,50 liposomes,51 and small molecules.52 However, successful clinical translation has been hindered by the fact that, upon dilution, these compounds lose efficacy as the virus-compound complex dissociates leaving viruses free to restart their replication cycle. Recently, it has been shown that this limitation can be overcome by synthesizing NPs that, after binding, are able to inhibit viral infectivity irreversibly by permanently damaging the virion, refueling the hope for a true, broad-spectrum antiviral drug.53 Because the focus is also on the development of a drug specific to SARS-CoV-2, a good entry inhibitor could be based on blocking the S spike protein interaction with the cellular ACE2 receptor.19,21−23 Regardless of the specific approach, it is imperative that novel, effective antivirals be based on compounds that exhibit very low or negligible toxicity profiles, as patients will most likely need to receive those drugs for extended periods of time and will already be weakened. For these reasons, when designing antiviral drugs, clearance mechanisms have to be kept in mind. An example of this process is the recent redesign of broad-spectrum antiviral NPs into equally effective modified cyclodextrins.54 Moreover, nanotechnology may offer nanotheranostic approaches to fill the existing gap between diagnostics and therapy.55−57 The simultaneous management of both diagnostics and therapy for those suffering from COVID-19 or in future pandemics, as for many other diseases, is an additional potential strategy to take into consideration in which nanomaterials have proven to be effective tools. The advantages of the capabilities of nanotechnology and nanomaterials for combined therapeutics and diagnostics has been widely explored in cancer research; however, there have been considerable efforts in the past few years to extend the scope of this approach to other areas including infectious diseases.58\n\nNanomaterial-Based Vaccine Development and Immunomodulation\nFollowing the publication of the genetic sequence of SARS-CoV-2 on January 11, 2020, intense research efforts have been devoted to developing a vaccine against COVID-19. With unprecedented speed, this extraordinary scientific mobilization led the first vaccine candidate to enter the Phase I human clinical trial on March 16, 2020, and other novel candidates are rapidly following.59 Up to May 22, 2020, there are 10 COVID-19 candidate vaccines in clinical evaluations and 114 in preclinical development.60\nConcerning vaccine and immunization research, nanomaterials can assist in multiple ways to boost the upregulation required by the immune system and to direct the immune response specifically against antigens. Immune-targeted nanotherapeutics can be developed through their rational manufacture at the nanoscale level by designing nanomaterials that are able to amplify host’s immune response, for instance as adjuvants in the context of vaccination.\nThe development of a vaccine will rely either on the direct administration of viral antigens (e.g., in the form of recombinant proteins, vectored vaccines, or whole inactivated or attenuated virus) or RNA- or DNA-encoding viral antigens.27 Candidate antigens for immunization are surface proteins such as the immunogenic spike protein (S1), which is already targeted by antibodies of convalescent patients.27 Because the S1 protein is also essential for cellular uptake, many researchers are using this protein as the primary target for a vaccine.\nThere are many issues related to the delivery of a drug, protein, or RNA into the patient as the cargo is often degraded, not bioavailable, or is swiftly cleared. Nanotechnology provides multiple solutions to these challenges though, as nanocarriers can overcome some of these limitations.\nBiocompatible polymeric-, lipid-based, or inorganic NPs can be tuned with respect to their physicochemical properties to encapsulate cargo proteins with high loading efficiency, improving protein delivery and pharmacokinetics over conventional approaches.38 Intranasal delivery of polymer-encapsulated antigen triggers a strong immune response, and the success of vaccination depends on the appropriate type of polymer in combination with the antigen.61,62 Similarly, researchers have developed lipid and lipid-based NPs as delivery platforms for mRNAs or siRNAs to enable the synthesis of key viral proteins for vaccination or to inactivate critical viral target genes, respectively.39 The field of therapeutic mRNAs to support vaccination has gained momentum, as well,63 at least in part due to nanotechnology-enabled strategies for cargo delivery. Moreover, the versatility of nanoplatforms as antigen-presenting systems offers great opportunities. Considering the possibility of random viral mutations that may alter the antigen shape, functionalizing nanomaterials with a wide number of molecules at the same time to target the virus, or motifs that are specific for pathogens, will increase the efficiency of vaccines and their ability to prevent viral infection.\nSeveral companies are working on mRNA vaccines encoding SARS-CoV-2 proteins such as the spike protein, encapsulated in nanoliposomes with specific physicochemical properties that are potentially akin to those documented for immunization against certain tumor antigens.64 The design of such nanocarriers, which will need to escape recognition by scavenger cells and to be nontoxic and nonimmunogenic, is a challenge that will require substantial time prior to clinical availability.\nOn March 16, 2020, Moderna, through a partnership with the Vaccine Research Center at the U.S. National Institutes of Health, enrolled the first participants into a Phase I clinical trial testing an mRNA vaccine (mRNA-1273) encapsulated in lipid NPs—a record time of just 63 days following sequence selection (NCT04283461).65 The enrollment of the first cohort of participants (18 to 55-year-old healthy subjects) concluded on April 16, 2020.66 CureVac and BioNTech (in partnership with Pfizer) are currently working on similar vaccines; Pfizer/BioNTech, in particular, have recently started the recruitment in Phase I/II trials (NCT04368728, NCT04380701). A DNA plasmid vaccine by Inovio Pharmaceuticals (INO-4800) has showed promising results in mice and guinea pigs according to a recent article published in Nature Communications(67) and has entered Phase I testing in humans (NCT04336410).\nAnother candidate COVID-19 vaccine for Phase I clinical trial is from the University of Oxford and AstraZeneca (NCT04324606).68 Around 1110 people will take part in the trial, which started recruitment at the end of April 2020. The vaccine is based on a chimpanzee adenovirus vaccine vector (ChAdOx1) and the SARS-CoV-2 spike protein. Chimpanzee adenoviral vectors against different pathogens have been already tested in thousands of subjects with demonstrated safety. To date, ChAdOx1 has been administered to six rhesus macaques exposed to high doses of SARS-CoV-2. The vaccine was unable to prevent infections, although it reduced the severity of the disease: no signs of virus replication were observed in the lungs, with significantly lower levels of respiratory disease and no lung damage compared to control animals, according to a recent article deposited in bioRxiv.69 Another adenoviral vector vaccine developed by CanSino Biological Inc. and Beijing Institute of Biotechnology using a genetically engineered replication-defective adenovirus type 5 vector to express the SARS-CoV-2 spike protein (Ad5-nCoV) is currently being tested in Phase I/II trials (NCT04398147, NCT04341389, NCT0431312).\nAlthough vaccines based on novel DNA and mRNA technologies might be promising, there are no such kinds of vaccines on the market, and it is unknown whether they can be effective in humans. Moderna, for instance, has generated preliminary safety data on different mRNA-based vaccines targeting other respiratory viruses, but their more advanced program (on a cytomegalovirus vaccine) is still in Phase II clinical testing. However, on May 18, Moderna announced that mRNA-1273 elicited antibody titers above the levels observed in convalescent individuals (and therefore considered potentially protective) in all eight initial participants across the 25 and 100 μg dose cohorts of the Phase I trial (NCT04283461).70 The company, after having received a fast-track approval from the FDA, is now moving on Phase II trials.\nConversely, strong evidence exists regarding the efficacy of protein-based vaccines such as recombinant-protein, viral-vector, attenuated, or inactivated vaccines across different infectious diseases, with licensed vaccines already existing for all of these platforms.27 All of the aforementioned approaches are currently being explored in the context of SARS-CoV-2.27 An inactivated vaccine developed by the China National Pharmaceutical Group (Sinopharm), in collaboration with the Wuhan Institute of Biological Products, is currently tested in a Phase I/II trial (ChiCTR2000031809), and a second inactivated vaccine (in collaboration with the Beijing Institute of Biological Products) has been currently approved for clinical testing (ChiCTR2000032459). A third aluminum salt (alum) adjuvanted inactivated vaccine, developed by Beijing-based Sinovac Biotech’s, was able to provide partial or complete protection in rhesus macaques, according to a recent article published in Science,71 and is currently being tested in Phase I/II trials (NCT04383574, NCT04352608).\nLive-attenuated vaccines are intrinsically immunogenic (e.g., due to the presence of viral DNA), but extensive safety tests are required due to the rare possibility of reversion to a pathogenic form able to cause infection. Vaccine candidates based in this application have previously been designed for SARS-CoV with high stability.72,73 Recombinant-protein vaccines and inactivated vaccines are safer but might require adjuvants to increase their immunogenicity. In the context of SARS-CoV-2, adjuvants are important for two reasons. First, adjuvants might increase the efficacy of the vaccine, especially in subjects with impaired immunological function, such as the elderly, or in subjects with comorbidities resulting in immune dysfunctions; in these patient cohorts, SARS-CoV-2 has a high lethality rate. Second, adjuvants can reduce the amount of vaccine protein(s) required per dose, which could facilitate scaling-up vaccine production in a reduced time frame.\nBeyond alum, which is in fact a nanoscale material74 that was developed in the 1920s for the tetanus and diphtheria toxoids,75 approval for a new adjuvant did not occur until 1997, with the introduction of the oil-in-water emulsion of squalene oil and polysorbate 80 and sorbitan trioleate surfactants (MF59) in the seasonal influenza vaccine for the elderly.76 The use of MF59 was further expanded to pandemic and avian influenza vaccines.77 Other adjuvants in licensed vaccines have been approved since 2000, as well: (a) AS03 (used for pandemic and avian influenza vaccines), similar to MF59, but including α-tocopherol as an additional immune stimulant; (b) AF 03 (used for pandemic influenza vaccines), an alternative squalene emulsion containing polyoxyethylene, cetostearyl ether, mannitol, and orbitan oleate;78 (c) AS 01 (used for herpes zoster vaccine), a liposome-based vaccine adjuvant system containing two immunostimulants, 3-O-desacyl-4′-monophosphoryl lipid A (MPL, a Toll-like receptor 4 agonist) and saponin QS-21, which activates the ACT-NLRP3 inflammasome pathway;79 and (d) AS04 (used for hepatitis B and human papilloma virus vaccines), which is a combination of MPL and aluminum hydroxide.76,80\nIn this context, the concept of “nanoimmunity by design” relies on the rational design of distinct physicochemical properties and specific functionalization of nanomaterials intended for fine-tuning their potential effects on the immune system.81 Nanomaterials have emerged as promising tools for immune modulation, either stimulating or suppressing the immune response. In fighting SARS-CoV-2, these properties may find applications for both prevention and therapy and in the context of vaccine development.\nMounting evidence indicates that nanomaterials such as graphene,82 nanodiamonds,83 carbon nanotubes,84 and polystyrene particles85 bear an intrinsic capacity to activate the immune system, depending on their functionalization.86 For instance, graphene oxide functionalized with amino groups (GO-NH2) induces activation of STAT1/IRF1 interferon signaling in monocytes and T cells, resulting in the production of T cell chemoattractants, and macrophage 1 (M1) 1/T-helper 1 (Th1) polarization of the immune response, with negligible toxicity.82 Remarkably, the ability of licensed adjuvants such as AS01 and AS03 to enhance adaptive immunity has been linked to their capacity to boost STAT1/IRF1 interferon signaling.87 In addition, recent SARS-CoV and MERS-CoV studies suggest that the development of a Th1-type response is central for controlling infection, which also may be true for SARS-CoV-2.88\nSeveral groups and consortia have been screening and characterizing nanomaterials according to their immunomodulatory properties and absence of cytotoxicity.56,57,82−84,86 The development of an adjuvant for clinical use is a lengthy process that requires extensive Phase III randomized trials in large and diverse cohorts of subjects, and the process generally requires several years.76 Pharmaceutical giants such as GlaxoSmithKline (GSK), which owns the ASs mentioned above and other adjuvant platforms, are engaged in several partnerships to embed their adjuvant systems with SARS-CoV-2-protein-based vaccines. A full-length recombinant SARS-CoV-2 glycoprotein nanoparticle vaccine adjuvanted with the saponin-based Matrix M developed by Novavax is in Phase I clinical testing (NCT04368988).89\nAlthough it is unlikely that novel adjuvants would be used in the context of the current pandemic, the SARS-CoV-2 pandemic offers an opportunity to reflect on the potential of nanotechnology for vaccine adjuvant development. In this context, it is critical to stream coherent pipelines covering in vitro and in vivo experiments specifically to select candidate materials that might be tested for clinical implementation as vaccine adjuvants.90\nIn this view, the status of nanomaterial-based vaccine adjuvants has been reviewed.91,92 In particular, immunomodulatory effects induced on the innate immune signaling have been demonstrated.91 For example, nanomaterials such as GO can elicit an inflammasome sensor (NLRP3)-dependent expression of IL-1β in macrophages.93 Notably, alum, the most commonly used adjuvant in human vaccines, induces the release of this cytokine in macrophages through the same NRLP-induced mechanism. These results suggest that nanomaterials such as GO and alum may be useful for medical applications.94\nConsidering that new vaccine development typically requires years to be approved and applied to the general population, there is also an urgent need to study how to improve treatment approaches. According to the first Phase III clinical studies, the antiviral drug Remdesivir, which was recently approved by the FDA for COVID-19 treatment in the United States, seems to be a promising treatment for adults diagnosed with COVID-19. Nano-based strategies have already been applied to enhance the effectiveness of Remdesivir in the context of other emerging viral infections (Nipah virus),95 suggesting the suitability of nanotechnology to assist with similar strategies for the treatment of COVID-19 as well as other possible pandemics in the future.96\n\nCan Nanotechnology Help to Control the Cytokine Storm?\nOne of the main features of COVID-19 is the triggering of a cytokine storm in the body, also known as cytokine release syndrome (CRS), which results from an excessive immune response and leads to the severe deterioration of patient health.25,97,98 This inflammatory storm is one of the major causes of the acute respiratory distress syndrome (ARDS) that is often associated with multiple-organ failure, representing the leading causes of death in critical patients.98 In particular, the role of interleukin (IL)-6 has been highlighted in patients requiring assisted ventilation. Ongoing clinical trials are testing drugs that block the receptor of IL-6 (Tocilizumab, an anti-IL-6 receptor antibody, and Sarilumab) or IL-6 itself (Siltuximab).\nWhereas a well-regulated cytokine response that is rapidly triggered by the host’s innate immunity can serve to prevent and to counteract an infection, an excessive, unbalanced, prolonged immune response can seriously harm the body. Therefore, therapeutic strategies aimed at effectively suppressing the cytokine storm are under investigation. Nanomaterials have been exploited to adjust the immune response to an optimized level, and such proprieties might be explored to inhibit cytokine releases.99 Nanosystems can enhance the specificity/efficiency of immunosuppressant delivery to target immune cells, with consequent reductions in drug dose, drug distribution to nontarget tissues and organs, and possible side effects. In addition, specific nanotools can be designed to evade the immune system and to enhance the solubility of poorly soluble immunosuppressant agents; the potential of finely tuning their surface charge opens possibilities for encapsulation strategies and offers accommodation for a high drug load. All of these mechanisms may also occur simultaneously, enhancing the activity of immunosuppressive agents.\nConcerning the role of macrophages in COVID-19, the presence of ACE2-expressing CD68+CD169+ macrophages containing SARS-CoV-2 nucleoprotein antigen and showing an increased release of IL-6 was observed in infected spleen and lymph nodes.100 Notably, immunohistochemical and immunofluorescence analyses of lymph nodes and spleen tissue from autopsy samples of patients who died from COVID-19 revealed lymphocytic apoptosis. The tissues infected by SARS-CoV-2 also showed an upregulated expression of Fas, suggesting a role for CD169+ macrophages in viral spreading, aberrant inflammation, and activation-induced lymphocyte apoptosis. Moreover, histological examinations of biopsy samples of patients who died from COVID-19 revealed an increased alveolar exudate due to the extended neutrophil and monocyte infiltrate in lung capillaries with fibrin deposition, probably leading to difficulties in gas exchange. Through nanomedicine, we envision therapeutic approaches aimed at targeting specific immune subpopulations to avoid these complications, and different nanomaterials have already been explored for their specific impact on different immune cell subpopulations.82,83,86 Octadecylamine-functionalized and dexamethasone-adsorbed nanodiamond promotes anti-inflammatory and proregenerative behavior in human macrophages in vitro.101 A low dose of this functionalized nanodiamond also reduced macrophage infiltration and expression of proinflammatory mediators iNOS and tumor necrosis factor (TNF)-α in mice. Overall, these results suggest that nanodiamond particles may be useful as an inherently immunomodulatory platform.\nFinally, the indiscriminate systemic suppression of the immune system may increase the susceptibility to sepsis due to underlying secondary bacterial infections that can exacerbate critical conditions in patients, causing death.102 Risk factors for mortality include acquired bacterial infections by opportunistic organisms and primary pathogens. Using nanocarriers to achieve targeted delivery of immunosuppressive drugs, or the specific and controlled inhibition of only a specific immune cell subpopulation, can play a critical role in limiting such complications.\nThe fast and complete removal of pro-inflammatory cytokines from the bloodstream has been shown to increase survival significantly in patients with early stage sepsis. Adsorption of cytokines from serum by extracorporeal perfusion through porous carbons is one of the most promising ways for their selective, quick, and complete removal from blood circulation. Hierarchical carbon materials with tuned porosity have shown effective adsorption of many cytokines, including IL-6 and even the largest ones, such as TNF-α.103 Based on their demonstrated outstanding performance in removing sepsis-associated pro-inflammatory cytokines from blood, mesoporous carbon adsorbents with hierarchical structure, tuned pore size, and surface chemistry, as well as graphene with open and accessible surfaces104 have significant potential to quickly remove inflammatory cytokines associated with CRS and to prevent mortality arising from an uncontrolled inflammatory cascade, thereby providing enough time for the defense mechanisms of the human body to fight the virus.\n\nPhotodynamic Inactivation of SARS-CoV-2\nIn addition to drug- and vaccine-based antiviral strategies, photodynamic therapy (PDT) stands as a unique approach to inactivate SARS-CoV-2. Using a light-based method, PDT attacks target cells via the excitation of photosensitive agents, called photosensitizers (PSs), with radiation characterized by a wavelength corresponding to its absorption spectrum to generate reactive oxygen species (ROS) in the presence of oxygen, which ultimately results in cell death. Photodynamic therapy is primarily used for the clinical treatment of various oncological disorders.105 It was not until the 1970s that PDT was first used clinically against viruses,106 exploiting ROS production to damage virus proteins, nucleic acids, and—if present—lipids.107 Even though there are research efforts for PDT-based virotherapies against different viruses, including herpes simplex virus, human papilloma virus, and human immunodeficiency virus,106−109 clinical use of PDT is limited due to hydrophobicity of PSs, poor target specificity, and limited tissue penetration ability.\nMost PSs are hydrophobic and aggregate in aqueous solutions, affecting their photochemical and photobiological properties.106,110 For this reason, Lim et al. have proposed a promising approach for photodynamic inactivation of viruses with NPs, developing sodium yttrium fluoride (NaYF4) upconversion NPs (UCNs) with zinc phthalocyanine PSs grafted onto their surfaces. Unlike most PSs, these UCNs are coated with polyethylenimine (PEI), which render them hydrophilic and easier to manipulate. These UCNs showed antiviral activity against Dengue virus serotype 2 and adenovirus type 5, which were used as models of enveloped and non-enveloped viruses, respectively.110 MXenes111,112 are a large family of 2D transition metal carbides, nitrides,113 and carbonitrides114 that exhibit unique electronic, optical, and catalytic properties. They have the general formula Mn+1XnTx, where M is an early transition metal (Ti, Zr, V, Mo, etc.), X is C and/or N, Tx represents the surface functional groups (=O, −OH, −F, −Cl), and n = 1–4.115,116 Some examples include Ti3C2Tx, V2CTx, and Nb2CTx, with over 30 stoichiometric compositions already synthesized with more than 100 predicted. Biocompatible MXenes, such as Ti3C2Tx, are hydrophilic and are among the most efficient light-to-heat transforming materials.117 The plasmon resonance extinction maxima of Ti3C2Tx is at 780 nm, enabling the use of near-infrared (IR) light for PDT. Several other MXenes have absorption maxima in the IR range and have shown outstanding performance in PDT and theranostic applications.118\nFullerene and graphene are also good candidates for virus inactivation by PDT and have proved to be effective against Semliki Forest virus (SFV), vesicular stomatitis virus (VSV), HSV-1, HIV-1, mosquito iridovirus (MIV), and influenza A virus (IAV), as well as the phage MS2.107 In addition, several 2D nanomaterials, including graphene-based materials, MXenes, black phosphorus, graphitic carbon nitride, tungsten disulfide, and molybdenum disulfide, have been reported to improve the efficacy of PDT considerably for cancer treatment.56,57 Therefore, determining if such nanomaterial-based PDT protocols could be exploited to inactivate SARS-CoV-2 is of great interest.\n\nBiomimetic Engineering of Nanodelivery Systems: Artificial Viruses in the Making\nIn an effort to engineer the next generation of nanoscale vectors, scientists have moved from using inorganic components aimed at obtaining inert structures to utilizing biological building blocks that are able to convey additional functionalities to the resulting construct. To cope with the complexity of the body and to evade the multiple layers of defense that tissues and organs have, it is critical to rely on the ability of certain materials to interact with, rather than to eschew, the biology of our body. Every NP system conceived to date faces one common fate: whether injected, inhaled, ingested, or absorbed through our epithelia, all will at some point come into contact with the mixture of fluids and organic compounds that comprise the body. Under such conditions, every material reacts in a unique way according to the conditions they individually face (i.e., the tissue or body region they are in), their composition (i.e., organic or inorganic), and their physical properties (i.e., size, shape, surface charge).119 Inorganic NPs can function as globular protein mimics because of their similar size, charge, shape, and surface features that can be chemically functionalized to resemble proteins. These similarities can be used in biotechnology to control virus pathways or cell receptor interactions with NPs.120\nAmong the many attempts, the legendary accomplishments, and the epic failures, we could list thousands of different NPs, differing from one another thanks to the creative endeavors of their designers. Despite their remarkable differences and researchers’ endeavors to make them one of a kind, they all aim to achieve one goal: to deliver in a specific way one particular form of payload, while remaining as unnoticed as possible by the body’s defense mechanisms. For everyone who has made an effort to create their own version of such a silver bullet, it has come to mind that nature in its incredible variety had already invented a few ingenious solutions to this problem. In the world of nano-based drug delivery, one entity dominates as the quintessential example of precision, efficiency, and stealth: the virus. Not by chance, these two worlds share many features that span from the physical laws that govern their assembly and stability to the chemical similarities in their overall composition.121\nHistorically, the field of nanomedicine and the global endeavor to generate NPs for drug delivery arose from one of the greatest struggles in medicine: gene therapy. At the core of that approach was the idea that viruses could be used as Trojan horses to deliver the correct sequence of the gene into the cells that harbored the mutated copy. In order to identify the virus that offered the best delivery service, a cadre of viral strains and species were tested, adapted, and engineered to fit the aims and hit the targets. It took decades of trial and error, of progressive adjustments, and a few tragic mistakes to identify the viruses that could provide the ideal backbone to develop viral vectors able to ferry genetic cargo into the target cell.122 In the midst of that global challenge, nanotechnology offered a safer and more controllable alternative: to generate bespoke structures that could replace viral vectors and do the same job, delivering a payload from the point of injection to the site of action.\nFast forward a few decades, and the promises made by both worlds seem to have finally become reality, with a number of active clinical trials, some clinical success stories, and a few commercial products in the market space. Interestingly, the two worlds seem to have maintained a safe distance so as to avoid any collision or dangerous proximity. Exchange of ideas between the fields of gene therapy and nanomedicine is limited, and the potential for disciplinary growth through knowledge exchange has remained elusive to scientists in both fields.5 Many scientists have suggested that nanotechnologists could find inspiration in the mechanisms devised by viruses to elude immune surveillance, to overcome biological barriers, and to deliver their genetic payload with high specificity. Similarly, gene therapists would find high-tech solutions to their scalability and safety issues in looking at the new generations of biomimetic particles being generated.\nThe principles of biomimicry and bioinspiration have been used to design and to engineer drug-delivery technologies that reproduce or recapitulate biological materials, for what pertains to not only their structure and chemistry but also, more importantly, their functions. In drug delivery, surface recognition and nanoscale interactions between materials and biological entities are key to the success of the delivery strategy, and the use of biological building blocks such as membrane proteins has been proposed as a way to convey targeting and shielding moieties simultaneously.123 One can only hope that the recent events and global attention that viruses are capturing in the scientific world will spur a renewed interest in finding ways to adapt viral features and mechanisms of action to the world of NPs. Increased focus in this field would be useful to create virus-like NPs able to circulate in the blood system, overcoming the endothelial barrier, and to deliver their therapeutic payload with high efficiency.124\n\nInterference with Cellular Uptake, Immobilization, and Inactivation of the Virus Outside of the Host Cell\nNanomaterials can be synthesized with a high specific surface area of a few hundred square meters per gram. Therefore, dependent on the surface properties, nanomaterials efficiently adsorb biomolecules and form a so-called biomolecular corona. This passive, nontargeted adsorption might be utilized to bind viruses, provided that the selected nanomaterial is relatively biocompatible. Viral surface proteins are often modified by sugar moieties or encompass positively charged amino acid patches that bind to lectins or glycosaminoglycans (GAGs) of heparan sulfate (HS), respectively.32 Robust interactions of virus particles with these host receptors is ensured by multivalent binding, which is likely why single-molecule inhibitors often are not capable of efficiently perturbing this key event but multivalent NPs are superior to block binding of different viruses to the host cell.125\nGold NPs capped with mercaptoethanesulfonate are effective inhibitors of HSV type 1 infection as they mimic cell-surface-receptor heparan sulfate and, therefore, competitively bind to the virus. Interestingly, polyvalent sulfated Au NPs inhibit virus binding to the host cell dependent on their size. Nanoparticles of diameters equal to and larger than the virus diameter (in this case, the stomatitis virus) more efficiently inhibit the binding to cells than smaller particles. Most likely, larger NPs efficiently cross-link virions, whereas smaller NPs simply decorate the viral surface.126 Papp et al. found that gold NPs decorated with SA effectively inhibited the binding of influenza virus to the target cells. In this case, viral recognition via its surface protein hemagglutinin of SA on the host cell membrane was a prerequisite for cellular entry.127 More recently, Cagno et al. reported antiviral NPs (Au and iron oxide core) with long and flexible linkers mimicking HS that strongly bind and inactivate viruses such as respiratory syncytial virus in vitro and in vivo in a lung infection model and even led to irreversible viral deformation.53 Hence, there is ample evidence that biocompatible, functionalized NPs can act as broad-spectrum antivirals. Notably, the receptor-binding domain of the spike S1 protein of SARS-CoV-2 binds not only to ACE2 but also to heparin128 and, thus, might be targeted by similar approaches as outlined above.\nOne of the most recent strategies to inhibit viral uptake is based on administration of recombinant ACE2 to inhibit binding competitively via the spike S1 protein.21 Knowing that multivalency is key to block virus–host interactions reliably, researchers have speculated that a nanostructured carrier could not only improve delivery and cargo stability but also might dramatically enhance binding strength.129\n\nSpeeding up the Nanomedicine-Based Approaches for COVID-19 by In Silico Analysis\nCurrently, repurposing drug molecules is a key strategy for identifying approved or investigational drugs outside the scope of the original medical indication that can be used to fight COVID-19.130,131 There are various advantages to this strategy, including already-established safety profiles, fast transition to clinical studies, and less investment needed, compared to the process of developing an entirely new drug.132,133 Therefore, these advantages have the potential to result in less risky and more rapid returns on investment in the development of repurposed drugs, benefits that are particularly important during pandemics. In addition to rapid diagnosis, making recommendations to physicians about rapid treatment methods can save the lives of many people. Combining in silico tools such as molecular docking, molecular dynamics, and computational chemistry with large drug databases provides a great advantage in selecting “possible candidates” from among thousands of pharmaceutically active substances (Figure 3). In silico analyses should be supported by the literature, expert opinions (pharmacologists and clinicians), and, when possible, preclinical and clinical findings. After selecting the best candidates following in silico analyses, detailed in vitro and in vivo experimentation should be undertaken prior to clinical studies. Recently, various drug-repurposing studies have been published to assist in the global COVID-19 pandemic response.134−136 Such studies aim to identify whether already-approved drugs have the capacity to interact with viral proteins or receptors on host cells.\nFigure 3 Schematic of how in silico analysis can be used to select candidate drug molecules for clinical studies. MD, molecular dynamics. In nanomedicine, computational models have recently garnered attention; such work may help to identify how nanomaterials interact with biological systems and to determine how the efficacy of these nanotherapeutics could be improved. Computational models indicate how NPs are taken up by healthy cells or tumor cells, enabling better predictions regarding the pharmacokinetic and pharmacodynamic properties of these materials.134 For example, Lunnoo et al. used a coarse-grained molecular dynamics (MD) simulation to observe the internalization pathways of various Au nanostructures (nanospheres, nanocages, nanorods, nanoplates, and nanohexapods) into an idealized mammalian plasma membrane.137 Other studies have simulated how different NPs can target tumor cells and deliver drugs.138,139 Therefore, in silico approaches that are currently used for drug repurposing, including molecular docking, molecular dynamics, and computational chemistry, constitute valuable tools to aid preclinical and clinical studies of nanomaterials directed for disease treatment. Considering the urgent need for nanomedicine against the current pandemic, in silico analyses may be especially useful in guiding the rational design of new NP formulations required to fight SARS-CoV-2."}
LitCovid-PD-GlycoEpitope
{"project":"LitCovid-PD-GlycoEpitope","denotations":[{"id":"T1","span":{"begin":2058,"end":2073},"obj":"GlycoEpitope"},{"id":"T2","span":{"begin":32700,"end":32715},"obj":"GlycoEpitope"},{"id":"T3","span":{"begin":32717,"end":32719},"obj":"GlycoEpitope"},{"id":"T4","span":{"begin":33170,"end":33185},"obj":"GlycoEpitope"},{"id":"T5","span":{"begin":34018,"end":34020},"obj":"GlycoEpitope"}],"attributes":[{"id":"A1","pred":"glyco_epitope_db_id","subj":"T1","obj":"http://www.glycoepitope.jp/epitopes/EP0086"},{"id":"A2","pred":"glyco_epitope_db_id","subj":"T2","obj":"http://www.glycoepitope.jp/epitopes/EP0086"},{"id":"A3","pred":"glyco_epitope_db_id","subj":"T3","obj":"http://www.glycoepitope.jp/epitopes/EP0086"},{"id":"A4","pred":"glyco_epitope_db_id","subj":"T4","obj":"http://www.glycoepitope.jp/epitopes/EP0086"},{"id":"A5","pred":"glyco_epitope_db_id","subj":"T5","obj":"http://www.glycoepitope.jp/epitopes/EP0086"}],"text":"Nanotechnology Tools to Inactivate SARS-CoV-2 in Patients\nThe main target of SARS-CoV-2 is the respiratory tract (upper airways, lung),36 although other organs might also be infected (e.g., gut, kidney) and vasculature21 also appears to be a prime target. The expression of ACE2 probably determines uptake by different tissues.37\nIn addition to discussing immune-based approaches, because the lung is the most critically affected organ, we will center our discussion on the various options to inactivate the virus in the deep lung and to target the essential host cells for drug delivery. The virus reaches the alveoli and enters alveolar epithelial type II cells (AECII), due to the relatively high abundance of ACE2 and a permissive cellular milieu. These cells serve as a reservoir of the virus, which finally spreads throughout the lung, leading to the lung function impairment seen in severe cases. Airborne nanomaterials are optimally suited to penetrate into the deep lung due to the physicochemical properties of such aerosols, existing on the same size scale particles that penetrate most readily to the deep airways. Hence, nanomedicine is already actively pursuing ideas to deliver drugs, therapeutic proteins, and mRNAs by exploiting nanodevices for pulmonary delivery.38,39\nMoreover, the rapid emergence of SARS-CoV-2 has exposed one of the main weaknesses in the current medical landscape: the lack of broad-spectrum antiviral drugs. At present, there are only a handful of approved antivirals, and they are mostly virus-specific. Hence, when a new virus emerges, little can be done pharmacologically to slow down its spread. Some research efforts have been focused on the development of broad-spectrum drugs, which could potentially offer some efficacy against future emerging viruses (and maybe SARS-CoV-2). The various approaches developed over the years are mainly based on the creation of entry inhibitors.40,41 A highly conserved part in viruses is the attachment ligand (VAL). In most known respiratory viruses,42 the VAL targets either heparan sulfate proteoglycans (HSPG)43 or sialic acids (SA).44 Both HSPG and SA mimics have shown in vitro ability to bind to viruses, blocking their interaction with cell membranes, and often in a broad-spectrum way.45−47\nIn the context of nanomedicine, many nanomaterials have been developed, ranging from polymers48 to dendrimers,49 oligomers, NPs,50 liposomes,51 and small molecules.52 However, successful clinical translation has been hindered by the fact that, upon dilution, these compounds lose efficacy as the virus-compound complex dissociates leaving viruses free to restart their replication cycle. Recently, it has been shown that this limitation can be overcome by synthesizing NPs that, after binding, are able to inhibit viral infectivity irreversibly by permanently damaging the virion, refueling the hope for a true, broad-spectrum antiviral drug.53 Because the focus is also on the development of a drug specific to SARS-CoV-2, a good entry inhibitor could be based on blocking the S spike protein interaction with the cellular ACE2 receptor.19,21−23 Regardless of the specific approach, it is imperative that novel, effective antivirals be based on compounds that exhibit very low or negligible toxicity profiles, as patients will most likely need to receive those drugs for extended periods of time and will already be weakened. For these reasons, when designing antiviral drugs, clearance mechanisms have to be kept in mind. An example of this process is the recent redesign of broad-spectrum antiviral NPs into equally effective modified cyclodextrins.54 Moreover, nanotechnology may offer nanotheranostic approaches to fill the existing gap between diagnostics and therapy.55−57 The simultaneous management of both diagnostics and therapy for those suffering from COVID-19 or in future pandemics, as for many other diseases, is an additional potential strategy to take into consideration in which nanomaterials have proven to be effective tools. The advantages of the capabilities of nanotechnology and nanomaterials for combined therapeutics and diagnostics has been widely explored in cancer research; however, there have been considerable efforts in the past few years to extend the scope of this approach to other areas including infectious diseases.58\n\nNanomaterial-Based Vaccine Development and Immunomodulation\nFollowing the publication of the genetic sequence of SARS-CoV-2 on January 11, 2020, intense research efforts have been devoted to developing a vaccine against COVID-19. With unprecedented speed, this extraordinary scientific mobilization led the first vaccine candidate to enter the Phase I human clinical trial on March 16, 2020, and other novel candidates are rapidly following.59 Up to May 22, 2020, there are 10 COVID-19 candidate vaccines in clinical evaluations and 114 in preclinical development.60\nConcerning vaccine and immunization research, nanomaterials can assist in multiple ways to boost the upregulation required by the immune system and to direct the immune response specifically against antigens. Immune-targeted nanotherapeutics can be developed through their rational manufacture at the nanoscale level by designing nanomaterials that are able to amplify host’s immune response, for instance as adjuvants in the context of vaccination.\nThe development of a vaccine will rely either on the direct administration of viral antigens (e.g., in the form of recombinant proteins, vectored vaccines, or whole inactivated or attenuated virus) or RNA- or DNA-encoding viral antigens.27 Candidate antigens for immunization are surface proteins such as the immunogenic spike protein (S1), which is already targeted by antibodies of convalescent patients.27 Because the S1 protein is also essential for cellular uptake, many researchers are using this protein as the primary target for a vaccine.\nThere are many issues related to the delivery of a drug, protein, or RNA into the patient as the cargo is often degraded, not bioavailable, or is swiftly cleared. Nanotechnology provides multiple solutions to these challenges though, as nanocarriers can overcome some of these limitations.\nBiocompatible polymeric-, lipid-based, or inorganic NPs can be tuned with respect to their physicochemical properties to encapsulate cargo proteins with high loading efficiency, improving protein delivery and pharmacokinetics over conventional approaches.38 Intranasal delivery of polymer-encapsulated antigen triggers a strong immune response, and the success of vaccination depends on the appropriate type of polymer in combination with the antigen.61,62 Similarly, researchers have developed lipid and lipid-based NPs as delivery platforms for mRNAs or siRNAs to enable the synthesis of key viral proteins for vaccination or to inactivate critical viral target genes, respectively.39 The field of therapeutic mRNAs to support vaccination has gained momentum, as well,63 at least in part due to nanotechnology-enabled strategies for cargo delivery. Moreover, the versatility of nanoplatforms as antigen-presenting systems offers great opportunities. Considering the possibility of random viral mutations that may alter the antigen shape, functionalizing nanomaterials with a wide number of molecules at the same time to target the virus, or motifs that are specific for pathogens, will increase the efficiency of vaccines and their ability to prevent viral infection.\nSeveral companies are working on mRNA vaccines encoding SARS-CoV-2 proteins such as the spike protein, encapsulated in nanoliposomes with specific physicochemical properties that are potentially akin to those documented for immunization against certain tumor antigens.64 The design of such nanocarriers, which will need to escape recognition by scavenger cells and to be nontoxic and nonimmunogenic, is a challenge that will require substantial time prior to clinical availability.\nOn March 16, 2020, Moderna, through a partnership with the Vaccine Research Center at the U.S. National Institutes of Health, enrolled the first participants into a Phase I clinical trial testing an mRNA vaccine (mRNA-1273) encapsulated in lipid NPs—a record time of just 63 days following sequence selection (NCT04283461).65 The enrollment of the first cohort of participants (18 to 55-year-old healthy subjects) concluded on April 16, 2020.66 CureVac and BioNTech (in partnership with Pfizer) are currently working on similar vaccines; Pfizer/BioNTech, in particular, have recently started the recruitment in Phase I/II trials (NCT04368728, NCT04380701). A DNA plasmid vaccine by Inovio Pharmaceuticals (INO-4800) has showed promising results in mice and guinea pigs according to a recent article published in Nature Communications(67) and has entered Phase I testing in humans (NCT04336410).\nAnother candidate COVID-19 vaccine for Phase I clinical trial is from the University of Oxford and AstraZeneca (NCT04324606).68 Around 1110 people will take part in the trial, which started recruitment at the end of April 2020. The vaccine is based on a chimpanzee adenovirus vaccine vector (ChAdOx1) and the SARS-CoV-2 spike protein. Chimpanzee adenoviral vectors against different pathogens have been already tested in thousands of subjects with demonstrated safety. To date, ChAdOx1 has been administered to six rhesus macaques exposed to high doses of SARS-CoV-2. The vaccine was unable to prevent infections, although it reduced the severity of the disease: no signs of virus replication were observed in the lungs, with significantly lower levels of respiratory disease and no lung damage compared to control animals, according to a recent article deposited in bioRxiv.69 Another adenoviral vector vaccine developed by CanSino Biological Inc. and Beijing Institute of Biotechnology using a genetically engineered replication-defective adenovirus type 5 vector to express the SARS-CoV-2 spike protein (Ad5-nCoV) is currently being tested in Phase I/II trials (NCT04398147, NCT04341389, NCT0431312).\nAlthough vaccines based on novel DNA and mRNA technologies might be promising, there are no such kinds of vaccines on the market, and it is unknown whether they can be effective in humans. Moderna, for instance, has generated preliminary safety data on different mRNA-based vaccines targeting other respiratory viruses, but their more advanced program (on a cytomegalovirus vaccine) is still in Phase II clinical testing. However, on May 18, Moderna announced that mRNA-1273 elicited antibody titers above the levels observed in convalescent individuals (and therefore considered potentially protective) in all eight initial participants across the 25 and 100 μg dose cohorts of the Phase I trial (NCT04283461).70 The company, after having received a fast-track approval from the FDA, is now moving on Phase II trials.\nConversely, strong evidence exists regarding the efficacy of protein-based vaccines such as recombinant-protein, viral-vector, attenuated, or inactivated vaccines across different infectious diseases, with licensed vaccines already existing for all of these platforms.27 All of the aforementioned approaches are currently being explored in the context of SARS-CoV-2.27 An inactivated vaccine developed by the China National Pharmaceutical Group (Sinopharm), in collaboration with the Wuhan Institute of Biological Products, is currently tested in a Phase I/II trial (ChiCTR2000031809), and a second inactivated vaccine (in collaboration with the Beijing Institute of Biological Products) has been currently approved for clinical testing (ChiCTR2000032459). A third aluminum salt (alum) adjuvanted inactivated vaccine, developed by Beijing-based Sinovac Biotech’s, was able to provide partial or complete protection in rhesus macaques, according to a recent article published in Science,71 and is currently being tested in Phase I/II trials (NCT04383574, NCT04352608).\nLive-attenuated vaccines are intrinsically immunogenic (e.g., due to the presence of viral DNA), but extensive safety tests are required due to the rare possibility of reversion to a pathogenic form able to cause infection. Vaccine candidates based in this application have previously been designed for SARS-CoV with high stability.72,73 Recombinant-protein vaccines and inactivated vaccines are safer but might require adjuvants to increase their immunogenicity. In the context of SARS-CoV-2, adjuvants are important for two reasons. First, adjuvants might increase the efficacy of the vaccine, especially in subjects with impaired immunological function, such as the elderly, or in subjects with comorbidities resulting in immune dysfunctions; in these patient cohorts, SARS-CoV-2 has a high lethality rate. Second, adjuvants can reduce the amount of vaccine protein(s) required per dose, which could facilitate scaling-up vaccine production in a reduced time frame.\nBeyond alum, which is in fact a nanoscale material74 that was developed in the 1920s for the tetanus and diphtheria toxoids,75 approval for a new adjuvant did not occur until 1997, with the introduction of the oil-in-water emulsion of squalene oil and polysorbate 80 and sorbitan trioleate surfactants (MF59) in the seasonal influenza vaccine for the elderly.76 The use of MF59 was further expanded to pandemic and avian influenza vaccines.77 Other adjuvants in licensed vaccines have been approved since 2000, as well: (a) AS03 (used for pandemic and avian influenza vaccines), similar to MF59, but including α-tocopherol as an additional immune stimulant; (b) AF 03 (used for pandemic influenza vaccines), an alternative squalene emulsion containing polyoxyethylene, cetostearyl ether, mannitol, and orbitan oleate;78 (c) AS 01 (used for herpes zoster vaccine), a liposome-based vaccine adjuvant system containing two immunostimulants, 3-O-desacyl-4′-monophosphoryl lipid A (MPL, a Toll-like receptor 4 agonist) and saponin QS-21, which activates the ACT-NLRP3 inflammasome pathway;79 and (d) AS04 (used for hepatitis B and human papilloma virus vaccines), which is a combination of MPL and aluminum hydroxide.76,80\nIn this context, the concept of “nanoimmunity by design” relies on the rational design of distinct physicochemical properties and specific functionalization of nanomaterials intended for fine-tuning their potential effects on the immune system.81 Nanomaterials have emerged as promising tools for immune modulation, either stimulating or suppressing the immune response. In fighting SARS-CoV-2, these properties may find applications for both prevention and therapy and in the context of vaccine development.\nMounting evidence indicates that nanomaterials such as graphene,82 nanodiamonds,83 carbon nanotubes,84 and polystyrene particles85 bear an intrinsic capacity to activate the immune system, depending on their functionalization.86 For instance, graphene oxide functionalized with amino groups (GO-NH2) induces activation of STAT1/IRF1 interferon signaling in monocytes and T cells, resulting in the production of T cell chemoattractants, and macrophage 1 (M1) 1/T-helper 1 (Th1) polarization of the immune response, with negligible toxicity.82 Remarkably, the ability of licensed adjuvants such as AS01 and AS03 to enhance adaptive immunity has been linked to their capacity to boost STAT1/IRF1 interferon signaling.87 In addition, recent SARS-CoV and MERS-CoV studies suggest that the development of a Th1-type response is central for controlling infection, which also may be true for SARS-CoV-2.88\nSeveral groups and consortia have been screening and characterizing nanomaterials according to their immunomodulatory properties and absence of cytotoxicity.56,57,82−84,86 The development of an adjuvant for clinical use is a lengthy process that requires extensive Phase III randomized trials in large and diverse cohorts of subjects, and the process generally requires several years.76 Pharmaceutical giants such as GlaxoSmithKline (GSK), which owns the ASs mentioned above and other adjuvant platforms, are engaged in several partnerships to embed their adjuvant systems with SARS-CoV-2-protein-based vaccines. A full-length recombinant SARS-CoV-2 glycoprotein nanoparticle vaccine adjuvanted with the saponin-based Matrix M developed by Novavax is in Phase I clinical testing (NCT04368988).89\nAlthough it is unlikely that novel adjuvants would be used in the context of the current pandemic, the SARS-CoV-2 pandemic offers an opportunity to reflect on the potential of nanotechnology for vaccine adjuvant development. In this context, it is critical to stream coherent pipelines covering in vitro and in vivo experiments specifically to select candidate materials that might be tested for clinical implementation as vaccine adjuvants.90\nIn this view, the status of nanomaterial-based vaccine adjuvants has been reviewed.91,92 In particular, immunomodulatory effects induced on the innate immune signaling have been demonstrated.91 For example, nanomaterials such as GO can elicit an inflammasome sensor (NLRP3)-dependent expression of IL-1β in macrophages.93 Notably, alum, the most commonly used adjuvant in human vaccines, induces the release of this cytokine in macrophages through the same NRLP-induced mechanism. These results suggest that nanomaterials such as GO and alum may be useful for medical applications.94\nConsidering that new vaccine development typically requires years to be approved and applied to the general population, there is also an urgent need to study how to improve treatment approaches. According to the first Phase III clinical studies, the antiviral drug Remdesivir, which was recently approved by the FDA for COVID-19 treatment in the United States, seems to be a promising treatment for adults diagnosed with COVID-19. Nano-based strategies have already been applied to enhance the effectiveness of Remdesivir in the context of other emerging viral infections (Nipah virus),95 suggesting the suitability of nanotechnology to assist with similar strategies for the treatment of COVID-19 as well as other possible pandemics in the future.96\n\nCan Nanotechnology Help to Control the Cytokine Storm?\nOne of the main features of COVID-19 is the triggering of a cytokine storm in the body, also known as cytokine release syndrome (CRS), which results from an excessive immune response and leads to the severe deterioration of patient health.25,97,98 This inflammatory storm is one of the major causes of the acute respiratory distress syndrome (ARDS) that is often associated with multiple-organ failure, representing the leading causes of death in critical patients.98 In particular, the role of interleukin (IL)-6 has been highlighted in patients requiring assisted ventilation. Ongoing clinical trials are testing drugs that block the receptor of IL-6 (Tocilizumab, an anti-IL-6 receptor antibody, and Sarilumab) or IL-6 itself (Siltuximab).\nWhereas a well-regulated cytokine response that is rapidly triggered by the host’s innate immunity can serve to prevent and to counteract an infection, an excessive, unbalanced, prolonged immune response can seriously harm the body. Therefore, therapeutic strategies aimed at effectively suppressing the cytokine storm are under investigation. Nanomaterials have been exploited to adjust the immune response to an optimized level, and such proprieties might be explored to inhibit cytokine releases.99 Nanosystems can enhance the specificity/efficiency of immunosuppressant delivery to target immune cells, with consequent reductions in drug dose, drug distribution to nontarget tissues and organs, and possible side effects. In addition, specific nanotools can be designed to evade the immune system and to enhance the solubility of poorly soluble immunosuppressant agents; the potential of finely tuning their surface charge opens possibilities for encapsulation strategies and offers accommodation for a high drug load. All of these mechanisms may also occur simultaneously, enhancing the activity of immunosuppressive agents.\nConcerning the role of macrophages in COVID-19, the presence of ACE2-expressing CD68+CD169+ macrophages containing SARS-CoV-2 nucleoprotein antigen and showing an increased release of IL-6 was observed in infected spleen and lymph nodes.100 Notably, immunohistochemical and immunofluorescence analyses of lymph nodes and spleen tissue from autopsy samples of patients who died from COVID-19 revealed lymphocytic apoptosis. The tissues infected by SARS-CoV-2 also showed an upregulated expression of Fas, suggesting a role for CD169+ macrophages in viral spreading, aberrant inflammation, and activation-induced lymphocyte apoptosis. Moreover, histological examinations of biopsy samples of patients who died from COVID-19 revealed an increased alveolar exudate due to the extended neutrophil and monocyte infiltrate in lung capillaries with fibrin deposition, probably leading to difficulties in gas exchange. Through nanomedicine, we envision therapeutic approaches aimed at targeting specific immune subpopulations to avoid these complications, and different nanomaterials have already been explored for their specific impact on different immune cell subpopulations.82,83,86 Octadecylamine-functionalized and dexamethasone-adsorbed nanodiamond promotes anti-inflammatory and proregenerative behavior in human macrophages in vitro.101 A low dose of this functionalized nanodiamond also reduced macrophage infiltration and expression of proinflammatory mediators iNOS and tumor necrosis factor (TNF)-α in mice. Overall, these results suggest that nanodiamond particles may be useful as an inherently immunomodulatory platform.\nFinally, the indiscriminate systemic suppression of the immune system may increase the susceptibility to sepsis due to underlying secondary bacterial infections that can exacerbate critical conditions in patients, causing death.102 Risk factors for mortality include acquired bacterial infections by opportunistic organisms and primary pathogens. Using nanocarriers to achieve targeted delivery of immunosuppressive drugs, or the specific and controlled inhibition of only a specific immune cell subpopulation, can play a critical role in limiting such complications.\nThe fast and complete removal of pro-inflammatory cytokines from the bloodstream has been shown to increase survival significantly in patients with early stage sepsis. Adsorption of cytokines from serum by extracorporeal perfusion through porous carbons is one of the most promising ways for their selective, quick, and complete removal from blood circulation. Hierarchical carbon materials with tuned porosity have shown effective adsorption of many cytokines, including IL-6 and even the largest ones, such as TNF-α.103 Based on their demonstrated outstanding performance in removing sepsis-associated pro-inflammatory cytokines from blood, mesoporous carbon adsorbents with hierarchical structure, tuned pore size, and surface chemistry, as well as graphene with open and accessible surfaces104 have significant potential to quickly remove inflammatory cytokines associated with CRS and to prevent mortality arising from an uncontrolled inflammatory cascade, thereby providing enough time for the defense mechanisms of the human body to fight the virus.\n\nPhotodynamic Inactivation of SARS-CoV-2\nIn addition to drug- and vaccine-based antiviral strategies, photodynamic therapy (PDT) stands as a unique approach to inactivate SARS-CoV-2. Using a light-based method, PDT attacks target cells via the excitation of photosensitive agents, called photosensitizers (PSs), with radiation characterized by a wavelength corresponding to its absorption spectrum to generate reactive oxygen species (ROS) in the presence of oxygen, which ultimately results in cell death. Photodynamic therapy is primarily used for the clinical treatment of various oncological disorders.105 It was not until the 1970s that PDT was first used clinically against viruses,106 exploiting ROS production to damage virus proteins, nucleic acids, and—if present—lipids.107 Even though there are research efforts for PDT-based virotherapies against different viruses, including herpes simplex virus, human papilloma virus, and human immunodeficiency virus,106−109 clinical use of PDT is limited due to hydrophobicity of PSs, poor target specificity, and limited tissue penetration ability.\nMost PSs are hydrophobic and aggregate in aqueous solutions, affecting their photochemical and photobiological properties.106,110 For this reason, Lim et al. have proposed a promising approach for photodynamic inactivation of viruses with NPs, developing sodium yttrium fluoride (NaYF4) upconversion NPs (UCNs) with zinc phthalocyanine PSs grafted onto their surfaces. Unlike most PSs, these UCNs are coated with polyethylenimine (PEI), which render them hydrophilic and easier to manipulate. These UCNs showed antiviral activity against Dengue virus serotype 2 and adenovirus type 5, which were used as models of enveloped and non-enveloped viruses, respectively.110 MXenes111,112 are a large family of 2D transition metal carbides, nitrides,113 and carbonitrides114 that exhibit unique electronic, optical, and catalytic properties. They have the general formula Mn+1XnTx, where M is an early transition metal (Ti, Zr, V, Mo, etc.), X is C and/or N, Tx represents the surface functional groups (=O, −OH, −F, −Cl), and n = 1–4.115,116 Some examples include Ti3C2Tx, V2CTx, and Nb2CTx, with over 30 stoichiometric compositions already synthesized with more than 100 predicted. Biocompatible MXenes, such as Ti3C2Tx, are hydrophilic and are among the most efficient light-to-heat transforming materials.117 The plasmon resonance extinction maxima of Ti3C2Tx is at 780 nm, enabling the use of near-infrared (IR) light for PDT. Several other MXenes have absorption maxima in the IR range and have shown outstanding performance in PDT and theranostic applications.118\nFullerene and graphene are also good candidates for virus inactivation by PDT and have proved to be effective against Semliki Forest virus (SFV), vesicular stomatitis virus (VSV), HSV-1, HIV-1, mosquito iridovirus (MIV), and influenza A virus (IAV), as well as the phage MS2.107 In addition, several 2D nanomaterials, including graphene-based materials, MXenes, black phosphorus, graphitic carbon nitride, tungsten disulfide, and molybdenum disulfide, have been reported to improve the efficacy of PDT considerably for cancer treatment.56,57 Therefore, determining if such nanomaterial-based PDT protocols could be exploited to inactivate SARS-CoV-2 is of great interest.\n\nBiomimetic Engineering of Nanodelivery Systems: Artificial Viruses in the Making\nIn an effort to engineer the next generation of nanoscale vectors, scientists have moved from using inorganic components aimed at obtaining inert structures to utilizing biological building blocks that are able to convey additional functionalities to the resulting construct. To cope with the complexity of the body and to evade the multiple layers of defense that tissues and organs have, it is critical to rely on the ability of certain materials to interact with, rather than to eschew, the biology of our body. Every NP system conceived to date faces one common fate: whether injected, inhaled, ingested, or absorbed through our epithelia, all will at some point come into contact with the mixture of fluids and organic compounds that comprise the body. Under such conditions, every material reacts in a unique way according to the conditions they individually face (i.e., the tissue or body region they are in), their composition (i.e., organic or inorganic), and their physical properties (i.e., size, shape, surface charge).119 Inorganic NPs can function as globular protein mimics because of their similar size, charge, shape, and surface features that can be chemically functionalized to resemble proteins. These similarities can be used in biotechnology to control virus pathways or cell receptor interactions with NPs.120\nAmong the many attempts, the legendary accomplishments, and the epic failures, we could list thousands of different NPs, differing from one another thanks to the creative endeavors of their designers. Despite their remarkable differences and researchers’ endeavors to make them one of a kind, they all aim to achieve one goal: to deliver in a specific way one particular form of payload, while remaining as unnoticed as possible by the body’s defense mechanisms. For everyone who has made an effort to create their own version of such a silver bullet, it has come to mind that nature in its incredible variety had already invented a few ingenious solutions to this problem. In the world of nano-based drug delivery, one entity dominates as the quintessential example of precision, efficiency, and stealth: the virus. Not by chance, these two worlds share many features that span from the physical laws that govern their assembly and stability to the chemical similarities in their overall composition.121\nHistorically, the field of nanomedicine and the global endeavor to generate NPs for drug delivery arose from one of the greatest struggles in medicine: gene therapy. At the core of that approach was the idea that viruses could be used as Trojan horses to deliver the correct sequence of the gene into the cells that harbored the mutated copy. In order to identify the virus that offered the best delivery service, a cadre of viral strains and species were tested, adapted, and engineered to fit the aims and hit the targets. It took decades of trial and error, of progressive adjustments, and a few tragic mistakes to identify the viruses that could provide the ideal backbone to develop viral vectors able to ferry genetic cargo into the target cell.122 In the midst of that global challenge, nanotechnology offered a safer and more controllable alternative: to generate bespoke structures that could replace viral vectors and do the same job, delivering a payload from the point of injection to the site of action.\nFast forward a few decades, and the promises made by both worlds seem to have finally become reality, with a number of active clinical trials, some clinical success stories, and a few commercial products in the market space. Interestingly, the two worlds seem to have maintained a safe distance so as to avoid any collision or dangerous proximity. Exchange of ideas between the fields of gene therapy and nanomedicine is limited, and the potential for disciplinary growth through knowledge exchange has remained elusive to scientists in both fields.5 Many scientists have suggested that nanotechnologists could find inspiration in the mechanisms devised by viruses to elude immune surveillance, to overcome biological barriers, and to deliver their genetic payload with high specificity. Similarly, gene therapists would find high-tech solutions to their scalability and safety issues in looking at the new generations of biomimetic particles being generated.\nThe principles of biomimicry and bioinspiration have been used to design and to engineer drug-delivery technologies that reproduce or recapitulate biological materials, for what pertains to not only their structure and chemistry but also, more importantly, their functions. In drug delivery, surface recognition and nanoscale interactions between materials and biological entities are key to the success of the delivery strategy, and the use of biological building blocks such as membrane proteins has been proposed as a way to convey targeting and shielding moieties simultaneously.123 One can only hope that the recent events and global attention that viruses are capturing in the scientific world will spur a renewed interest in finding ways to adapt viral features and mechanisms of action to the world of NPs. Increased focus in this field would be useful to create virus-like NPs able to circulate in the blood system, overcoming the endothelial barrier, and to deliver their therapeutic payload with high efficiency.124\n\nInterference with Cellular Uptake, Immobilization, and Inactivation of the Virus Outside of the Host Cell\nNanomaterials can be synthesized with a high specific surface area of a few hundred square meters per gram. Therefore, dependent on the surface properties, nanomaterials efficiently adsorb biomolecules and form a so-called biomolecular corona. This passive, nontargeted adsorption might be utilized to bind viruses, provided that the selected nanomaterial is relatively biocompatible. Viral surface proteins are often modified by sugar moieties or encompass positively charged amino acid patches that bind to lectins or glycosaminoglycans (GAGs) of heparan sulfate (HS), respectively.32 Robust interactions of virus particles with these host receptors is ensured by multivalent binding, which is likely why single-molecule inhibitors often are not capable of efficiently perturbing this key event but multivalent NPs are superior to block binding of different viruses to the host cell.125\nGold NPs capped with mercaptoethanesulfonate are effective inhibitors of HSV type 1 infection as they mimic cell-surface-receptor heparan sulfate and, therefore, competitively bind to the virus. Interestingly, polyvalent sulfated Au NPs inhibit virus binding to the host cell dependent on their size. Nanoparticles of diameters equal to and larger than the virus diameter (in this case, the stomatitis virus) more efficiently inhibit the binding to cells than smaller particles. Most likely, larger NPs efficiently cross-link virions, whereas smaller NPs simply decorate the viral surface.126 Papp et al. found that gold NPs decorated with SA effectively inhibited the binding of influenza virus to the target cells. In this case, viral recognition via its surface protein hemagglutinin of SA on the host cell membrane was a prerequisite for cellular entry.127 More recently, Cagno et al. reported antiviral NPs (Au and iron oxide core) with long and flexible linkers mimicking HS that strongly bind and inactivate viruses such as respiratory syncytial virus in vitro and in vivo in a lung infection model and even led to irreversible viral deformation.53 Hence, there is ample evidence that biocompatible, functionalized NPs can act as broad-spectrum antivirals. Notably, the receptor-binding domain of the spike S1 protein of SARS-CoV-2 binds not only to ACE2 but also to heparin128 and, thus, might be targeted by similar approaches as outlined above.\nOne of the most recent strategies to inhibit viral uptake is based on administration of recombinant ACE2 to inhibit binding competitively via the spike S1 protein.21 Knowing that multivalency is key to block virus–host interactions reliably, researchers have speculated that a nanostructured carrier could not only improve delivery and cargo stability but also might dramatically enhance binding strength.129\n\nSpeeding up the Nanomedicine-Based Approaches for COVID-19 by In Silico Analysis\nCurrently, repurposing drug molecules is a key strategy for identifying approved or investigational drugs outside the scope of the original medical indication that can be used to fight COVID-19.130,131 There are various advantages to this strategy, including already-established safety profiles, fast transition to clinical studies, and less investment needed, compared to the process of developing an entirely new drug.132,133 Therefore, these advantages have the potential to result in less risky and more rapid returns on investment in the development of repurposed drugs, benefits that are particularly important during pandemics. In addition to rapid diagnosis, making recommendations to physicians about rapid treatment methods can save the lives of many people. Combining in silico tools such as molecular docking, molecular dynamics, and computational chemistry with large drug databases provides a great advantage in selecting “possible candidates” from among thousands of pharmaceutically active substances (Figure 3). In silico analyses should be supported by the literature, expert opinions (pharmacologists and clinicians), and, when possible, preclinical and clinical findings. After selecting the best candidates following in silico analyses, detailed in vitro and in vivo experimentation should be undertaken prior to clinical studies. Recently, various drug-repurposing studies have been published to assist in the global COVID-19 pandemic response.134−136 Such studies aim to identify whether already-approved drugs have the capacity to interact with viral proteins or receptors on host cells.\nFigure 3 Schematic of how in silico analysis can be used to select candidate drug molecules for clinical studies. MD, molecular dynamics. In nanomedicine, computational models have recently garnered attention; such work may help to identify how nanomaterials interact with biological systems and to determine how the efficacy of these nanotherapeutics could be improved. Computational models indicate how NPs are taken up by healthy cells or tumor cells, enabling better predictions regarding the pharmacokinetic and pharmacodynamic properties of these materials.134 For example, Lunnoo et al. used a coarse-grained molecular dynamics (MD) simulation to observe the internalization pathways of various Au nanostructures (nanospheres, nanocages, nanorods, nanoplates, and nanohexapods) into an idealized mammalian plasma membrane.137 Other studies have simulated how different NPs can target tumor cells and deliver drugs.138,139 Therefore, in silico approaches that are currently used for drug repurposing, including molecular docking, molecular dynamics, and computational chemistry, constitute valuable tools to aid preclinical and clinical studies of nanomaterials directed for disease treatment. Considering the urgent need for nanomedicine against the current pandemic, in silico analyses may be especially useful in guiding the rational design of new NP formulations required to fight SARS-CoV-2."}
LitCovid-sentences
{"project":"LitCovid-sentences","denotations":[{"id":"T53","span":{"begin":0,"end":57},"obj":"Sentence"},{"id":"T54","span":{"begin":58,"end":255},"obj":"Sentence"},{"id":"T55","span":{"begin":256,"end":329},"obj":"Sentence"},{"id":"T56","span":{"begin":330,"end":588},"obj":"Sentence"},{"id":"T57","span":{"begin":589,"end":751},"obj":"Sentence"},{"id":"T58","span":{"begin":752,"end":903},"obj":"Sentence"},{"id":"T59","span":{"begin":904,"end":1126},"obj":"Sentence"},{"id":"T60","span":{"begin":1127,"end":1286},"obj":"Sentence"},{"id":"T61","span":{"begin":1287,"end":1447},"obj":"Sentence"},{"id":"T62","span":{"begin":1448,"end":1544},"obj":"Sentence"},{"id":"T63","span":{"begin":1545,"end":1639},"obj":"Sentence"},{"id":"T64","span":{"begin":1640,"end":1823},"obj":"Sentence"},{"id":"T65","span":{"begin":1824,"end":1997},"obj":"Sentence"},{"id":"T66","span":{"begin":1998,"end":2280},"obj":"Sentence"},{"id":"T67","span":{"begin":2281,"end":2668},"obj":"Sentence"},{"id":"T68","span":{"begin":2669,"end":3407},"obj":"Sentence"},{"id":"T69","span":{"begin":3408,"end":3504},"obj":"Sentence"},{"id":"T70","span":{"begin":3505,"end":4027},"obj":"Sentence"},{"id":"T71","span":{"begin":4028,"end":4338},"obj":"Sentence"},{"id":"T72","span":{"begin":4340,"end":4399},"obj":"Sentence"},{"id":"T73","span":{"begin":4400,"end":4569},"obj":"Sentence"},{"id":"T74","span":{"begin":4570,"end":4906},"obj":"Sentence"},{"id":"T75","span":{"begin":4907,"end":5115},"obj":"Sentence"},{"id":"T76","span":{"begin":5116,"end":5356},"obj":"Sentence"},{"id":"T77","span":{"begin":5357,"end":5904},"obj":"Sentence"},{"id":"T78","span":{"begin":5905,"end":6067},"obj":"Sentence"},{"id":"T79","span":{"begin":6068,"end":6194},"obj":"Sentence"},{"id":"T80","span":{"begin":6195,"end":7045},"obj":"Sentence"},{"id":"T81","span":{"begin":7046,"end":7146},"obj":"Sentence"},{"id":"T82","span":{"begin":7147,"end":7464},"obj":"Sentence"},{"id":"T83","span":{"begin":7465,"end":7946},"obj":"Sentence"},{"id":"T84","span":{"begin":7947,"end":8041},"obj":"Sentence"},{"id":"T85","span":{"begin":8042,"end":8603},"obj":"Sentence"},{"id":"T86","span":{"begin":8604,"end":8841},"obj":"Sentence"},{"id":"T87","span":{"begin":8842,"end":9069},"obj":"Sentence"},{"id":"T88","span":{"begin":9070,"end":9176},"obj":"Sentence"},{"id":"T89","span":{"begin":9177,"end":9310},"obj":"Sentence"},{"id":"T90","span":{"begin":9311,"end":9409},"obj":"Sentence"},{"id":"T91","span":{"begin":9410,"end":10045},"obj":"Sentence"},{"id":"T92","span":{"begin":10046,"end":10234},"obj":"Sentence"},{"id":"T93","span":{"begin":10235,"end":10467},"obj":"Sentence"},{"id":"T94","span":{"begin":10468,"end":10864},"obj":"Sentence"},{"id":"T95","span":{"begin":10865,"end":11621},"obj":"Sentence"},{"id":"T96","span":{"begin":11622,"end":11932},"obj":"Sentence"},{"id":"T97","span":{"begin":11933,"end":12156},"obj":"Sentence"},{"id":"T98","span":{"begin":12157,"end":12396},"obj":"Sentence"},{"id":"T99","span":{"begin":12397,"end":12467},"obj":"Sentence"},{"id":"T100","span":{"begin":12468,"end":12742},"obj":"Sentence"},{"id":"T101","span":{"begin":12743,"end":12901},"obj":"Sentence"},{"id":"T102","span":{"begin":12902,"end":14119},"obj":"Sentence"},{"id":"T103","span":{"begin":14120,"end":14490},"obj":"Sentence"},{"id":"T104","span":{"begin":14491,"end":14628},"obj":"Sentence"},{"id":"T105","span":{"begin":14629,"end":15526},"obj":"Sentence"},{"id":"T106","span":{"begin":15527,"end":16139},"obj":"Sentence"},{"id":"T107","span":{"begin":16140,"end":16322},"obj":"Sentence"},{"id":"T108","span":{"begin":16323,"end":16547},"obj":"Sentence"},{"id":"T109","span":{"begin":16548,"end":16766},"obj":"Sentence"},{"id":"T110","span":{"begin":16767,"end":17247},"obj":"Sentence"},{"id":"T111","span":{"begin":17248,"end":17350},"obj":"Sentence"},{"id":"T112","span":{"begin":17351,"end":17545},"obj":"Sentence"},{"id":"T113","span":{"begin":17546,"end":17781},"obj":"Sentence"},{"id":"T114","span":{"begin":17782,"end":18101},"obj":"Sentence"},{"id":"T115","span":{"begin":18103,"end":18157},"obj":"Sentence"},{"id":"T116","span":{"begin":18158,"end":18736},"obj":"Sentence"},{"id":"T117","span":{"begin":18737,"end":18900},"obj":"Sentence"},{"id":"T118","span":{"begin":18901,"end":19133},"obj":"Sentence"},{"id":"T119","span":{"begin":19134,"end":19244},"obj":"Sentence"},{"id":"T120","span":{"begin":19245,"end":19626},"obj":"Sentence"},{"id":"T121","span":{"begin":19627,"end":19923},"obj":"Sentence"},{"id":"T122","span":{"begin":19924,"end":20030},"obj":"Sentence"},{"id":"T123","span":{"begin":20031,"end":20453},"obj":"Sentence"},{"id":"T124","span":{"begin":20454,"end":20663},"obj":"Sentence"},{"id":"T125","span":{"begin":20664,"end":20940},"obj":"Sentence"},{"id":"T126","span":{"begin":20941,"end":21541},"obj":"Sentence"},{"id":"T127","span":{"begin":21542,"end":21657},"obj":"Sentence"},{"id":"T128","span":{"begin":21658,"end":22004},"obj":"Sentence"},{"id":"T129","span":{"begin":22005,"end":22225},"obj":"Sentence"},{"id":"T130","span":{"begin":22226,"end":22393},"obj":"Sentence"},{"id":"T131","span":{"begin":22394,"end":22586},"obj":"Sentence"},{"id":"T132","span":{"begin":22587,"end":23282},"obj":"Sentence"},{"id":"T133","span":{"begin":23284,"end":23323},"obj":"Sentence"},{"id":"T134","span":{"begin":23324,"end":23465},"obj":"Sentence"},{"id":"T135","span":{"begin":23466,"end":23789},"obj":"Sentence"},{"id":"T136","span":{"begin":23790,"end":24383},"obj":"Sentence"},{"id":"T137","span":{"begin":24384,"end":24752},"obj":"Sentence"},{"id":"T138","span":{"begin":24753,"end":24876},"obj":"Sentence"},{"id":"T139","span":{"begin":24877,"end":25218},"obj":"Sentence"},{"id":"T140","span":{"begin":25219,"end":25560},"obj":"Sentence"},{"id":"T141","span":{"begin":25561,"end":25808},"obj":"Sentence"},{"id":"T142","span":{"begin":25809,"end":25947},"obj":"Sentence"},{"id":"T143","span":{"begin":25948,"end":26619},"obj":"Sentence"},{"id":"T144","span":{"begin":26621,"end":26668},"obj":"Sentence"},{"id":"T145","span":{"begin":26669,"end":26701},"obj":"Sentence"},{"id":"T146","span":{"begin":26702,"end":26977},"obj":"Sentence"},{"id":"T147","span":{"begin":26978,"end":27216},"obj":"Sentence"},{"id":"T148","span":{"begin":27217,"end":27459},"obj":"Sentence"},{"id":"T149","span":{"begin":27460,"end":27917},"obj":"Sentence"},{"id":"T150","span":{"begin":27918,"end":28034},"obj":"Sentence"},{"id":"T151","span":{"begin":28035,"end":28235},"obj":"Sentence"},{"id":"T152","span":{"begin":28236,"end":28497},"obj":"Sentence"},{"id":"T153","span":{"begin":28498,"end":28708},"obj":"Sentence"},{"id":"T154","span":{"begin":28709,"end":28851},"obj":"Sentence"},{"id":"T155","span":{"begin":28852,"end":29039},"obj":"Sentence"},{"id":"T156","span":{"begin":29040,"end":29205},"obj":"Sentence"},{"id":"T157","span":{"begin":29206,"end":29382},"obj":"Sentence"},{"id":"T158","span":{"begin":29383,"end":29564},"obj":"Sentence"},{"id":"T159","span":{"begin":29565,"end":30056},"obj":"Sentence"},{"id":"T160","span":{"begin":30057,"end":30281},"obj":"Sentence"},{"id":"T161","span":{"begin":30282,"end":30404},"obj":"Sentence"},{"id":"T162","span":{"begin":30405,"end":30844},"obj":"Sentence"},{"id":"T163","span":{"begin":30845,"end":31016},"obj":"Sentence"},{"id":"T164","span":{"begin":31017,"end":31290},"obj":"Sentence"},{"id":"T165","span":{"begin":31291,"end":31831},"obj":"Sentence"},{"id":"T166","span":{"begin":31832,"end":32043},"obj":"Sentence"},{"id":"T167","span":{"begin":32045,"end":32150},"obj":"Sentence"},{"id":"T168","span":{"begin":32151,"end":32258},"obj":"Sentence"},{"id":"T169","span":{"begin":32259,"end":32394},"obj":"Sentence"},{"id":"T170","span":{"begin":32395,"end":32535},"obj":"Sentence"},{"id":"T171","span":{"begin":32536,"end":33039},"obj":"Sentence"},{"id":"T172","span":{"begin":33040,"end":33234},"obj":"Sentence"},{"id":"T173","span":{"begin":33235,"end":33340},"obj":"Sentence"},{"id":"T174","span":{"begin":33341,"end":33518},"obj":"Sentence"},{"id":"T175","span":{"begin":33519,"end":33756},"obj":"Sentence"},{"id":"T176","span":{"begin":33757,"end":34303},"obj":"Sentence"},{"id":"T177","span":{"begin":34304,"end":34494},"obj":"Sentence"},{"id":"T178","span":{"begin":34495,"end":34903},"obj":"Sentence"},{"id":"T179","span":{"begin":34905,"end":34985},"obj":"Sentence"},{"id":"T180","span":{"begin":34986,"end":35620},"obj":"Sentence"},{"id":"T181","span":{"begin":35621,"end":35754},"obj":"Sentence"},{"id":"T182","span":{"begin":35755,"end":36014},"obj":"Sentence"},{"id":"T183","span":{"begin":36015,"end":36177},"obj":"Sentence"},{"id":"T184","span":{"begin":36178,"end":36337},"obj":"Sentence"},{"id":"T185","span":{"begin":36338,"end":36597},"obj":"Sentence"},{"id":"T186","span":{"begin":36598,"end":36712},"obj":"Sentence"},{"id":"T187","span":{"begin":36713,"end":36736},"obj":"Sentence"},{"id":"T188","span":{"begin":36737,"end":36969},"obj":"Sentence"},{"id":"T189","span":{"begin":36970,"end":37798},"obj":"Sentence"},{"id":"T190","span":{"begin":37799,"end":38001},"obj":"Sentence"}],"namespaces":[{"prefix":"_base","uri":"http://pubannotation.org/ontology/tao.owl#"}],"text":"Nanotechnology Tools to Inactivate SARS-CoV-2 in Patients\nThe main target of SARS-CoV-2 is the respiratory tract (upper airways, lung),36 although other organs might also be infected (e.g., gut, kidney) and vasculature21 also appears to be a prime target. The expression of ACE2 probably determines uptake by different tissues.37\nIn addition to discussing immune-based approaches, because the lung is the most critically affected organ, we will center our discussion on the various options to inactivate the virus in the deep lung and to target the essential host cells for drug delivery. The virus reaches the alveoli and enters alveolar epithelial type II cells (AECII), due to the relatively high abundance of ACE2 and a permissive cellular milieu. These cells serve as a reservoir of the virus, which finally spreads throughout the lung, leading to the lung function impairment seen in severe cases. Airborne nanomaterials are optimally suited to penetrate into the deep lung due to the physicochemical properties of such aerosols, existing on the same size scale particles that penetrate most readily to the deep airways. Hence, nanomedicine is already actively pursuing ideas to deliver drugs, therapeutic proteins, and mRNAs by exploiting nanodevices for pulmonary delivery.38,39\nMoreover, the rapid emergence of SARS-CoV-2 has exposed one of the main weaknesses in the current medical landscape: the lack of broad-spectrum antiviral drugs. At present, there are only a handful of approved antivirals, and they are mostly virus-specific. Hence, when a new virus emerges, little can be done pharmacologically to slow down its spread. Some research efforts have been focused on the development of broad-spectrum drugs, which could potentially offer some efficacy against future emerging viruses (and maybe SARS-CoV-2). The various approaches developed over the years are mainly based on the creation of entry inhibitors.40,41 A highly conserved part in viruses is the attachment ligand (VAL). In most known respiratory viruses,42 the VAL targets either heparan sulfate proteoglycans (HSPG)43 or sialic acids (SA).44 Both HSPG and SA mimics have shown in vitro ability to bind to viruses, blocking their interaction with cell membranes, and often in a broad-spectrum way.45−47\nIn the context of nanomedicine, many nanomaterials have been developed, ranging from polymers48 to dendrimers,49 oligomers, NPs,50 liposomes,51 and small molecules.52 However, successful clinical translation has been hindered by the fact that, upon dilution, these compounds lose efficacy as the virus-compound complex dissociates leaving viruses free to restart their replication cycle. Recently, it has been shown that this limitation can be overcome by synthesizing NPs that, after binding, are able to inhibit viral infectivity irreversibly by permanently damaging the virion, refueling the hope for a true, broad-spectrum antiviral drug.53 Because the focus is also on the development of a drug specific to SARS-CoV-2, a good entry inhibitor could be based on blocking the S spike protein interaction with the cellular ACE2 receptor.19,21−23 Regardless of the specific approach, it is imperative that novel, effective antivirals be based on compounds that exhibit very low or negligible toxicity profiles, as patients will most likely need to receive those drugs for extended periods of time and will already be weakened. For these reasons, when designing antiviral drugs, clearance mechanisms have to be kept in mind. An example of this process is the recent redesign of broad-spectrum antiviral NPs into equally effective modified cyclodextrins.54 Moreover, nanotechnology may offer nanotheranostic approaches to fill the existing gap between diagnostics and therapy.55−57 The simultaneous management of both diagnostics and therapy for those suffering from COVID-19 or in future pandemics, as for many other diseases, is an additional potential strategy to take into consideration in which nanomaterials have proven to be effective tools. The advantages of the capabilities of nanotechnology and nanomaterials for combined therapeutics and diagnostics has been widely explored in cancer research; however, there have been considerable efforts in the past few years to extend the scope of this approach to other areas including infectious diseases.58\n\nNanomaterial-Based Vaccine Development and Immunomodulation\nFollowing the publication of the genetic sequence of SARS-CoV-2 on January 11, 2020, intense research efforts have been devoted to developing a vaccine against COVID-19. With unprecedented speed, this extraordinary scientific mobilization led the first vaccine candidate to enter the Phase I human clinical trial on March 16, 2020, and other novel candidates are rapidly following.59 Up to May 22, 2020, there are 10 COVID-19 candidate vaccines in clinical evaluations and 114 in preclinical development.60\nConcerning vaccine and immunization research, nanomaterials can assist in multiple ways to boost the upregulation required by the immune system and to direct the immune response specifically against antigens. Immune-targeted nanotherapeutics can be developed through their rational manufacture at the nanoscale level by designing nanomaterials that are able to amplify host’s immune response, for instance as adjuvants in the context of vaccination.\nThe development of a vaccine will rely either on the direct administration of viral antigens (e.g., in the form of recombinant proteins, vectored vaccines, or whole inactivated or attenuated virus) or RNA- or DNA-encoding viral antigens.27 Candidate antigens for immunization are surface proteins such as the immunogenic spike protein (S1), which is already targeted by antibodies of convalescent patients.27 Because the S1 protein is also essential for cellular uptake, many researchers are using this protein as the primary target for a vaccine.\nThere are many issues related to the delivery of a drug, protein, or RNA into the patient as the cargo is often degraded, not bioavailable, or is swiftly cleared. Nanotechnology provides multiple solutions to these challenges though, as nanocarriers can overcome some of these limitations.\nBiocompatible polymeric-, lipid-based, or inorganic NPs can be tuned with respect to their physicochemical properties to encapsulate cargo proteins with high loading efficiency, improving protein delivery and pharmacokinetics over conventional approaches.38 Intranasal delivery of polymer-encapsulated antigen triggers a strong immune response, and the success of vaccination depends on the appropriate type of polymer in combination with the antigen.61,62 Similarly, researchers have developed lipid and lipid-based NPs as delivery platforms for mRNAs or siRNAs to enable the synthesis of key viral proteins for vaccination or to inactivate critical viral target genes, respectively.39 The field of therapeutic mRNAs to support vaccination has gained momentum, as well,63 at least in part due to nanotechnology-enabled strategies for cargo delivery. Moreover, the versatility of nanoplatforms as antigen-presenting systems offers great opportunities. Considering the possibility of random viral mutations that may alter the antigen shape, functionalizing nanomaterials with a wide number of molecules at the same time to target the virus, or motifs that are specific for pathogens, will increase the efficiency of vaccines and their ability to prevent viral infection.\nSeveral companies are working on mRNA vaccines encoding SARS-CoV-2 proteins such as the spike protein, encapsulated in nanoliposomes with specific physicochemical properties that are potentially akin to those documented for immunization against certain tumor antigens.64 The design of such nanocarriers, which will need to escape recognition by scavenger cells and to be nontoxic and nonimmunogenic, is a challenge that will require substantial time prior to clinical availability.\nOn March 16, 2020, Moderna, through a partnership with the Vaccine Research Center at the U.S. National Institutes of Health, enrolled the first participants into a Phase I clinical trial testing an mRNA vaccine (mRNA-1273) encapsulated in lipid NPs—a record time of just 63 days following sequence selection (NCT04283461).65 The enrollment of the first cohort of participants (18 to 55-year-old healthy subjects) concluded on April 16, 2020.66 CureVac and BioNTech (in partnership with Pfizer) are currently working on similar vaccines; Pfizer/BioNTech, in particular, have recently started the recruitment in Phase I/II trials (NCT04368728, NCT04380701). A DNA plasmid vaccine by Inovio Pharmaceuticals (INO-4800) has showed promising results in mice and guinea pigs according to a recent article published in Nature Communications(67) and has entered Phase I testing in humans (NCT04336410).\nAnother candidate COVID-19 vaccine for Phase I clinical trial is from the University of Oxford and AstraZeneca (NCT04324606).68 Around 1110 people will take part in the trial, which started recruitment at the end of April 2020. The vaccine is based on a chimpanzee adenovirus vaccine vector (ChAdOx1) and the SARS-CoV-2 spike protein. Chimpanzee adenoviral vectors against different pathogens have been already tested in thousands of subjects with demonstrated safety. To date, ChAdOx1 has been administered to six rhesus macaques exposed to high doses of SARS-CoV-2. The vaccine was unable to prevent infections, although it reduced the severity of the disease: no signs of virus replication were observed in the lungs, with significantly lower levels of respiratory disease and no lung damage compared to control animals, according to a recent article deposited in bioRxiv.69 Another adenoviral vector vaccine developed by CanSino Biological Inc. and Beijing Institute of Biotechnology using a genetically engineered replication-defective adenovirus type 5 vector to express the SARS-CoV-2 spike protein (Ad5-nCoV) is currently being tested in Phase I/II trials (NCT04398147, NCT04341389, NCT0431312).\nAlthough vaccines based on novel DNA and mRNA technologies might be promising, there are no such kinds of vaccines on the market, and it is unknown whether they can be effective in humans. Moderna, for instance, has generated preliminary safety data on different mRNA-based vaccines targeting other respiratory viruses, but their more advanced program (on a cytomegalovirus vaccine) is still in Phase II clinical testing. However, on May 18, Moderna announced that mRNA-1273 elicited antibody titers above the levels observed in convalescent individuals (and therefore considered potentially protective) in all eight initial participants across the 25 and 100 μg dose cohorts of the Phase I trial (NCT04283461).70 The company, after having received a fast-track approval from the FDA, is now moving on Phase II trials.\nConversely, strong evidence exists regarding the efficacy of protein-based vaccines such as recombinant-protein, viral-vector, attenuated, or inactivated vaccines across different infectious diseases, with licensed vaccines already existing for all of these platforms.27 All of the aforementioned approaches are currently being explored in the context of SARS-CoV-2.27 An inactivated vaccine developed by the China National Pharmaceutical Group (Sinopharm), in collaboration with the Wuhan Institute of Biological Products, is currently tested in a Phase I/II trial (ChiCTR2000031809), and a second inactivated vaccine (in collaboration with the Beijing Institute of Biological Products) has been currently approved for clinical testing (ChiCTR2000032459). A third aluminum salt (alum) adjuvanted inactivated vaccine, developed by Beijing-based Sinovac Biotech’s, was able to provide partial or complete protection in rhesus macaques, according to a recent article published in Science,71 and is currently being tested in Phase I/II trials (NCT04383574, NCT04352608).\nLive-attenuated vaccines are intrinsically immunogenic (e.g., due to the presence of viral DNA), but extensive safety tests are required due to the rare possibility of reversion to a pathogenic form able to cause infection. Vaccine candidates based in this application have previously been designed for SARS-CoV with high stability.72,73 Recombinant-protein vaccines and inactivated vaccines are safer but might require adjuvants to increase their immunogenicity. In the context of SARS-CoV-2, adjuvants are important for two reasons. First, adjuvants might increase the efficacy of the vaccine, especially in subjects with impaired immunological function, such as the elderly, or in subjects with comorbidities resulting in immune dysfunctions; in these patient cohorts, SARS-CoV-2 has a high lethality rate. Second, adjuvants can reduce the amount of vaccine protein(s) required per dose, which could facilitate scaling-up vaccine production in a reduced time frame.\nBeyond alum, which is in fact a nanoscale material74 that was developed in the 1920s for the tetanus and diphtheria toxoids,75 approval for a new adjuvant did not occur until 1997, with the introduction of the oil-in-water emulsion of squalene oil and polysorbate 80 and sorbitan trioleate surfactants (MF59) in the seasonal influenza vaccine for the elderly.76 The use of MF59 was further expanded to pandemic and avian influenza vaccines.77 Other adjuvants in licensed vaccines have been approved since 2000, as well: (a) AS03 (used for pandemic and avian influenza vaccines), similar to MF59, but including α-tocopherol as an additional immune stimulant; (b) AF 03 (used for pandemic influenza vaccines), an alternative squalene emulsion containing polyoxyethylene, cetostearyl ether, mannitol, and orbitan oleate;78 (c) AS 01 (used for herpes zoster vaccine), a liposome-based vaccine adjuvant system containing two immunostimulants, 3-O-desacyl-4′-monophosphoryl lipid A (MPL, a Toll-like receptor 4 agonist) and saponin QS-21, which activates the ACT-NLRP3 inflammasome pathway;79 and (d) AS04 (used for hepatitis B and human papilloma virus vaccines), which is a combination of MPL and aluminum hydroxide.76,80\nIn this context, the concept of “nanoimmunity by design” relies on the rational design of distinct physicochemical properties and specific functionalization of nanomaterials intended for fine-tuning their potential effects on the immune system.81 Nanomaterials have emerged as promising tools for immune modulation, either stimulating or suppressing the immune response. In fighting SARS-CoV-2, these properties may find applications for both prevention and therapy and in the context of vaccine development.\nMounting evidence indicates that nanomaterials such as graphene,82 nanodiamonds,83 carbon nanotubes,84 and polystyrene particles85 bear an intrinsic capacity to activate the immune system, depending on their functionalization.86 For instance, graphene oxide functionalized with amino groups (GO-NH2) induces activation of STAT1/IRF1 interferon signaling in monocytes and T cells, resulting in the production of T cell chemoattractants, and macrophage 1 (M1) 1/T-helper 1 (Th1) polarization of the immune response, with negligible toxicity.82 Remarkably, the ability of licensed adjuvants such as AS01 and AS03 to enhance adaptive immunity has been linked to their capacity to boost STAT1/IRF1 interferon signaling.87 In addition, recent SARS-CoV and MERS-CoV studies suggest that the development of a Th1-type response is central for controlling infection, which also may be true for SARS-CoV-2.88\nSeveral groups and consortia have been screening and characterizing nanomaterials according to their immunomodulatory properties and absence of cytotoxicity.56,57,82−84,86 The development of an adjuvant for clinical use is a lengthy process that requires extensive Phase III randomized trials in large and diverse cohorts of subjects, and the process generally requires several years.76 Pharmaceutical giants such as GlaxoSmithKline (GSK), which owns the ASs mentioned above and other adjuvant platforms, are engaged in several partnerships to embed their adjuvant systems with SARS-CoV-2-protein-based vaccines. A full-length recombinant SARS-CoV-2 glycoprotein nanoparticle vaccine adjuvanted with the saponin-based Matrix M developed by Novavax is in Phase I clinical testing (NCT04368988).89\nAlthough it is unlikely that novel adjuvants would be used in the context of the current pandemic, the SARS-CoV-2 pandemic offers an opportunity to reflect on the potential of nanotechnology for vaccine adjuvant development. In this context, it is critical to stream coherent pipelines covering in vitro and in vivo experiments specifically to select candidate materials that might be tested for clinical implementation as vaccine adjuvants.90\nIn this view, the status of nanomaterial-based vaccine adjuvants has been reviewed.91,92 In particular, immunomodulatory effects induced on the innate immune signaling have been demonstrated.91 For example, nanomaterials such as GO can elicit an inflammasome sensor (NLRP3)-dependent expression of IL-1β in macrophages.93 Notably, alum, the most commonly used adjuvant in human vaccines, induces the release of this cytokine in macrophages through the same NRLP-induced mechanism. These results suggest that nanomaterials such as GO and alum may be useful for medical applications.94\nConsidering that new vaccine development typically requires years to be approved and applied to the general population, there is also an urgent need to study how to improve treatment approaches. According to the first Phase III clinical studies, the antiviral drug Remdesivir, which was recently approved by the FDA for COVID-19 treatment in the United States, seems to be a promising treatment for adults diagnosed with COVID-19. Nano-based strategies have already been applied to enhance the effectiveness of Remdesivir in the context of other emerging viral infections (Nipah virus),95 suggesting the suitability of nanotechnology to assist with similar strategies for the treatment of COVID-19 as well as other possible pandemics in the future.96\n\nCan Nanotechnology Help to Control the Cytokine Storm?\nOne of the main features of COVID-19 is the triggering of a cytokine storm in the body, also known as cytokine release syndrome (CRS), which results from an excessive immune response and leads to the severe deterioration of patient health.25,97,98 This inflammatory storm is one of the major causes of the acute respiratory distress syndrome (ARDS) that is often associated with multiple-organ failure, representing the leading causes of death in critical patients.98 In particular, the role of interleukin (IL)-6 has been highlighted in patients requiring assisted ventilation. Ongoing clinical trials are testing drugs that block the receptor of IL-6 (Tocilizumab, an anti-IL-6 receptor antibody, and Sarilumab) or IL-6 itself (Siltuximab).\nWhereas a well-regulated cytokine response that is rapidly triggered by the host’s innate immunity can serve to prevent and to counteract an infection, an excessive, unbalanced, prolonged immune response can seriously harm the body. Therefore, therapeutic strategies aimed at effectively suppressing the cytokine storm are under investigation. Nanomaterials have been exploited to adjust the immune response to an optimized level, and such proprieties might be explored to inhibit cytokine releases.99 Nanosystems can enhance the specificity/efficiency of immunosuppressant delivery to target immune cells, with consequent reductions in drug dose, drug distribution to nontarget tissues and organs, and possible side effects. In addition, specific nanotools can be designed to evade the immune system and to enhance the solubility of poorly soluble immunosuppressant agents; the potential of finely tuning their surface charge opens possibilities for encapsulation strategies and offers accommodation for a high drug load. All of these mechanisms may also occur simultaneously, enhancing the activity of immunosuppressive agents.\nConcerning the role of macrophages in COVID-19, the presence of ACE2-expressing CD68+CD169+ macrophages containing SARS-CoV-2 nucleoprotein antigen and showing an increased release of IL-6 was observed in infected spleen and lymph nodes.100 Notably, immunohistochemical and immunofluorescence analyses of lymph nodes and spleen tissue from autopsy samples of patients who died from COVID-19 revealed lymphocytic apoptosis. The tissues infected by SARS-CoV-2 also showed an upregulated expression of Fas, suggesting a role for CD169+ macrophages in viral spreading, aberrant inflammation, and activation-induced lymphocyte apoptosis. Moreover, histological examinations of biopsy samples of patients who died from COVID-19 revealed an increased alveolar exudate due to the extended neutrophil and monocyte infiltrate in lung capillaries with fibrin deposition, probably leading to difficulties in gas exchange. Through nanomedicine, we envision therapeutic approaches aimed at targeting specific immune subpopulations to avoid these complications, and different nanomaterials have already been explored for their specific impact on different immune cell subpopulations.82,83,86 Octadecylamine-functionalized and dexamethasone-adsorbed nanodiamond promotes anti-inflammatory and proregenerative behavior in human macrophages in vitro.101 A low dose of this functionalized nanodiamond also reduced macrophage infiltration and expression of proinflammatory mediators iNOS and tumor necrosis factor (TNF)-α in mice. Overall, these results suggest that nanodiamond particles may be useful as an inherently immunomodulatory platform.\nFinally, the indiscriminate systemic suppression of the immune system may increase the susceptibility to sepsis due to underlying secondary bacterial infections that can exacerbate critical conditions in patients, causing death.102 Risk factors for mortality include acquired bacterial infections by opportunistic organisms and primary pathogens. Using nanocarriers to achieve targeted delivery of immunosuppressive drugs, or the specific and controlled inhibition of only a specific immune cell subpopulation, can play a critical role in limiting such complications.\nThe fast and complete removal of pro-inflammatory cytokines from the bloodstream has been shown to increase survival significantly in patients with early stage sepsis. Adsorption of cytokines from serum by extracorporeal perfusion through porous carbons is one of the most promising ways for their selective, quick, and complete removal from blood circulation. Hierarchical carbon materials with tuned porosity have shown effective adsorption of many cytokines, including IL-6 and even the largest ones, such as TNF-α.103 Based on their demonstrated outstanding performance in removing sepsis-associated pro-inflammatory cytokines from blood, mesoporous carbon adsorbents with hierarchical structure, tuned pore size, and surface chemistry, as well as graphene with open and accessible surfaces104 have significant potential to quickly remove inflammatory cytokines associated with CRS and to prevent mortality arising from an uncontrolled inflammatory cascade, thereby providing enough time for the defense mechanisms of the human body to fight the virus.\n\nPhotodynamic Inactivation of SARS-CoV-2\nIn addition to drug- and vaccine-based antiviral strategies, photodynamic therapy (PDT) stands as a unique approach to inactivate SARS-CoV-2. Using a light-based method, PDT attacks target cells via the excitation of photosensitive agents, called photosensitizers (PSs), with radiation characterized by a wavelength corresponding to its absorption spectrum to generate reactive oxygen species (ROS) in the presence of oxygen, which ultimately results in cell death. Photodynamic therapy is primarily used for the clinical treatment of various oncological disorders.105 It was not until the 1970s that PDT was first used clinically against viruses,106 exploiting ROS production to damage virus proteins, nucleic acids, and—if present—lipids.107 Even though there are research efforts for PDT-based virotherapies against different viruses, including herpes simplex virus, human papilloma virus, and human immunodeficiency virus,106−109 clinical use of PDT is limited due to hydrophobicity of PSs, poor target specificity, and limited tissue penetration ability.\nMost PSs are hydrophobic and aggregate in aqueous solutions, affecting their photochemical and photobiological properties.106,110 For this reason, Lim et al. have proposed a promising approach for photodynamic inactivation of viruses with NPs, developing sodium yttrium fluoride (NaYF4) upconversion NPs (UCNs) with zinc phthalocyanine PSs grafted onto their surfaces. Unlike most PSs, these UCNs are coated with polyethylenimine (PEI), which render them hydrophilic and easier to manipulate. These UCNs showed antiviral activity against Dengue virus serotype 2 and adenovirus type 5, which were used as models of enveloped and non-enveloped viruses, respectively.110 MXenes111,112 are a large family of 2D transition metal carbides, nitrides,113 and carbonitrides114 that exhibit unique electronic, optical, and catalytic properties. They have the general formula Mn+1XnTx, where M is an early transition metal (Ti, Zr, V, Mo, etc.), X is C and/or N, Tx represents the surface functional groups (=O, −OH, −F, −Cl), and n = 1–4.115,116 Some examples include Ti3C2Tx, V2CTx, and Nb2CTx, with over 30 stoichiometric compositions already synthesized with more than 100 predicted. Biocompatible MXenes, such as Ti3C2Tx, are hydrophilic and are among the most efficient light-to-heat transforming materials.117 The plasmon resonance extinction maxima of Ti3C2Tx is at 780 nm, enabling the use of near-infrared (IR) light for PDT. Several other MXenes have absorption maxima in the IR range and have shown outstanding performance in PDT and theranostic applications.118\nFullerene and graphene are also good candidates for virus inactivation by PDT and have proved to be effective against Semliki Forest virus (SFV), vesicular stomatitis virus (VSV), HSV-1, HIV-1, mosquito iridovirus (MIV), and influenza A virus (IAV), as well as the phage MS2.107 In addition, several 2D nanomaterials, including graphene-based materials, MXenes, black phosphorus, graphitic carbon nitride, tungsten disulfide, and molybdenum disulfide, have been reported to improve the efficacy of PDT considerably for cancer treatment.56,57 Therefore, determining if such nanomaterial-based PDT protocols could be exploited to inactivate SARS-CoV-2 is of great interest.\n\nBiomimetic Engineering of Nanodelivery Systems: Artificial Viruses in the Making\nIn an effort to engineer the next generation of nanoscale vectors, scientists have moved from using inorganic components aimed at obtaining inert structures to utilizing biological building blocks that are able to convey additional functionalities to the resulting construct. To cope with the complexity of the body and to evade the multiple layers of defense that tissues and organs have, it is critical to rely on the ability of certain materials to interact with, rather than to eschew, the biology of our body. Every NP system conceived to date faces one common fate: whether injected, inhaled, ingested, or absorbed through our epithelia, all will at some point come into contact with the mixture of fluids and organic compounds that comprise the body. Under such conditions, every material reacts in a unique way according to the conditions they individually face (i.e., the tissue or body region they are in), their composition (i.e., organic or inorganic), and their physical properties (i.e., size, shape, surface charge).119 Inorganic NPs can function as globular protein mimics because of their similar size, charge, shape, and surface features that can be chemically functionalized to resemble proteins. These similarities can be used in biotechnology to control virus pathways or cell receptor interactions with NPs.120\nAmong the many attempts, the legendary accomplishments, and the epic failures, we could list thousands of different NPs, differing from one another thanks to the creative endeavors of their designers. Despite their remarkable differences and researchers’ endeavors to make them one of a kind, they all aim to achieve one goal: to deliver in a specific way one particular form of payload, while remaining as unnoticed as possible by the body’s defense mechanisms. For everyone who has made an effort to create their own version of such a silver bullet, it has come to mind that nature in its incredible variety had already invented a few ingenious solutions to this problem. In the world of nano-based drug delivery, one entity dominates as the quintessential example of precision, efficiency, and stealth: the virus. Not by chance, these two worlds share many features that span from the physical laws that govern their assembly and stability to the chemical similarities in their overall composition.121\nHistorically, the field of nanomedicine and the global endeavor to generate NPs for drug delivery arose from one of the greatest struggles in medicine: gene therapy. At the core of that approach was the idea that viruses could be used as Trojan horses to deliver the correct sequence of the gene into the cells that harbored the mutated copy. In order to identify the virus that offered the best delivery service, a cadre of viral strains and species were tested, adapted, and engineered to fit the aims and hit the targets. It took decades of trial and error, of progressive adjustments, and a few tragic mistakes to identify the viruses that could provide the ideal backbone to develop viral vectors able to ferry genetic cargo into the target cell.122 In the midst of that global challenge, nanotechnology offered a safer and more controllable alternative: to generate bespoke structures that could replace viral vectors and do the same job, delivering a payload from the point of injection to the site of action.\nFast forward a few decades, and the promises made by both worlds seem to have finally become reality, with a number of active clinical trials, some clinical success stories, and a few commercial products in the market space. Interestingly, the two worlds seem to have maintained a safe distance so as to avoid any collision or dangerous proximity. Exchange of ideas between the fields of gene therapy and nanomedicine is limited, and the potential for disciplinary growth through knowledge exchange has remained elusive to scientists in both fields.5 Many scientists have suggested that nanotechnologists could find inspiration in the mechanisms devised by viruses to elude immune surveillance, to overcome biological barriers, and to deliver their genetic payload with high specificity. Similarly, gene therapists would find high-tech solutions to their scalability and safety issues in looking at the new generations of biomimetic particles being generated.\nThe principles of biomimicry and bioinspiration have been used to design and to engineer drug-delivery technologies that reproduce or recapitulate biological materials, for what pertains to not only their structure and chemistry but also, more importantly, their functions. In drug delivery, surface recognition and nanoscale interactions between materials and biological entities are key to the success of the delivery strategy, and the use of biological building blocks such as membrane proteins has been proposed as a way to convey targeting and shielding moieties simultaneously.123 One can only hope that the recent events and global attention that viruses are capturing in the scientific world will spur a renewed interest in finding ways to adapt viral features and mechanisms of action to the world of NPs. Increased focus in this field would be useful to create virus-like NPs able to circulate in the blood system, overcoming the endothelial barrier, and to deliver their therapeutic payload with high efficiency.124\n\nInterference with Cellular Uptake, Immobilization, and Inactivation of the Virus Outside of the Host Cell\nNanomaterials can be synthesized with a high specific surface area of a few hundred square meters per gram. Therefore, dependent on the surface properties, nanomaterials efficiently adsorb biomolecules and form a so-called biomolecular corona. This passive, nontargeted adsorption might be utilized to bind viruses, provided that the selected nanomaterial is relatively biocompatible. Viral surface proteins are often modified by sugar moieties or encompass positively charged amino acid patches that bind to lectins or glycosaminoglycans (GAGs) of heparan sulfate (HS), respectively.32 Robust interactions of virus particles with these host receptors is ensured by multivalent binding, which is likely why single-molecule inhibitors often are not capable of efficiently perturbing this key event but multivalent NPs are superior to block binding of different viruses to the host cell.125\nGold NPs capped with mercaptoethanesulfonate are effective inhibitors of HSV type 1 infection as they mimic cell-surface-receptor heparan sulfate and, therefore, competitively bind to the virus. Interestingly, polyvalent sulfated Au NPs inhibit virus binding to the host cell dependent on their size. Nanoparticles of diameters equal to and larger than the virus diameter (in this case, the stomatitis virus) more efficiently inhibit the binding to cells than smaller particles. Most likely, larger NPs efficiently cross-link virions, whereas smaller NPs simply decorate the viral surface.126 Papp et al. found that gold NPs decorated with SA effectively inhibited the binding of influenza virus to the target cells. In this case, viral recognition via its surface protein hemagglutinin of SA on the host cell membrane was a prerequisite for cellular entry.127 More recently, Cagno et al. reported antiviral NPs (Au and iron oxide core) with long and flexible linkers mimicking HS that strongly bind and inactivate viruses such as respiratory syncytial virus in vitro and in vivo in a lung infection model and even led to irreversible viral deformation.53 Hence, there is ample evidence that biocompatible, functionalized NPs can act as broad-spectrum antivirals. Notably, the receptor-binding domain of the spike S1 protein of SARS-CoV-2 binds not only to ACE2 but also to heparin128 and, thus, might be targeted by similar approaches as outlined above.\nOne of the most recent strategies to inhibit viral uptake is based on administration of recombinant ACE2 to inhibit binding competitively via the spike S1 protein.21 Knowing that multivalency is key to block virus–host interactions reliably, researchers have speculated that a nanostructured carrier could not only improve delivery and cargo stability but also might dramatically enhance binding strength.129\n\nSpeeding up the Nanomedicine-Based Approaches for COVID-19 by In Silico Analysis\nCurrently, repurposing drug molecules is a key strategy for identifying approved or investigational drugs outside the scope of the original medical indication that can be used to fight COVID-19.130,131 There are various advantages to this strategy, including already-established safety profiles, fast transition to clinical studies, and less investment needed, compared to the process of developing an entirely new drug.132,133 Therefore, these advantages have the potential to result in less risky and more rapid returns on investment in the development of repurposed drugs, benefits that are particularly important during pandemics. In addition to rapid diagnosis, making recommendations to physicians about rapid treatment methods can save the lives of many people. Combining in silico tools such as molecular docking, molecular dynamics, and computational chemistry with large drug databases provides a great advantage in selecting “possible candidates” from among thousands of pharmaceutically active substances (Figure 3). In silico analyses should be supported by the literature, expert opinions (pharmacologists and clinicians), and, when possible, preclinical and clinical findings. After selecting the best candidates following in silico analyses, detailed in vitro and in vivo experimentation should be undertaken prior to clinical studies. Recently, various drug-repurposing studies have been published to assist in the global COVID-19 pandemic response.134−136 Such studies aim to identify whether already-approved drugs have the capacity to interact with viral proteins or receptors on host cells.\nFigure 3 Schematic of how in silico analysis can be used to select candidate drug molecules for clinical studies. MD, molecular dynamics. In nanomedicine, computational models have recently garnered attention; such work may help to identify how nanomaterials interact with biological systems and to determine how the efficacy of these nanotherapeutics could be improved. Computational models indicate how NPs are taken up by healthy cells or tumor cells, enabling better predictions regarding the pharmacokinetic and pharmacodynamic properties of these materials.134 For example, Lunnoo et al. used a coarse-grained molecular dynamics (MD) simulation to observe the internalization pathways of various Au nanostructures (nanospheres, nanocages, nanorods, nanoplates, and nanohexapods) into an idealized mammalian plasma membrane.137 Other studies have simulated how different NPs can target tumor cells and deliver drugs.138,139 Therefore, in silico approaches that are currently used for drug repurposing, including molecular docking, molecular dynamics, and computational chemistry, constitute valuable tools to aid preclinical and clinical studies of nanomaterials directed for disease treatment. Considering the urgent need for nanomedicine against the current pandemic, in silico analyses may be especially useful in guiding the rational design of new NP formulations required to fight SARS-CoV-2."}
LitCovid-PubTator
{"project":"LitCovid-PubTator","denotations":[{"id":"195","span":{"begin":35,"end":45},"obj":"Species"},{"id":"200","span":{"begin":274,"end":278},"obj":"Gene"},{"id":"201","span":{"begin":77,"end":87},"obj":"Species"},{"id":"202","span":{"begin":95,"end":106},"obj":"Species"},{"id":"203","span":{"begin":174,"end":182},"obj":"Disease"},{"id":"205","span":{"begin":713,"end":717},"obj":"Gene"},{"id":"217","span":{"begin":2126,"end":2130},"obj":"Gene"},{"id":"218","span":{"begin":2089,"end":2093},"obj":"Gene"},{"id":"219","span":{"begin":1320,"end":1330},"obj":"Species"},{"id":"220","span":{"begin":1811,"end":1821},"obj":"Species"},{"id":"221","span":{"begin":2012,"end":2023},"obj":"Species"},{"id":"222","span":{"begin":1992,"end":1995},"obj":"Chemical"},{"id":"223","span":{"begin":2039,"end":2042},"obj":"Chemical"},{"id":"224","span":{"begin":2058,"end":2073},"obj":"Chemical"},{"id":"225","span":{"begin":2100,"end":2112},"obj":"Chemical"},{"id":"226","span":{"begin":2114,"end":2116},"obj":"Chemical"},{"id":"227","span":{"begin":2135,"end":2137},"obj":"Chemical"},{"id":"238","span":{"begin":3105,"end":3109},"obj":"Gene"},{"id":"239","span":{"begin":3059,"end":3060},"obj":"Gene"},{"id":"240","span":{"begin":3061,"end":3066},"obj":"Gene"},{"id":"241","span":{"begin":2993,"end":3003},"obj":"Species"},{"id":"242","span":{"begin":3295,"end":3303},"obj":"Species"},{"id":"243","span":{"begin":3619,"end":3632},"obj":"Chemical"},{"id":"244","span":{"begin":3273,"end":3281},"obj":"Disease"},{"id":"245","span":{"begin":3846,"end":3854},"obj":"Disease"},{"id":"246","span":{"begin":4169,"end":4175},"obj":"Disease"},{"id":"247","span":{"begin":4316,"end":4335},"obj":"Disease"},{"id":"252","span":{"begin":4453,"end":4463},"obj":"Species"},{"id":"253","span":{"begin":4692,"end":4697},"obj":"Species"},{"id":"254","span":{"begin":4560,"end":4568},"obj":"Disease"},{"id":"255","span":{"begin":4817,"end":4825},"obj":"Disease"},{"id":"258","span":{"begin":5678,"end":5683},"obj":"Gene"},{"id":"259","span":{"begin":5754,"end":5762},"obj":"Species"},{"id":"261","span":{"begin":5987,"end":5994},"obj":"Species"},{"id":"266","span":{"begin":6221,"end":6226},"obj":"Chemical"},{"id":"267","span":{"begin":6690,"end":6695},"obj":"Chemical"},{"id":"268","span":{"begin":6700,"end":6705},"obj":"Chemical"},{"id":"269","span":{"begin":7448,"end":7463},"obj":"Disease"},{"id":"273","span":{"begin":7553,"end":7558},"obj":"Gene"},{"id":"274","span":{"begin":7521,"end":7531},"obj":"Species"},{"id":"275","span":{"begin":7718,"end":7723},"obj":"Disease"},{"id":"284","span":{"begin":8558,"end":8568},"obj":"Gene"},{"id":"285","span":{"begin":8039,"end":8040},"obj":"Gene"},{"id":"286","span":{"begin":8092,"end":8104},"obj":"Species"},{"id":"287","span":{"begin":8311,"end":8323},"obj":"Species"},{"id":"288","span":{"begin":8695,"end":8699},"obj":"Species"},{"id":"289","span":{"begin":8704,"end":8715},"obj":"Species"},{"id":"290","span":{"begin":8820,"end":8826},"obj":"Species"},{"id":"291","span":{"begin":8187,"end":8192},"obj":"Chemical"},{"id":"308","span":{"begin":9949,"end":9952},"obj":"Gene"},{"id":"309","span":{"begin":9934,"end":9939},"obj":"Gene"},{"id":"310","span":{"begin":9162,"end":9167},"obj":"Gene"},{"id":"311","span":{"begin":9988,"end":9998},"obj":"Gene"},{"id":"312","span":{"begin":8982,"end":8988},"obj":"Species"},{"id":"313","span":{"begin":9096,"end":9117},"obj":"Species"},{"id":"314","span":{"begin":9151,"end":9161},"obj":"Species"},{"id":"315","span":{"begin":9177,"end":9187},"obj":"Species"},{"id":"316","span":{"begin":9357,"end":9372},"obj":"Species"},{"id":"317","span":{"begin":9398,"end":9408},"obj":"Species"},{"id":"318","span":{"begin":9883,"end":9900},"obj":"Species"},{"id":"319","span":{"begin":9923,"end":9933},"obj":"Species"},{"id":"320","span":{"begin":9953,"end":9957},"obj":"Species"},{"id":"321","span":{"begin":8860,"end":8868},"obj":"Disease"},{"id":"322","span":{"begin":9444,"end":9454},"obj":"Disease"},{"id":"323","span":{"begin":9598,"end":9617},"obj":"Disease"},{"id":"327","span":{"begin":10227,"end":10233},"obj":"Species"},{"id":"328","span":{"begin":10671,"end":10683},"obj":"Species"},{"id":"329","span":{"begin":10345,"end":10356},"obj":"Species"},{"id":"336","span":{"begin":11414,"end":11424},"obj":"Gene"},{"id":"337","span":{"begin":11220,"end":11230},"obj":"Species"},{"id":"338","span":{"begin":11783,"end":11798},"obj":"Species"},{"id":"339","span":{"begin":11630,"end":11643},"obj":"Chemical"},{"id":"340","span":{"begin":11645,"end":11649},"obj":"Chemical"},{"id":"341","span":{"begin":11045,"end":11064},"obj":"Disease"},{"id":"347","span":{"begin":12236,"end":12244},"obj":"Species"},{"id":"348","span":{"begin":12415,"end":12425},"obj":"Species"},{"id":"349","span":{"begin":12688,"end":12695},"obj":"Species"},{"id":"350","span":{"begin":12705,"end":12715},"obj":"Species"},{"id":"351","span":{"begin":12146,"end":12155},"obj":"Disease"},{"id":"366","span":{"begin":13886,"end":13906},"obj":"Gene"},{"id":"367","span":{"begin":13959,"end":13964},"obj":"Gene"},{"id":"368","span":{"begin":14028,"end":14049},"obj":"Species"},{"id":"369","span":{"begin":13227,"end":13236},"obj":"Species"},{"id":"370","span":{"begin":13323,"end":13332},"obj":"Species"},{"id":"371","span":{"begin":13460,"end":13469},"obj":"Species"},{"id":"372","span":{"begin":13589,"end":13598},"obj":"Species"},{"id":"373","span":{"begin":13112,"end":13115},"obj":"Chemical"},{"id":"374","span":{"begin":13119,"end":13124},"obj":"Chemical"},{"id":"375","span":{"begin":13137,"end":13149},"obj":"Chemical"},{"id":"376","span":{"begin":13154,"end":13168},"obj":"Chemical"},{"id":"377","span":{"begin":12995,"end":13002},"obj":"Disease"},{"id":"378","span":{"begin":13564,"end":13566},"obj":"Disease"},{"id":"379","span":{"begin":14012,"end":14023},"obj":"Disease"},{"id":"381","span":{"begin":14503,"end":14513},"obj":"Species"},{"id":"395","span":{"begin":14951,"end":14956},"obj":"Gene"},{"id":"396","span":{"begin":14957,"end":14961},"obj":"Gene"},{"id":"397","span":{"begin":15311,"end":15316},"obj":"Gene"},{"id":"398","span":{"begin":15317,"end":15321},"obj":"Gene"},{"id":"399","span":{"begin":15366,"end":15374},"obj":"Species"},{"id":"400","span":{"begin":15379,"end":15387},"obj":"Species"},{"id":"401","span":{"begin":15513,"end":15523},"obj":"Species"},{"id":"402","span":{"begin":14684,"end":14692},"obj":"Chemical"},{"id":"403","span":{"begin":14712,"end":14718},"obj":"Chemical"},{"id":"404","span":{"begin":14736,"end":14747},"obj":"Chemical"},{"id":"405","span":{"begin":14872,"end":14886},"obj":"Chemical"},{"id":"406","span":{"begin":15159,"end":15167},"obj":"Disease"},{"id":"407","span":{"begin":15475,"end":15484},"obj":"Disease"},{"id":"413","span":{"begin":15944,"end":15959},"obj":"Gene"},{"id":"414","span":{"begin":16105,"end":16115},"obj":"Species"},{"id":"415","span":{"begin":16166,"end":16176},"obj":"Species"},{"id":"416","span":{"begin":16231,"end":16238},"obj":"Chemical"},{"id":"417","span":{"begin":15660,"end":15683},"obj":"Disease"},{"id":"419","span":{"begin":16426,"end":16436},"obj":"Species"},{"id":"427","span":{"begin":17034,"end":17039},"obj":"Gene"},{"id":"428","span":{"begin":17065,"end":17070},"obj":"Gene"},{"id":"429","span":{"begin":17139,"end":17144},"obj":"Species"},{"id":"430","span":{"begin":16996,"end":16998},"obj":"Chemical"},{"id":"431","span":{"begin":17098,"end":17102},"obj":"Chemical"},{"id":"432","span":{"begin":17297,"end":17299},"obj":"Chemical"},{"id":"433","span":{"begin":17304,"end":17308},"obj":"Chemical"},{"id":"441","span":{"begin":17924,"end":17935},"obj":"Species"},{"id":"442","span":{"begin":17616,"end":17626},"obj":"Chemical"},{"id":"443","span":{"begin":17862,"end":17872},"obj":"Chemical"},{"id":"444","span":{"begin":17671,"end":17679},"obj":"Disease"},{"id":"445","span":{"begin":17772,"end":17780},"obj":"Disease"},{"id":"446","span":{"begin":17906,"end":17922},"obj":"Disease"},{"id":"447","span":{"begin":18040,"end":18048},"obj":"Disease"},{"id":"464","span":{"begin":18653,"end":18671},"obj":"Gene"},{"id":"465","span":{"begin":18806,"end":18810},"obj":"Gene"},{"id":"466","span":{"begin":18833,"end":18837},"obj":"Gene"},{"id":"467","span":{"begin":18875,"end":18879},"obj":"Gene"},{"id":"468","span":{"begin":18382,"end":18389},"obj":"Species"},{"id":"469","span":{"begin":18614,"end":18622},"obj":"Species"},{"id":"470","span":{"begin":18696,"end":18704},"obj":"Species"},{"id":"471","span":{"begin":18812,"end":18823},"obj":"Chemical"},{"id":"472","span":{"begin":18861,"end":18870},"obj":"Chemical"},{"id":"473","span":{"begin":18888,"end":18898},"obj":"Chemical"},{"id":"474","span":{"begin":18186,"end":18194},"obj":"Disease"},{"id":"475","span":{"begin":18287,"end":18290},"obj":"Disease"},{"id":"476","span":{"begin":18464,"end":18499},"obj":"Disease"},{"id":"477","span":{"begin":18501,"end":18505},"obj":"Disease"},{"id":"478","span":{"begin":18537,"end":18559},"obj":"Disease"},{"id":"479","span":{"begin":18596,"end":18601},"obj":"Disease"},{"id":"481","span":{"begin":19042,"end":19051},"obj":"Disease"},{"id":"505","span":{"begin":20095,"end":20099},"obj":"Gene"},{"id":"506","span":{"begin":20111,"end":20115},"obj":"Gene"},{"id":"507","span":{"begin":20116,"end":20121},"obj":"Gene"},{"id":"508","span":{"begin":20215,"end":20219},"obj":"Gene"},{"id":"509","span":{"begin":20557,"end":20562},"obj":"Gene"},{"id":"510","span":{"begin":21494,"end":21498},"obj":"Gene"},{"id":"511","span":{"begin":21503,"end":21532},"obj":"Gene"},{"id":"512","span":{"begin":20390,"end":20398},"obj":"Species"},{"id":"513","span":{"begin":20478,"end":20488},"obj":"Species"},{"id":"514","span":{"begin":20721,"end":20729},"obj":"Species"},{"id":"515","span":{"begin":21336,"end":21341},"obj":"Species"},{"id":"516","span":{"begin":21536,"end":21540},"obj":"Species"},{"id":"517","span":{"begin":20146,"end":20156},"obj":"Species"},{"id":"518","span":{"begin":21208,"end":21222},"obj":"Chemical"},{"id":"519","span":{"begin":21242,"end":21255},"obj":"Chemical"},{"id":"520","span":{"begin":20069,"end":20077},"obj":"Disease"},{"id":"521","span":{"begin":20236,"end":20244},"obj":"Disease"},{"id":"522","span":{"begin":20403,"end":20407},"obj":"Disease"},{"id":"523","span":{"begin":20413,"end":20421},"obj":"Disease"},{"id":"524","span":{"begin":20466,"end":20474},"obj":"Disease"},{"id":"525","span":{"begin":20605,"end":20617},"obj":"Disease"},{"id":"526","span":{"begin":20734,"end":20738},"obj":"Disease"},{"id":"527","span":{"begin":20744,"end":20752},"obj":"Disease"},{"id":"535","span":{"begin":21862,"end":21870},"obj":"Species"},{"id":"536","span":{"begin":21671,"end":21685},"obj":"Chemical"},{"id":"537","span":{"begin":21763,"end":21769},"obj":"Disease"},{"id":"538","span":{"begin":21808,"end":21818},"obj":"Disease"},{"id":"539","span":{"begin":21880,"end":21885},"obj":"Disease"},{"id":"540","span":{"begin":21907,"end":21916},"obj":"Disease"},{"id":"541","span":{"begin":21934,"end":21954},"obj":"Disease"},{"id":"554","span":{"begin":22698,"end":22702},"obj":"Gene"},{"id":"555","span":{"begin":22738,"end":22743},"obj":"Gene"},{"id":"556","span":{"begin":22360,"end":22368},"obj":"Species"},{"id":"557","span":{"begin":23252,"end":23257},"obj":"Species"},{"id":"558","span":{"begin":22472,"end":22479},"obj":"Chemical"},{"id":"559","span":{"begin":22600,"end":22606},"obj":"Chemical"},{"id":"560","span":{"begin":22869,"end":22886},"obj":"Chemical"},{"id":"561","span":{"begin":22978,"end":22986},"obj":"Chemical"},{"id":"562","span":{"begin":22386,"end":22392},"obj":"Disease"},{"id":"563","span":{"begin":22812,"end":22818},"obj":"Disease"},{"id":"564","span":{"begin":23108,"end":23111},"obj":"Disease"},{"id":"565","span":{"begin":23127,"end":23136},"obj":"Disease"},{"id":"575","span":{"begin":23454,"end":23464},"obj":"Species"},{"id":"576","span":{"begin":24194,"end":24215},"obj":"Species"},{"id":"577","span":{"begin":24221,"end":24249},"obj":"Species"},{"id":"578","span":{"begin":23693,"end":23716},"obj":"Chemical"},{"id":"579","span":{"begin":23718,"end":23721},"obj":"Chemical"},{"id":"580","span":{"begin":23742,"end":23748},"obj":"Chemical"},{"id":"581","span":{"begin":23986,"end":23989},"obj":"Chemical"},{"id":"582","span":{"begin":24057,"end":24063},"obj":"Chemical"},{"id":"583","span":{"begin":23783,"end":23788},"obj":"Disease"},{"id":"598","span":{"begin":24531,"end":24534},"obj":"Gene"},{"id":"599","span":{"begin":24922,"end":24934},"obj":"Species"},{"id":"600","span":{"begin":24950,"end":24967},"obj":"Species"},{"id":"601","span":{"begin":24639,"end":24662},"obj":"Chemical"},{"id":"602","span":{"begin":24664,"end":24669},"obj":"Chemical"},{"id":"603","span":{"begin":24700,"end":24719},"obj":"Chemical"},{"id":"604","span":{"begin":24797,"end":24813},"obj":"Chemical"},{"id":"605","span":{"begin":24815,"end":24818},"obj":"Chemical"},{"id":"606","span":{"begin":25052,"end":25058},"obj":"Chemical"},{"id":"607","span":{"begin":25088,"end":25116},"obj":"Chemical"},{"id":"608","span":{"begin":25118,"end":25126},"obj":"Chemical"},{"id":"609","span":{"begin":25135,"end":25148},"obj":"Chemical"},{"id":"610","span":{"begin":25290,"end":25295},"obj":"Chemical"},{"id":"611","span":{"begin":25391,"end":25392},"obj":"Chemical"},{"id":"630","span":{"begin":26066,"end":26086},"obj":"Species"},{"id":"631","span":{"begin":26094,"end":26120},"obj":"Species"},{"id":"632","span":{"begin":26128,"end":26133},"obj":"Species"},{"id":"633","span":{"begin":26135,"end":26140},"obj":"Species"},{"id":"634","span":{"begin":26173,"end":26190},"obj":"Species"},{"id":"635","span":{"begin":26213,"end":26222},"obj":"Species"},{"id":"636","span":{"begin":26587,"end":26597},"obj":"Species"},{"id":"637","span":{"begin":26088,"end":26091},"obj":"Species"},{"id":"638","span":{"begin":26122,"end":26125},"obj":"Species"},{"id":"639","span":{"begin":26192,"end":26195},"obj":"Species"},{"id":"640","span":{"begin":25948,"end":25957},"obj":"Chemical"},{"id":"641","span":{"begin":25962,"end":25970},"obj":"Chemical"},{"id":"642","span":{"begin":26276,"end":26284},"obj":"Chemical"},{"id":"643","span":{"begin":26302,"end":26308},"obj":"Chemical"},{"id":"644","span":{"begin":26310,"end":26326},"obj":"Chemical"},{"id":"645","span":{"begin":26354,"end":26372},"obj":"Chemical"},{"id":"646","span":{"begin":26378,"end":26398},"obj":"Chemical"},{"id":"647","span":{"begin":26467,"end":26473},"obj":"Disease"},{"id":"649","span":{"begin":29285,"end":29291},"obj":"Species"},{"id":"654","span":{"begin":32581,"end":32586},"obj":"Chemical"},{"id":"655","span":{"begin":32671,"end":32689},"obj":"Chemical"},{"id":"656","span":{"begin":32700,"end":32715},"obj":"Chemical"},{"id":"657","span":{"begin":32717,"end":32719},"obj":"Chemical"},{"id":"673","span":{"begin":33148,"end":33169},"obj":"Gene"},{"id":"674","span":{"begin":34397,"end":34401},"obj":"Gene"},{"id":"675","span":{"begin":33720,"end":33735},"obj":"Species"},{"id":"676","span":{"begin":34071,"end":34098},"obj":"Species"},{"id":"677","span":{"begin":34368,"end":34378},"obj":"Species"},{"id":"678","span":{"begin":33061,"end":33084},"obj":"Chemical"},{"id":"679","span":{"begin":33170,"end":33185},"obj":"Chemical"},{"id":"680","span":{"begin":33680,"end":33682},"obj":"Chemical"},{"id":"681","span":{"begin":33830,"end":33832},"obj":"Chemical"},{"id":"682","span":{"begin":33960,"end":33970},"obj":"Chemical"},{"id":"683","span":{"begin":34414,"end":34421},"obj":"Chemical"},{"id":"684","span":{"begin":33113,"end":33133},"obj":"Disease"},{"id":"685","span":{"begin":33431,"end":33447},"obj":"Disease"},{"id":"686","span":{"begin":34125,"end":34139},"obj":"Disease"},{"id":"687","span":{"begin":34175,"end":34192},"obj":"Disease"},{"id":"689","span":{"begin":34595,"end":34599},"obj":"Gene"},{"id":"691","span":{"begin":34955,"end":34963},"obj":"Disease"},{"id":"695","span":{"begin":35747,"end":35753},"obj":"Species"},{"id":"696","span":{"begin":35171,"end":35179},"obj":"Disease"},{"id":"697","span":{"begin":36425,"end":36433},"obj":"Disease"},{"id":"703","span":{"begin":37402,"end":37411},"obj":"Species"},{"id":"704","span":{"begin":37990,"end":38000},"obj":"Species"},{"id":"705","span":{"begin":37301,"end":37303},"obj":"Chemical"},{"id":"706","span":{"begin":37041,"end":37046},"obj":"Disease"},{"id":"707","span":{"begin":37490,"end":37495},"obj":"Disease"}],"attributes":[{"id":"A195","pred":"tao:has_database_id","subj":"195","obj":"Tax:2697049"},{"id":"A200","pred":"tao:has_database_id","subj":"200","obj":"Gene:59272"},{"id":"A201","pred":"tao:has_database_id","subj":"201","obj":"Tax:2697049"},{"id":"A202","pred":"tao:has_database_id","subj":"202","obj":"Tax:12814"},{"id":"A203","pred":"tao:has_database_id","subj":"203","obj":"MESH:D007239"},{"id":"A205","pred":"tao:has_database_id","subj":"205","obj":"Gene:59272"},{"id":"A217","pred":"tao:has_database_id","subj":"217","obj":"Gene:6383"},{"id":"A218","pred":"tao:has_database_id","subj":"218","obj":"Gene:6383"},{"id":"A219","pred":"tao:has_database_id","subj":"219","obj":"Tax:2697049"},{"id":"A220","pred":"tao:has_database_id","subj":"220","obj":"Tax:2697049"},{"id":"A221","pred":"tao:has_database_id","subj":"221","obj":"Tax:12814"},{"id":"A224","pred":"tao:has_database_id","subj":"224","obj":"MESH:D006497"},{"id":"A225","pred":"tao:has_database_id","subj":"225","obj":"MESH:D012794"},{"id":"A226","pred":"tao:has_database_id","subj":"226","obj":"MESH:D012794"},{"id":"A227","pred":"tao:has_database_id","subj":"227","obj":"MESH:D012794"},{"id":"A238","pred":"tao:has_database_id","subj":"238","obj":"Gene:59272"},{"id":"A239","pred":"tao:has_database_id","subj":"239","obj":"Gene:43740568"},{"id":"A240","pred":"tao:has_database_id","subj":"240","obj":"Gene:43740568"},{"id":"A241","pred":"tao:has_database_id","subj":"241","obj":"Tax:2697049"},{"id":"A242","pred":"tao:has_database_id","subj":"242","obj":"Tax:9606"},{"id":"A243","pred":"tao:has_database_id","subj":"243","obj":"MESH:D003505"},{"id":"A244","pred":"tao:has_database_id","subj":"244","obj":"MESH:D064420"},{"id":"A245","pred":"tao:has_database_id","subj":"245","obj":"MESH:C000657245"},{"id":"A246","pred":"tao:has_database_id","subj":"246","obj":"MESH:D009369"},{"id":"A247","pred":"tao:has_database_id","subj":"247","obj":"MESH:D003141"},{"id":"A252","pred":"tao:has_database_id","subj":"252","obj":"Tax:2697049"},{"id":"A253","pred":"tao:has_database_id","subj":"253","obj":"Tax:9606"},{"id":"A254","pred":"tao:has_database_id","subj":"254","obj":"MESH:C000657245"},{"id":"A255","pred":"tao:has_database_id","subj":"255","obj":"MESH:C000657245"},{"id":"A258","pred":"tao:has_database_id","subj":"258","obj":"Gene:43740568"},{"id":"A259","pred":"tao:has_database_id","subj":"259","obj":"Tax:9606"},{"id":"A261","pred":"tao:has_database_id","subj":"261","obj":"Tax:9606"},{"id":"A266","pred":"tao:has_database_id","subj":"266","obj":"MESH:D008055"},{"id":"A267","pred":"tao:has_database_id","subj":"267","obj":"MESH:D008055"},{"id":"A268","pred":"tao:has_database_id","subj":"268","obj":"MESH:D008055"},{"id":"A269","pred":"tao:has_database_id","subj":"269","obj":"MESH:D001102"},{"id":"A273","pred":"tao:has_database_id","subj":"273","obj":"Gene:43740568"},{"id":"A274","pred":"tao:has_database_id","subj":"274","obj":"Tax:2697049"},{"id":"A275","pred":"tao:has_database_id","subj":"275","obj":"MESH:D009369"},{"id":"A285","pred":"tao:has_database_id","subj":"285","obj":"Gene:43740568"},{"id":"A286","pred":"tao:has_database_id","subj":"286","obj":"Tax:9606"},{"id":"A287","pred":"tao:has_database_id","subj":"287","obj":"Tax:9606"},{"id":"A288","pred":"tao:has_database_id","subj":"288","obj":"Tax:10090"},{"id":"A289","pred":"tao:has_database_id","subj":"289","obj":"Tax:10141"},{"id":"A290","pred":"tao:has_database_id","subj":"290","obj":"Tax:9606"},{"id":"A291","pred":"tao:has_database_id","subj":"291","obj":"MESH:D008055"},{"id":"A308","pred":"tao:has_database_id","subj":"308","obj":"Gene:8081"},{"id":"A309","pred":"tao:has_database_id","subj":"309","obj":"Gene:43740568"},{"id":"A310","pred":"tao:has_database_id","subj":"310","obj":"Gene:43740568"},{"id":"A312","pred":"tao:has_database_id","subj":"312","obj":"Tax:9606"},{"id":"A313","pred":"tao:has_database_id","subj":"313","obj":"Tax:310542"},{"id":"A314","pred":"tao:has_database_id","subj":"314","obj":"Tax:2697049"},{"id":"A315","pred":"tao:has_database_id","subj":"315","obj":"Tax:9598"},{"id":"A316","pred":"tao:has_database_id","subj":"316","obj":"Tax:9544"},{"id":"A317","pred":"tao:has_database_id","subj":"317","obj":"Tax:2697049"},{"id":"A318","pred":"tao:has_database_id","subj":"318","obj":"Tax:28285"},{"id":"A319","pred":"tao:has_database_id","subj":"319","obj":"Tax:2697049"},{"id":"A320","pred":"tao:has_database_id","subj":"320","obj":"Tax:2697049"},{"id":"A321","pred":"tao:has_database_id","subj":"321","obj":"MESH:C000657245"},{"id":"A322","pred":"tao:has_database_id","subj":"322","obj":"MESH:D007239"},{"id":"A323","pred":"tao:has_database_id","subj":"323","obj":"MESH:D012140"},{"id":"A327","pred":"tao:has_database_id","subj":"327","obj":"Tax:9606"},{"id":"A328","pred":"tao:has_database_id","subj":"328","obj":"Tax:9606"},{"id":"A329","pred":"tao:has_database_id","subj":"329","obj":"Tax:12814"},{"id":"A337","pred":"tao:has_database_id","subj":"337","obj":"Tax:2697049"},{"id":"A338","pred":"tao:has_database_id","subj":"338","obj":"Tax:9544"},{"id":"A341","pred":"tao:has_database_id","subj":"341","obj":"MESH:D003141"},{"id":"A347","pred":"tao:has_database_id","subj":"347","obj":"Tax:694009"},{"id":"A348","pred":"tao:has_database_id","subj":"348","obj":"Tax:2697049"},{"id":"A349","pred":"tao:has_database_id","subj":"349","obj":"Tax:9606"},{"id":"A350","pred":"tao:has_database_id","subj":"350","obj":"Tax:2697049"},{"id":"A351","pred":"tao:has_database_id","subj":"351","obj":"MESH:D007239"},{"id":"A366","pred":"tao:has_database_id","subj":"366","obj":"Gene:7099"},{"id":"A367","pred":"tao:has_database_id","subj":"367","obj":"Gene:114548"},{"id":"A368","pred":"tao:has_database_id","subj":"368","obj":"Tax:10566"},{"id":"A369","pred":"tao:has_database_id","subj":"369","obj":"Tax:11320"},{"id":"A370","pred":"tao:has_database_id","subj":"370","obj":"Tax:11320"},{"id":"A371","pred":"tao:has_database_id","subj":"371","obj":"Tax:11320"},{"id":"A372","pred":"tao:has_database_id","subj":"372","obj":"Tax:11320"},{"id":"A373","pred":"tao:has_database_id","subj":"373","obj":"MESH:D009821"},{"id":"A374","pred":"tao:has_database_id","subj":"374","obj":"MESH:D014867"},{"id":"A375","pred":"tao:has_database_id","subj":"375","obj":"MESH:D013185"},{"id":"A376","pred":"tao:has_database_id","subj":"376","obj":"MESH:D011136"},{"id":"A377","pred":"tao:has_database_id","subj":"377","obj":"MESH:D013746"},{"id":"A378","pred":"tao:has_database_id","subj":"378","obj":"MESH:D001281"},{"id":"A379","pred":"tao:has_database_id","subj":"379","obj":"MESH:D006509"},{"id":"A381","pred":"tao:has_database_id","subj":"381","obj":"Tax:2697049"},{"id":"A395","pred":"tao:has_database_id","subj":"395","obj":"Gene:6772"},{"id":"A396","pred":"tao:has_database_id","subj":"396","obj":"Gene:3659"},{"id":"A397","pred":"tao:has_database_id","subj":"397","obj":"Gene:6772"},{"id":"A398","pred":"tao:has_database_id","subj":"398","obj":"Gene:3659"},{"id":"A399","pred":"tao:has_database_id","subj":"399","obj":"Tax:694009"},{"id":"A400","pred":"tao:has_database_id","subj":"400","obj":"Tax:1335626"},{"id":"A401","pred":"tao:has_database_id","subj":"401","obj":"Tax:2697049"},{"id":"A402","pred":"tao:has_database_id","subj":"402","obj":"MESH:D006108"},{"id":"A403","pred":"tao:has_database_id","subj":"403","obj":"MESH:D002244"},{"id":"A404","pred":"tao:has_database_id","subj":"404","obj":"MESH:D011137"},{"id":"A405","pred":"tao:has_database_id","subj":"405","obj":"MESH:C000628730"},{"id":"A406","pred":"tao:has_database_id","subj":"406","obj":"MESH:D064420"},{"id":"A407","pred":"tao:has_database_id","subj":"407","obj":"MESH:D007239"},{"id":"A414","pred":"tao:has_database_id","subj":"414","obj":"Tax:2697049"},{"id":"A415","pred":"tao:has_database_id","subj":"415","obj":"Tax:2697049"},{"id":"A416","pred":"tao:has_database_id","subj":"416","obj":"MESH:D012503"},{"id":"A417","pred":"tao:has_database_id","subj":"417","obj":"MESH:D064420"},{"id":"A419","pred":"tao:has_database_id","subj":"419","obj":"Tax:2697049"},{"id":"A427","pred":"tao:has_database_id","subj":"427","obj":"Gene:114548"},{"id":"A428","pred":"tao:has_database_id","subj":"428","obj":"Gene:3552"},{"id":"A429","pred":"tao:has_database_id","subj":"429","obj":"Tax:9606"},{"id":"A430","pred":"tao:has_database_id","subj":"430","obj":"MESH:C000628730"},{"id":"A432","pred":"tao:has_database_id","subj":"432","obj":"MESH:C000628730"},{"id":"A441","pred":"tao:has_database_id","subj":"441","obj":"Tax:121791"},{"id":"A442","pred":"tao:has_database_id","subj":"442","obj":"MESH:C000606551"},{"id":"A443","pred":"tao:has_database_id","subj":"443","obj":"MESH:C000606551"},{"id":"A444","pred":"tao:has_database_id","subj":"444","obj":"MESH:C000657245"},{"id":"A445","pred":"tao:has_database_id","subj":"445","obj":"MESH:C000657245"},{"id":"A446","pred":"tao:has_database_id","subj":"446","obj":"MESH:D001102"},{"id":"A447","pred":"tao:has_database_id","subj":"447","obj":"MESH:C000657245"},{"id":"A464","pred":"tao:has_database_id","subj":"464","obj":"Gene:3569"},{"id":"A465","pred":"tao:has_database_id","subj":"465","obj":"Gene:3569"},{"id":"A466","pred":"tao:has_database_id","subj":"466","obj":"Gene:3569"},{"id":"A467","pred":"tao:has_database_id","subj":"467","obj":"Gene:3569"},{"id":"A468","pred":"tao:has_database_id","subj":"468","obj":"Tax:9606"},{"id":"A469","pred":"tao:has_database_id","subj":"469","obj":"Tax:9606"},{"id":"A470","pred":"tao:has_database_id","subj":"470","obj":"Tax:9606"},{"id":"A471","pred":"tao:has_database_id","subj":"471","obj":"MESH:C502936"},{"id":"A472","pred":"tao:has_database_id","subj":"472","obj":"MESH:C000592401"},{"id":"A473","pred":"tao:has_database_id","subj":"473","obj":"MESH:C504234"},{"id":"A474","pred":"tao:has_database_id","subj":"474","obj":"MESH:C000657245"},{"id":"A475","pred":"tao:has_database_id","subj":"475","obj":"MESH:D003398"},{"id":"A476","pred":"tao:has_database_id","subj":"476","obj":"MESH:D012128"},{"id":"A477","pred":"tao:has_database_id","subj":"477","obj":"MESH:D012128"},{"id":"A478","pred":"tao:has_database_id","subj":"478","obj":"MESH:D009102"},{"id":"A479","pred":"tao:has_database_id","subj":"479","obj":"MESH:D003643"},{"id":"A481","pred":"tao:has_database_id","subj":"481","obj":"MESH:D007239"},{"id":"A505","pred":"tao:has_database_id","subj":"505","obj":"Gene:59272"},{"id":"A506","pred":"tao:has_database_id","subj":"506","obj":"Gene:968"},{"id":"A507","pred":"tao:has_database_id","subj":"507","obj":"Gene:6614"},{"id":"A508","pred":"tao:has_database_id","subj":"508","obj":"Gene:3569"},{"id":"A509","pred":"tao:has_database_id","subj":"509","obj":"Gene:6614"},{"id":"A510","pred":"tao:has_database_id","subj":"510","obj":"Gene:18126"},{"id":"A511","pred":"tao:has_database_id","subj":"511","obj":"Gene:21926"},{"id":"A512","pred":"tao:has_database_id","subj":"512","obj":"Tax:9606"},{"id":"A513","pred":"tao:has_database_id","subj":"513","obj":"Tax:2697049"},{"id":"A514","pred":"tao:has_database_id","subj":"514","obj":"Tax:9606"},{"id":"A515","pred":"tao:has_database_id","subj":"515","obj":"Tax:9606"},{"id":"A516","pred":"tao:has_database_id","subj":"516","obj":"Tax:10090"},{"id":"A517","pred":"tao:has_database_id","subj":"517","obj":"Tax:2697049"},{"id":"A518","pred":"tao:has_database_id","subj":"518","obj":"MESH:C009317"},{"id":"A519","pred":"tao:has_database_id","subj":"519","obj":"MESH:D003907"},{"id":"A520","pred":"tao:has_database_id","subj":"520","obj":"MESH:C000657245"},{"id":"A521","pred":"tao:has_database_id","subj":"521","obj":"MESH:D007239"},{"id":"A522","pred":"tao:has_database_id","subj":"522","obj":"MESH:D003643"},{"id":"A523","pred":"tao:has_database_id","subj":"523","obj":"MESH:C000657245"},{"id":"A524","pred":"tao:has_database_id","subj":"524","obj":"MESH:D007239"},{"id":"A525","pred":"tao:has_database_id","subj":"525","obj":"MESH:D007249"},{"id":"A526","pred":"tao:has_database_id","subj":"526","obj":"MESH:D003643"},{"id":"A527","pred":"tao:has_database_id","subj":"527","obj":"MESH:C000657245"},{"id":"A535","pred":"tao:has_database_id","subj":"535","obj":"Tax:9606"},{"id":"A537","pred":"tao:has_database_id","subj":"537","obj":"MESH:D018805"},{"id":"A538","pred":"tao:has_database_id","subj":"538","obj":"MESH:D007239"},{"id":"A539","pred":"tao:has_database_id","subj":"539","obj":"MESH:D003643"},{"id":"A540","pred":"tao:has_database_id","subj":"540","obj":"MESH:D003643"},{"id":"A541","pred":"tao:has_database_id","subj":"541","obj":"MESH:D001424"},{"id":"A554","pred":"tao:has_database_id","subj":"554","obj":"Gene:3569"},{"id":"A555","pred":"tao:has_database_id","subj":"555","obj":"Gene:7124"},{"id":"A556","pred":"tao:has_database_id","subj":"556","obj":"Tax:9606"},{"id":"A557","pred":"tao:has_database_id","subj":"557","obj":"Tax:9606"},{"id":"A558","pred":"tao:has_database_id","subj":"558","obj":"MESH:D002244"},{"id":"A559","pred":"tao:has_database_id","subj":"559","obj":"MESH:D002244"},{"id":"A561","pred":"tao:has_database_id","subj":"561","obj":"MESH:D006108"},{"id":"A562","pred":"tao:has_database_id","subj":"562","obj":"MESH:D018805"},{"id":"A563","pred":"tao:has_database_id","subj":"563","obj":"MESH:D018805"},{"id":"A564","pred":"tao:has_database_id","subj":"564","obj":"MESH:D003398"},{"id":"A565","pred":"tao:has_database_id","subj":"565","obj":"MESH:D003643"},{"id":"A575","pred":"tao:has_database_id","subj":"575","obj":"Tax:2697049"},{"id":"A576","pred":"tao:has_database_id","subj":"576","obj":"Tax:10566"},{"id":"A577","pred":"tao:has_database_id","subj":"577","obj":"Tax:12721"},{"id":"A578","pred":"tao:has_database_id","subj":"578","obj":"MESH:D017382"},{"id":"A579","pred":"tao:has_database_id","subj":"579","obj":"MESH:D017382"},{"id":"A580","pred":"tao:has_database_id","subj":"580","obj":"MESH:D010100"},{"id":"A581","pred":"tao:has_database_id","subj":"581","obj":"MESH:D017382"},{"id":"A582","pred":"tao:has_database_id","subj":"582","obj":"MESH:D008055"},{"id":"A583","pred":"tao:has_database_id","subj":"583","obj":"MESH:D003643"},{"id":"A598","pred":"tao:has_database_id","subj":"598","obj":"Gene:10611"},{"id":"A599","pred":"tao:has_database_id","subj":"599","obj":"Tax:12637"},{"id":"A600","pred":"tao:has_database_id","subj":"600","obj":"Tax:28285"},{"id":"A603","pred":"tao:has_database_id","subj":"603","obj":"MESH:C052159"},{"id":"A604","pred":"tao:has_database_id","subj":"604","obj":"MESH:D011094"},{"id":"A605","pred":"tao:has_database_id","subj":"605","obj":"MESH:D011094"},{"id":"A610","pred":"tao:has_database_id","subj":"610","obj":"MESH:D008670"},{"id":"A611","pred":"tao:has_database_id","subj":"611","obj":"MESH:D005461"},{"id":"A630","pred":"tao:has_database_id","subj":"630","obj":"Tax:11033"},{"id":"A631","pred":"tao:has_database_id","subj":"631","obj":"Tax:11276"},{"id":"A632","pred":"tao:has_database_id","subj":"632","obj":"Tax:10298"},{"id":"A633","pred":"tao:has_database_id","subj":"633","obj":"Tax:11676"},{"id":"A634","pred":"tao:has_database_id","subj":"634","obj":"Tax:11320"},{"id":"A635","pred":"tao:has_database_id","subj":"635","obj":"Tax:12022"},{"id":"A636","pred":"tao:has_database_id","subj":"636","obj":"Tax:2697049"},{"id":"A637","pred":"tao:has_database_id","subj":"637","obj":"Tax:11033"},{"id":"A638","pred":"tao:has_database_id","subj":"638","obj":"Tax:11276"},{"id":"A639","pred":"tao:has_database_id","subj":"639","obj":"Tax:11320"},{"id":"A640","pred":"tao:has_database_id","subj":"640","obj":"MESH:D037741"},{"id":"A641","pred":"tao:has_database_id","subj":"641","obj":"MESH:D006108"},{"id":"A642","pred":"tao:has_database_id","subj":"642","obj":"MESH:D006108"},{"id":"A644","pred":"tao:has_database_id","subj":"644","obj":"MESH:D010758"},{"id":"A646","pred":"tao:has_database_id","subj":"646","obj":"MESH:C082964"},{"id":"A647","pred":"tao:has_database_id","subj":"647","obj":"MESH:D009369"},{"id":"A649","pred":"tao:has_database_id","subj":"649","obj":"Tax:9796"},{"id":"A654","pred":"tao:has_database_id","subj":"654","obj":"MESH:D000073893"},{"id":"A655","pred":"tao:has_database_id","subj":"655","obj":"MESH:D006025"},{"id":"A656","pred":"tao:has_database_id","subj":"656","obj":"MESH:D006497"},{"id":"A657","pred":"tao:has_database_id","subj":"657","obj":"MESH:D006497"},{"id":"A673","pred":"tao:has_database_id","subj":"673","obj":"Gene:57126"},{"id":"A674","pred":"tao:has_database_id","subj":"674","obj":"Gene:59272"},{"id":"A675","pred":"tao:has_database_id","subj":"675","obj":"Tax:11309"},{"id":"A676","pred":"tao:has_database_id","subj":"676","obj":"Tax:12814"},{"id":"A677","pred":"tao:has_database_id","subj":"677","obj":"Tax:2697049"},{"id":"A678","pred":"tao:has_database_id","subj":"678","obj":"MESH:D015080"},{"id":"A679","pred":"tao:has_database_id","subj":"679","obj":"MESH:D006497"},{"id":"A680","pred":"tao:has_database_id","subj":"680","obj":"MESH:D012794"},{"id":"A681","pred":"tao:has_database_id","subj":"681","obj":"MESH:D012794"},{"id":"A682","pred":"tao:has_database_id","subj":"682","obj":"MESH:C000499"},{"id":"A683","pred":"tao:has_database_id","subj":"683","obj":"MESH:D006493"},{"id":"A684","pred":"tao:has_database_id","subj":"684","obj":"MESH:C536395"},{"id":"A685","pred":"tao:has_database_id","subj":"685","obj":"MESH:D013280"},{"id":"A686","pred":"tao:has_database_id","subj":"686","obj":"MESH:D012141"},{"id":"A689","pred":"tao:has_database_id","subj":"689","obj":"Gene:59272"},{"id":"A691","pred":"tao:has_database_id","subj":"691","obj":"MESH:C000657245"},{"id":"A695","pred":"tao:has_database_id","subj":"695","obj":"Tax:9606"},{"id":"A696","pred":"tao:has_database_id","subj":"696","obj":"MESH:C000657245"},{"id":"A697","pred":"tao:has_database_id","subj":"697","obj":"MESH:C000657245"},{"id":"A703","pred":"tao:has_database_id","subj":"703","obj":"Tax:9606"},{"id":"A704","pred":"tao:has_database_id","subj":"704","obj":"Tax:2697049"},{"id":"A705","pred":"tao:has_database_id","subj":"705","obj":"MESH:D006046"},{"id":"A706","pred":"tao:has_database_id","subj":"706","obj":"MESH:D009369"},{"id":"A707","pred":"tao:has_database_id","subj":"707","obj":"MESH:D009369"}],"namespaces":[{"prefix":"Tax","uri":"https://www.ncbi.nlm.nih.gov/taxonomy/"},{"prefix":"MESH","uri":"https://id.nlm.nih.gov/mesh/"},{"prefix":"Gene","uri":"https://www.ncbi.nlm.nih.gov/gene/"},{"prefix":"CVCL","uri":"https://web.expasy.org/cellosaurus/CVCL_"}],"text":"Nanotechnology Tools to Inactivate SARS-CoV-2 in Patients\nThe main target of SARS-CoV-2 is the respiratory tract (upper airways, lung),36 although other organs might also be infected (e.g., gut, kidney) and vasculature21 also appears to be a prime target. The expression of ACE2 probably determines uptake by different tissues.37\nIn addition to discussing immune-based approaches, because the lung is the most critically affected organ, we will center our discussion on the various options to inactivate the virus in the deep lung and to target the essential host cells for drug delivery. The virus reaches the alveoli and enters alveolar epithelial type II cells (AECII), due to the relatively high abundance of ACE2 and a permissive cellular milieu. These cells serve as a reservoir of the virus, which finally spreads throughout the lung, leading to the lung function impairment seen in severe cases. Airborne nanomaterials are optimally suited to penetrate into the deep lung due to the physicochemical properties of such aerosols, existing on the same size scale particles that penetrate most readily to the deep airways. Hence, nanomedicine is already actively pursuing ideas to deliver drugs, therapeutic proteins, and mRNAs by exploiting nanodevices for pulmonary delivery.38,39\nMoreover, the rapid emergence of SARS-CoV-2 has exposed one of the main weaknesses in the current medical landscape: the lack of broad-spectrum antiviral drugs. At present, there are only a handful of approved antivirals, and they are mostly virus-specific. Hence, when a new virus emerges, little can be done pharmacologically to slow down its spread. Some research efforts have been focused on the development of broad-spectrum drugs, which could potentially offer some efficacy against future emerging viruses (and maybe SARS-CoV-2). The various approaches developed over the years are mainly based on the creation of entry inhibitors.40,41 A highly conserved part in viruses is the attachment ligand (VAL). In most known respiratory viruses,42 the VAL targets either heparan sulfate proteoglycans (HSPG)43 or sialic acids (SA).44 Both HSPG and SA mimics have shown in vitro ability to bind to viruses, blocking their interaction with cell membranes, and often in a broad-spectrum way.45−47\nIn the context of nanomedicine, many nanomaterials have been developed, ranging from polymers48 to dendrimers,49 oligomers, NPs,50 liposomes,51 and small molecules.52 However, successful clinical translation has been hindered by the fact that, upon dilution, these compounds lose efficacy as the virus-compound complex dissociates leaving viruses free to restart their replication cycle. Recently, it has been shown that this limitation can be overcome by synthesizing NPs that, after binding, are able to inhibit viral infectivity irreversibly by permanently damaging the virion, refueling the hope for a true, broad-spectrum antiviral drug.53 Because the focus is also on the development of a drug specific to SARS-CoV-2, a good entry inhibitor could be based on blocking the S spike protein interaction with the cellular ACE2 receptor.19,21−23 Regardless of the specific approach, it is imperative that novel, effective antivirals be based on compounds that exhibit very low or negligible toxicity profiles, as patients will most likely need to receive those drugs for extended periods of time and will already be weakened. For these reasons, when designing antiviral drugs, clearance mechanisms have to be kept in mind. An example of this process is the recent redesign of broad-spectrum antiviral NPs into equally effective modified cyclodextrins.54 Moreover, nanotechnology may offer nanotheranostic approaches to fill the existing gap between diagnostics and therapy.55−57 The simultaneous management of both diagnostics and therapy for those suffering from COVID-19 or in future pandemics, as for many other diseases, is an additional potential strategy to take into consideration in which nanomaterials have proven to be effective tools. The advantages of the capabilities of nanotechnology and nanomaterials for combined therapeutics and diagnostics has been widely explored in cancer research; however, there have been considerable efforts in the past few years to extend the scope of this approach to other areas including infectious diseases.58\n\nNanomaterial-Based Vaccine Development and Immunomodulation\nFollowing the publication of the genetic sequence of SARS-CoV-2 on January 11, 2020, intense research efforts have been devoted to developing a vaccine against COVID-19. With unprecedented speed, this extraordinary scientific mobilization led the first vaccine candidate to enter the Phase I human clinical trial on March 16, 2020, and other novel candidates are rapidly following.59 Up to May 22, 2020, there are 10 COVID-19 candidate vaccines in clinical evaluations and 114 in preclinical development.60\nConcerning vaccine and immunization research, nanomaterials can assist in multiple ways to boost the upregulation required by the immune system and to direct the immune response specifically against antigens. Immune-targeted nanotherapeutics can be developed through their rational manufacture at the nanoscale level by designing nanomaterials that are able to amplify host’s immune response, for instance as adjuvants in the context of vaccination.\nThe development of a vaccine will rely either on the direct administration of viral antigens (e.g., in the form of recombinant proteins, vectored vaccines, or whole inactivated or attenuated virus) or RNA- or DNA-encoding viral antigens.27 Candidate antigens for immunization are surface proteins such as the immunogenic spike protein (S1), which is already targeted by antibodies of convalescent patients.27 Because the S1 protein is also essential for cellular uptake, many researchers are using this protein as the primary target for a vaccine.\nThere are many issues related to the delivery of a drug, protein, or RNA into the patient as the cargo is often degraded, not bioavailable, or is swiftly cleared. Nanotechnology provides multiple solutions to these challenges though, as nanocarriers can overcome some of these limitations.\nBiocompatible polymeric-, lipid-based, or inorganic NPs can be tuned with respect to their physicochemical properties to encapsulate cargo proteins with high loading efficiency, improving protein delivery and pharmacokinetics over conventional approaches.38 Intranasal delivery of polymer-encapsulated antigen triggers a strong immune response, and the success of vaccination depends on the appropriate type of polymer in combination with the antigen.61,62 Similarly, researchers have developed lipid and lipid-based NPs as delivery platforms for mRNAs or siRNAs to enable the synthesis of key viral proteins for vaccination or to inactivate critical viral target genes, respectively.39 The field of therapeutic mRNAs to support vaccination has gained momentum, as well,63 at least in part due to nanotechnology-enabled strategies for cargo delivery. Moreover, the versatility of nanoplatforms as antigen-presenting systems offers great opportunities. Considering the possibility of random viral mutations that may alter the antigen shape, functionalizing nanomaterials with a wide number of molecules at the same time to target the virus, or motifs that are specific for pathogens, will increase the efficiency of vaccines and their ability to prevent viral infection.\nSeveral companies are working on mRNA vaccines encoding SARS-CoV-2 proteins such as the spike protein, encapsulated in nanoliposomes with specific physicochemical properties that are potentially akin to those documented for immunization against certain tumor antigens.64 The design of such nanocarriers, which will need to escape recognition by scavenger cells and to be nontoxic and nonimmunogenic, is a challenge that will require substantial time prior to clinical availability.\nOn March 16, 2020, Moderna, through a partnership with the Vaccine Research Center at the U.S. National Institutes of Health, enrolled the first participants into a Phase I clinical trial testing an mRNA vaccine (mRNA-1273) encapsulated in lipid NPs—a record time of just 63 days following sequence selection (NCT04283461).65 The enrollment of the first cohort of participants (18 to 55-year-old healthy subjects) concluded on April 16, 2020.66 CureVac and BioNTech (in partnership with Pfizer) are currently working on similar vaccines; Pfizer/BioNTech, in particular, have recently started the recruitment in Phase I/II trials (NCT04368728, NCT04380701). A DNA plasmid vaccine by Inovio Pharmaceuticals (INO-4800) has showed promising results in mice and guinea pigs according to a recent article published in Nature Communications(67) and has entered Phase I testing in humans (NCT04336410).\nAnother candidate COVID-19 vaccine for Phase I clinical trial is from the University of Oxford and AstraZeneca (NCT04324606).68 Around 1110 people will take part in the trial, which started recruitment at the end of April 2020. The vaccine is based on a chimpanzee adenovirus vaccine vector (ChAdOx1) and the SARS-CoV-2 spike protein. Chimpanzee adenoviral vectors against different pathogens have been already tested in thousands of subjects with demonstrated safety. To date, ChAdOx1 has been administered to six rhesus macaques exposed to high doses of SARS-CoV-2. The vaccine was unable to prevent infections, although it reduced the severity of the disease: no signs of virus replication were observed in the lungs, with significantly lower levels of respiratory disease and no lung damage compared to control animals, according to a recent article deposited in bioRxiv.69 Another adenoviral vector vaccine developed by CanSino Biological Inc. and Beijing Institute of Biotechnology using a genetically engineered replication-defective adenovirus type 5 vector to express the SARS-CoV-2 spike protein (Ad5-nCoV) is currently being tested in Phase I/II trials (NCT04398147, NCT04341389, NCT0431312).\nAlthough vaccines based on novel DNA and mRNA technologies might be promising, there are no such kinds of vaccines on the market, and it is unknown whether they can be effective in humans. Moderna, for instance, has generated preliminary safety data on different mRNA-based vaccines targeting other respiratory viruses, but their more advanced program (on a cytomegalovirus vaccine) is still in Phase II clinical testing. However, on May 18, Moderna announced that mRNA-1273 elicited antibody titers above the levels observed in convalescent individuals (and therefore considered potentially protective) in all eight initial participants across the 25 and 100 μg dose cohorts of the Phase I trial (NCT04283461).70 The company, after having received a fast-track approval from the FDA, is now moving on Phase II trials.\nConversely, strong evidence exists regarding the efficacy of protein-based vaccines such as recombinant-protein, viral-vector, attenuated, or inactivated vaccines across different infectious diseases, with licensed vaccines already existing for all of these platforms.27 All of the aforementioned approaches are currently being explored in the context of SARS-CoV-2.27 An inactivated vaccine developed by the China National Pharmaceutical Group (Sinopharm), in collaboration with the Wuhan Institute of Biological Products, is currently tested in a Phase I/II trial (ChiCTR2000031809), and a second inactivated vaccine (in collaboration with the Beijing Institute of Biological Products) has been currently approved for clinical testing (ChiCTR2000032459). A third aluminum salt (alum) adjuvanted inactivated vaccine, developed by Beijing-based Sinovac Biotech’s, was able to provide partial or complete protection in rhesus macaques, according to a recent article published in Science,71 and is currently being tested in Phase I/II trials (NCT04383574, NCT04352608).\nLive-attenuated vaccines are intrinsically immunogenic (e.g., due to the presence of viral DNA), but extensive safety tests are required due to the rare possibility of reversion to a pathogenic form able to cause infection. Vaccine candidates based in this application have previously been designed for SARS-CoV with high stability.72,73 Recombinant-protein vaccines and inactivated vaccines are safer but might require adjuvants to increase their immunogenicity. In the context of SARS-CoV-2, adjuvants are important for two reasons. First, adjuvants might increase the efficacy of the vaccine, especially in subjects with impaired immunological function, such as the elderly, or in subjects with comorbidities resulting in immune dysfunctions; in these patient cohorts, SARS-CoV-2 has a high lethality rate. Second, adjuvants can reduce the amount of vaccine protein(s) required per dose, which could facilitate scaling-up vaccine production in a reduced time frame.\nBeyond alum, which is in fact a nanoscale material74 that was developed in the 1920s for the tetanus and diphtheria toxoids,75 approval for a new adjuvant did not occur until 1997, with the introduction of the oil-in-water emulsion of squalene oil and polysorbate 80 and sorbitan trioleate surfactants (MF59) in the seasonal influenza vaccine for the elderly.76 The use of MF59 was further expanded to pandemic and avian influenza vaccines.77 Other adjuvants in licensed vaccines have been approved since 2000, as well: (a) AS03 (used for pandemic and avian influenza vaccines), similar to MF59, but including α-tocopherol as an additional immune stimulant; (b) AF 03 (used for pandemic influenza vaccines), an alternative squalene emulsion containing polyoxyethylene, cetostearyl ether, mannitol, and orbitan oleate;78 (c) AS 01 (used for herpes zoster vaccine), a liposome-based vaccine adjuvant system containing two immunostimulants, 3-O-desacyl-4′-monophosphoryl lipid A (MPL, a Toll-like receptor 4 agonist) and saponin QS-21, which activates the ACT-NLRP3 inflammasome pathway;79 and (d) AS04 (used for hepatitis B and human papilloma virus vaccines), which is a combination of MPL and aluminum hydroxide.76,80\nIn this context, the concept of “nanoimmunity by design” relies on the rational design of distinct physicochemical properties and specific functionalization of nanomaterials intended for fine-tuning their potential effects on the immune system.81 Nanomaterials have emerged as promising tools for immune modulation, either stimulating or suppressing the immune response. In fighting SARS-CoV-2, these properties may find applications for both prevention and therapy and in the context of vaccine development.\nMounting evidence indicates that nanomaterials such as graphene,82 nanodiamonds,83 carbon nanotubes,84 and polystyrene particles85 bear an intrinsic capacity to activate the immune system, depending on their functionalization.86 For instance, graphene oxide functionalized with amino groups (GO-NH2) induces activation of STAT1/IRF1 interferon signaling in monocytes and T cells, resulting in the production of T cell chemoattractants, and macrophage 1 (M1) 1/T-helper 1 (Th1) polarization of the immune response, with negligible toxicity.82 Remarkably, the ability of licensed adjuvants such as AS01 and AS03 to enhance adaptive immunity has been linked to their capacity to boost STAT1/IRF1 interferon signaling.87 In addition, recent SARS-CoV and MERS-CoV studies suggest that the development of a Th1-type response is central for controlling infection, which also may be true for SARS-CoV-2.88\nSeveral groups and consortia have been screening and characterizing nanomaterials according to their immunomodulatory properties and absence of cytotoxicity.56,57,82−84,86 The development of an adjuvant for clinical use is a lengthy process that requires extensive Phase III randomized trials in large and diverse cohorts of subjects, and the process generally requires several years.76 Pharmaceutical giants such as GlaxoSmithKline (GSK), which owns the ASs mentioned above and other adjuvant platforms, are engaged in several partnerships to embed their adjuvant systems with SARS-CoV-2-protein-based vaccines. A full-length recombinant SARS-CoV-2 glycoprotein nanoparticle vaccine adjuvanted with the saponin-based Matrix M developed by Novavax is in Phase I clinical testing (NCT04368988).89\nAlthough it is unlikely that novel adjuvants would be used in the context of the current pandemic, the SARS-CoV-2 pandemic offers an opportunity to reflect on the potential of nanotechnology for vaccine adjuvant development. In this context, it is critical to stream coherent pipelines covering in vitro and in vivo experiments specifically to select candidate materials that might be tested for clinical implementation as vaccine adjuvants.90\nIn this view, the status of nanomaterial-based vaccine adjuvants has been reviewed.91,92 In particular, immunomodulatory effects induced on the innate immune signaling have been demonstrated.91 For example, nanomaterials such as GO can elicit an inflammasome sensor (NLRP3)-dependent expression of IL-1β in macrophages.93 Notably, alum, the most commonly used adjuvant in human vaccines, induces the release of this cytokine in macrophages through the same NRLP-induced mechanism. These results suggest that nanomaterials such as GO and alum may be useful for medical applications.94\nConsidering that new vaccine development typically requires years to be approved and applied to the general population, there is also an urgent need to study how to improve treatment approaches. According to the first Phase III clinical studies, the antiviral drug Remdesivir, which was recently approved by the FDA for COVID-19 treatment in the United States, seems to be a promising treatment for adults diagnosed with COVID-19. Nano-based strategies have already been applied to enhance the effectiveness of Remdesivir in the context of other emerging viral infections (Nipah virus),95 suggesting the suitability of nanotechnology to assist with similar strategies for the treatment of COVID-19 as well as other possible pandemics in the future.96\n\nCan Nanotechnology Help to Control the Cytokine Storm?\nOne of the main features of COVID-19 is the triggering of a cytokine storm in the body, also known as cytokine release syndrome (CRS), which results from an excessive immune response and leads to the severe deterioration of patient health.25,97,98 This inflammatory storm is one of the major causes of the acute respiratory distress syndrome (ARDS) that is often associated with multiple-organ failure, representing the leading causes of death in critical patients.98 In particular, the role of interleukin (IL)-6 has been highlighted in patients requiring assisted ventilation. Ongoing clinical trials are testing drugs that block the receptor of IL-6 (Tocilizumab, an anti-IL-6 receptor antibody, and Sarilumab) or IL-6 itself (Siltuximab).\nWhereas a well-regulated cytokine response that is rapidly triggered by the host’s innate immunity can serve to prevent and to counteract an infection, an excessive, unbalanced, prolonged immune response can seriously harm the body. Therefore, therapeutic strategies aimed at effectively suppressing the cytokine storm are under investigation. Nanomaterials have been exploited to adjust the immune response to an optimized level, and such proprieties might be explored to inhibit cytokine releases.99 Nanosystems can enhance the specificity/efficiency of immunosuppressant delivery to target immune cells, with consequent reductions in drug dose, drug distribution to nontarget tissues and organs, and possible side effects. In addition, specific nanotools can be designed to evade the immune system and to enhance the solubility of poorly soluble immunosuppressant agents; the potential of finely tuning their surface charge opens possibilities for encapsulation strategies and offers accommodation for a high drug load. All of these mechanisms may also occur simultaneously, enhancing the activity of immunosuppressive agents.\nConcerning the role of macrophages in COVID-19, the presence of ACE2-expressing CD68+CD169+ macrophages containing SARS-CoV-2 nucleoprotein antigen and showing an increased release of IL-6 was observed in infected spleen and lymph nodes.100 Notably, immunohistochemical and immunofluorescence analyses of lymph nodes and spleen tissue from autopsy samples of patients who died from COVID-19 revealed lymphocytic apoptosis. The tissues infected by SARS-CoV-2 also showed an upregulated expression of Fas, suggesting a role for CD169+ macrophages in viral spreading, aberrant inflammation, and activation-induced lymphocyte apoptosis. Moreover, histological examinations of biopsy samples of patients who died from COVID-19 revealed an increased alveolar exudate due to the extended neutrophil and monocyte infiltrate in lung capillaries with fibrin deposition, probably leading to difficulties in gas exchange. Through nanomedicine, we envision therapeutic approaches aimed at targeting specific immune subpopulations to avoid these complications, and different nanomaterials have already been explored for their specific impact on different immune cell subpopulations.82,83,86 Octadecylamine-functionalized and dexamethasone-adsorbed nanodiamond promotes anti-inflammatory and proregenerative behavior in human macrophages in vitro.101 A low dose of this functionalized nanodiamond also reduced macrophage infiltration and expression of proinflammatory mediators iNOS and tumor necrosis factor (TNF)-α in mice. Overall, these results suggest that nanodiamond particles may be useful as an inherently immunomodulatory platform.\nFinally, the indiscriminate systemic suppression of the immune system may increase the susceptibility to sepsis due to underlying secondary bacterial infections that can exacerbate critical conditions in patients, causing death.102 Risk factors for mortality include acquired bacterial infections by opportunistic organisms and primary pathogens. Using nanocarriers to achieve targeted delivery of immunosuppressive drugs, or the specific and controlled inhibition of only a specific immune cell subpopulation, can play a critical role in limiting such complications.\nThe fast and complete removal of pro-inflammatory cytokines from the bloodstream has been shown to increase survival significantly in patients with early stage sepsis. Adsorption of cytokines from serum by extracorporeal perfusion through porous carbons is one of the most promising ways for their selective, quick, and complete removal from blood circulation. Hierarchical carbon materials with tuned porosity have shown effective adsorption of many cytokines, including IL-6 and even the largest ones, such as TNF-α.103 Based on their demonstrated outstanding performance in removing sepsis-associated pro-inflammatory cytokines from blood, mesoporous carbon adsorbents with hierarchical structure, tuned pore size, and surface chemistry, as well as graphene with open and accessible surfaces104 have significant potential to quickly remove inflammatory cytokines associated with CRS and to prevent mortality arising from an uncontrolled inflammatory cascade, thereby providing enough time for the defense mechanisms of the human body to fight the virus.\n\nPhotodynamic Inactivation of SARS-CoV-2\nIn addition to drug- and vaccine-based antiviral strategies, photodynamic therapy (PDT) stands as a unique approach to inactivate SARS-CoV-2. Using a light-based method, PDT attacks target cells via the excitation of photosensitive agents, called photosensitizers (PSs), with radiation characterized by a wavelength corresponding to its absorption spectrum to generate reactive oxygen species (ROS) in the presence of oxygen, which ultimately results in cell death. Photodynamic therapy is primarily used for the clinical treatment of various oncological disorders.105 It was not until the 1970s that PDT was first used clinically against viruses,106 exploiting ROS production to damage virus proteins, nucleic acids, and—if present—lipids.107 Even though there are research efforts for PDT-based virotherapies against different viruses, including herpes simplex virus, human papilloma virus, and human immunodeficiency virus,106−109 clinical use of PDT is limited due to hydrophobicity of PSs, poor target specificity, and limited tissue penetration ability.\nMost PSs are hydrophobic and aggregate in aqueous solutions, affecting their photochemical and photobiological properties.106,110 For this reason, Lim et al. have proposed a promising approach for photodynamic inactivation of viruses with NPs, developing sodium yttrium fluoride (NaYF4) upconversion NPs (UCNs) with zinc phthalocyanine PSs grafted onto their surfaces. Unlike most PSs, these UCNs are coated with polyethylenimine (PEI), which render them hydrophilic and easier to manipulate. These UCNs showed antiviral activity against Dengue virus serotype 2 and adenovirus type 5, which were used as models of enveloped and non-enveloped viruses, respectively.110 MXenes111,112 are a large family of 2D transition metal carbides, nitrides,113 and carbonitrides114 that exhibit unique electronic, optical, and catalytic properties. They have the general formula Mn+1XnTx, where M is an early transition metal (Ti, Zr, V, Mo, etc.), X is C and/or N, Tx represents the surface functional groups (=O, −OH, −F, −Cl), and n = 1–4.115,116 Some examples include Ti3C2Tx, V2CTx, and Nb2CTx, with over 30 stoichiometric compositions already synthesized with more than 100 predicted. Biocompatible MXenes, such as Ti3C2Tx, are hydrophilic and are among the most efficient light-to-heat transforming materials.117 The plasmon resonance extinction maxima of Ti3C2Tx is at 780 nm, enabling the use of near-infrared (IR) light for PDT. Several other MXenes have absorption maxima in the IR range and have shown outstanding performance in PDT and theranostic applications.118\nFullerene and graphene are also good candidates for virus inactivation by PDT and have proved to be effective against Semliki Forest virus (SFV), vesicular stomatitis virus (VSV), HSV-1, HIV-1, mosquito iridovirus (MIV), and influenza A virus (IAV), as well as the phage MS2.107 In addition, several 2D nanomaterials, including graphene-based materials, MXenes, black phosphorus, graphitic carbon nitride, tungsten disulfide, and molybdenum disulfide, have been reported to improve the efficacy of PDT considerably for cancer treatment.56,57 Therefore, determining if such nanomaterial-based PDT protocols could be exploited to inactivate SARS-CoV-2 is of great interest.\n\nBiomimetic Engineering of Nanodelivery Systems: Artificial Viruses in the Making\nIn an effort to engineer the next generation of nanoscale vectors, scientists have moved from using inorganic components aimed at obtaining inert structures to utilizing biological building blocks that are able to convey additional functionalities to the resulting construct. To cope with the complexity of the body and to evade the multiple layers of defense that tissues and organs have, it is critical to rely on the ability of certain materials to interact with, rather than to eschew, the biology of our body. Every NP system conceived to date faces one common fate: whether injected, inhaled, ingested, or absorbed through our epithelia, all will at some point come into contact with the mixture of fluids and organic compounds that comprise the body. Under such conditions, every material reacts in a unique way according to the conditions they individually face (i.e., the tissue or body region they are in), their composition (i.e., organic or inorganic), and their physical properties (i.e., size, shape, surface charge).119 Inorganic NPs can function as globular protein mimics because of their similar size, charge, shape, and surface features that can be chemically functionalized to resemble proteins. These similarities can be used in biotechnology to control virus pathways or cell receptor interactions with NPs.120\nAmong the many attempts, the legendary accomplishments, and the epic failures, we could list thousands of different NPs, differing from one another thanks to the creative endeavors of their designers. Despite their remarkable differences and researchers’ endeavors to make them one of a kind, they all aim to achieve one goal: to deliver in a specific way one particular form of payload, while remaining as unnoticed as possible by the body’s defense mechanisms. For everyone who has made an effort to create their own version of such a silver bullet, it has come to mind that nature in its incredible variety had already invented a few ingenious solutions to this problem. In the world of nano-based drug delivery, one entity dominates as the quintessential example of precision, efficiency, and stealth: the virus. Not by chance, these two worlds share many features that span from the physical laws that govern their assembly and stability to the chemical similarities in their overall composition.121\nHistorically, the field of nanomedicine and the global endeavor to generate NPs for drug delivery arose from one of the greatest struggles in medicine: gene therapy. At the core of that approach was the idea that viruses could be used as Trojan horses to deliver the correct sequence of the gene into the cells that harbored the mutated copy. In order to identify the virus that offered the best delivery service, a cadre of viral strains and species were tested, adapted, and engineered to fit the aims and hit the targets. It took decades of trial and error, of progressive adjustments, and a few tragic mistakes to identify the viruses that could provide the ideal backbone to develop viral vectors able to ferry genetic cargo into the target cell.122 In the midst of that global challenge, nanotechnology offered a safer and more controllable alternative: to generate bespoke structures that could replace viral vectors and do the same job, delivering a payload from the point of injection to the site of action.\nFast forward a few decades, and the promises made by both worlds seem to have finally become reality, with a number of active clinical trials, some clinical success stories, and a few commercial products in the market space. Interestingly, the two worlds seem to have maintained a safe distance so as to avoid any collision or dangerous proximity. Exchange of ideas between the fields of gene therapy and nanomedicine is limited, and the potential for disciplinary growth through knowledge exchange has remained elusive to scientists in both fields.5 Many scientists have suggested that nanotechnologists could find inspiration in the mechanisms devised by viruses to elude immune surveillance, to overcome biological barriers, and to deliver their genetic payload with high specificity. Similarly, gene therapists would find high-tech solutions to their scalability and safety issues in looking at the new generations of biomimetic particles being generated.\nThe principles of biomimicry and bioinspiration have been used to design and to engineer drug-delivery technologies that reproduce or recapitulate biological materials, for what pertains to not only their structure and chemistry but also, more importantly, their functions. In drug delivery, surface recognition and nanoscale interactions between materials and biological entities are key to the success of the delivery strategy, and the use of biological building blocks such as membrane proteins has been proposed as a way to convey targeting and shielding moieties simultaneously.123 One can only hope that the recent events and global attention that viruses are capturing in the scientific world will spur a renewed interest in finding ways to adapt viral features and mechanisms of action to the world of NPs. Increased focus in this field would be useful to create virus-like NPs able to circulate in the blood system, overcoming the endothelial barrier, and to deliver their therapeutic payload with high efficiency.124\n\nInterference with Cellular Uptake, Immobilization, and Inactivation of the Virus Outside of the Host Cell\nNanomaterials can be synthesized with a high specific surface area of a few hundred square meters per gram. Therefore, dependent on the surface properties, nanomaterials efficiently adsorb biomolecules and form a so-called biomolecular corona. This passive, nontargeted adsorption might be utilized to bind viruses, provided that the selected nanomaterial is relatively biocompatible. Viral surface proteins are often modified by sugar moieties or encompass positively charged amino acid patches that bind to lectins or glycosaminoglycans (GAGs) of heparan sulfate (HS), respectively.32 Robust interactions of virus particles with these host receptors is ensured by multivalent binding, which is likely why single-molecule inhibitors often are not capable of efficiently perturbing this key event but multivalent NPs are superior to block binding of different viruses to the host cell.125\nGold NPs capped with mercaptoethanesulfonate are effective inhibitors of HSV type 1 infection as they mimic cell-surface-receptor heparan sulfate and, therefore, competitively bind to the virus. Interestingly, polyvalent sulfated Au NPs inhibit virus binding to the host cell dependent on their size. Nanoparticles of diameters equal to and larger than the virus diameter (in this case, the stomatitis virus) more efficiently inhibit the binding to cells than smaller particles. Most likely, larger NPs efficiently cross-link virions, whereas smaller NPs simply decorate the viral surface.126 Papp et al. found that gold NPs decorated with SA effectively inhibited the binding of influenza virus to the target cells. In this case, viral recognition via its surface protein hemagglutinin of SA on the host cell membrane was a prerequisite for cellular entry.127 More recently, Cagno et al. reported antiviral NPs (Au and iron oxide core) with long and flexible linkers mimicking HS that strongly bind and inactivate viruses such as respiratory syncytial virus in vitro and in vivo in a lung infection model and even led to irreversible viral deformation.53 Hence, there is ample evidence that biocompatible, functionalized NPs can act as broad-spectrum antivirals. Notably, the receptor-binding domain of the spike S1 protein of SARS-CoV-2 binds not only to ACE2 but also to heparin128 and, thus, might be targeted by similar approaches as outlined above.\nOne of the most recent strategies to inhibit viral uptake is based on administration of recombinant ACE2 to inhibit binding competitively via the spike S1 protein.21 Knowing that multivalency is key to block virus–host interactions reliably, researchers have speculated that a nanostructured carrier could not only improve delivery and cargo stability but also might dramatically enhance binding strength.129\n\nSpeeding up the Nanomedicine-Based Approaches for COVID-19 by In Silico Analysis\nCurrently, repurposing drug molecules is a key strategy for identifying approved or investigational drugs outside the scope of the original medical indication that can be used to fight COVID-19.130,131 There are various advantages to this strategy, including already-established safety profiles, fast transition to clinical studies, and less investment needed, compared to the process of developing an entirely new drug.132,133 Therefore, these advantages have the potential to result in less risky and more rapid returns on investment in the development of repurposed drugs, benefits that are particularly important during pandemics. In addition to rapid diagnosis, making recommendations to physicians about rapid treatment methods can save the lives of many people. Combining in silico tools such as molecular docking, molecular dynamics, and computational chemistry with large drug databases provides a great advantage in selecting “possible candidates” from among thousands of pharmaceutically active substances (Figure 3). In silico analyses should be supported by the literature, expert opinions (pharmacologists and clinicians), and, when possible, preclinical and clinical findings. After selecting the best candidates following in silico analyses, detailed in vitro and in vivo experimentation should be undertaken prior to clinical studies. Recently, various drug-repurposing studies have been published to assist in the global COVID-19 pandemic response.134−136 Such studies aim to identify whether already-approved drugs have the capacity to interact with viral proteins or receptors on host cells.\nFigure 3 Schematic of how in silico analysis can be used to select candidate drug molecules for clinical studies. MD, molecular dynamics. In nanomedicine, computational models have recently garnered attention; such work may help to identify how nanomaterials interact with biological systems and to determine how the efficacy of these nanotherapeutics could be improved. Computational models indicate how NPs are taken up by healthy cells or tumor cells, enabling better predictions regarding the pharmacokinetic and pharmacodynamic properties of these materials.134 For example, Lunnoo et al. used a coarse-grained molecular dynamics (MD) simulation to observe the internalization pathways of various Au nanostructures (nanospheres, nanocages, nanorods, nanoplates, and nanohexapods) into an idealized mammalian plasma membrane.137 Other studies have simulated how different NPs can target tumor cells and deliver drugs.138,139 Therefore, in silico approaches that are currently used for drug repurposing, including molecular docking, molecular dynamics, and computational chemistry, constitute valuable tools to aid preclinical and clinical studies of nanomaterials directed for disease treatment. Considering the urgent need for nanomedicine against the current pandemic, in silico analyses may be especially useful in guiding the rational design of new NP formulations required to fight SARS-CoV-2."}
2_test
{"project":"2_test","denotations":[{"id":"32519842-32246845-158468","span":{"begin":135,"end":137},"obj":"32246845"},{"id":"32519842-32333836-158469","span":{"begin":218,"end":220},"obj":"32333836"},{"id":"32519842-32413319-158470","span":{"begin":327,"end":329},"obj":"32413319"},{"id":"32519842-25263223-158471","span":{"begin":2118,"end":2120},"obj":"25263223"},{"id":"32519842-21384484-158472","span":{"begin":2275,"end":2277},"obj":"21384484"},{"id":"32519842-19447134-158473","span":{"begin":2278,"end":2280},"obj":"19447134"},{"id":"32519842-29894156-158474","span":{"begin":2391,"end":2393},"obj":"29894156"},{"id":"32519842-29472127-158475","span":{"begin":2422,"end":2424},"obj":"29472127"},{"id":"32519842-30846569-158476","span":{"begin":2445,"end":2447},"obj":"30846569"},{"id":"32519842-29251725-158477","span":{"begin":2923,"end":2925},"obj":"29251725"},{"id":"32519842-32015507-158478","span":{"begin":3119,"end":3121},"obj":"32015507"},{"id":"32519842-32333836-158479","span":{"begin":3122,"end":3124},"obj":"32333836"},{"id":"32519842-32142651-158480","span":{"begin":3125,"end":3127},"obj":"32142651"},{"id":"32519842-32064341-158481","span":{"begin":3633,"end":3635},"obj":"32064341"},{"id":"32519842-32031781-158482","span":{"begin":3755,"end":3757},"obj":"32031781"},{"id":"32519842-32373222-158483","span":{"begin":3758,"end":3760},"obj":"32373222"},{"id":"32519842-30599229-158484","span":{"begin":4336,"end":4338},"obj":"30599229"},{"id":"32519842-32259480-158485","span":{"begin":5594,"end":5596},"obj":"32259480"},{"id":"32519842-32259480-158486","span":{"begin":5763,"end":5765},"obj":"32259480"},{"id":"32519842-20562880-158487","span":{"begin":6646,"end":6648},"obj":"20562880"},{"id":"32519842-22073184-158488","span":{"begin":6649,"end":6651},"obj":"22073184"},{"id":"32519842-26040878-158489","span":{"begin":6965,"end":6967},"obj":"26040878"},{"id":"32519842-27281205-158490","span":{"begin":7733,"end":7735},"obj":"27281205"},{"id":"32519842-32433465-158491","span":{"begin":8781,"end":8783},"obj":"32433465"},{"id":"32519842-32259480-158492","span":{"begin":11133,"end":11135},"obj":"32259480"},{"id":"32519842-32259480-158493","span":{"begin":11231,"end":11233},"obj":"32259480"},{"id":"32519842-32376603-158494","span":{"begin":11851,"end":11853},"obj":"32376603"},{"id":"32519842-26513244-158495","span":{"begin":12265,"end":12267},"obj":"26513244"},{"id":"32519842-25609816-158496","span":{"begin":12268,"end":12270},"obj":"25609816"},{"id":"32519842-30323958-158497","span":{"begin":12952,"end":12954},"obj":"30323958"},{"id":"32519842-28734174-158498","span":{"begin":13026,"end":13028},"obj":"28734174"},{"id":"32519842-29801750-158499","span":{"begin":13261,"end":13263},"obj":"29801750"},{"id":"32519842-22941944-158500","span":{"begin":13719,"end":13721},"obj":"22941944"},{"id":"32519842-27448771-158501","span":{"begin":13986,"end":13988},"obj":"27448771"},{"id":"32519842-29801750-158502","span":{"begin":14114,"end":14116},"obj":"29801750"},{"id":"32519842-21815697-158503","span":{"begin":14117,"end":14119},"obj":"21815697"},{"id":"32519842-29061960-158504","span":{"begin":14693,"end":14695},"obj":"29061960"},{"id":"32519842-23507086-158505","span":{"begin":14729,"end":14731},"obj":"23507086"},{"id":"32519842-26854393-158506","span":{"begin":14757,"end":14759},"obj":"26854393"},{"id":"32519842-24885781-158507","span":{"begin":14855,"end":14857},"obj":"24885781"},{"id":"32519842-29061960-158508","span":{"begin":15168,"end":15170},"obj":"29061960"},{"id":"32519842-28855902-158509","span":{"begin":15343,"end":15345},"obj":"28855902"},{"id":"32519842-32105090-158510","span":{"begin":15524,"end":15526},"obj":"32105090"},{"id":"32519842-32373222-158511","span":{"begin":15687,"end":15689},"obj":"32373222"},{"id":"32519842-29061960-158512","span":{"begin":15690,"end":15692},"obj":"29061960"},{"id":"32519842-23507086-158513","span":{"begin":15693,"end":15695},"obj":"23507086"},{"id":"32519842-24885781-158514","span":{"begin":15696,"end":15698},"obj":"24885781"},{"id":"32519842-29801750-158515","span":{"begin":15911,"end":15913},"obj":"29801750"},{"id":"32519842-26791076-158516","span":{"begin":16764,"end":16766},"obj":"26791076"},{"id":"32519842-30774955-158517","span":{"begin":16853,"end":16855},"obj":"30774955"},{"id":"32519842-26389709-158518","span":{"begin":17086,"end":17088},"obj":"26389709"},{"id":"32519842-30387986-158519","span":{"begin":17348,"end":17350},"obj":"30387986"},{"id":"32519842-30904587-158520","span":{"begin":17937,"end":17939},"obj":"30904587"},{"id":"32519842-32325025-158521","span":{"begin":18397,"end":18399},"obj":"32325025"},{"id":"32519842-32192578-158522","span":{"begin":18400,"end":18402},"obj":"32192578"},{"id":"32519842-29061960-158523","span":{"begin":21199,"end":21201},"obj":"29061960"},{"id":"32519842-24885781-158524","span":{"begin":21205,"end":21207},"obj":"24885781"},{"id":"32519842-31198584-158525","span":{"begin":21363,"end":21366},"obj":"31198584"},{"id":"32519842-32171076-158526","span":{"begin":21886,"end":21889},"obj":"32171076"},{"id":"32519842-21617154-158527","span":{"begin":23889,"end":23892},"obj":"21617154"},{"id":"32519842-12791463-158528","span":{"begin":23971,"end":23974},"obj":"12791463"},{"id":"32519842-31397467-158529","span":{"begin":24064,"end":24067},"obj":"31397467"},{"id":"32519842-12791463-158530","span":{"begin":24250,"end":24253},"obj":"12791463"},{"id":"32519842-22852040-158531","span":{"begin":24254,"end":24257},"obj":"22852040"},{"id":"32519842-12791463-158532","span":{"begin":24506,"end":24509},"obj":"12791463"},{"id":"32519842-22153019-158533","span":{"begin":24510,"end":24513},"obj":"22153019"},{"id":"32519842-22153019-158534","span":{"begin":25048,"end":25051},"obj":"22153019"},{"id":"32519842-21861270-158535","span":{"begin":25058,"end":25061},"obj":"21861270"},{"id":"32519842-27211286-158536","span":{"begin":25127,"end":25130},"obj":"27211286"},{"id":"32519842-22279971-158537","span":{"begin":25148,"end":25151},"obj":"22279971"},{"id":"32519842-31804797-158538","span":{"begin":25416,"end":25419},"obj":"31804797"},{"id":"32519842-31397467-158539","span":{"begin":26223,"end":26226},"obj":"31397467"},{"id":"32519842-32373222-158540","span":{"begin":26487,"end":26489},"obj":"32373222"},{"id":"32519842-28946131-158541","span":{"begin":27733,"end":27736},"obj":"28946131"},{"id":"32519842-20929766-158542","span":{"begin":28031,"end":28034},"obj":"20929766"},{"id":"32519842-21720407-158543","span":{"begin":29036,"end":29039},"obj":"21720407"},{"id":"32519842-1303270-158544","span":{"begin":29791,"end":29794},"obj":"1303270"},{"id":"32519842-25022906-158545","span":{"begin":30606,"end":30607},"obj":"25022906"},{"id":"32519842-27213956-158546","span":{"begin":31600,"end":31603},"obj":"27213956"},{"id":"32519842-26982250-158547","span":{"begin":32040,"end":32043},"obj":"26982250"},{"id":"32519842-27575283-158548","span":{"begin":32735,"end":32737},"obj":"27575283"},{"id":"32519842-20394070-158549","span":{"begin":33036,"end":33039},"obj":"20394070"},{"id":"32519842-24430614-158550","span":{"begin":33629,"end":33632},"obj":"24430614"},{"id":"32519842-21104827-158551","span":{"begin":33897,"end":33900},"obj":"21104827"},{"id":"32519842-29251725-158552","span":{"begin":34193,"end":34195},"obj":"29251725"},{"id":"32519842-32333836-158553","span":{"begin":34658,"end":34660},"obj":"32333836"},{"id":"32519842-32286790-158554","span":{"begin":34900,"end":34903},"obj":"32286790"},{"id":"32519842-30310233-158555","span":{"begin":35406,"end":35409},"obj":"30310233"},{"id":"32519842-32305088-158556","span":{"begin":36452,"end":36455},"obj":"32305088"},{"id":"32519842-32305088-158557","span":{"begin":37162,"end":37165},"obj":"32305088"},{"id":"32519842-31065337-158558","span":{"begin":37524,"end":37527},"obj":"31065337"}],"text":"Nanotechnology Tools to Inactivate SARS-CoV-2 in Patients\nThe main target of SARS-CoV-2 is the respiratory tract (upper airways, lung),36 although other organs might also be infected (e.g., gut, kidney) and vasculature21 also appears to be a prime target. The expression of ACE2 probably determines uptake by different tissues.37\nIn addition to discussing immune-based approaches, because the lung is the most critically affected organ, we will center our discussion on the various options to inactivate the virus in the deep lung and to target the essential host cells for drug delivery. The virus reaches the alveoli and enters alveolar epithelial type II cells (AECII), due to the relatively high abundance of ACE2 and a permissive cellular milieu. These cells serve as a reservoir of the virus, which finally spreads throughout the lung, leading to the lung function impairment seen in severe cases. Airborne nanomaterials are optimally suited to penetrate into the deep lung due to the physicochemical properties of such aerosols, existing on the same size scale particles that penetrate most readily to the deep airways. Hence, nanomedicine is already actively pursuing ideas to deliver drugs, therapeutic proteins, and mRNAs by exploiting nanodevices for pulmonary delivery.38,39\nMoreover, the rapid emergence of SARS-CoV-2 has exposed one of the main weaknesses in the current medical landscape: the lack of broad-spectrum antiviral drugs. At present, there are only a handful of approved antivirals, and they are mostly virus-specific. Hence, when a new virus emerges, little can be done pharmacologically to slow down its spread. Some research efforts have been focused on the development of broad-spectrum drugs, which could potentially offer some efficacy against future emerging viruses (and maybe SARS-CoV-2). The various approaches developed over the years are mainly based on the creation of entry inhibitors.40,41 A highly conserved part in viruses is the attachment ligand (VAL). In most known respiratory viruses,42 the VAL targets either heparan sulfate proteoglycans (HSPG)43 or sialic acids (SA).44 Both HSPG and SA mimics have shown in vitro ability to bind to viruses, blocking their interaction with cell membranes, and often in a broad-spectrum way.45−47\nIn the context of nanomedicine, many nanomaterials have been developed, ranging from polymers48 to dendrimers,49 oligomers, NPs,50 liposomes,51 and small molecules.52 However, successful clinical translation has been hindered by the fact that, upon dilution, these compounds lose efficacy as the virus-compound complex dissociates leaving viruses free to restart their replication cycle. Recently, it has been shown that this limitation can be overcome by synthesizing NPs that, after binding, are able to inhibit viral infectivity irreversibly by permanently damaging the virion, refueling the hope for a true, broad-spectrum antiviral drug.53 Because the focus is also on the development of a drug specific to SARS-CoV-2, a good entry inhibitor could be based on blocking the S spike protein interaction with the cellular ACE2 receptor.19,21−23 Regardless of the specific approach, it is imperative that novel, effective antivirals be based on compounds that exhibit very low or negligible toxicity profiles, as patients will most likely need to receive those drugs for extended periods of time and will already be weakened. For these reasons, when designing antiviral drugs, clearance mechanisms have to be kept in mind. An example of this process is the recent redesign of broad-spectrum antiviral NPs into equally effective modified cyclodextrins.54 Moreover, nanotechnology may offer nanotheranostic approaches to fill the existing gap between diagnostics and therapy.55−57 The simultaneous management of both diagnostics and therapy for those suffering from COVID-19 or in future pandemics, as for many other diseases, is an additional potential strategy to take into consideration in which nanomaterials have proven to be effective tools. The advantages of the capabilities of nanotechnology and nanomaterials for combined therapeutics and diagnostics has been widely explored in cancer research; however, there have been considerable efforts in the past few years to extend the scope of this approach to other areas including infectious diseases.58\n\nNanomaterial-Based Vaccine Development and Immunomodulation\nFollowing the publication of the genetic sequence of SARS-CoV-2 on January 11, 2020, intense research efforts have been devoted to developing a vaccine against COVID-19. With unprecedented speed, this extraordinary scientific mobilization led the first vaccine candidate to enter the Phase I human clinical trial on March 16, 2020, and other novel candidates are rapidly following.59 Up to May 22, 2020, there are 10 COVID-19 candidate vaccines in clinical evaluations and 114 in preclinical development.60\nConcerning vaccine and immunization research, nanomaterials can assist in multiple ways to boost the upregulation required by the immune system and to direct the immune response specifically against antigens. Immune-targeted nanotherapeutics can be developed through their rational manufacture at the nanoscale level by designing nanomaterials that are able to amplify host’s immune response, for instance as adjuvants in the context of vaccination.\nThe development of a vaccine will rely either on the direct administration of viral antigens (e.g., in the form of recombinant proteins, vectored vaccines, or whole inactivated or attenuated virus) or RNA- or DNA-encoding viral antigens.27 Candidate antigens for immunization are surface proteins such as the immunogenic spike protein (S1), which is already targeted by antibodies of convalescent patients.27 Because the S1 protein is also essential for cellular uptake, many researchers are using this protein as the primary target for a vaccine.\nThere are many issues related to the delivery of a drug, protein, or RNA into the patient as the cargo is often degraded, not bioavailable, or is swiftly cleared. Nanotechnology provides multiple solutions to these challenges though, as nanocarriers can overcome some of these limitations.\nBiocompatible polymeric-, lipid-based, or inorganic NPs can be tuned with respect to their physicochemical properties to encapsulate cargo proteins with high loading efficiency, improving protein delivery and pharmacokinetics over conventional approaches.38 Intranasal delivery of polymer-encapsulated antigen triggers a strong immune response, and the success of vaccination depends on the appropriate type of polymer in combination with the antigen.61,62 Similarly, researchers have developed lipid and lipid-based NPs as delivery platforms for mRNAs or siRNAs to enable the synthesis of key viral proteins for vaccination or to inactivate critical viral target genes, respectively.39 The field of therapeutic mRNAs to support vaccination has gained momentum, as well,63 at least in part due to nanotechnology-enabled strategies for cargo delivery. Moreover, the versatility of nanoplatforms as antigen-presenting systems offers great opportunities. Considering the possibility of random viral mutations that may alter the antigen shape, functionalizing nanomaterials with a wide number of molecules at the same time to target the virus, or motifs that are specific for pathogens, will increase the efficiency of vaccines and their ability to prevent viral infection.\nSeveral companies are working on mRNA vaccines encoding SARS-CoV-2 proteins such as the spike protein, encapsulated in nanoliposomes with specific physicochemical properties that are potentially akin to those documented for immunization against certain tumor antigens.64 The design of such nanocarriers, which will need to escape recognition by scavenger cells and to be nontoxic and nonimmunogenic, is a challenge that will require substantial time prior to clinical availability.\nOn March 16, 2020, Moderna, through a partnership with the Vaccine Research Center at the U.S. National Institutes of Health, enrolled the first participants into a Phase I clinical trial testing an mRNA vaccine (mRNA-1273) encapsulated in lipid NPs—a record time of just 63 days following sequence selection (NCT04283461).65 The enrollment of the first cohort of participants (18 to 55-year-old healthy subjects) concluded on April 16, 2020.66 CureVac and BioNTech (in partnership with Pfizer) are currently working on similar vaccines; Pfizer/BioNTech, in particular, have recently started the recruitment in Phase I/II trials (NCT04368728, NCT04380701). A DNA plasmid vaccine by Inovio Pharmaceuticals (INO-4800) has showed promising results in mice and guinea pigs according to a recent article published in Nature Communications(67) and has entered Phase I testing in humans (NCT04336410).\nAnother candidate COVID-19 vaccine for Phase I clinical trial is from the University of Oxford and AstraZeneca (NCT04324606).68 Around 1110 people will take part in the trial, which started recruitment at the end of April 2020. The vaccine is based on a chimpanzee adenovirus vaccine vector (ChAdOx1) and the SARS-CoV-2 spike protein. Chimpanzee adenoviral vectors against different pathogens have been already tested in thousands of subjects with demonstrated safety. To date, ChAdOx1 has been administered to six rhesus macaques exposed to high doses of SARS-CoV-2. The vaccine was unable to prevent infections, although it reduced the severity of the disease: no signs of virus replication were observed in the lungs, with significantly lower levels of respiratory disease and no lung damage compared to control animals, according to a recent article deposited in bioRxiv.69 Another adenoviral vector vaccine developed by CanSino Biological Inc. and Beijing Institute of Biotechnology using a genetically engineered replication-defective adenovirus type 5 vector to express the SARS-CoV-2 spike protein (Ad5-nCoV) is currently being tested in Phase I/II trials (NCT04398147, NCT04341389, NCT0431312).\nAlthough vaccines based on novel DNA and mRNA technologies might be promising, there are no such kinds of vaccines on the market, and it is unknown whether they can be effective in humans. Moderna, for instance, has generated preliminary safety data on different mRNA-based vaccines targeting other respiratory viruses, but their more advanced program (on a cytomegalovirus vaccine) is still in Phase II clinical testing. However, on May 18, Moderna announced that mRNA-1273 elicited antibody titers above the levels observed in convalescent individuals (and therefore considered potentially protective) in all eight initial participants across the 25 and 100 μg dose cohorts of the Phase I trial (NCT04283461).70 The company, after having received a fast-track approval from the FDA, is now moving on Phase II trials.\nConversely, strong evidence exists regarding the efficacy of protein-based vaccines such as recombinant-protein, viral-vector, attenuated, or inactivated vaccines across different infectious diseases, with licensed vaccines already existing for all of these platforms.27 All of the aforementioned approaches are currently being explored in the context of SARS-CoV-2.27 An inactivated vaccine developed by the China National Pharmaceutical Group (Sinopharm), in collaboration with the Wuhan Institute of Biological Products, is currently tested in a Phase I/II trial (ChiCTR2000031809), and a second inactivated vaccine (in collaboration with the Beijing Institute of Biological Products) has been currently approved for clinical testing (ChiCTR2000032459). A third aluminum salt (alum) adjuvanted inactivated vaccine, developed by Beijing-based Sinovac Biotech’s, was able to provide partial or complete protection in rhesus macaques, according to a recent article published in Science,71 and is currently being tested in Phase I/II trials (NCT04383574, NCT04352608).\nLive-attenuated vaccines are intrinsically immunogenic (e.g., due to the presence of viral DNA), but extensive safety tests are required due to the rare possibility of reversion to a pathogenic form able to cause infection. Vaccine candidates based in this application have previously been designed for SARS-CoV with high stability.72,73 Recombinant-protein vaccines and inactivated vaccines are safer but might require adjuvants to increase their immunogenicity. In the context of SARS-CoV-2, adjuvants are important for two reasons. First, adjuvants might increase the efficacy of the vaccine, especially in subjects with impaired immunological function, such as the elderly, or in subjects with comorbidities resulting in immune dysfunctions; in these patient cohorts, SARS-CoV-2 has a high lethality rate. Second, adjuvants can reduce the amount of vaccine protein(s) required per dose, which could facilitate scaling-up vaccine production in a reduced time frame.\nBeyond alum, which is in fact a nanoscale material74 that was developed in the 1920s for the tetanus and diphtheria toxoids,75 approval for a new adjuvant did not occur until 1997, with the introduction of the oil-in-water emulsion of squalene oil and polysorbate 80 and sorbitan trioleate surfactants (MF59) in the seasonal influenza vaccine for the elderly.76 The use of MF59 was further expanded to pandemic and avian influenza vaccines.77 Other adjuvants in licensed vaccines have been approved since 2000, as well: (a) AS03 (used for pandemic and avian influenza vaccines), similar to MF59, but including α-tocopherol as an additional immune stimulant; (b) AF 03 (used for pandemic influenza vaccines), an alternative squalene emulsion containing polyoxyethylene, cetostearyl ether, mannitol, and orbitan oleate;78 (c) AS 01 (used for herpes zoster vaccine), a liposome-based vaccine adjuvant system containing two immunostimulants, 3-O-desacyl-4′-monophosphoryl lipid A (MPL, a Toll-like receptor 4 agonist) and saponin QS-21, which activates the ACT-NLRP3 inflammasome pathway;79 and (d) AS04 (used for hepatitis B and human papilloma virus vaccines), which is a combination of MPL and aluminum hydroxide.76,80\nIn this context, the concept of “nanoimmunity by design” relies on the rational design of distinct physicochemical properties and specific functionalization of nanomaterials intended for fine-tuning their potential effects on the immune system.81 Nanomaterials have emerged as promising tools for immune modulation, either stimulating or suppressing the immune response. In fighting SARS-CoV-2, these properties may find applications for both prevention and therapy and in the context of vaccine development.\nMounting evidence indicates that nanomaterials such as graphene,82 nanodiamonds,83 carbon nanotubes,84 and polystyrene particles85 bear an intrinsic capacity to activate the immune system, depending on their functionalization.86 For instance, graphene oxide functionalized with amino groups (GO-NH2) induces activation of STAT1/IRF1 interferon signaling in monocytes and T cells, resulting in the production of T cell chemoattractants, and macrophage 1 (M1) 1/T-helper 1 (Th1) polarization of the immune response, with negligible toxicity.82 Remarkably, the ability of licensed adjuvants such as AS01 and AS03 to enhance adaptive immunity has been linked to their capacity to boost STAT1/IRF1 interferon signaling.87 In addition, recent SARS-CoV and MERS-CoV studies suggest that the development of a Th1-type response is central for controlling infection, which also may be true for SARS-CoV-2.88\nSeveral groups and consortia have been screening and characterizing nanomaterials according to their immunomodulatory properties and absence of cytotoxicity.56,57,82−84,86 The development of an adjuvant for clinical use is a lengthy process that requires extensive Phase III randomized trials in large and diverse cohorts of subjects, and the process generally requires several years.76 Pharmaceutical giants such as GlaxoSmithKline (GSK), which owns the ASs mentioned above and other adjuvant platforms, are engaged in several partnerships to embed their adjuvant systems with SARS-CoV-2-protein-based vaccines. A full-length recombinant SARS-CoV-2 glycoprotein nanoparticle vaccine adjuvanted with the saponin-based Matrix M developed by Novavax is in Phase I clinical testing (NCT04368988).89\nAlthough it is unlikely that novel adjuvants would be used in the context of the current pandemic, the SARS-CoV-2 pandemic offers an opportunity to reflect on the potential of nanotechnology for vaccine adjuvant development. In this context, it is critical to stream coherent pipelines covering in vitro and in vivo experiments specifically to select candidate materials that might be tested for clinical implementation as vaccine adjuvants.90\nIn this view, the status of nanomaterial-based vaccine adjuvants has been reviewed.91,92 In particular, immunomodulatory effects induced on the innate immune signaling have been demonstrated.91 For example, nanomaterials such as GO can elicit an inflammasome sensor (NLRP3)-dependent expression of IL-1β in macrophages.93 Notably, alum, the most commonly used adjuvant in human vaccines, induces the release of this cytokine in macrophages through the same NRLP-induced mechanism. These results suggest that nanomaterials such as GO and alum may be useful for medical applications.94\nConsidering that new vaccine development typically requires years to be approved and applied to the general population, there is also an urgent need to study how to improve treatment approaches. According to the first Phase III clinical studies, the antiviral drug Remdesivir, which was recently approved by the FDA for COVID-19 treatment in the United States, seems to be a promising treatment for adults diagnosed with COVID-19. Nano-based strategies have already been applied to enhance the effectiveness of Remdesivir in the context of other emerging viral infections (Nipah virus),95 suggesting the suitability of nanotechnology to assist with similar strategies for the treatment of COVID-19 as well as other possible pandemics in the future.96\n\nCan Nanotechnology Help to Control the Cytokine Storm?\nOne of the main features of COVID-19 is the triggering of a cytokine storm in the body, also known as cytokine release syndrome (CRS), which results from an excessive immune response and leads to the severe deterioration of patient health.25,97,98 This inflammatory storm is one of the major causes of the acute respiratory distress syndrome (ARDS) that is often associated with multiple-organ failure, representing the leading causes of death in critical patients.98 In particular, the role of interleukin (IL)-6 has been highlighted in patients requiring assisted ventilation. Ongoing clinical trials are testing drugs that block the receptor of IL-6 (Tocilizumab, an anti-IL-6 receptor antibody, and Sarilumab) or IL-6 itself (Siltuximab).\nWhereas a well-regulated cytokine response that is rapidly triggered by the host’s innate immunity can serve to prevent and to counteract an infection, an excessive, unbalanced, prolonged immune response can seriously harm the body. Therefore, therapeutic strategies aimed at effectively suppressing the cytokine storm are under investigation. Nanomaterials have been exploited to adjust the immune response to an optimized level, and such proprieties might be explored to inhibit cytokine releases.99 Nanosystems can enhance the specificity/efficiency of immunosuppressant delivery to target immune cells, with consequent reductions in drug dose, drug distribution to nontarget tissues and organs, and possible side effects. In addition, specific nanotools can be designed to evade the immune system and to enhance the solubility of poorly soluble immunosuppressant agents; the potential of finely tuning their surface charge opens possibilities for encapsulation strategies and offers accommodation for a high drug load. All of these mechanisms may also occur simultaneously, enhancing the activity of immunosuppressive agents.\nConcerning the role of macrophages in COVID-19, the presence of ACE2-expressing CD68+CD169+ macrophages containing SARS-CoV-2 nucleoprotein antigen and showing an increased release of IL-6 was observed in infected spleen and lymph nodes.100 Notably, immunohistochemical and immunofluorescence analyses of lymph nodes and spleen tissue from autopsy samples of patients who died from COVID-19 revealed lymphocytic apoptosis. The tissues infected by SARS-CoV-2 also showed an upregulated expression of Fas, suggesting a role for CD169+ macrophages in viral spreading, aberrant inflammation, and activation-induced lymphocyte apoptosis. Moreover, histological examinations of biopsy samples of patients who died from COVID-19 revealed an increased alveolar exudate due to the extended neutrophil and monocyte infiltrate in lung capillaries with fibrin deposition, probably leading to difficulties in gas exchange. Through nanomedicine, we envision therapeutic approaches aimed at targeting specific immune subpopulations to avoid these complications, and different nanomaterials have already been explored for their specific impact on different immune cell subpopulations.82,83,86 Octadecylamine-functionalized and dexamethasone-adsorbed nanodiamond promotes anti-inflammatory and proregenerative behavior in human macrophages in vitro.101 A low dose of this functionalized nanodiamond also reduced macrophage infiltration and expression of proinflammatory mediators iNOS and tumor necrosis factor (TNF)-α in mice. Overall, these results suggest that nanodiamond particles may be useful as an inherently immunomodulatory platform.\nFinally, the indiscriminate systemic suppression of the immune system may increase the susceptibility to sepsis due to underlying secondary bacterial infections that can exacerbate critical conditions in patients, causing death.102 Risk factors for mortality include acquired bacterial infections by opportunistic organisms and primary pathogens. Using nanocarriers to achieve targeted delivery of immunosuppressive drugs, or the specific and controlled inhibition of only a specific immune cell subpopulation, can play a critical role in limiting such complications.\nThe fast and complete removal of pro-inflammatory cytokines from the bloodstream has been shown to increase survival significantly in patients with early stage sepsis. Adsorption of cytokines from serum by extracorporeal perfusion through porous carbons is one of the most promising ways for their selective, quick, and complete removal from blood circulation. Hierarchical carbon materials with tuned porosity have shown effective adsorption of many cytokines, including IL-6 and even the largest ones, such as TNF-α.103 Based on their demonstrated outstanding performance in removing sepsis-associated pro-inflammatory cytokines from blood, mesoporous carbon adsorbents with hierarchical structure, tuned pore size, and surface chemistry, as well as graphene with open and accessible surfaces104 have significant potential to quickly remove inflammatory cytokines associated with CRS and to prevent mortality arising from an uncontrolled inflammatory cascade, thereby providing enough time for the defense mechanisms of the human body to fight the virus.\n\nPhotodynamic Inactivation of SARS-CoV-2\nIn addition to drug- and vaccine-based antiviral strategies, photodynamic therapy (PDT) stands as a unique approach to inactivate SARS-CoV-2. Using a light-based method, PDT attacks target cells via the excitation of photosensitive agents, called photosensitizers (PSs), with radiation characterized by a wavelength corresponding to its absorption spectrum to generate reactive oxygen species (ROS) in the presence of oxygen, which ultimately results in cell death. Photodynamic therapy is primarily used for the clinical treatment of various oncological disorders.105 It was not until the 1970s that PDT was first used clinically against viruses,106 exploiting ROS production to damage virus proteins, nucleic acids, and—if present—lipids.107 Even though there are research efforts for PDT-based virotherapies against different viruses, including herpes simplex virus, human papilloma virus, and human immunodeficiency virus,106−109 clinical use of PDT is limited due to hydrophobicity of PSs, poor target specificity, and limited tissue penetration ability.\nMost PSs are hydrophobic and aggregate in aqueous solutions, affecting their photochemical and photobiological properties.106,110 For this reason, Lim et al. have proposed a promising approach for photodynamic inactivation of viruses with NPs, developing sodium yttrium fluoride (NaYF4) upconversion NPs (UCNs) with zinc phthalocyanine PSs grafted onto their surfaces. Unlike most PSs, these UCNs are coated with polyethylenimine (PEI), which render them hydrophilic and easier to manipulate. These UCNs showed antiviral activity against Dengue virus serotype 2 and adenovirus type 5, which were used as models of enveloped and non-enveloped viruses, respectively.110 MXenes111,112 are a large family of 2D transition metal carbides, nitrides,113 and carbonitrides114 that exhibit unique electronic, optical, and catalytic properties. They have the general formula Mn+1XnTx, where M is an early transition metal (Ti, Zr, V, Mo, etc.), X is C and/or N, Tx represents the surface functional groups (=O, −OH, −F, −Cl), and n = 1–4.115,116 Some examples include Ti3C2Tx, V2CTx, and Nb2CTx, with over 30 stoichiometric compositions already synthesized with more than 100 predicted. Biocompatible MXenes, such as Ti3C2Tx, are hydrophilic and are among the most efficient light-to-heat transforming materials.117 The plasmon resonance extinction maxima of Ti3C2Tx is at 780 nm, enabling the use of near-infrared (IR) light for PDT. Several other MXenes have absorption maxima in the IR range and have shown outstanding performance in PDT and theranostic applications.118\nFullerene and graphene are also good candidates for virus inactivation by PDT and have proved to be effective against Semliki Forest virus (SFV), vesicular stomatitis virus (VSV), HSV-1, HIV-1, mosquito iridovirus (MIV), and influenza A virus (IAV), as well as the phage MS2.107 In addition, several 2D nanomaterials, including graphene-based materials, MXenes, black phosphorus, graphitic carbon nitride, tungsten disulfide, and molybdenum disulfide, have been reported to improve the efficacy of PDT considerably for cancer treatment.56,57 Therefore, determining if such nanomaterial-based PDT protocols could be exploited to inactivate SARS-CoV-2 is of great interest.\n\nBiomimetic Engineering of Nanodelivery Systems: Artificial Viruses in the Making\nIn an effort to engineer the next generation of nanoscale vectors, scientists have moved from using inorganic components aimed at obtaining inert structures to utilizing biological building blocks that are able to convey additional functionalities to the resulting construct. To cope with the complexity of the body and to evade the multiple layers of defense that tissues and organs have, it is critical to rely on the ability of certain materials to interact with, rather than to eschew, the biology of our body. Every NP system conceived to date faces one common fate: whether injected, inhaled, ingested, or absorbed through our epithelia, all will at some point come into contact with the mixture of fluids and organic compounds that comprise the body. Under such conditions, every material reacts in a unique way according to the conditions they individually face (i.e., the tissue or body region they are in), their composition (i.e., organic or inorganic), and their physical properties (i.e., size, shape, surface charge).119 Inorganic NPs can function as globular protein mimics because of their similar size, charge, shape, and surface features that can be chemically functionalized to resemble proteins. These similarities can be used in biotechnology to control virus pathways or cell receptor interactions with NPs.120\nAmong the many attempts, the legendary accomplishments, and the epic failures, we could list thousands of different NPs, differing from one another thanks to the creative endeavors of their designers. Despite their remarkable differences and researchers’ endeavors to make them one of a kind, they all aim to achieve one goal: to deliver in a specific way one particular form of payload, while remaining as unnoticed as possible by the body’s defense mechanisms. For everyone who has made an effort to create their own version of such a silver bullet, it has come to mind that nature in its incredible variety had already invented a few ingenious solutions to this problem. In the world of nano-based drug delivery, one entity dominates as the quintessential example of precision, efficiency, and stealth: the virus. Not by chance, these two worlds share many features that span from the physical laws that govern their assembly and stability to the chemical similarities in their overall composition.121\nHistorically, the field of nanomedicine and the global endeavor to generate NPs for drug delivery arose from one of the greatest struggles in medicine: gene therapy. At the core of that approach was the idea that viruses could be used as Trojan horses to deliver the correct sequence of the gene into the cells that harbored the mutated copy. In order to identify the virus that offered the best delivery service, a cadre of viral strains and species were tested, adapted, and engineered to fit the aims and hit the targets. It took decades of trial and error, of progressive adjustments, and a few tragic mistakes to identify the viruses that could provide the ideal backbone to develop viral vectors able to ferry genetic cargo into the target cell.122 In the midst of that global challenge, nanotechnology offered a safer and more controllable alternative: to generate bespoke structures that could replace viral vectors and do the same job, delivering a payload from the point of injection to the site of action.\nFast forward a few decades, and the promises made by both worlds seem to have finally become reality, with a number of active clinical trials, some clinical success stories, and a few commercial products in the market space. Interestingly, the two worlds seem to have maintained a safe distance so as to avoid any collision or dangerous proximity. Exchange of ideas between the fields of gene therapy and nanomedicine is limited, and the potential for disciplinary growth through knowledge exchange has remained elusive to scientists in both fields.5 Many scientists have suggested that nanotechnologists could find inspiration in the mechanisms devised by viruses to elude immune surveillance, to overcome biological barriers, and to deliver their genetic payload with high specificity. Similarly, gene therapists would find high-tech solutions to their scalability and safety issues in looking at the new generations of biomimetic particles being generated.\nThe principles of biomimicry and bioinspiration have been used to design and to engineer drug-delivery technologies that reproduce or recapitulate biological materials, for what pertains to not only their structure and chemistry but also, more importantly, their functions. In drug delivery, surface recognition and nanoscale interactions between materials and biological entities are key to the success of the delivery strategy, and the use of biological building blocks such as membrane proteins has been proposed as a way to convey targeting and shielding moieties simultaneously.123 One can only hope that the recent events and global attention that viruses are capturing in the scientific world will spur a renewed interest in finding ways to adapt viral features and mechanisms of action to the world of NPs. Increased focus in this field would be useful to create virus-like NPs able to circulate in the blood system, overcoming the endothelial barrier, and to deliver their therapeutic payload with high efficiency.124\n\nInterference with Cellular Uptake, Immobilization, and Inactivation of the Virus Outside of the Host Cell\nNanomaterials can be synthesized with a high specific surface area of a few hundred square meters per gram. Therefore, dependent on the surface properties, nanomaterials efficiently adsorb biomolecules and form a so-called biomolecular corona. This passive, nontargeted adsorption might be utilized to bind viruses, provided that the selected nanomaterial is relatively biocompatible. Viral surface proteins are often modified by sugar moieties or encompass positively charged amino acid patches that bind to lectins or glycosaminoglycans (GAGs) of heparan sulfate (HS), respectively.32 Robust interactions of virus particles with these host receptors is ensured by multivalent binding, which is likely why single-molecule inhibitors often are not capable of efficiently perturbing this key event but multivalent NPs are superior to block binding of different viruses to the host cell.125\nGold NPs capped with mercaptoethanesulfonate are effective inhibitors of HSV type 1 infection as they mimic cell-surface-receptor heparan sulfate and, therefore, competitively bind to the virus. Interestingly, polyvalent sulfated Au NPs inhibit virus binding to the host cell dependent on their size. Nanoparticles of diameters equal to and larger than the virus diameter (in this case, the stomatitis virus) more efficiently inhibit the binding to cells than smaller particles. Most likely, larger NPs efficiently cross-link virions, whereas smaller NPs simply decorate the viral surface.126 Papp et al. found that gold NPs decorated with SA effectively inhibited the binding of influenza virus to the target cells. In this case, viral recognition via its surface protein hemagglutinin of SA on the host cell membrane was a prerequisite for cellular entry.127 More recently, Cagno et al. reported antiviral NPs (Au and iron oxide core) with long and flexible linkers mimicking HS that strongly bind and inactivate viruses such as respiratory syncytial virus in vitro and in vivo in a lung infection model and even led to irreversible viral deformation.53 Hence, there is ample evidence that biocompatible, functionalized NPs can act as broad-spectrum antivirals. Notably, the receptor-binding domain of the spike S1 protein of SARS-CoV-2 binds not only to ACE2 but also to heparin128 and, thus, might be targeted by similar approaches as outlined above.\nOne of the most recent strategies to inhibit viral uptake is based on administration of recombinant ACE2 to inhibit binding competitively via the spike S1 protein.21 Knowing that multivalency is key to block virus–host interactions reliably, researchers have speculated that a nanostructured carrier could not only improve delivery and cargo stability but also might dramatically enhance binding strength.129\n\nSpeeding up the Nanomedicine-Based Approaches for COVID-19 by In Silico Analysis\nCurrently, repurposing drug molecules is a key strategy for identifying approved or investigational drugs outside the scope of the original medical indication that can be used to fight COVID-19.130,131 There are various advantages to this strategy, including already-established safety profiles, fast transition to clinical studies, and less investment needed, compared to the process of developing an entirely new drug.132,133 Therefore, these advantages have the potential to result in less risky and more rapid returns on investment in the development of repurposed drugs, benefits that are particularly important during pandemics. In addition to rapid diagnosis, making recommendations to physicians about rapid treatment methods can save the lives of many people. Combining in silico tools such as molecular docking, molecular dynamics, and computational chemistry with large drug databases provides a great advantage in selecting “possible candidates” from among thousands of pharmaceutically active substances (Figure 3). In silico analyses should be supported by the literature, expert opinions (pharmacologists and clinicians), and, when possible, preclinical and clinical findings. After selecting the best candidates following in silico analyses, detailed in vitro and in vivo experimentation should be undertaken prior to clinical studies. Recently, various drug-repurposing studies have been published to assist in the global COVID-19 pandemic response.134−136 Such studies aim to identify whether already-approved drugs have the capacity to interact with viral proteins or receptors on host cells.\nFigure 3 Schematic of how in silico analysis can be used to select candidate drug molecules for clinical studies. MD, molecular dynamics. In nanomedicine, computational models have recently garnered attention; such work may help to identify how nanomaterials interact with biological systems and to determine how the efficacy of these nanotherapeutics could be improved. Computational models indicate how NPs are taken up by healthy cells or tumor cells, enabling better predictions regarding the pharmacokinetic and pharmacodynamic properties of these materials.134 For example, Lunnoo et al. used a coarse-grained molecular dynamics (MD) simulation to observe the internalization pathways of various Au nanostructures (nanospheres, nanocages, nanorods, nanoplates, and nanohexapods) into an idealized mammalian plasma membrane.137 Other studies have simulated how different NPs can target tumor cells and deliver drugs.138,139 Therefore, in silico approaches that are currently used for drug repurposing, including molecular docking, molecular dynamics, and computational chemistry, constitute valuable tools to aid preclinical and clinical studies of nanomaterials directed for disease treatment. Considering the urgent need for nanomedicine against the current pandemic, in silico analyses may be especially useful in guiding the rational design of new NP formulations required to fight SARS-CoV-2."}