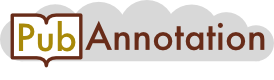
PMC:7152911 / 59810-72704
Annnotations
LitCovid-PubTator
{"project":"LitCovid-PubTator","denotations":[{"id":"1363","span":{"begin":1331,"end":1339},"obj":"Chemical"},{"id":"1379","span":{"begin":5312,"end":5321},"obj":"Gene"},{"id":"1380","span":{"begin":5142,"end":5151},"obj":"Gene"},{"id":"1381","span":{"begin":4912,"end":4914},"obj":"Gene"},{"id":"1382","span":{"begin":4771,"end":4773},"obj":"Gene"},{"id":"1383","span":{"begin":4746,"end":4748},"obj":"Gene"},{"id":"1384","span":{"begin":4693,"end":4700},"obj":"Species"},{"id":"1385","span":{"begin":4852,"end":4859},"obj":"Species"},{"id":"1386","span":{"begin":4988,"end":5009},"obj":"Species"},{"id":"1387","span":{"begin":5010,"end":5014},"obj":"Species"},{"id":"1388","span":{"begin":5018,"end":5025},"obj":"Species"},{"id":"1389","span":{"begin":5223,"end":5245},"obj":"Species"},{"id":"1390","span":{"begin":5247,"end":5256},"obj":"Species"},{"id":"1391","span":{"begin":4635,"end":4642},"obj":"Species"},{"id":"1392","span":{"begin":4827,"end":4834},"obj":"Species"},{"id":"1393","span":{"begin":3563,"end":3571},"obj":"Disease"},{"id":"1397","span":{"begin":6705,"end":6712},"obj":"Species"},{"id":"1398","span":{"begin":6942,"end":6955},"obj":"Chemical"},{"id":"1399","span":{"begin":7047,"end":7060},"obj":"Disease"},{"id":"1401","span":{"begin":7098,"end":7114},"obj":"Chemical"},{"id":"1411","span":{"begin":7479,"end":7502},"obj":"Species"},{"id":"1412","span":{"begin":7504,"end":7508},"obj":"Species"},{"id":"1413","span":{"begin":7115,"end":7129},"obj":"Chemical"},{"id":"1414","span":{"begin":7134,"end":7147},"obj":"Chemical"},{"id":"1415","span":{"begin":7174,"end":7186},"obj":"Chemical"},{"id":"1416","span":{"begin":7226,"end":7239},"obj":"Chemical"},{"id":"1417","span":{"begin":7436,"end":7449},"obj":"Chemical"},{"id":"1418","span":{"begin":7540,"end":7553},"obj":"Chemical"},{"id":"1419","span":{"begin":7628,"end":7640},"obj":"Chemical"},{"id":"1421","span":{"begin":7772,"end":7788},"obj":"Chemical"},{"id":"1425","span":{"begin":9079,"end":9088},"obj":"Species"},{"id":"1426","span":{"begin":8082,"end":8098},"obj":"Chemical"},{"id":"1427","span":{"begin":8484,"end":8499},"obj":"Chemical"},{"id":"1437","span":{"begin":10691,"end":10693},"obj":"Gene"},{"id":"1438","span":{"begin":9881,"end":9894},"obj":"Species"},{"id":"1439","span":{"begin":10009,"end":10016},"obj":"Species"},{"id":"1440","span":{"begin":10205,"end":10212},"obj":"Species"},{"id":"1441","span":{"begin":10549,"end":10562},"obj":"Species"},{"id":"1442","span":{"begin":10645,"end":10655},"obj":"Species"},{"id":"1443","span":{"begin":10303,"end":10308},"obj":"Chemical"},{"id":"1444","span":{"begin":10599,"end":10601},"obj":"Chemical"},{"id":"1445","span":{"begin":10812,"end":10817},"obj":"Chemical"},{"id":"1448","span":{"begin":11491,"end":11495},"obj":"Disease"},{"id":"1449","span":{"begin":11539,"end":11543},"obj":"Disease"},{"id":"1462","span":{"begin":11891,"end":11898},"obj":"Species"},{"id":"1463","span":{"begin":12045,"end":12071},"obj":"Species"},{"id":"1464","span":{"begin":12073,"end":12087},"obj":"Species"},{"id":"1465","span":{"begin":11770,"end":11782},"obj":"Chemical"},{"id":"1466","span":{"begin":11822,"end":11856},"obj":"Chemical"},{"id":"1467","span":{"begin":11858,"end":11862},"obj":"Chemical"},{"id":"1468","span":{"begin":11990,"end":12021},"obj":"Chemical"},{"id":"1469","span":{"begin":11791,"end":11817},"obj":"Disease"},{"id":"1470","span":{"begin":12187,"end":12191},"obj":"Disease"},{"id":"1471","span":{"begin":12370,"end":12374},"obj":"Disease"},{"id":"1472","span":{"begin":12630,"end":12634},"obj":"Disease"},{"id":"1473","span":{"begin":12683,"end":12687},"obj":"Disease"}],"attributes":[{"id":"A1363","pred":"tao:has_database_id","subj":"1363","obj":"MESH:D011108"},{"id":"A1379","pred":"tao:has_database_id","subj":"1379","obj":"Gene:2960"},{"id":"A1380","pred":"tao:has_database_id","subj":"1380","obj":"Gene:2960"},{"id":"A1381","pred":"tao:has_database_id","subj":"1381","obj":"Gene:21832"},{"id":"A1382","pred":"tao:has_database_id","subj":"1382","obj":"Gene:21832"},{"id":"A1383","pred":"tao:has_database_id","subj":"1383","obj":"Gene:21832"},{"id":"A1384","pred":"tao:has_database_id","subj":"1384","obj":"Tax:562"},{"id":"A1385","pred":"tao:has_database_id","subj":"1385","obj":"Tax:562"},{"id":"A1386","pred":"tao:has_database_id","subj":"1386","obj":"Tax:11309"},{"id":"A1387","pred":"tao:has_database_id","subj":"1387","obj":"Tax:102793"},{"id":"A1388","pred":"tao:has_database_id","subj":"1388","obj":"Tax:9031"},{"id":"A1389","pred":"tao:has_database_id","subj":"1389","obj":"Tax:5807"},{"id":"A1390","pred":"tao:has_database_id","subj":"1390","obj":"Tax:5807"},{"id":"A1391","pred":"tao:has_database_id","subj":"1391","obj":"Tax:562"},{"id":"A1392","pred":"tao:has_database_id","subj":"1392","obj":"Tax:562"},{"id":"A1393","pred":"tao:has_database_id","subj":"1393","obj":"MESH:D007239"},{"id":"A1397","pred":"tao:has_database_id","subj":"1397","obj":"Tax:562"},{"id":"A1398","pred":"tao:has_database_id","subj":"1398","obj":"MESH:D009842"},{"id":"A1399","pred":"tao:has_database_id","subj":"1399","obj":"MESH:D001943"},{"id":"A1401","pred":"tao:has_database_id","subj":"1401","obj":"MESH:D009844"},{"id":"A1411","pred":"tao:has_database_id","subj":"1411","obj":"Tax:11320"},{"id":"A1412","pred":"tao:has_database_id","subj":"1412","obj":"Tax:114727"},{"id":"A1413","pred":"tao:has_database_id","subj":"1413","obj":"MESH:D014312"},{"id":"A1414","pred":"tao:has_database_id","subj":"1414","obj":"MESH:D002241"},{"id":"A1415","pred":"tao:has_database_id","subj":"1415","obj":"MESH:D002241"},{"id":"A1416","pred":"tao:has_database_id","subj":"1416","obj":"MESH:D014312"},{"id":"A1417","pred":"tao:has_database_id","subj":"1417","obj":"MESH:D014312"},{"id":"A1418","pred":"tao:has_database_id","subj":"1418","obj":"MESH:D002241"},{"id":"A1419","pred":"tao:has_database_id","subj":"1419","obj":"MESH:D002241"},{"id":"A1421","pred":"tao:has_database_id","subj":"1421","obj":"MESH:D009841"},{"id":"A1425","pred":"tao:has_database_id","subj":"1425","obj":"Tax:5807"},{"id":"A1426","pred":"tao:has_database_id","subj":"1426","obj":"MESH:D009841"},{"id":"A1427","pred":"tao:has_database_id","subj":"1427","obj":"MESH:D009841"},{"id":"A1437","pred":"tao:has_database_id","subj":"1437","obj":"Gene:21832"},{"id":"A1438","pred":"tao:has_database_id","subj":"1438","obj":"Tax:38018"},{"id":"A1439","pred":"tao:has_database_id","subj":"1439","obj":"Tax:562"},{"id":"A1440","pred":"tao:has_database_id","subj":"1440","obj":"Tax:562"},{"id":"A1441","pred":"tao:has_database_id","subj":"1441","obj":"Tax:38018"},{"id":"A1442","pred":"tao:has_database_id","subj":"1442","obj":"Tax:1642"},{"id":"A1443","pred":"tao:has_database_id","subj":"1443","obj":"MESH:D014867"},{"id":"A1444","pred":"tao:has_database_id","subj":"1444","obj":"MESH:D006046"},{"id":"A1445","pred":"tao:has_database_id","subj":"1445","obj":"MESH:D014867"},{"id":"A1462","pred":"tao:has_database_id","subj":"1462","obj":"Tax:562"},{"id":"A1463","pred":"tao:has_database_id","subj":"1463","obj":"Tax:1282"},{"id":"A1464","pred":"tao:has_database_id","subj":"1464","obj":"Tax:1282"},{"id":"A1466","pred":"tao:has_database_id","subj":"1466","obj":"MESH:C102833"},{"id":"A1467","pred":"tao:has_database_id","subj":"1467","obj":"MESH:C102833"}],"namespaces":[{"prefix":"Tax","uri":"https://www.ncbi.nlm.nih.gov/taxonomy/"},{"prefix":"MESH","uri":"https://id.nlm.nih.gov/mesh/"},{"prefix":"Gene","uri":"https://www.ncbi.nlm.nih.gov/gene/"},{"prefix":"CVCL","uri":"https://web.expasy.org/cellosaurus/CVCL_"}],"text":"2.2 Biorecognition elements\nThe previous section discussed the transduction elements associated with pathogen detection using electrochemical biosensors. Given a biosensor is a device composed of integrated transducer and biorecognition elements, we next discuss the biorecognition elements used for selective detection of pathogens and corresponding immobilization techniques for their coupling to electrodes.\nBiorecognition elements for electrochemical biosensors can be defined as (1) biocatalytic or (2) biocomplexing. In the case of biocatalytic biorecognition elements, the biosensor response is based on a reaction catalyzed by macromolecules. Enzymes, whole cells, and tissues are the most commonly used biocatalytic biorecognition element. While enzyzmes provide biorecognition elements in various chemical sensing applications, they are often used as labels for pathogen detection applications and most commonly introduced via secondary binding steps. In the case of biocomplexing biorecognition elements, the biosensor response is based on the interaction of analytes with macromolecules or organized molecular assemblies. As shown in Table 1, Table 2, antibodies, peptides, and phages are the most commonly used biocomplexing biorecognition elements for pathogen detection. In addition to biomacromolecules, imprinted polymers have also been examined as biocomplexing biorecognition elements for pathogen detection using electrochemical biosensors.\n\n2.2.1 Antibodies and antibody fragments\nAntibodies and antibody fragments are among the most commonly utilized biorecognition elements for pathogen detection using electrochemical biosensors. Biosensors employing antibody-based biorecognition elements are commonly referred to as immunosensors. Given antibodies exhibit high selectivity and binding affinity for target species and can be generated for a wide range of infectious agents, antibodies are the gold-standard biorecognition element for pathogen detection. Antibodies contain recognition sites that selectively bind to antigens through a specific region of the antigen, referred to as an epitope (Patris et al. 2016). Antibodies can be labeled with fluorescent or enzymatic tags, which leads to the designation of the approach as label-based. While label-based approaches present measurement constraints associated with the use of additional reagents and processing steps (Cooper, 2009; Sang et al. 2016), antibody labeling may also alter the binding affinity to the antigen, which could affect the biosensor's selectivity. A detailed discussion of label-based biosensing approaches for pathogen detection has been reported elsewhere (Ahmed et al. 2014; Alahi and Mukhopadhyay, 2017; Bozal-Palabiyik et al. 2018; Leonard et al. 2003). A list of recent label-based approaches for pathogen detection using electrochemical biosensors, however, is provided in Table 2.\nWhile both monoclonal and polyclonal antibodies enable the selective detection of pathogens (Patris et al. 2016), they vary in terms of production method, selectivity, and binding affinity. Monoclonal antibodies are produced by hybridoma technology (Birch and Racher, 2006; James and Bell, 1987). Thus, monoclonal antibodies are highly selective and bind to a single epitope, making them less vulnerable to cross-reactivity. While monoclonal antibodies tend to have a higher degree of selectivity, they are more expensive and take longer to develop than polyclonal antibodies. Polyclonal antibodies are produced by separation of immunoglobulin proteins from the blood of an infected host (Birch and Racher, 2006). Polyclonal antibodies target different epitopes on a single antigen. While polyclonal antibodies exhibit increased variability between batches, they are relatively less expensive to produce than monoclonal antibodies and facilitate robust measurements in various settings (Byrne et al. 2009). Drawbacks to antibody use include high cost and stability challenges, such as the need for low-temperature storage. As shown in Table 1, Table 2, both monoclonal and polyclonal antibodies are used as biorecognition elements for pathogen detection. For assays involving secondary binding steps, monoclonal antibodies typically serve as the primary biorecognition element and are immobilized on the electrode, while polyclonal antibodies serve as the secondary biorecognition element and often facilitate target labeling. For assays that do not require secondary binding steps, polyclonal antibodies are also commonly used as immobilized biorecognition elements for pathogen detection. For example, Pandey et al. immobilized monoclonal anti-E. coli on a composite nanostructured electrode to detect E. coli across a wide dynamic range of 10 to 108 CFU/mL with a LOD of 3.8 CFU/mL (Pandey et al. 2017). Wu et al. used polyclonal anti-E. coli for detection of E. coli via amperometry that exhibited a LOD of 5 × 103 CFU/mL (Wu et al. 2016). Lin et al. used monoclonal antibodies for detection of avian influenza virus H5N1 in chicken swabs across a dynamic range of 2- 1 to 24 hemagglutination units (HAU)/50 μL using EIS and the ferri/ferrocyanide (Fe(CN)6 3 - /4-) couple as a redox probe (Lin et al. 2015). Luka et al. detected Cryptosporidium parvum (C. parvum) with a LOD of 40 cells/mm2 via capacitive sensing and Fe(CN)6 3 - /4- (Luka et al. 2019).\nAntibody fragments, such as single-chain variable fragments (scFvs), offer selectivity similar to antibodies, but they have the advantage of achieving relatively higher packing densities on electrode surfaces due to their relatively smaller size. For example, half-antibody fragments have been shown to improve biosensor sensitivity without the loss of selectivity, which warrants further investigation of reduced antibodies as biorecognition elements for pathogen detection applications (Sharma and Mutharasan, 2013). In addition to scFvs, Fabs, re-engineered IgGs, and dimers can also potentially be used as biorecognition elements for pathogen detection (Byrne et al. 2009).\n\n2.2.2 Carbohydrate-binding proteins\nCarbohydrate-binding proteins, such as lectins, also provide selective biorecognition elements for pathogen detection based on their ability to selectively bind ligands on target species. Peptide-based biorecognition elements are relatively low-cost, can be produced with high yield automated synthesis processes, and are modifiable (Pavan and Berti, 2012). For example, lectins have been investigated as biorecognition elements for pathogen detection through their ability to selectively bind glycosylated proteins on the surfaces of viruses and cells (Reina et al. 2008). Concanavalin A (ConA) lectin has been extensively investigated for E. coli detection (see Table 1) (Jantra et al. 2011; Saucedo et al. 2019; Xi et al. 2011; Yang et al. 2016b). While not yet widely investigated for pathogen detection using electrochemical biosensors, Etayash et al. recently showed that oligopeptides also provide attractive biorecognition elements for real-time biosensor-based detection of breast cancer cells (Etayash et al. 2015).\n\n2.2.3 Oligosaccharides\nTrisaccharides are carbohydrates that can selectively bind carbohydrate-specific receptors on pathogens. Thus, trisaccharide ligands have been used as biorecognition elements for pathogen detection using electrochemical biosensors. For example, Hai et al. used a hybrid E-QCM biosensor coated with hemagglutinin-specific trisaccharide ligands for the detection of human influenza A virus (H1N1) (Hai et al. 2017). The use of carbohydrates as biorecognition elements is limited in part due to the weak affinity of carbohydrate-protein interactions and low selectivity, which are currently mitigated through secondary interactions (Zeng et al. 2012).\n\n2.2.4 Oligonucleotides\nSingle-stranded DNA (ssDNA) is a useful biorecognition element for the detection of pathogens. While ssDNA is commonly used as a biorecognition element for DNA-based assays, ssDNA aptamers are commonly used for pathogen detection using electrochemical biosensors. Aptamers are single-stranded oligonucleotides capable of binding various molecules with high affinity and selectivity (Lakhin et al. 2013; Reverdatto et al. 2015). Aptamers are isolated from a large random sequence pool through a selection process that utilizes systematic evolution of ligands by exponential enrichment, also known as SELEX (Stoltenburg et al. 2007). Suitable binding sequences can be isolated from a large random oligonucleotide sequence pool and subsequently amplified for use. Thus, aptamers can exhibit high selectivity to target species (Stoltenburg et al. 2007). Aptamers can also be produced at a lower cost than alternative biorecognition elements, such as antibodies. Giamberardino et al. used SELEX to discover an aptamer for norovirus detection, which showed a million-fold higher binding affinity for the target than a random DNA strand that served as a negative control (Giamberardino et al. 2013). Iqbal et al. performed 10 rounds of SELEX to discover 14 aptamer clones with high affinities for C. parvum for detection in fruit samples (Iqbal et al. 2015). However, the use of aptamers as biorecognition elements has not yet replaced traditional biorecognition elements, such as antibodies, because of several challenges, such as aptamer stability, degradation, cross-reactivity, and reproducibility using alternative processing approaches (Lakhin et al. 2013).\n\n2.2.5 Phages\nPhages, also referred to as bacteriophages, are viruses that infect and replicate in bacteria through selective binding via tail-spike proteins (Haq et al. 2012). Thus, they have been examined as biorecognition elements for pathogen detection using electrochemical biosensors (Kutter and Sulakvelidze, 2004). Bacteriophages exhibit varying morphologies and are thus classified by selectivity and structure. A variety of bacteriophage-based electrochemical biosensors for pathogen detection can be found in Table 1. For example, Shabani et al. used E. coli-specific T4 bacteriophages for selective impedimetric detection studies (Shabani et al. 2008). Mejri et al. compared the use of bacteriophages to antibodies as biorecognition elements for E. coli detection (Mejri et al. 2010). In that study, they found that bacteriophages improved the water stability of the biosensor and increased the sensitivity by approximately a factor of four relative to the response obtained with antibodies based on EIS measurements (Mejri et al. 2010). In another study, Tolba et al. utilized immobilized bacteriophage-encoded peptidoglycan hydrolases on Au screen-printed electrodes for detection of L. innocua in pure milk with a LOD of 105 CFU/mL (Tolba et al. 2012). These results suggest that bacteriophages are potentially attractive biorecognition elements for water safety and environmental monitoring applications that require chronic monitoring of liquids.\n\n2.2.6 Cell- and molecularly-imprinted polymers\nGiven traditional biorecognition elements used in biosensing exhibit stability concerns, such as antibodies or aptamers, as discussed in Sections 2.2.1–2.2.4, there have been efforts to create engineered molecular biorecognition elements, such as scFvs. In contrast, materials-based biorecognition elements exploit the principle of target-specific morphology for selective capture (Pan et al. 2018; Zhou et al. 2019). The most common approach in materials-based biorecognition is based on cell- and molecularly-imprinted polymers (CIPs and MIPs, respectively) (Gui et al. 2018). CIPs and MIPs have been created using various processes, including bacteria-mediated lithography, micro-contact stamping, and colloid imprints (Chen et al. 2016a; Pan et al. 2018).\nAs shown in Fig. 3b, Jafari et al. used imprinted organosilica sol-gel films of tetraethoxysilane and (3-mercaptopropyl)trimethoxysilane (MPTS) for selective detection of E. coli using an impedimetric method (Jafari et al. 2019). Similarly, Golabi et al. used imprinted poly(3-aminophenylboronic acid) films for detection of Staphylococcus epidermidis (S. epidermidis) (Golabi et al. 2017). Despite the absence of a highly selective molecular biorecognition element, CIPs and MIPs exhibit selectivity when exposed to samples that contain multiple analytes (i.e., non-target species) (Golabi et al. 2017; Jafari et al. 2019; Qi et al. 2013). MIPs and CIPs are also of interest with regard to opportunities in biosensor regeneration. Common adverse effects of regeneration on biosensors that employ molecular biorecognition elements, such as irreversible changes in structure, are less likely to affect MIPs and CIPs. However, it is generally accepted that current CIPs and MIPs exhibit lower selectivity to target species than antibodies and aptamers due to reduction of available chemical selectivity (Cheong et al. 2013; Kryscio and Peppas, 2012; Yáñez-Sedeño et al. 2017)."}
LitCovid-PD-FMA-UBERON
{"project":"LitCovid-PD-FMA-UBERON","denotations":[{"id":"T121","span":{"begin":667,"end":672},"obj":"Body_part"},{"id":"T122","span":{"begin":678,"end":685},"obj":"Body_part"},{"id":"T123","span":{"begin":1470,"end":1480},"obj":"Body_part"},{"id":"T124","span":{"begin":1485,"end":1493},"obj":"Body_part"},{"id":"T125","span":{"begin":1504,"end":1514},"obj":"Body_part"},{"id":"T126","span":{"begin":1519,"end":1527},"obj":"Body_part"},{"id":"T127","span":{"begin":1677,"end":1685},"obj":"Body_part"},{"id":"T128","span":{"begin":1981,"end":1991},"obj":"Body_part"},{"id":"T129","span":{"begin":2142,"end":2152},"obj":"Body_part"},{"id":"T130","span":{"begin":2430,"end":2438},"obj":"Body_part"},{"id":"T131","span":{"begin":3518,"end":3532},"obj":"Body_part"},{"id":"T132","span":{"begin":3533,"end":3541},"obj":"Body_part"},{"id":"T133","span":{"begin":3551,"end":3556},"obj":"Body_part"},{"id":"T134","span":{"begin":3909,"end":3917},"obj":"Body_part"},{"id":"T135","span":{"begin":5275,"end":5280},"obj":"Body_part"},{"id":"T136","span":{"begin":5348,"end":5356},"obj":"Body_part"},{"id":"T137","span":{"begin":5613,"end":5621},"obj":"Body_part"},{"id":"T138","span":{"begin":6034,"end":6046},"obj":"Body_part"},{"id":"T139","span":{"begin":6055,"end":6063},"obj":"Body_part"},{"id":"T140","span":{"begin":6064,"end":6076},"obj":"Body_part"},{"id":"T141","span":{"begin":6085,"end":6093},"obj":"Body_part"},{"id":"T142","span":{"begin":6571,"end":6579},"obj":"Body_part"},{"id":"T143","span":{"begin":6611,"end":6616},"obj":"Body_part"},{"id":"T144","span":{"begin":7047,"end":7053},"obj":"Body_part"},{"id":"T145","span":{"begin":7061,"end":7066},"obj":"Body_part"},{"id":"T146","span":{"begin":7098,"end":7114},"obj":"Body_part"},{"id":"T147","span":{"begin":7134,"end":7147},"obj":"Body_part"},{"id":"T148","span":{"begin":7174,"end":7186},"obj":"Body_part"},{"id":"T149","span":{"begin":7540,"end":7553},"obj":"Body_part"},{"id":"T150","span":{"begin":7628,"end":7640},"obj":"Body_part"},{"id":"T151","span":{"begin":7641,"end":7648},"obj":"Body_part"},{"id":"T152","span":{"begin":7805,"end":7808},"obj":"Body_part"},{"id":"T153","span":{"begin":7945,"end":7948},"obj":"Body_part"},{"id":"T154","span":{"begin":8908,"end":8911},"obj":"Body_part"},{"id":"T155","span":{"begin":9596,"end":9604},"obj":"Body_part"},{"id":"T156","span":{"begin":10571,"end":10584},"obj":"Body_part"},{"id":"T157","span":{"begin":10664,"end":10668},"obj":"Body_part"},{"id":"T158","span":{"begin":10919,"end":10923},"obj":"Body_part"},{"id":"T159","span":{"begin":11449,"end":11453},"obj":"Body_part"}],"attributes":[{"id":"A121","pred":"fma_id","subj":"T121","obj":"http://purl.org/sig/ont/fma/fma68646"},{"id":"A122","pred":"fma_id","subj":"T122","obj":"http://purl.org/sig/ont/fma/fma9637"},{"id":"A123","pred":"fma_id","subj":"T123","obj":"http://purl.org/sig/ont/fma/fma62871"},{"id":"A124","pred":"fma_id","subj":"T124","obj":"http://purl.org/sig/ont/fma/fma62871"},{"id":"A125","pred":"fma_id","subj":"T125","obj":"http://purl.org/sig/ont/fma/fma62871"},{"id":"A126","pred":"fma_id","subj":"T126","obj":"http://purl.org/sig/ont/fma/fma62871"},{"id":"A127","pred":"fma_id","subj":"T127","obj":"http://purl.org/sig/ont/fma/fma62871"},{"id":"A128","pred":"fma_id","subj":"T128","obj":"http://purl.org/sig/ont/fma/fma62871"},{"id":"A129","pred":"fma_id","subj":"T129","obj":"http://purl.org/sig/ont/fma/fma62871"},{"id":"A130","pred":"fma_id","subj":"T130","obj":"http://purl.org/sig/ont/fma/fma62871"},{"id":"A131","pred":"fma_id","subj":"T131","obj":"http://purl.org/sig/ont/fma/fma62871"},{"id":"A132","pred":"fma_id","subj":"T132","obj":"http://purl.org/sig/ont/fma/fma67257"},{"id":"A133","pred":"fma_id","subj":"T133","obj":"http://purl.org/sig/ont/fma/fma9670"},{"id":"A134","pred":"fma_id","subj":"T134","obj":"http://purl.org/sig/ont/fma/fma62871"},{"id":"A135","pred":"fma_id","subj":"T135","obj":"http://purl.org/sig/ont/fma/fma68646"},{"id":"A136","pred":"fma_id","subj":"T136","obj":"http://purl.org/sig/ont/fma/fma62871"},{"id":"A137","pred":"fma_id","subj":"T137","obj":"http://purl.org/sig/ont/fma/fma62871"},{"id":"A138","pred":"fma_id","subj":"T138","obj":"http://purl.org/sig/ont/fma/fma82737"},{"id":"A139","pred":"fma_id","subj":"T139","obj":"http://purl.org/sig/ont/fma/fma67257"},{"id":"A140","pred":"fma_id","subj":"T140","obj":"http://purl.org/sig/ont/fma/fma82737"},{"id":"A141","pred":"fma_id","subj":"T141","obj":"http://purl.org/sig/ont/fma/fma67257"},{"id":"A142","pred":"fma_id","subj":"T142","obj":"http://purl.org/sig/ont/fma/fma67257"},{"id":"A143","pred":"fma_id","subj":"T143","obj":"http://purl.org/sig/ont/fma/fma68646"},{"id":"A144","pred":"fma_id","subj":"T144","obj":"http://purl.org/sig/ont/fma/fma9601"},{"id":"A145","pred":"fma_id","subj":"T145","obj":"http://purl.org/sig/ont/fma/fma68646"},{"id":"A146","pred":"fma_id","subj":"T146","obj":"http://purl.org/sig/ont/fma/fma82742"},{"id":"A147","pred":"fma_id","subj":"T147","obj":"http://purl.org/sig/ont/fma/fma82737"},{"id":"A148","pred":"fma_id","subj":"T148","obj":"http://purl.org/sig/ont/fma/fma82737"},{"id":"A149","pred":"fma_id","subj":"T149","obj":"http://purl.org/sig/ont/fma/fma82737"},{"id":"A150","pred":"fma_id","subj":"T150","obj":"http://purl.org/sig/ont/fma/fma82737"},{"id":"A151","pred":"fma_id","subj":"T151","obj":"http://purl.org/sig/ont/fma/fma67257"},{"id":"A152","pred":"fma_id","subj":"T152","obj":"http://purl.org/sig/ont/fma/fma74412"},{"id":"A153","pred":"fma_id","subj":"T153","obj":"http://purl.org/sig/ont/fma/fma74412"},{"id":"A154","pred":"fma_id","subj":"T154","obj":"http://purl.org/sig/ont/fma/fma74412"},{"id":"A155","pred":"fma_id","subj":"T155","obj":"http://purl.org/sig/ont/fma/fma67257"},{"id":"A156","pred":"fma_id","subj":"T156","obj":"http://purl.org/sig/ont/fma/fma82783"},{"id":"A157","pred":"fma_id","subj":"T157","obj":"http://purl.org/sig/ont/fma/fma62100"},{"id":"A158","pred":"fma_id","subj":"T158","obj":"http://purl.org/sig/ont/fma/fma68646"},{"id":"A159","pred":"fma_id","subj":"T159","obj":"http://purl.org/sig/ont/fma/fma68646"}],"text":"2.2 Biorecognition elements\nThe previous section discussed the transduction elements associated with pathogen detection using electrochemical biosensors. Given a biosensor is a device composed of integrated transducer and biorecognition elements, we next discuss the biorecognition elements used for selective detection of pathogens and corresponding immobilization techniques for their coupling to electrodes.\nBiorecognition elements for electrochemical biosensors can be defined as (1) biocatalytic or (2) biocomplexing. In the case of biocatalytic biorecognition elements, the biosensor response is based on a reaction catalyzed by macromolecules. Enzymes, whole cells, and tissues are the most commonly used biocatalytic biorecognition element. While enzyzmes provide biorecognition elements in various chemical sensing applications, they are often used as labels for pathogen detection applications and most commonly introduced via secondary binding steps. In the case of biocomplexing biorecognition elements, the biosensor response is based on the interaction of analytes with macromolecules or organized molecular assemblies. As shown in Table 1, Table 2, antibodies, peptides, and phages are the most commonly used biocomplexing biorecognition elements for pathogen detection. In addition to biomacromolecules, imprinted polymers have also been examined as biocomplexing biorecognition elements for pathogen detection using electrochemical biosensors.\n\n2.2.1 Antibodies and antibody fragments\nAntibodies and antibody fragments are among the most commonly utilized biorecognition elements for pathogen detection using electrochemical biosensors. Biosensors employing antibody-based biorecognition elements are commonly referred to as immunosensors. Given antibodies exhibit high selectivity and binding affinity for target species and can be generated for a wide range of infectious agents, antibodies are the gold-standard biorecognition element for pathogen detection. Antibodies contain recognition sites that selectively bind to antigens through a specific region of the antigen, referred to as an epitope (Patris et al. 2016). Antibodies can be labeled with fluorescent or enzymatic tags, which leads to the designation of the approach as label-based. While label-based approaches present measurement constraints associated with the use of additional reagents and processing steps (Cooper, 2009; Sang et al. 2016), antibody labeling may also alter the binding affinity to the antigen, which could affect the biosensor's selectivity. A detailed discussion of label-based biosensing approaches for pathogen detection has been reported elsewhere (Ahmed et al. 2014; Alahi and Mukhopadhyay, 2017; Bozal-Palabiyik et al. 2018; Leonard et al. 2003). A list of recent label-based approaches for pathogen detection using electrochemical biosensors, however, is provided in Table 2.\nWhile both monoclonal and polyclonal antibodies enable the selective detection of pathogens (Patris et al. 2016), they vary in terms of production method, selectivity, and binding affinity. Monoclonal antibodies are produced by hybridoma technology (Birch and Racher, 2006; James and Bell, 1987). Thus, monoclonal antibodies are highly selective and bind to a single epitope, making them less vulnerable to cross-reactivity. While monoclonal antibodies tend to have a higher degree of selectivity, they are more expensive and take longer to develop than polyclonal antibodies. Polyclonal antibodies are produced by separation of immunoglobulin proteins from the blood of an infected host (Birch and Racher, 2006). Polyclonal antibodies target different epitopes on a single antigen. While polyclonal antibodies exhibit increased variability between batches, they are relatively less expensive to produce than monoclonal antibodies and facilitate robust measurements in various settings (Byrne et al. 2009). Drawbacks to antibody use include high cost and stability challenges, such as the need for low-temperature storage. As shown in Table 1, Table 2, both monoclonal and polyclonal antibodies are used as biorecognition elements for pathogen detection. For assays involving secondary binding steps, monoclonal antibodies typically serve as the primary biorecognition element and are immobilized on the electrode, while polyclonal antibodies serve as the secondary biorecognition element and often facilitate target labeling. For assays that do not require secondary binding steps, polyclonal antibodies are also commonly used as immobilized biorecognition elements for pathogen detection. For example, Pandey et al. immobilized monoclonal anti-E. coli on a composite nanostructured electrode to detect E. coli across a wide dynamic range of 10 to 108 CFU/mL with a LOD of 3.8 CFU/mL (Pandey et al. 2017). Wu et al. used polyclonal anti-E. coli for detection of E. coli via amperometry that exhibited a LOD of 5 × 103 CFU/mL (Wu et al. 2016). Lin et al. used monoclonal antibodies for detection of avian influenza virus H5N1 in chicken swabs across a dynamic range of 2- 1 to 24 hemagglutination units (HAU)/50 μL using EIS and the ferri/ferrocyanide (Fe(CN)6 3 - /4-) couple as a redox probe (Lin et al. 2015). Luka et al. detected Cryptosporidium parvum (C. parvum) with a LOD of 40 cells/mm2 via capacitive sensing and Fe(CN)6 3 - /4- (Luka et al. 2019).\nAntibody fragments, such as single-chain variable fragments (scFvs), offer selectivity similar to antibodies, but they have the advantage of achieving relatively higher packing densities on electrode surfaces due to their relatively smaller size. For example, half-antibody fragments have been shown to improve biosensor sensitivity without the loss of selectivity, which warrants further investigation of reduced antibodies as biorecognition elements for pathogen detection applications (Sharma and Mutharasan, 2013). In addition to scFvs, Fabs, re-engineered IgGs, and dimers can also potentially be used as biorecognition elements for pathogen detection (Byrne et al. 2009).\n\n2.2.2 Carbohydrate-binding proteins\nCarbohydrate-binding proteins, such as lectins, also provide selective biorecognition elements for pathogen detection based on their ability to selectively bind ligands on target species. Peptide-based biorecognition elements are relatively low-cost, can be produced with high yield automated synthesis processes, and are modifiable (Pavan and Berti, 2012). For example, lectins have been investigated as biorecognition elements for pathogen detection through their ability to selectively bind glycosylated proteins on the surfaces of viruses and cells (Reina et al. 2008). Concanavalin A (ConA) lectin has been extensively investigated for E. coli detection (see Table 1) (Jantra et al. 2011; Saucedo et al. 2019; Xi et al. 2011; Yang et al. 2016b). While not yet widely investigated for pathogen detection using electrochemical biosensors, Etayash et al. recently showed that oligopeptides also provide attractive biorecognition elements for real-time biosensor-based detection of breast cancer cells (Etayash et al. 2015).\n\n2.2.3 Oligosaccharides\nTrisaccharides are carbohydrates that can selectively bind carbohydrate-specific receptors on pathogens. Thus, trisaccharide ligands have been used as biorecognition elements for pathogen detection using electrochemical biosensors. For example, Hai et al. used a hybrid E-QCM biosensor coated with hemagglutinin-specific trisaccharide ligands for the detection of human influenza A virus (H1N1) (Hai et al. 2017). The use of carbohydrates as biorecognition elements is limited in part due to the weak affinity of carbohydrate-protein interactions and low selectivity, which are currently mitigated through secondary interactions (Zeng et al. 2012).\n\n2.2.4 Oligonucleotides\nSingle-stranded DNA (ssDNA) is a useful biorecognition element for the detection of pathogens. While ssDNA is commonly used as a biorecognition element for DNA-based assays, ssDNA aptamers are commonly used for pathogen detection using electrochemical biosensors. Aptamers are single-stranded oligonucleotides capable of binding various molecules with high affinity and selectivity (Lakhin et al. 2013; Reverdatto et al. 2015). Aptamers are isolated from a large random sequence pool through a selection process that utilizes systematic evolution of ligands by exponential enrichment, also known as SELEX (Stoltenburg et al. 2007). Suitable binding sequences can be isolated from a large random oligonucleotide sequence pool and subsequently amplified for use. Thus, aptamers can exhibit high selectivity to target species (Stoltenburg et al. 2007). Aptamers can also be produced at a lower cost than alternative biorecognition elements, such as antibodies. Giamberardino et al. used SELEX to discover an aptamer for norovirus detection, which showed a million-fold higher binding affinity for the target than a random DNA strand that served as a negative control (Giamberardino et al. 2013). Iqbal et al. performed 10 rounds of SELEX to discover 14 aptamer clones with high affinities for C. parvum for detection in fruit samples (Iqbal et al. 2015). However, the use of aptamers as biorecognition elements has not yet replaced traditional biorecognition elements, such as antibodies, because of several challenges, such as aptamer stability, degradation, cross-reactivity, and reproducibility using alternative processing approaches (Lakhin et al. 2013).\n\n2.2.5 Phages\nPhages, also referred to as bacteriophages, are viruses that infect and replicate in bacteria through selective binding via tail-spike proteins (Haq et al. 2012). Thus, they have been examined as biorecognition elements for pathogen detection using electrochemical biosensors (Kutter and Sulakvelidze, 2004). Bacteriophages exhibit varying morphologies and are thus classified by selectivity and structure. A variety of bacteriophage-based electrochemical biosensors for pathogen detection can be found in Table 1. For example, Shabani et al. used E. coli-specific T4 bacteriophages for selective impedimetric detection studies (Shabani et al. 2008). Mejri et al. compared the use of bacteriophages to antibodies as biorecognition elements for E. coli detection (Mejri et al. 2010). In that study, they found that bacteriophages improved the water stability of the biosensor and increased the sensitivity by approximately a factor of four relative to the response obtained with antibodies based on EIS measurements (Mejri et al. 2010). In another study, Tolba et al. utilized immobilized bacteriophage-encoded peptidoglycan hydrolases on Au screen-printed electrodes for detection of L. innocua in pure milk with a LOD of 105 CFU/mL (Tolba et al. 2012). These results suggest that bacteriophages are potentially attractive biorecognition elements for water safety and environmental monitoring applications that require chronic monitoring of liquids.\n\n2.2.6 Cell- and molecularly-imprinted polymers\nGiven traditional biorecognition elements used in biosensing exhibit stability concerns, such as antibodies or aptamers, as discussed in Sections 2.2.1–2.2.4, there have been efforts to create engineered molecular biorecognition elements, such as scFvs. In contrast, materials-based biorecognition elements exploit the principle of target-specific morphology for selective capture (Pan et al. 2018; Zhou et al. 2019). The most common approach in materials-based biorecognition is based on cell- and molecularly-imprinted polymers (CIPs and MIPs, respectively) (Gui et al. 2018). CIPs and MIPs have been created using various processes, including bacteria-mediated lithography, micro-contact stamping, and colloid imprints (Chen et al. 2016a; Pan et al. 2018).\nAs shown in Fig. 3b, Jafari et al. used imprinted organosilica sol-gel films of tetraethoxysilane and (3-mercaptopropyl)trimethoxysilane (MPTS) for selective detection of E. coli using an impedimetric method (Jafari et al. 2019). Similarly, Golabi et al. used imprinted poly(3-aminophenylboronic acid) films for detection of Staphylococcus epidermidis (S. epidermidis) (Golabi et al. 2017). Despite the absence of a highly selective molecular biorecognition element, CIPs and MIPs exhibit selectivity when exposed to samples that contain multiple analytes (i.e., non-target species) (Golabi et al. 2017; Jafari et al. 2019; Qi et al. 2013). MIPs and CIPs are also of interest with regard to opportunities in biosensor regeneration. Common adverse effects of regeneration on biosensors that employ molecular biorecognition elements, such as irreversible changes in structure, are less likely to affect MIPs and CIPs. However, it is generally accepted that current CIPs and MIPs exhibit lower selectivity to target species than antibodies and aptamers due to reduction of available chemical selectivity (Cheong et al. 2013; Kryscio and Peppas, 2012; Yáñez-Sedeño et al. 2017)."}
LitCovid-PD-UBERON
{"project":"LitCovid-PD-UBERON","denotations":[{"id":"T11","span":{"begin":3551,"end":3556},"obj":"Body_part"},{"id":"T12","span":{"begin":7047,"end":7053},"obj":"Body_part"},{"id":"T13","span":{"begin":9585,"end":9589},"obj":"Body_part"},{"id":"T14","span":{"begin":10664,"end":10668},"obj":"Body_part"}],"attributes":[{"id":"A11","pred":"uberon_id","subj":"T11","obj":"http://purl.obolibrary.org/obo/UBERON_0000178"},{"id":"A12","pred":"uberon_id","subj":"T12","obj":"http://purl.obolibrary.org/obo/UBERON_0000310"},{"id":"A13","pred":"uberon_id","subj":"T13","obj":"http://purl.obolibrary.org/obo/UBERON_0002415"},{"id":"A14","pred":"uberon_id","subj":"T14","obj":"http://purl.obolibrary.org/obo/UBERON_0001913"}],"text":"2.2 Biorecognition elements\nThe previous section discussed the transduction elements associated with pathogen detection using electrochemical biosensors. Given a biosensor is a device composed of integrated transducer and biorecognition elements, we next discuss the biorecognition elements used for selective detection of pathogens and corresponding immobilization techniques for their coupling to electrodes.\nBiorecognition elements for electrochemical biosensors can be defined as (1) biocatalytic or (2) biocomplexing. In the case of biocatalytic biorecognition elements, the biosensor response is based on a reaction catalyzed by macromolecules. Enzymes, whole cells, and tissues are the most commonly used biocatalytic biorecognition element. While enzyzmes provide biorecognition elements in various chemical sensing applications, they are often used as labels for pathogen detection applications and most commonly introduced via secondary binding steps. In the case of biocomplexing biorecognition elements, the biosensor response is based on the interaction of analytes with macromolecules or organized molecular assemblies. As shown in Table 1, Table 2, antibodies, peptides, and phages are the most commonly used biocomplexing biorecognition elements for pathogen detection. In addition to biomacromolecules, imprinted polymers have also been examined as biocomplexing biorecognition elements for pathogen detection using electrochemical biosensors.\n\n2.2.1 Antibodies and antibody fragments\nAntibodies and antibody fragments are among the most commonly utilized biorecognition elements for pathogen detection using electrochemical biosensors. Biosensors employing antibody-based biorecognition elements are commonly referred to as immunosensors. Given antibodies exhibit high selectivity and binding affinity for target species and can be generated for a wide range of infectious agents, antibodies are the gold-standard biorecognition element for pathogen detection. Antibodies contain recognition sites that selectively bind to antigens through a specific region of the antigen, referred to as an epitope (Patris et al. 2016). Antibodies can be labeled with fluorescent or enzymatic tags, which leads to the designation of the approach as label-based. While label-based approaches present measurement constraints associated with the use of additional reagents and processing steps (Cooper, 2009; Sang et al. 2016), antibody labeling may also alter the binding affinity to the antigen, which could affect the biosensor's selectivity. A detailed discussion of label-based biosensing approaches for pathogen detection has been reported elsewhere (Ahmed et al. 2014; Alahi and Mukhopadhyay, 2017; Bozal-Palabiyik et al. 2018; Leonard et al. 2003). A list of recent label-based approaches for pathogen detection using electrochemical biosensors, however, is provided in Table 2.\nWhile both monoclonal and polyclonal antibodies enable the selective detection of pathogens (Patris et al. 2016), they vary in terms of production method, selectivity, and binding affinity. Monoclonal antibodies are produced by hybridoma technology (Birch and Racher, 2006; James and Bell, 1987). Thus, monoclonal antibodies are highly selective and bind to a single epitope, making them less vulnerable to cross-reactivity. While monoclonal antibodies tend to have a higher degree of selectivity, they are more expensive and take longer to develop than polyclonal antibodies. Polyclonal antibodies are produced by separation of immunoglobulin proteins from the blood of an infected host (Birch and Racher, 2006). Polyclonal antibodies target different epitopes on a single antigen. While polyclonal antibodies exhibit increased variability between batches, they are relatively less expensive to produce than monoclonal antibodies and facilitate robust measurements in various settings (Byrne et al. 2009). Drawbacks to antibody use include high cost and stability challenges, such as the need for low-temperature storage. As shown in Table 1, Table 2, both monoclonal and polyclonal antibodies are used as biorecognition elements for pathogen detection. For assays involving secondary binding steps, monoclonal antibodies typically serve as the primary biorecognition element and are immobilized on the electrode, while polyclonal antibodies serve as the secondary biorecognition element and often facilitate target labeling. For assays that do not require secondary binding steps, polyclonal antibodies are also commonly used as immobilized biorecognition elements for pathogen detection. For example, Pandey et al. immobilized monoclonal anti-E. coli on a composite nanostructured electrode to detect E. coli across a wide dynamic range of 10 to 108 CFU/mL with a LOD of 3.8 CFU/mL (Pandey et al. 2017). Wu et al. used polyclonal anti-E. coli for detection of E. coli via amperometry that exhibited a LOD of 5 × 103 CFU/mL (Wu et al. 2016). Lin et al. used monoclonal antibodies for detection of avian influenza virus H5N1 in chicken swabs across a dynamic range of 2- 1 to 24 hemagglutination units (HAU)/50 μL using EIS and the ferri/ferrocyanide (Fe(CN)6 3 - /4-) couple as a redox probe (Lin et al. 2015). Luka et al. detected Cryptosporidium parvum (C. parvum) with a LOD of 40 cells/mm2 via capacitive sensing and Fe(CN)6 3 - /4- (Luka et al. 2019).\nAntibody fragments, such as single-chain variable fragments (scFvs), offer selectivity similar to antibodies, but they have the advantage of achieving relatively higher packing densities on electrode surfaces due to their relatively smaller size. For example, half-antibody fragments have been shown to improve biosensor sensitivity without the loss of selectivity, which warrants further investigation of reduced antibodies as biorecognition elements for pathogen detection applications (Sharma and Mutharasan, 2013). In addition to scFvs, Fabs, re-engineered IgGs, and dimers can also potentially be used as biorecognition elements for pathogen detection (Byrne et al. 2009).\n\n2.2.2 Carbohydrate-binding proteins\nCarbohydrate-binding proteins, such as lectins, also provide selective biorecognition elements for pathogen detection based on their ability to selectively bind ligands on target species. Peptide-based biorecognition elements are relatively low-cost, can be produced with high yield automated synthesis processes, and are modifiable (Pavan and Berti, 2012). For example, lectins have been investigated as biorecognition elements for pathogen detection through their ability to selectively bind glycosylated proteins on the surfaces of viruses and cells (Reina et al. 2008). Concanavalin A (ConA) lectin has been extensively investigated for E. coli detection (see Table 1) (Jantra et al. 2011; Saucedo et al. 2019; Xi et al. 2011; Yang et al. 2016b). While not yet widely investigated for pathogen detection using electrochemical biosensors, Etayash et al. recently showed that oligopeptides also provide attractive biorecognition elements for real-time biosensor-based detection of breast cancer cells (Etayash et al. 2015).\n\n2.2.3 Oligosaccharides\nTrisaccharides are carbohydrates that can selectively bind carbohydrate-specific receptors on pathogens. Thus, trisaccharide ligands have been used as biorecognition elements for pathogen detection using electrochemical biosensors. For example, Hai et al. used a hybrid E-QCM biosensor coated with hemagglutinin-specific trisaccharide ligands for the detection of human influenza A virus (H1N1) (Hai et al. 2017). The use of carbohydrates as biorecognition elements is limited in part due to the weak affinity of carbohydrate-protein interactions and low selectivity, which are currently mitigated through secondary interactions (Zeng et al. 2012).\n\n2.2.4 Oligonucleotides\nSingle-stranded DNA (ssDNA) is a useful biorecognition element for the detection of pathogens. While ssDNA is commonly used as a biorecognition element for DNA-based assays, ssDNA aptamers are commonly used for pathogen detection using electrochemical biosensors. Aptamers are single-stranded oligonucleotides capable of binding various molecules with high affinity and selectivity (Lakhin et al. 2013; Reverdatto et al. 2015). Aptamers are isolated from a large random sequence pool through a selection process that utilizes systematic evolution of ligands by exponential enrichment, also known as SELEX (Stoltenburg et al. 2007). Suitable binding sequences can be isolated from a large random oligonucleotide sequence pool and subsequently amplified for use. Thus, aptamers can exhibit high selectivity to target species (Stoltenburg et al. 2007). Aptamers can also be produced at a lower cost than alternative biorecognition elements, such as antibodies. Giamberardino et al. used SELEX to discover an aptamer for norovirus detection, which showed a million-fold higher binding affinity for the target than a random DNA strand that served as a negative control (Giamberardino et al. 2013). Iqbal et al. performed 10 rounds of SELEX to discover 14 aptamer clones with high affinities for C. parvum for detection in fruit samples (Iqbal et al. 2015). However, the use of aptamers as biorecognition elements has not yet replaced traditional biorecognition elements, such as antibodies, because of several challenges, such as aptamer stability, degradation, cross-reactivity, and reproducibility using alternative processing approaches (Lakhin et al. 2013).\n\n2.2.5 Phages\nPhages, also referred to as bacteriophages, are viruses that infect and replicate in bacteria through selective binding via tail-spike proteins (Haq et al. 2012). Thus, they have been examined as biorecognition elements for pathogen detection using electrochemical biosensors (Kutter and Sulakvelidze, 2004). Bacteriophages exhibit varying morphologies and are thus classified by selectivity and structure. A variety of bacteriophage-based electrochemical biosensors for pathogen detection can be found in Table 1. For example, Shabani et al. used E. coli-specific T4 bacteriophages for selective impedimetric detection studies (Shabani et al. 2008). Mejri et al. compared the use of bacteriophages to antibodies as biorecognition elements for E. coli detection (Mejri et al. 2010). In that study, they found that bacteriophages improved the water stability of the biosensor and increased the sensitivity by approximately a factor of four relative to the response obtained with antibodies based on EIS measurements (Mejri et al. 2010). In another study, Tolba et al. utilized immobilized bacteriophage-encoded peptidoglycan hydrolases on Au screen-printed electrodes for detection of L. innocua in pure milk with a LOD of 105 CFU/mL (Tolba et al. 2012). These results suggest that bacteriophages are potentially attractive biorecognition elements for water safety and environmental monitoring applications that require chronic monitoring of liquids.\n\n2.2.6 Cell- and molecularly-imprinted polymers\nGiven traditional biorecognition elements used in biosensing exhibit stability concerns, such as antibodies or aptamers, as discussed in Sections 2.2.1–2.2.4, there have been efforts to create engineered molecular biorecognition elements, such as scFvs. In contrast, materials-based biorecognition elements exploit the principle of target-specific morphology for selective capture (Pan et al. 2018; Zhou et al. 2019). The most common approach in materials-based biorecognition is based on cell- and molecularly-imprinted polymers (CIPs and MIPs, respectively) (Gui et al. 2018). CIPs and MIPs have been created using various processes, including bacteria-mediated lithography, micro-contact stamping, and colloid imprints (Chen et al. 2016a; Pan et al. 2018).\nAs shown in Fig. 3b, Jafari et al. used imprinted organosilica sol-gel films of tetraethoxysilane and (3-mercaptopropyl)trimethoxysilane (MPTS) for selective detection of E. coli using an impedimetric method (Jafari et al. 2019). Similarly, Golabi et al. used imprinted poly(3-aminophenylboronic acid) films for detection of Staphylococcus epidermidis (S. epidermidis) (Golabi et al. 2017). Despite the absence of a highly selective molecular biorecognition element, CIPs and MIPs exhibit selectivity when exposed to samples that contain multiple analytes (i.e., non-target species) (Golabi et al. 2017; Jafari et al. 2019; Qi et al. 2013). MIPs and CIPs are also of interest with regard to opportunities in biosensor regeneration. Common adverse effects of regeneration on biosensors that employ molecular biorecognition elements, such as irreversible changes in structure, are less likely to affect MIPs and CIPs. However, it is generally accepted that current CIPs and MIPs exhibit lower selectivity to target species than antibodies and aptamers due to reduction of available chemical selectivity (Cheong et al. 2013; Kryscio and Peppas, 2012; Yáñez-Sedeño et al. 2017)."}
LitCovid-PD-MONDO
{"project":"LitCovid-PD-MONDO","denotations":[{"id":"T63","span":{"begin":1882,"end":1892},"obj":"Disease"},{"id":"T64","span":{"begin":4988,"end":5003},"obj":"Disease"},{"id":"T65","span":{"begin":4994,"end":5003},"obj":"Disease"},{"id":"T66","span":{"begin":7047,"end":7060},"obj":"Disease"},{"id":"T67","span":{"begin":7054,"end":7060},"obj":"Disease"},{"id":"T68","span":{"begin":7485,"end":7494},"obj":"Disease"}],"attributes":[{"id":"A63","pred":"mondo_id","subj":"T63","obj":"http://purl.obolibrary.org/obo/MONDO_0005550"},{"id":"A64","pred":"mondo_id","subj":"T64","obj":"http://purl.obolibrary.org/obo/MONDO_0018695"},{"id":"A65","pred":"mondo_id","subj":"T65","obj":"http://purl.obolibrary.org/obo/MONDO_0005812"},{"id":"A66","pred":"mondo_id","subj":"T66","obj":"http://purl.obolibrary.org/obo/MONDO_0007254"},{"id":"A67","pred":"mondo_id","subj":"T67","obj":"http://purl.obolibrary.org/obo/MONDO_0004992"},{"id":"A68","pred":"mondo_id","subj":"T68","obj":"http://purl.obolibrary.org/obo/MONDO_0005812"}],"text":"2.2 Biorecognition elements\nThe previous section discussed the transduction elements associated with pathogen detection using electrochemical biosensors. Given a biosensor is a device composed of integrated transducer and biorecognition elements, we next discuss the biorecognition elements used for selective detection of pathogens and corresponding immobilization techniques for their coupling to electrodes.\nBiorecognition elements for electrochemical biosensors can be defined as (1) biocatalytic or (2) biocomplexing. In the case of biocatalytic biorecognition elements, the biosensor response is based on a reaction catalyzed by macromolecules. Enzymes, whole cells, and tissues are the most commonly used biocatalytic biorecognition element. While enzyzmes provide biorecognition elements in various chemical sensing applications, they are often used as labels for pathogen detection applications and most commonly introduced via secondary binding steps. In the case of biocomplexing biorecognition elements, the biosensor response is based on the interaction of analytes with macromolecules or organized molecular assemblies. As shown in Table 1, Table 2, antibodies, peptides, and phages are the most commonly used biocomplexing biorecognition elements for pathogen detection. In addition to biomacromolecules, imprinted polymers have also been examined as biocomplexing biorecognition elements for pathogen detection using electrochemical biosensors.\n\n2.2.1 Antibodies and antibody fragments\nAntibodies and antibody fragments are among the most commonly utilized biorecognition elements for pathogen detection using electrochemical biosensors. Biosensors employing antibody-based biorecognition elements are commonly referred to as immunosensors. Given antibodies exhibit high selectivity and binding affinity for target species and can be generated for a wide range of infectious agents, antibodies are the gold-standard biorecognition element for pathogen detection. Antibodies contain recognition sites that selectively bind to antigens through a specific region of the antigen, referred to as an epitope (Patris et al. 2016). Antibodies can be labeled with fluorescent or enzymatic tags, which leads to the designation of the approach as label-based. While label-based approaches present measurement constraints associated with the use of additional reagents and processing steps (Cooper, 2009; Sang et al. 2016), antibody labeling may also alter the binding affinity to the antigen, which could affect the biosensor's selectivity. A detailed discussion of label-based biosensing approaches for pathogen detection has been reported elsewhere (Ahmed et al. 2014; Alahi and Mukhopadhyay, 2017; Bozal-Palabiyik et al. 2018; Leonard et al. 2003). A list of recent label-based approaches for pathogen detection using electrochemical biosensors, however, is provided in Table 2.\nWhile both monoclonal and polyclonal antibodies enable the selective detection of pathogens (Patris et al. 2016), they vary in terms of production method, selectivity, and binding affinity. Monoclonal antibodies are produced by hybridoma technology (Birch and Racher, 2006; James and Bell, 1987). Thus, monoclonal antibodies are highly selective and bind to a single epitope, making them less vulnerable to cross-reactivity. While monoclonal antibodies tend to have a higher degree of selectivity, they are more expensive and take longer to develop than polyclonal antibodies. Polyclonal antibodies are produced by separation of immunoglobulin proteins from the blood of an infected host (Birch and Racher, 2006). Polyclonal antibodies target different epitopes on a single antigen. While polyclonal antibodies exhibit increased variability between batches, they are relatively less expensive to produce than monoclonal antibodies and facilitate robust measurements in various settings (Byrne et al. 2009). Drawbacks to antibody use include high cost and stability challenges, such as the need for low-temperature storage. As shown in Table 1, Table 2, both monoclonal and polyclonal antibodies are used as biorecognition elements for pathogen detection. For assays involving secondary binding steps, monoclonal antibodies typically serve as the primary biorecognition element and are immobilized on the electrode, while polyclonal antibodies serve as the secondary biorecognition element and often facilitate target labeling. For assays that do not require secondary binding steps, polyclonal antibodies are also commonly used as immobilized biorecognition elements for pathogen detection. For example, Pandey et al. immobilized monoclonal anti-E. coli on a composite nanostructured electrode to detect E. coli across a wide dynamic range of 10 to 108 CFU/mL with a LOD of 3.8 CFU/mL (Pandey et al. 2017). Wu et al. used polyclonal anti-E. coli for detection of E. coli via amperometry that exhibited a LOD of 5 × 103 CFU/mL (Wu et al. 2016). Lin et al. used monoclonal antibodies for detection of avian influenza virus H5N1 in chicken swabs across a dynamic range of 2- 1 to 24 hemagglutination units (HAU)/50 μL using EIS and the ferri/ferrocyanide (Fe(CN)6 3 - /4-) couple as a redox probe (Lin et al. 2015). Luka et al. detected Cryptosporidium parvum (C. parvum) with a LOD of 40 cells/mm2 via capacitive sensing and Fe(CN)6 3 - /4- (Luka et al. 2019).\nAntibody fragments, such as single-chain variable fragments (scFvs), offer selectivity similar to antibodies, but they have the advantage of achieving relatively higher packing densities on electrode surfaces due to their relatively smaller size. For example, half-antibody fragments have been shown to improve biosensor sensitivity without the loss of selectivity, which warrants further investigation of reduced antibodies as biorecognition elements for pathogen detection applications (Sharma and Mutharasan, 2013). In addition to scFvs, Fabs, re-engineered IgGs, and dimers can also potentially be used as biorecognition elements for pathogen detection (Byrne et al. 2009).\n\n2.2.2 Carbohydrate-binding proteins\nCarbohydrate-binding proteins, such as lectins, also provide selective biorecognition elements for pathogen detection based on their ability to selectively bind ligands on target species. Peptide-based biorecognition elements are relatively low-cost, can be produced with high yield automated synthesis processes, and are modifiable (Pavan and Berti, 2012). For example, lectins have been investigated as biorecognition elements for pathogen detection through their ability to selectively bind glycosylated proteins on the surfaces of viruses and cells (Reina et al. 2008). Concanavalin A (ConA) lectin has been extensively investigated for E. coli detection (see Table 1) (Jantra et al. 2011; Saucedo et al. 2019; Xi et al. 2011; Yang et al. 2016b). While not yet widely investigated for pathogen detection using electrochemical biosensors, Etayash et al. recently showed that oligopeptides also provide attractive biorecognition elements for real-time biosensor-based detection of breast cancer cells (Etayash et al. 2015).\n\n2.2.3 Oligosaccharides\nTrisaccharides are carbohydrates that can selectively bind carbohydrate-specific receptors on pathogens. Thus, trisaccharide ligands have been used as biorecognition elements for pathogen detection using electrochemical biosensors. For example, Hai et al. used a hybrid E-QCM biosensor coated with hemagglutinin-specific trisaccharide ligands for the detection of human influenza A virus (H1N1) (Hai et al. 2017). The use of carbohydrates as biorecognition elements is limited in part due to the weak affinity of carbohydrate-protein interactions and low selectivity, which are currently mitigated through secondary interactions (Zeng et al. 2012).\n\n2.2.4 Oligonucleotides\nSingle-stranded DNA (ssDNA) is a useful biorecognition element for the detection of pathogens. While ssDNA is commonly used as a biorecognition element for DNA-based assays, ssDNA aptamers are commonly used for pathogen detection using electrochemical biosensors. Aptamers are single-stranded oligonucleotides capable of binding various molecules with high affinity and selectivity (Lakhin et al. 2013; Reverdatto et al. 2015). Aptamers are isolated from a large random sequence pool through a selection process that utilizes systematic evolution of ligands by exponential enrichment, also known as SELEX (Stoltenburg et al. 2007). Suitable binding sequences can be isolated from a large random oligonucleotide sequence pool and subsequently amplified for use. Thus, aptamers can exhibit high selectivity to target species (Stoltenburg et al. 2007). Aptamers can also be produced at a lower cost than alternative biorecognition elements, such as antibodies. Giamberardino et al. used SELEX to discover an aptamer for norovirus detection, which showed a million-fold higher binding affinity for the target than a random DNA strand that served as a negative control (Giamberardino et al. 2013). Iqbal et al. performed 10 rounds of SELEX to discover 14 aptamer clones with high affinities for C. parvum for detection in fruit samples (Iqbal et al. 2015). However, the use of aptamers as biorecognition elements has not yet replaced traditional biorecognition elements, such as antibodies, because of several challenges, such as aptamer stability, degradation, cross-reactivity, and reproducibility using alternative processing approaches (Lakhin et al. 2013).\n\n2.2.5 Phages\nPhages, also referred to as bacteriophages, are viruses that infect and replicate in bacteria through selective binding via tail-spike proteins (Haq et al. 2012). Thus, they have been examined as biorecognition elements for pathogen detection using electrochemical biosensors (Kutter and Sulakvelidze, 2004). Bacteriophages exhibit varying morphologies and are thus classified by selectivity and structure. A variety of bacteriophage-based electrochemical biosensors for pathogen detection can be found in Table 1. For example, Shabani et al. used E. coli-specific T4 bacteriophages for selective impedimetric detection studies (Shabani et al. 2008). Mejri et al. compared the use of bacteriophages to antibodies as biorecognition elements for E. coli detection (Mejri et al. 2010). In that study, they found that bacteriophages improved the water stability of the biosensor and increased the sensitivity by approximately a factor of four relative to the response obtained with antibodies based on EIS measurements (Mejri et al. 2010). In another study, Tolba et al. utilized immobilized bacteriophage-encoded peptidoglycan hydrolases on Au screen-printed electrodes for detection of L. innocua in pure milk with a LOD of 105 CFU/mL (Tolba et al. 2012). These results suggest that bacteriophages are potentially attractive biorecognition elements for water safety and environmental monitoring applications that require chronic monitoring of liquids.\n\n2.2.6 Cell- and molecularly-imprinted polymers\nGiven traditional biorecognition elements used in biosensing exhibit stability concerns, such as antibodies or aptamers, as discussed in Sections 2.2.1–2.2.4, there have been efforts to create engineered molecular biorecognition elements, such as scFvs. In contrast, materials-based biorecognition elements exploit the principle of target-specific morphology for selective capture (Pan et al. 2018; Zhou et al. 2019). The most common approach in materials-based biorecognition is based on cell- and molecularly-imprinted polymers (CIPs and MIPs, respectively) (Gui et al. 2018). CIPs and MIPs have been created using various processes, including bacteria-mediated lithography, micro-contact stamping, and colloid imprints (Chen et al. 2016a; Pan et al. 2018).\nAs shown in Fig. 3b, Jafari et al. used imprinted organosilica sol-gel films of tetraethoxysilane and (3-mercaptopropyl)trimethoxysilane (MPTS) for selective detection of E. coli using an impedimetric method (Jafari et al. 2019). Similarly, Golabi et al. used imprinted poly(3-aminophenylboronic acid) films for detection of Staphylococcus epidermidis (S. epidermidis) (Golabi et al. 2017). Despite the absence of a highly selective molecular biorecognition element, CIPs and MIPs exhibit selectivity when exposed to samples that contain multiple analytes (i.e., non-target species) (Golabi et al. 2017; Jafari et al. 2019; Qi et al. 2013). MIPs and CIPs are also of interest with regard to opportunities in biosensor regeneration. Common adverse effects of regeneration on biosensors that employ molecular biorecognition elements, such as irreversible changes in structure, are less likely to affect MIPs and CIPs. However, it is generally accepted that current CIPs and MIPs exhibit lower selectivity to target species than antibodies and aptamers due to reduction of available chemical selectivity (Cheong et al. 2013; Kryscio and Peppas, 2012; Yáñez-Sedeño et al. 2017)."}
LitCovid-PD-CLO
{"project":"LitCovid-PD-CLO","denotations":[{"id":"T413","span":{"begin":161,"end":162},"obj":"http://purl.obolibrary.org/obo/CLO_0001020"},{"id":"T414","span":{"begin":176,"end":177},"obj":"http://purl.obolibrary.org/obo/CLO_0001020"},{"id":"T415","span":{"begin":178,"end":184},"obj":"http://purl.obolibrary.org/obo/OBI_0000968"},{"id":"T416","span":{"begin":612,"end":613},"obj":"http://purl.obolibrary.org/obo/CLO_0001020"},{"id":"T417","span":{"begin":667,"end":672},"obj":"http://purl.obolibrary.org/obo/GO_0005623"},{"id":"T418","span":{"begin":862,"end":868},"obj":"http://purl.obolibrary.org/obo/CLO_0007225"},{"id":"T419","span":{"begin":1103,"end":1112},"obj":"http://purl.obolibrary.org/obo/OBI_0000245"},{"id":"T420","span":{"begin":1177,"end":1185},"obj":"http://purl.obolibrary.org/obo/PR_000018263"},{"id":"T421","span":{"begin":1866,"end":1867},"obj":"http://purl.obolibrary.org/obo/CLO_0001020"},{"id":"T422","span":{"begin":2060,"end":2061},"obj":"http://purl.obolibrary.org/obo/CLO_0001020"},{"id":"T423","span":{"begin":2160,"end":2167},"obj":"http://purl.obolibrary.org/obo/CLO_0007225"},{"id":"T424","span":{"begin":2254,"end":2259},"obj":"http://purl.obolibrary.org/obo/CLO_0007225"},{"id":"T425","span":{"begin":2273,"end":2278},"obj":"http://purl.obolibrary.org/obo/CLO_0007225"},{"id":"T426","span":{"begin":2439,"end":2447},"obj":"http://purl.obolibrary.org/obo/CLO_0007225"},{"id":"T427","span":{"begin":2548,"end":2549},"obj":"http://purl.obolibrary.org/obo/CLO_0001020"},{"id":"T428","span":{"begin":2573,"end":2578},"obj":"http://purl.obolibrary.org/obo/CLO_0007225"},{"id":"T429","span":{"begin":2630,"end":2633},"obj":"http://purl.obolibrary.org/obo/CLO_0051582"},{"id":"T430","span":{"begin":2731,"end":2735},"obj":"http://purl.obolibrary.org/obo/CLO_0001185"},{"id":"T431","span":{"begin":2759,"end":2760},"obj":"http://purl.obolibrary.org/obo/CLO_0001020"},{"id":"T432","span":{"begin":2776,"end":2781},"obj":"http://purl.obolibrary.org/obo/CLO_0007225"},{"id":"T433","span":{"begin":3117,"end":3126},"obj":"http://purl.obolibrary.org/obo/CLO_0036932"},{"id":"T434","span":{"begin":3247,"end":3248},"obj":"http://purl.obolibrary.org/obo/CLO_0001020"},{"id":"T435","span":{"begin":3355,"end":3356},"obj":"http://purl.obolibrary.org/obo/CLO_0001020"},{"id":"T436","span":{"begin":3551,"end":3556},"obj":"http://purl.obolibrary.org/obo/UBERON_0000178"},{"id":"T437","span":{"begin":3551,"end":3556},"obj":"http://www.ebi.ac.uk/efo/EFO_0000296"},{"id":"T438","span":{"begin":3654,"end":3655},"obj":"http://purl.obolibrary.org/obo/CLO_0001020"},{"id":"T439","span":{"begin":4406,"end":4414},"obj":"http://purl.obolibrary.org/obo/CLO_0007225"},{"id":"T440","span":{"begin":4646,"end":4647},"obj":"http://purl.obolibrary.org/obo/CLO_0001020"},{"id":"T441","span":{"begin":4708,"end":4709},"obj":"http://purl.obolibrary.org/obo/CLO_0001020"},{"id":"T442","span":{"begin":4754,"end":4755},"obj":"http://purl.obolibrary.org/obo/CLO_0001020"},{"id":"T443","span":{"begin":4891,"end":4892},"obj":"http://purl.obolibrary.org/obo/CLO_0001020"},{"id":"T444","span":{"begin":5004,"end":5009},"obj":"http://purl.obolibrary.org/obo/NCBITaxon_10239"},{"id":"T445","span":{"begin":5018,"end":5025},"obj":"http://purl.obolibrary.org/obo/NCBITaxon_9031"},{"id":"T446","span":{"begin":5039,"end":5040},"obj":"http://purl.obolibrary.org/obo/CLO_0001020"},{"id":"T447","span":{"begin":5150,"end":5156},"obj":"http://purl.obolibrary.org/obo/CLO_0001302"},{"id":"T448","span":{"begin":5169,"end":5170},"obj":"http://purl.obolibrary.org/obo/CLO_0001020"},{"id":"T449","span":{"begin":5263,"end":5264},"obj":"http://purl.obolibrary.org/obo/CLO_0001020"},{"id":"T450","span":{"begin":5275,"end":5280},"obj":"http://purl.obolibrary.org/obo/GO_0005623"},{"id":"T451","span":{"begin":5320,"end":5326},"obj":"http://purl.obolibrary.org/obo/CLO_0001302"},{"id":"T452","span":{"begin":6252,"end":6259},"obj":"http://purl.obolibrary.org/obo/PR_000018263"},{"id":"T453","span":{"begin":6599,"end":6606},"obj":"http://purl.obolibrary.org/obo/NCBITaxon_10239"},{"id":"T454","span":{"begin":6611,"end":6616},"obj":"http://purl.obolibrary.org/obo/GO_0005623"},{"id":"T455","span":{"begin":6651,"end":6652},"obj":"http://purl.obolibrary.org/obo/CLO_0001020"},{"id":"T456","span":{"begin":6667,"end":6670},"obj":"http://purl.obolibrary.org/obo/CLO_0051582"},{"id":"T457","span":{"begin":7047,"end":7053},"obj":"http://purl.obolibrary.org/obo/UBERON_0000310"},{"id":"T458","span":{"begin":7061,"end":7066},"obj":"http://purl.obolibrary.org/obo/GO_0005623"},{"id":"T459","span":{"begin":7376,"end":7377},"obj":"http://purl.obolibrary.org/obo/CLO_0001020"},{"id":"T460","span":{"begin":7479,"end":7484},"obj":"http://purl.obolibrary.org/obo/NCBITaxon_9606"},{"id":"T461","span":{"begin":7495,"end":7496},"obj":"http://purl.obolibrary.org/obo/CLO_0001020"},{"id":"T462","span":{"begin":7497,"end":7502},"obj":"http://purl.obolibrary.org/obo/NCBITaxon_10239"},{"id":"T463","span":{"begin":7820,"end":7821},"obj":"http://purl.obolibrary.org/obo/CLO_0001020"},{"id":"T464","span":{"begin":7916,"end":7917},"obj":"http://purl.obolibrary.org/obo/CLO_0001020"},{"id":"T465","span":{"begin":8244,"end":8245},"obj":"http://purl.obolibrary.org/obo/CLO_0001020"},{"id":"T466","span":{"begin":8281,"end":8282},"obj":"http://purl.obolibrary.org/obo/CLO_0001020"},{"id":"T467","span":{"begin":8469,"end":8470},"obj":"http://purl.obolibrary.org/obo/CLO_0001020"},{"id":"T468","span":{"begin":8672,"end":8673},"obj":"http://purl.obolibrary.org/obo/CLO_0001020"},{"id":"T469","span":{"begin":8840,"end":8841},"obj":"http://purl.obolibrary.org/obo/CLO_0001020"},{"id":"T470","span":{"begin":8899,"end":8900},"obj":"http://purl.obolibrary.org/obo/CLO_0001020"},{"id":"T471","span":{"begin":8934,"end":8935},"obj":"http://purl.obolibrary.org/obo/CLO_0001020"},{"id":"T472","span":{"begin":9197,"end":9200},"obj":"http://purl.obolibrary.org/obo/CLO_0051582"},{"id":"T473","span":{"begin":9509,"end":9516},"obj":"http://purl.obolibrary.org/obo/NCBITaxon_10239"},{"id":"T474","span":{"begin":9546,"end":9554},"obj":"http://purl.obolibrary.org/obo/NCBITaxon_2"},{"id":"T475","span":{"begin":9585,"end":9589},"obj":"http://purl.obolibrary.org/obo/UBERON_0002415"},{"id":"T476","span":{"begin":9868,"end":9869},"obj":"http://purl.obolibrary.org/obo/CLO_0001020"},{"id":"T477","span":{"begin":10383,"end":10384},"obj":"http://purl.obolibrary.org/obo/CLO_0001020"},{"id":"T478","span":{"begin":10674,"end":10675},"obj":"http://purl.obolibrary.org/obo/CLO_0001020"},{"id":"T479","span":{"begin":10919,"end":10923},"obj":"http://purl.obolibrary.org/obo/GO_0005623"},{"id":"T480","span":{"begin":11342,"end":11345},"obj":"http://purl.obolibrary.org/obo/NCBITaxon_9596"},{"id":"T481","span":{"begin":11353,"end":11357},"obj":"http://purl.obolibrary.org/obo/CLO_0001185"},{"id":"T482","span":{"begin":11449,"end":11453},"obj":"http://purl.obolibrary.org/obo/GO_0005623"},{"id":"T483","span":{"begin":11532,"end":11536},"obj":"http://purl.obolibrary.org/obo/CLO_0001185"},{"id":"T484","span":{"begin":11606,"end":11614},"obj":"http://purl.obolibrary.org/obo/NCBITaxon_2"},{"id":"T485","span":{"begin":11702,"end":11705},"obj":"http://purl.obolibrary.org/obo/NCBITaxon_9596"},{"id":"T486","span":{"begin":11713,"end":11717},"obj":"http://purl.obolibrary.org/obo/CLO_0001185"},{"id":"T487","span":{"begin":12134,"end":12135},"obj":"http://purl.obolibrary.org/obo/CLO_0001020"}],"text":"2.2 Biorecognition elements\nThe previous section discussed the transduction elements associated with pathogen detection using electrochemical biosensors. Given a biosensor is a device composed of integrated transducer and biorecognition elements, we next discuss the biorecognition elements used for selective detection of pathogens and corresponding immobilization techniques for their coupling to electrodes.\nBiorecognition elements for electrochemical biosensors can be defined as (1) biocatalytic or (2) biocomplexing. In the case of biocatalytic biorecognition elements, the biosensor response is based on a reaction catalyzed by macromolecules. Enzymes, whole cells, and tissues are the most commonly used biocatalytic biorecognition element. While enzyzmes provide biorecognition elements in various chemical sensing applications, they are often used as labels for pathogen detection applications and most commonly introduced via secondary binding steps. In the case of biocomplexing biorecognition elements, the biosensor response is based on the interaction of analytes with macromolecules or organized molecular assemblies. As shown in Table 1, Table 2, antibodies, peptides, and phages are the most commonly used biocomplexing biorecognition elements for pathogen detection. In addition to biomacromolecules, imprinted polymers have also been examined as biocomplexing biorecognition elements for pathogen detection using electrochemical biosensors.\n\n2.2.1 Antibodies and antibody fragments\nAntibodies and antibody fragments are among the most commonly utilized biorecognition elements for pathogen detection using electrochemical biosensors. Biosensors employing antibody-based biorecognition elements are commonly referred to as immunosensors. Given antibodies exhibit high selectivity and binding affinity for target species and can be generated for a wide range of infectious agents, antibodies are the gold-standard biorecognition element for pathogen detection. Antibodies contain recognition sites that selectively bind to antigens through a specific region of the antigen, referred to as an epitope (Patris et al. 2016). Antibodies can be labeled with fluorescent or enzymatic tags, which leads to the designation of the approach as label-based. While label-based approaches present measurement constraints associated with the use of additional reagents and processing steps (Cooper, 2009; Sang et al. 2016), antibody labeling may also alter the binding affinity to the antigen, which could affect the biosensor's selectivity. A detailed discussion of label-based biosensing approaches for pathogen detection has been reported elsewhere (Ahmed et al. 2014; Alahi and Mukhopadhyay, 2017; Bozal-Palabiyik et al. 2018; Leonard et al. 2003). A list of recent label-based approaches for pathogen detection using electrochemical biosensors, however, is provided in Table 2.\nWhile both monoclonal and polyclonal antibodies enable the selective detection of pathogens (Patris et al. 2016), they vary in terms of production method, selectivity, and binding affinity. Monoclonal antibodies are produced by hybridoma technology (Birch and Racher, 2006; James and Bell, 1987). Thus, monoclonal antibodies are highly selective and bind to a single epitope, making them less vulnerable to cross-reactivity. While monoclonal antibodies tend to have a higher degree of selectivity, they are more expensive and take longer to develop than polyclonal antibodies. Polyclonal antibodies are produced by separation of immunoglobulin proteins from the blood of an infected host (Birch and Racher, 2006). Polyclonal antibodies target different epitopes on a single antigen. While polyclonal antibodies exhibit increased variability between batches, they are relatively less expensive to produce than monoclonal antibodies and facilitate robust measurements in various settings (Byrne et al. 2009). Drawbacks to antibody use include high cost and stability challenges, such as the need for low-temperature storage. As shown in Table 1, Table 2, both monoclonal and polyclonal antibodies are used as biorecognition elements for pathogen detection. For assays involving secondary binding steps, monoclonal antibodies typically serve as the primary biorecognition element and are immobilized on the electrode, while polyclonal antibodies serve as the secondary biorecognition element and often facilitate target labeling. For assays that do not require secondary binding steps, polyclonal antibodies are also commonly used as immobilized biorecognition elements for pathogen detection. For example, Pandey et al. immobilized monoclonal anti-E. coli on a composite nanostructured electrode to detect E. coli across a wide dynamic range of 10 to 108 CFU/mL with a LOD of 3.8 CFU/mL (Pandey et al. 2017). Wu et al. used polyclonal anti-E. coli for detection of E. coli via amperometry that exhibited a LOD of 5 × 103 CFU/mL (Wu et al. 2016). Lin et al. used monoclonal antibodies for detection of avian influenza virus H5N1 in chicken swabs across a dynamic range of 2- 1 to 24 hemagglutination units (HAU)/50 μL using EIS and the ferri/ferrocyanide (Fe(CN)6 3 - /4-) couple as a redox probe (Lin et al. 2015). Luka et al. detected Cryptosporidium parvum (C. parvum) with a LOD of 40 cells/mm2 via capacitive sensing and Fe(CN)6 3 - /4- (Luka et al. 2019).\nAntibody fragments, such as single-chain variable fragments (scFvs), offer selectivity similar to antibodies, but they have the advantage of achieving relatively higher packing densities on electrode surfaces due to their relatively smaller size. For example, half-antibody fragments have been shown to improve biosensor sensitivity without the loss of selectivity, which warrants further investigation of reduced antibodies as biorecognition elements for pathogen detection applications (Sharma and Mutharasan, 2013). In addition to scFvs, Fabs, re-engineered IgGs, and dimers can also potentially be used as biorecognition elements for pathogen detection (Byrne et al. 2009).\n\n2.2.2 Carbohydrate-binding proteins\nCarbohydrate-binding proteins, such as lectins, also provide selective biorecognition elements for pathogen detection based on their ability to selectively bind ligands on target species. Peptide-based biorecognition elements are relatively low-cost, can be produced with high yield automated synthesis processes, and are modifiable (Pavan and Berti, 2012). For example, lectins have been investigated as biorecognition elements for pathogen detection through their ability to selectively bind glycosylated proteins on the surfaces of viruses and cells (Reina et al. 2008). Concanavalin A (ConA) lectin has been extensively investigated for E. coli detection (see Table 1) (Jantra et al. 2011; Saucedo et al. 2019; Xi et al. 2011; Yang et al. 2016b). While not yet widely investigated for pathogen detection using electrochemical biosensors, Etayash et al. recently showed that oligopeptides also provide attractive biorecognition elements for real-time biosensor-based detection of breast cancer cells (Etayash et al. 2015).\n\n2.2.3 Oligosaccharides\nTrisaccharides are carbohydrates that can selectively bind carbohydrate-specific receptors on pathogens. Thus, trisaccharide ligands have been used as biorecognition elements for pathogen detection using electrochemical biosensors. For example, Hai et al. used a hybrid E-QCM biosensor coated with hemagglutinin-specific trisaccharide ligands for the detection of human influenza A virus (H1N1) (Hai et al. 2017). The use of carbohydrates as biorecognition elements is limited in part due to the weak affinity of carbohydrate-protein interactions and low selectivity, which are currently mitigated through secondary interactions (Zeng et al. 2012).\n\n2.2.4 Oligonucleotides\nSingle-stranded DNA (ssDNA) is a useful biorecognition element for the detection of pathogens. While ssDNA is commonly used as a biorecognition element for DNA-based assays, ssDNA aptamers are commonly used for pathogen detection using electrochemical biosensors. Aptamers are single-stranded oligonucleotides capable of binding various molecules with high affinity and selectivity (Lakhin et al. 2013; Reverdatto et al. 2015). Aptamers are isolated from a large random sequence pool through a selection process that utilizes systematic evolution of ligands by exponential enrichment, also known as SELEX (Stoltenburg et al. 2007). Suitable binding sequences can be isolated from a large random oligonucleotide sequence pool and subsequently amplified for use. Thus, aptamers can exhibit high selectivity to target species (Stoltenburg et al. 2007). Aptamers can also be produced at a lower cost than alternative biorecognition elements, such as antibodies. Giamberardino et al. used SELEX to discover an aptamer for norovirus detection, which showed a million-fold higher binding affinity for the target than a random DNA strand that served as a negative control (Giamberardino et al. 2013). Iqbal et al. performed 10 rounds of SELEX to discover 14 aptamer clones with high affinities for C. parvum for detection in fruit samples (Iqbal et al. 2015). However, the use of aptamers as biorecognition elements has not yet replaced traditional biorecognition elements, such as antibodies, because of several challenges, such as aptamer stability, degradation, cross-reactivity, and reproducibility using alternative processing approaches (Lakhin et al. 2013).\n\n2.2.5 Phages\nPhages, also referred to as bacteriophages, are viruses that infect and replicate in bacteria through selective binding via tail-spike proteins (Haq et al. 2012). Thus, they have been examined as biorecognition elements for pathogen detection using electrochemical biosensors (Kutter and Sulakvelidze, 2004). Bacteriophages exhibit varying morphologies and are thus classified by selectivity and structure. A variety of bacteriophage-based electrochemical biosensors for pathogen detection can be found in Table 1. For example, Shabani et al. used E. coli-specific T4 bacteriophages for selective impedimetric detection studies (Shabani et al. 2008). Mejri et al. compared the use of bacteriophages to antibodies as biorecognition elements for E. coli detection (Mejri et al. 2010). In that study, they found that bacteriophages improved the water stability of the biosensor and increased the sensitivity by approximately a factor of four relative to the response obtained with antibodies based on EIS measurements (Mejri et al. 2010). In another study, Tolba et al. utilized immobilized bacteriophage-encoded peptidoglycan hydrolases on Au screen-printed electrodes for detection of L. innocua in pure milk with a LOD of 105 CFU/mL (Tolba et al. 2012). These results suggest that bacteriophages are potentially attractive biorecognition elements for water safety and environmental monitoring applications that require chronic monitoring of liquids.\n\n2.2.6 Cell- and molecularly-imprinted polymers\nGiven traditional biorecognition elements used in biosensing exhibit stability concerns, such as antibodies or aptamers, as discussed in Sections 2.2.1–2.2.4, there have been efforts to create engineered molecular biorecognition elements, such as scFvs. In contrast, materials-based biorecognition elements exploit the principle of target-specific morphology for selective capture (Pan et al. 2018; Zhou et al. 2019). The most common approach in materials-based biorecognition is based on cell- and molecularly-imprinted polymers (CIPs and MIPs, respectively) (Gui et al. 2018). CIPs and MIPs have been created using various processes, including bacteria-mediated lithography, micro-contact stamping, and colloid imprints (Chen et al. 2016a; Pan et al. 2018).\nAs shown in Fig. 3b, Jafari et al. used imprinted organosilica sol-gel films of tetraethoxysilane and (3-mercaptopropyl)trimethoxysilane (MPTS) for selective detection of E. coli using an impedimetric method (Jafari et al. 2019). Similarly, Golabi et al. used imprinted poly(3-aminophenylboronic acid) films for detection of Staphylococcus epidermidis (S. epidermidis) (Golabi et al. 2017). Despite the absence of a highly selective molecular biorecognition element, CIPs and MIPs exhibit selectivity when exposed to samples that contain multiple analytes (i.e., non-target species) (Golabi et al. 2017; Jafari et al. 2019; Qi et al. 2013). MIPs and CIPs are also of interest with regard to opportunities in biosensor regeneration. Common adverse effects of regeneration on biosensors that employ molecular biorecognition elements, such as irreversible changes in structure, are less likely to affect MIPs and CIPs. However, it is generally accepted that current CIPs and MIPs exhibit lower selectivity to target species than antibodies and aptamers due to reduction of available chemical selectivity (Cheong et al. 2013; Kryscio and Peppas, 2012; Yáñez-Sedeño et al. 2017)."}
LitCovid-PD-CHEBI
{"project":"LitCovid-PD-CHEBI","denotations":[{"id":"T8714","span":{"begin":636,"end":650},"obj":"Chemical"},{"id":"T82184","span":{"begin":1085,"end":1099},"obj":"Chemical"},{"id":"T43","span":{"begin":1177,"end":1185},"obj":"Chemical"},{"id":"T43840","span":{"begin":1302,"end":1319},"obj":"Chemical"},{"id":"T45","span":{"begin":1331,"end":1339},"obj":"Chemical"},{"id":"T62334","span":{"begin":1920,"end":1924},"obj":"Chemical"},{"id":"T48","span":{"begin":2043,"end":2051},"obj":"Chemical"},{"id":"T62779","span":{"begin":2085,"end":2092},"obj":"Chemical"},{"id":"T2780","span":{"begin":2112,"end":2119},"obj":"Chemical"},{"id":"T5133","span":{"begin":2254,"end":2259},"obj":"Chemical"},{"id":"T37986","span":{"begin":2273,"end":2278},"obj":"Chemical"},{"id":"T98324","span":{"begin":2366,"end":2374},"obj":"Chemical"},{"id":"T33842","span":{"begin":2491,"end":2498},"obj":"Chemical"},{"id":"T16181","span":{"begin":2573,"end":2578},"obj":"Chemical"},{"id":"T21390","span":{"begin":2776,"end":2781},"obj":"Chemical"},{"id":"T9692","span":{"begin":3256,"end":3263},"obj":"Chemical"},{"id":"T58","span":{"begin":3533,"end":3541},"obj":"Chemical"},{"id":"T18587","span":{"begin":3663,"end":3670},"obj":"Chemical"},{"id":"T60","span":{"begin":4933,"end":4936},"obj":"Chemical"},{"id":"T82620","span":{"begin":5110,"end":5113},"obj":"Chemical"},{"id":"T96787","span":{"begin":5128,"end":5140},"obj":"Chemical"},{"id":"T63","span":{"begin":5142,"end":5144},"obj":"Chemical"},{"id":"T50019","span":{"begin":5177,"end":5182},"obj":"Chemical"},{"id":"T26443","span":{"begin":5184,"end":5187},"obj":"Chemical"},{"id":"T65271","span":{"begin":5312,"end":5314},"obj":"Chemical"},{"id":"T22889","span":{"begin":6055,"end":6063},"obj":"Chemical"},{"id":"T68","span":{"begin":6085,"end":6093},"obj":"Chemical"},{"id":"T69","span":{"begin":6225,"end":6232},"obj":"Chemical"},{"id":"T84319","span":{"begin":6252,"end":6259},"obj":"Chemical"},{"id":"T31243","span":{"begin":6571,"end":6579},"obj":"Chemical"},{"id":"T64538","span":{"begin":6942,"end":6955},"obj":"Chemical"},{"id":"T30093","span":{"begin":7134,"end":7147},"obj":"Chemical"},{"id":"T34862","span":{"begin":7174,"end":7186},"obj":"Chemical"},{"id":"T13773","span":{"begin":7226,"end":7239},"obj":"Chemical"},{"id":"T52864","span":{"begin":7240,"end":7247},"obj":"Chemical"},{"id":"T44200","span":{"begin":7436,"end":7449},"obj":"Chemical"},{"id":"T70626","span":{"begin":7450,"end":7457},"obj":"Chemical"},{"id":"T79","span":{"begin":7540,"end":7553},"obj":"Chemical"},{"id":"T58945","span":{"begin":7628,"end":7640},"obj":"Chemical"},{"id":"T81","span":{"begin":7641,"end":7648},"obj":"Chemical"},{"id":"T83556","span":{"begin":7789,"end":7808},"obj":"Chemical"},{"id":"T94086","span":{"begin":7805,"end":7808},"obj":"Chemical"},{"id":"T14585","span":{"begin":7810,"end":7815},"obj":"Chemical"},{"id":"T20829","span":{"begin":7890,"end":7895},"obj":"Chemical"},{"id":"T89112","span":{"begin":7945,"end":7948},"obj":"Chemical"},{"id":"T93354","span":{"begin":7963,"end":7968},"obj":"Chemical"},{"id":"T35047","span":{"begin":7969,"end":7977},"obj":"Chemical"},{"id":"T63521","span":{"begin":8082,"end":8098},"obj":"Chemical"},{"id":"T41981","span":{"begin":8126,"end":8135},"obj":"Chemical"},{"id":"T62122","span":{"begin":8339,"end":8346},"obj":"Chemical"},{"id":"T9235","span":{"begin":8484,"end":8499},"obj":"Chemical"},{"id":"T54261","span":{"begin":8556,"end":8564},"obj":"Chemical"},{"id":"T49855","span":{"begin":8794,"end":8801},"obj":"Chemical"},{"id":"T32746","span":{"begin":8908,"end":8911},"obj":"Chemical"},{"id":"T99337","span":{"begin":9039,"end":9046},"obj":"Chemical"},{"id":"T97","span":{"begin":9161,"end":9169},"obj":"Chemical"},{"id":"T86290","span":{"begin":9314,"end":9321},"obj":"Chemical"},{"id":"T44669","span":{"begin":9596,"end":9604},"obj":"Chemical"},{"id":"T56913","span":{"begin":10026,"end":10028},"obj":"Chemical"},{"id":"T5510","span":{"begin":10303,"end":10308},"obj":"Chemical"},{"id":"T53479","span":{"begin":10459,"end":10462},"obj":"Chemical"},{"id":"T8615","span":{"begin":10571,"end":10584},"obj":"Chemical"},{"id":"T105","span":{"begin":10599,"end":10601},"obj":"Chemical"},{"id":"T43880","span":{"begin":10812,"end":10817},"obj":"Chemical"},{"id":"T66902","span":{"begin":10951,"end":10959},"obj":"Chemical"},{"id":"T37286","span":{"begin":11071,"end":11079},"obj":"Chemical"},{"id":"T54658","span":{"begin":11481,"end":11489},"obj":"Chemical"},{"id":"T67116","span":{"begin":12016,"end":12020},"obj":"Chemical"},{"id":"T50783","span":{"begin":12761,"end":12769},"obj":"Chemical"}],"attributes":[{"id":"A46631","pred":"chebi_id","subj":"T8714","obj":"http://purl.obolibrary.org/obo/CHEBI_33839"},{"id":"A4538","pred":"chebi_id","subj":"T82184","obj":"http://purl.obolibrary.org/obo/CHEBI_33839"},{"id":"A42643","pred":"chebi_id","subj":"T43","obj":"http://purl.obolibrary.org/obo/CHEBI_16670"},{"id":"A55385","pred":"chebi_id","subj":"T43840","obj":"http://purl.obolibrary.org/obo/CHEBI_33694"},{"id":"A47118","pred":"chebi_id","subj":"T45","obj":"http://purl.obolibrary.org/obo/CHEBI_33839"},{"id":"A18189","pred":"chebi_id","subj":"T62334","obj":"http://purl.obolibrary.org/obo/CHEBI_29287"},{"id":"A34053","pred":"chebi_id","subj":"T62334","obj":"http://purl.obolibrary.org/obo/CHEBI_30050"},{"id":"A30553","pred":"chebi_id","subj":"T48","obj":"http://purl.obolibrary.org/obo/CHEBI_59132"},{"id":"A80514","pred":"chebi_id","subj":"T62779","obj":"http://purl.obolibrary.org/obo/CHEBI_59132"},{"id":"A36720","pred":"chebi_id","subj":"T2780","obj":"http://purl.obolibrary.org/obo/CHEBI_53000"},{"id":"A41096","pred":"chebi_id","subj":"T5133","obj":"http://purl.obolibrary.org/obo/CHEBI_35209"},{"id":"A27536","pred":"chebi_id","subj":"T37986","obj":"http://purl.obolibrary.org/obo/CHEBI_35209"},{"id":"A67659","pred":"chebi_id","subj":"T98324","obj":"http://purl.obolibrary.org/obo/CHEBI_33893"},{"id":"A5523","pred":"chebi_id","subj":"T33842","obj":"http://purl.obolibrary.org/obo/CHEBI_59132"},{"id":"A96276","pred":"chebi_id","subj":"T16181","obj":"http://purl.obolibrary.org/obo/CHEBI_35209"},{"id":"A39464","pred":"chebi_id","subj":"T21390","obj":"http://purl.obolibrary.org/obo/CHEBI_35209"},{"id":"A37416","pred":"chebi_id","subj":"T9692","obj":"http://purl.obolibrary.org/obo/CHEBI_53000"},{"id":"A53087","pred":"chebi_id","subj":"T58","obj":"http://purl.obolibrary.org/obo/CHEBI_36080"},{"id":"A74125","pred":"chebi_id","subj":"T18587","obj":"http://purl.obolibrary.org/obo/CHEBI_59132"},{"id":"A92226","pred":"chebi_id","subj":"T60","obj":"http://purl.obolibrary.org/obo/CHEBI_32386"},{"id":"A55657","pred":"chebi_id","subj":"T82620","obj":"http://purl.obolibrary.org/obo/CHEBI_73498"},{"id":"A94130","pred":"chebi_id","subj":"T96787","obj":"http://purl.obolibrary.org/obo/CHEBI_5032"},{"id":"A85576","pred":"chebi_id","subj":"T63","obj":"http://purl.obolibrary.org/obo/CHEBI_18248"},{"id":"A18980","pred":"chebi_id","subj":"T50019","obj":"http://purl.obolibrary.org/obo/CHEBI_50406"},{"id":"A50462","pred":"chebi_id","subj":"T26443","obj":"http://purl.obolibrary.org/obo/CHEBI_32386"},{"id":"A76046","pred":"chebi_id","subj":"T65271","obj":"http://purl.obolibrary.org/obo/CHEBI_18248"},{"id":"A63564","pred":"chebi_id","subj":"T22889","obj":"http://purl.obolibrary.org/obo/CHEBI_36080"},{"id":"A27307","pred":"chebi_id","subj":"T68","obj":"http://purl.obolibrary.org/obo/CHEBI_36080"},{"id":"A41287","pred":"chebi_id","subj":"T69","obj":"http://purl.obolibrary.org/obo/CHEBI_52214"},{"id":"A40756","pred":"chebi_id","subj":"T84319","obj":"http://purl.obolibrary.org/obo/CHEBI_16670"},{"id":"A31288","pred":"chebi_id","subj":"T31243","obj":"http://purl.obolibrary.org/obo/CHEBI_36080"},{"id":"A2686","pred":"chebi_id","subj":"T64538","obj":"http://purl.obolibrary.org/obo/CHEBI_25676"},{"id":"A30308","pred":"chebi_id","subj":"T30093","obj":"http://purl.obolibrary.org/obo/CHEBI_16646"},{"id":"A89937","pred":"chebi_id","subj":"T34862","obj":"http://purl.obolibrary.org/obo/CHEBI_16646"},{"id":"A47868","pred":"chebi_id","subj":"T13773","obj":"http://purl.obolibrary.org/obo/CHEBI_27150"},{"id":"A88434","pred":"chebi_id","subj":"T52864","obj":"http://purl.obolibrary.org/obo/CHEBI_52214"},{"id":"A43932","pred":"chebi_id","subj":"T44200","obj":"http://purl.obolibrary.org/obo/CHEBI_27150"},{"id":"A23267","pred":"chebi_id","subj":"T70626","obj":"http://purl.obolibrary.org/obo/CHEBI_52214"},{"id":"A14715","pred":"chebi_id","subj":"T79","obj":"http://purl.obolibrary.org/obo/CHEBI_16646"},{"id":"A10852","pred":"chebi_id","subj":"T58945","obj":"http://purl.obolibrary.org/obo/CHEBI_16646"},{"id":"A86855","pred":"chebi_id","subj":"T81","obj":"http://purl.obolibrary.org/obo/CHEBI_36080"},{"id":"A28859","pred":"chebi_id","subj":"T83556","obj":"http://purl.obolibrary.org/obo/CHEBI_9160"},{"id":"A5943","pred":"chebi_id","subj":"T94086","obj":"http://purl.obolibrary.org/obo/CHEBI_16991"},{"id":"A54950","pred":"chebi_id","subj":"T14585","obj":"http://purl.obolibrary.org/obo/CHEBI_9160"},{"id":"A9785","pred":"chebi_id","subj":"T20829","obj":"http://purl.obolibrary.org/obo/CHEBI_9160"},{"id":"A6700","pred":"chebi_id","subj":"T89112","obj":"http://purl.obolibrary.org/obo/CHEBI_16991"},{"id":"A30931","pred":"chebi_id","subj":"T93354","obj":"http://purl.obolibrary.org/obo/CHEBI_9160"},{"id":"A97159","pred":"chebi_id","subj":"T35047","obj":"http://purl.obolibrary.org/obo/CHEBI_140488"},{"id":"A89712","pred":"chebi_id","subj":"T63521","obj":"http://purl.obolibrary.org/obo/CHEBI_7754"},{"id":"A49126","pred":"chebi_id","subj":"T41981","obj":"http://purl.obolibrary.org/obo/CHEBI_25367"},{"id":"A4635","pred":"chebi_id","subj":"T62122","obj":"http://purl.obolibrary.org/obo/CHEBI_52214"},{"id":"A40956","pred":"chebi_id","subj":"T9235","obj":"http://purl.obolibrary.org/obo/CHEBI_7754"},{"id":"A37222","pred":"chebi_id","subj":"T54261","obj":"http://purl.obolibrary.org/obo/CHEBI_140488"},{"id":"A51504","pred":"chebi_id","subj":"T49855","obj":"http://purl.obolibrary.org/obo/CHEBI_140488"},{"id":"A47519","pred":"chebi_id","subj":"T32746","obj":"http://purl.obolibrary.org/obo/CHEBI_16991"},{"id":"A74972","pred":"chebi_id","subj":"T99337","obj":"http://purl.obolibrary.org/obo/CHEBI_140488"},{"id":"A14510","pred":"chebi_id","subj":"T97","obj":"http://purl.obolibrary.org/obo/CHEBI_140488"},{"id":"A60333","pred":"chebi_id","subj":"T86290","obj":"http://purl.obolibrary.org/obo/CHEBI_140488"},{"id":"A87542","pred":"chebi_id","subj":"T44669","obj":"http://purl.obolibrary.org/obo/CHEBI_36080"},{"id":"A64640","pred":"chebi_id","subj":"T56913","obj":"http://purl.obolibrary.org/obo/CHEBI_18332"},{"id":"A83588","pred":"chebi_id","subj":"T56913","obj":"http://purl.obolibrary.org/obo/CHEBI_75602"},{"id":"A25641","pred":"chebi_id","subj":"T5510","obj":"http://purl.obolibrary.org/obo/CHEBI_15377"},{"id":"A44498","pred":"chebi_id","subj":"T53479","obj":"http://purl.obolibrary.org/obo/CHEBI_73498"},{"id":"A66037","pred":"chebi_id","subj":"T8615","obj":"http://purl.obolibrary.org/obo/CHEBI_8005"},{"id":"A16676","pred":"chebi_id","subj":"T105","obj":"http://purl.obolibrary.org/obo/CHEBI_29287"},{"id":"A55914","pred":"chebi_id","subj":"T43880","obj":"http://purl.obolibrary.org/obo/CHEBI_15377"},{"id":"A20491","pred":"chebi_id","subj":"T66902","obj":"http://purl.obolibrary.org/obo/CHEBI_33839"},{"id":"A99849","pred":"chebi_id","subj":"T37286","obj":"http://purl.obolibrary.org/obo/CHEBI_140488"},{"id":"A91428","pred":"chebi_id","subj":"T54658","obj":"http://purl.obolibrary.org/obo/CHEBI_33839"},{"id":"A18468","pred":"chebi_id","subj":"T67116","obj":"http://purl.obolibrary.org/obo/CHEBI_37527"},{"id":"A46269","pred":"chebi_id","subj":"T50783","obj":"http://purl.obolibrary.org/obo/CHEBI_140488"}],"text":"2.2 Biorecognition elements\nThe previous section discussed the transduction elements associated with pathogen detection using electrochemical biosensors. Given a biosensor is a device composed of integrated transducer and biorecognition elements, we next discuss the biorecognition elements used for selective detection of pathogens and corresponding immobilization techniques for their coupling to electrodes.\nBiorecognition elements for electrochemical biosensors can be defined as (1) biocatalytic or (2) biocomplexing. In the case of biocatalytic biorecognition elements, the biosensor response is based on a reaction catalyzed by macromolecules. Enzymes, whole cells, and tissues are the most commonly used biocatalytic biorecognition element. While enzyzmes provide biorecognition elements in various chemical sensing applications, they are often used as labels for pathogen detection applications and most commonly introduced via secondary binding steps. In the case of biocomplexing biorecognition elements, the biosensor response is based on the interaction of analytes with macromolecules or organized molecular assemblies. As shown in Table 1, Table 2, antibodies, peptides, and phages are the most commonly used biocomplexing biorecognition elements for pathogen detection. In addition to biomacromolecules, imprinted polymers have also been examined as biocomplexing biorecognition elements for pathogen detection using electrochemical biosensors.\n\n2.2.1 Antibodies and antibody fragments\nAntibodies and antibody fragments are among the most commonly utilized biorecognition elements for pathogen detection using electrochemical biosensors. Biosensors employing antibody-based biorecognition elements are commonly referred to as immunosensors. Given antibodies exhibit high selectivity and binding affinity for target species and can be generated for a wide range of infectious agents, antibodies are the gold-standard biorecognition element for pathogen detection. Antibodies contain recognition sites that selectively bind to antigens through a specific region of the antigen, referred to as an epitope (Patris et al. 2016). Antibodies can be labeled with fluorescent or enzymatic tags, which leads to the designation of the approach as label-based. While label-based approaches present measurement constraints associated with the use of additional reagents and processing steps (Cooper, 2009; Sang et al. 2016), antibody labeling may also alter the binding affinity to the antigen, which could affect the biosensor's selectivity. A detailed discussion of label-based biosensing approaches for pathogen detection has been reported elsewhere (Ahmed et al. 2014; Alahi and Mukhopadhyay, 2017; Bozal-Palabiyik et al. 2018; Leonard et al. 2003). A list of recent label-based approaches for pathogen detection using electrochemical biosensors, however, is provided in Table 2.\nWhile both monoclonal and polyclonal antibodies enable the selective detection of pathogens (Patris et al. 2016), they vary in terms of production method, selectivity, and binding affinity. Monoclonal antibodies are produced by hybridoma technology (Birch and Racher, 2006; James and Bell, 1987). Thus, monoclonal antibodies are highly selective and bind to a single epitope, making them less vulnerable to cross-reactivity. While monoclonal antibodies tend to have a higher degree of selectivity, they are more expensive and take longer to develop than polyclonal antibodies. Polyclonal antibodies are produced by separation of immunoglobulin proteins from the blood of an infected host (Birch and Racher, 2006). Polyclonal antibodies target different epitopes on a single antigen. While polyclonal antibodies exhibit increased variability between batches, they are relatively less expensive to produce than monoclonal antibodies and facilitate robust measurements in various settings (Byrne et al. 2009). Drawbacks to antibody use include high cost and stability challenges, such as the need for low-temperature storage. As shown in Table 1, Table 2, both monoclonal and polyclonal antibodies are used as biorecognition elements for pathogen detection. For assays involving secondary binding steps, monoclonal antibodies typically serve as the primary biorecognition element and are immobilized on the electrode, while polyclonal antibodies serve as the secondary biorecognition element and often facilitate target labeling. For assays that do not require secondary binding steps, polyclonal antibodies are also commonly used as immobilized biorecognition elements for pathogen detection. For example, Pandey et al. immobilized monoclonal anti-E. coli on a composite nanostructured electrode to detect E. coli across a wide dynamic range of 10 to 108 CFU/mL with a LOD of 3.8 CFU/mL (Pandey et al. 2017). Wu et al. used polyclonal anti-E. coli for detection of E. coli via amperometry that exhibited a LOD of 5 × 103 CFU/mL (Wu et al. 2016). Lin et al. used monoclonal antibodies for detection of avian influenza virus H5N1 in chicken swabs across a dynamic range of 2- 1 to 24 hemagglutination units (HAU)/50 μL using EIS and the ferri/ferrocyanide (Fe(CN)6 3 - /4-) couple as a redox probe (Lin et al. 2015). Luka et al. detected Cryptosporidium parvum (C. parvum) with a LOD of 40 cells/mm2 via capacitive sensing and Fe(CN)6 3 - /4- (Luka et al. 2019).\nAntibody fragments, such as single-chain variable fragments (scFvs), offer selectivity similar to antibodies, but they have the advantage of achieving relatively higher packing densities on electrode surfaces due to their relatively smaller size. For example, half-antibody fragments have been shown to improve biosensor sensitivity without the loss of selectivity, which warrants further investigation of reduced antibodies as biorecognition elements for pathogen detection applications (Sharma and Mutharasan, 2013). In addition to scFvs, Fabs, re-engineered IgGs, and dimers can also potentially be used as biorecognition elements for pathogen detection (Byrne et al. 2009).\n\n2.2.2 Carbohydrate-binding proteins\nCarbohydrate-binding proteins, such as lectins, also provide selective biorecognition elements for pathogen detection based on their ability to selectively bind ligands on target species. Peptide-based biorecognition elements are relatively low-cost, can be produced with high yield automated synthesis processes, and are modifiable (Pavan and Berti, 2012). For example, lectins have been investigated as biorecognition elements for pathogen detection through their ability to selectively bind glycosylated proteins on the surfaces of viruses and cells (Reina et al. 2008). Concanavalin A (ConA) lectin has been extensively investigated for E. coli detection (see Table 1) (Jantra et al. 2011; Saucedo et al. 2019; Xi et al. 2011; Yang et al. 2016b). While not yet widely investigated for pathogen detection using electrochemical biosensors, Etayash et al. recently showed that oligopeptides also provide attractive biorecognition elements for real-time biosensor-based detection of breast cancer cells (Etayash et al. 2015).\n\n2.2.3 Oligosaccharides\nTrisaccharides are carbohydrates that can selectively bind carbohydrate-specific receptors on pathogens. Thus, trisaccharide ligands have been used as biorecognition elements for pathogen detection using electrochemical biosensors. For example, Hai et al. used a hybrid E-QCM biosensor coated with hemagglutinin-specific trisaccharide ligands for the detection of human influenza A virus (H1N1) (Hai et al. 2017). The use of carbohydrates as biorecognition elements is limited in part due to the weak affinity of carbohydrate-protein interactions and low selectivity, which are currently mitigated through secondary interactions (Zeng et al. 2012).\n\n2.2.4 Oligonucleotides\nSingle-stranded DNA (ssDNA) is a useful biorecognition element for the detection of pathogens. While ssDNA is commonly used as a biorecognition element for DNA-based assays, ssDNA aptamers are commonly used for pathogen detection using electrochemical biosensors. Aptamers are single-stranded oligonucleotides capable of binding various molecules with high affinity and selectivity (Lakhin et al. 2013; Reverdatto et al. 2015). Aptamers are isolated from a large random sequence pool through a selection process that utilizes systematic evolution of ligands by exponential enrichment, also known as SELEX (Stoltenburg et al. 2007). Suitable binding sequences can be isolated from a large random oligonucleotide sequence pool and subsequently amplified for use. Thus, aptamers can exhibit high selectivity to target species (Stoltenburg et al. 2007). Aptamers can also be produced at a lower cost than alternative biorecognition elements, such as antibodies. Giamberardino et al. used SELEX to discover an aptamer for norovirus detection, which showed a million-fold higher binding affinity for the target than a random DNA strand that served as a negative control (Giamberardino et al. 2013). Iqbal et al. performed 10 rounds of SELEX to discover 14 aptamer clones with high affinities for C. parvum for detection in fruit samples (Iqbal et al. 2015). However, the use of aptamers as biorecognition elements has not yet replaced traditional biorecognition elements, such as antibodies, because of several challenges, such as aptamer stability, degradation, cross-reactivity, and reproducibility using alternative processing approaches (Lakhin et al. 2013).\n\n2.2.5 Phages\nPhages, also referred to as bacteriophages, are viruses that infect and replicate in bacteria through selective binding via tail-spike proteins (Haq et al. 2012). Thus, they have been examined as biorecognition elements for pathogen detection using electrochemical biosensors (Kutter and Sulakvelidze, 2004). Bacteriophages exhibit varying morphologies and are thus classified by selectivity and structure. A variety of bacteriophage-based electrochemical biosensors for pathogen detection can be found in Table 1. For example, Shabani et al. used E. coli-specific T4 bacteriophages for selective impedimetric detection studies (Shabani et al. 2008). Mejri et al. compared the use of bacteriophages to antibodies as biorecognition elements for E. coli detection (Mejri et al. 2010). In that study, they found that bacteriophages improved the water stability of the biosensor and increased the sensitivity by approximately a factor of four relative to the response obtained with antibodies based on EIS measurements (Mejri et al. 2010). In another study, Tolba et al. utilized immobilized bacteriophage-encoded peptidoglycan hydrolases on Au screen-printed electrodes for detection of L. innocua in pure milk with a LOD of 105 CFU/mL (Tolba et al. 2012). These results suggest that bacteriophages are potentially attractive biorecognition elements for water safety and environmental monitoring applications that require chronic monitoring of liquids.\n\n2.2.6 Cell- and molecularly-imprinted polymers\nGiven traditional biorecognition elements used in biosensing exhibit stability concerns, such as antibodies or aptamers, as discussed in Sections 2.2.1–2.2.4, there have been efforts to create engineered molecular biorecognition elements, such as scFvs. In contrast, materials-based biorecognition elements exploit the principle of target-specific morphology for selective capture (Pan et al. 2018; Zhou et al. 2019). The most common approach in materials-based biorecognition is based on cell- and molecularly-imprinted polymers (CIPs and MIPs, respectively) (Gui et al. 2018). CIPs and MIPs have been created using various processes, including bacteria-mediated lithography, micro-contact stamping, and colloid imprints (Chen et al. 2016a; Pan et al. 2018).\nAs shown in Fig. 3b, Jafari et al. used imprinted organosilica sol-gel films of tetraethoxysilane and (3-mercaptopropyl)trimethoxysilane (MPTS) for selective detection of E. coli using an impedimetric method (Jafari et al. 2019). Similarly, Golabi et al. used imprinted poly(3-aminophenylboronic acid) films for detection of Staphylococcus epidermidis (S. epidermidis) (Golabi et al. 2017). Despite the absence of a highly selective molecular biorecognition element, CIPs and MIPs exhibit selectivity when exposed to samples that contain multiple analytes (i.e., non-target species) (Golabi et al. 2017; Jafari et al. 2019; Qi et al. 2013). MIPs and CIPs are also of interest with regard to opportunities in biosensor regeneration. Common adverse effects of regeneration on biosensors that employ molecular biorecognition elements, such as irreversible changes in structure, are less likely to affect MIPs and CIPs. However, it is generally accepted that current CIPs and MIPs exhibit lower selectivity to target species than antibodies and aptamers due to reduction of available chemical selectivity (Cheong et al. 2013; Kryscio and Peppas, 2012; Yáñez-Sedeño et al. 2017)."}
LitCovid-PD-GO-BP
{"project":"LitCovid-PD-GO-BP","denotations":[{"id":"T13152","span":{"begin":64,"end":76},"obj":"http://purl.obolibrary.org/obo/GO_0009293"},{"id":"T34150","span":{"begin":4003,"end":4010},"obj":"http://purl.obolibrary.org/obo/GO_0051235"},{"id":"T85365","span":{"begin":6357,"end":6366},"obj":"http://purl.obolibrary.org/obo/GO_0009058"},{"id":"T28","span":{"begin":9333,"end":9344},"obj":"http://purl.obolibrary.org/obo/GO_0009056"},{"id":"T29","span":{"begin":12438,"end":12450},"obj":"http://purl.obolibrary.org/obo/GO_0031099"},{"id":"T30","span":{"begin":12478,"end":12490},"obj":"http://purl.obolibrary.org/obo/GO_0031099"}],"text":"2.2 Biorecognition elements\nThe previous section discussed the transduction elements associated with pathogen detection using electrochemical biosensors. Given a biosensor is a device composed of integrated transducer and biorecognition elements, we next discuss the biorecognition elements used for selective detection of pathogens and corresponding immobilization techniques for their coupling to electrodes.\nBiorecognition elements for electrochemical biosensors can be defined as (1) biocatalytic or (2) biocomplexing. In the case of biocatalytic biorecognition elements, the biosensor response is based on a reaction catalyzed by macromolecules. Enzymes, whole cells, and tissues are the most commonly used biocatalytic biorecognition element. While enzyzmes provide biorecognition elements in various chemical sensing applications, they are often used as labels for pathogen detection applications and most commonly introduced via secondary binding steps. In the case of biocomplexing biorecognition elements, the biosensor response is based on the interaction of analytes with macromolecules or organized molecular assemblies. As shown in Table 1, Table 2, antibodies, peptides, and phages are the most commonly used biocomplexing biorecognition elements for pathogen detection. In addition to biomacromolecules, imprinted polymers have also been examined as biocomplexing biorecognition elements for pathogen detection using electrochemical biosensors.\n\n2.2.1 Antibodies and antibody fragments\nAntibodies and antibody fragments are among the most commonly utilized biorecognition elements for pathogen detection using electrochemical biosensors. Biosensors employing antibody-based biorecognition elements are commonly referred to as immunosensors. Given antibodies exhibit high selectivity and binding affinity for target species and can be generated for a wide range of infectious agents, antibodies are the gold-standard biorecognition element for pathogen detection. Antibodies contain recognition sites that selectively bind to antigens through a specific region of the antigen, referred to as an epitope (Patris et al. 2016). Antibodies can be labeled with fluorescent or enzymatic tags, which leads to the designation of the approach as label-based. While label-based approaches present measurement constraints associated with the use of additional reagents and processing steps (Cooper, 2009; Sang et al. 2016), antibody labeling may also alter the binding affinity to the antigen, which could affect the biosensor's selectivity. A detailed discussion of label-based biosensing approaches for pathogen detection has been reported elsewhere (Ahmed et al. 2014; Alahi and Mukhopadhyay, 2017; Bozal-Palabiyik et al. 2018; Leonard et al. 2003). A list of recent label-based approaches for pathogen detection using electrochemical biosensors, however, is provided in Table 2.\nWhile both monoclonal and polyclonal antibodies enable the selective detection of pathogens (Patris et al. 2016), they vary in terms of production method, selectivity, and binding affinity. Monoclonal antibodies are produced by hybridoma technology (Birch and Racher, 2006; James and Bell, 1987). Thus, monoclonal antibodies are highly selective and bind to a single epitope, making them less vulnerable to cross-reactivity. While monoclonal antibodies tend to have a higher degree of selectivity, they are more expensive and take longer to develop than polyclonal antibodies. Polyclonal antibodies are produced by separation of immunoglobulin proteins from the blood of an infected host (Birch and Racher, 2006). Polyclonal antibodies target different epitopes on a single antigen. While polyclonal antibodies exhibit increased variability between batches, they are relatively less expensive to produce than monoclonal antibodies and facilitate robust measurements in various settings (Byrne et al. 2009). Drawbacks to antibody use include high cost and stability challenges, such as the need for low-temperature storage. As shown in Table 1, Table 2, both monoclonal and polyclonal antibodies are used as biorecognition elements for pathogen detection. For assays involving secondary binding steps, monoclonal antibodies typically serve as the primary biorecognition element and are immobilized on the electrode, while polyclonal antibodies serve as the secondary biorecognition element and often facilitate target labeling. For assays that do not require secondary binding steps, polyclonal antibodies are also commonly used as immobilized biorecognition elements for pathogen detection. For example, Pandey et al. immobilized monoclonal anti-E. coli on a composite nanostructured electrode to detect E. coli across a wide dynamic range of 10 to 108 CFU/mL with a LOD of 3.8 CFU/mL (Pandey et al. 2017). Wu et al. used polyclonal anti-E. coli for detection of E. coli via amperometry that exhibited a LOD of 5 × 103 CFU/mL (Wu et al. 2016). Lin et al. used monoclonal antibodies for detection of avian influenza virus H5N1 in chicken swabs across a dynamic range of 2- 1 to 24 hemagglutination units (HAU)/50 μL using EIS and the ferri/ferrocyanide (Fe(CN)6 3 - /4-) couple as a redox probe (Lin et al. 2015). Luka et al. detected Cryptosporidium parvum (C. parvum) with a LOD of 40 cells/mm2 via capacitive sensing and Fe(CN)6 3 - /4- (Luka et al. 2019).\nAntibody fragments, such as single-chain variable fragments (scFvs), offer selectivity similar to antibodies, but they have the advantage of achieving relatively higher packing densities on electrode surfaces due to their relatively smaller size. For example, half-antibody fragments have been shown to improve biosensor sensitivity without the loss of selectivity, which warrants further investigation of reduced antibodies as biorecognition elements for pathogen detection applications (Sharma and Mutharasan, 2013). In addition to scFvs, Fabs, re-engineered IgGs, and dimers can also potentially be used as biorecognition elements for pathogen detection (Byrne et al. 2009).\n\n2.2.2 Carbohydrate-binding proteins\nCarbohydrate-binding proteins, such as lectins, also provide selective biorecognition elements for pathogen detection based on their ability to selectively bind ligands on target species. Peptide-based biorecognition elements are relatively low-cost, can be produced with high yield automated synthesis processes, and are modifiable (Pavan and Berti, 2012). For example, lectins have been investigated as biorecognition elements for pathogen detection through their ability to selectively bind glycosylated proteins on the surfaces of viruses and cells (Reina et al. 2008). Concanavalin A (ConA) lectin has been extensively investigated for E. coli detection (see Table 1) (Jantra et al. 2011; Saucedo et al. 2019; Xi et al. 2011; Yang et al. 2016b). While not yet widely investigated for pathogen detection using electrochemical biosensors, Etayash et al. recently showed that oligopeptides also provide attractive biorecognition elements for real-time biosensor-based detection of breast cancer cells (Etayash et al. 2015).\n\n2.2.3 Oligosaccharides\nTrisaccharides are carbohydrates that can selectively bind carbohydrate-specific receptors on pathogens. Thus, trisaccharide ligands have been used as biorecognition elements for pathogen detection using electrochemical biosensors. For example, Hai et al. used a hybrid E-QCM biosensor coated with hemagglutinin-specific trisaccharide ligands for the detection of human influenza A virus (H1N1) (Hai et al. 2017). The use of carbohydrates as biorecognition elements is limited in part due to the weak affinity of carbohydrate-protein interactions and low selectivity, which are currently mitigated through secondary interactions (Zeng et al. 2012).\n\n2.2.4 Oligonucleotides\nSingle-stranded DNA (ssDNA) is a useful biorecognition element for the detection of pathogens. While ssDNA is commonly used as a biorecognition element for DNA-based assays, ssDNA aptamers are commonly used for pathogen detection using electrochemical biosensors. Aptamers are single-stranded oligonucleotides capable of binding various molecules with high affinity and selectivity (Lakhin et al. 2013; Reverdatto et al. 2015). Aptamers are isolated from a large random sequence pool through a selection process that utilizes systematic evolution of ligands by exponential enrichment, also known as SELEX (Stoltenburg et al. 2007). Suitable binding sequences can be isolated from a large random oligonucleotide sequence pool and subsequently amplified for use. Thus, aptamers can exhibit high selectivity to target species (Stoltenburg et al. 2007). Aptamers can also be produced at a lower cost than alternative biorecognition elements, such as antibodies. Giamberardino et al. used SELEX to discover an aptamer for norovirus detection, which showed a million-fold higher binding affinity for the target than a random DNA strand that served as a negative control (Giamberardino et al. 2013). Iqbal et al. performed 10 rounds of SELEX to discover 14 aptamer clones with high affinities for C. parvum for detection in fruit samples (Iqbal et al. 2015). However, the use of aptamers as biorecognition elements has not yet replaced traditional biorecognition elements, such as antibodies, because of several challenges, such as aptamer stability, degradation, cross-reactivity, and reproducibility using alternative processing approaches (Lakhin et al. 2013).\n\n2.2.5 Phages\nPhages, also referred to as bacteriophages, are viruses that infect and replicate in bacteria through selective binding via tail-spike proteins (Haq et al. 2012). Thus, they have been examined as biorecognition elements for pathogen detection using electrochemical biosensors (Kutter and Sulakvelidze, 2004). Bacteriophages exhibit varying morphologies and are thus classified by selectivity and structure. A variety of bacteriophage-based electrochemical biosensors for pathogen detection can be found in Table 1. For example, Shabani et al. used E. coli-specific T4 bacteriophages for selective impedimetric detection studies (Shabani et al. 2008). Mejri et al. compared the use of bacteriophages to antibodies as biorecognition elements for E. coli detection (Mejri et al. 2010). In that study, they found that bacteriophages improved the water stability of the biosensor and increased the sensitivity by approximately a factor of four relative to the response obtained with antibodies based on EIS measurements (Mejri et al. 2010). In another study, Tolba et al. utilized immobilized bacteriophage-encoded peptidoglycan hydrolases on Au screen-printed electrodes for detection of L. innocua in pure milk with a LOD of 105 CFU/mL (Tolba et al. 2012). These results suggest that bacteriophages are potentially attractive biorecognition elements for water safety and environmental monitoring applications that require chronic monitoring of liquids.\n\n2.2.6 Cell- and molecularly-imprinted polymers\nGiven traditional biorecognition elements used in biosensing exhibit stability concerns, such as antibodies or aptamers, as discussed in Sections 2.2.1–2.2.4, there have been efforts to create engineered molecular biorecognition elements, such as scFvs. In contrast, materials-based biorecognition elements exploit the principle of target-specific morphology for selective capture (Pan et al. 2018; Zhou et al. 2019). The most common approach in materials-based biorecognition is based on cell- and molecularly-imprinted polymers (CIPs and MIPs, respectively) (Gui et al. 2018). CIPs and MIPs have been created using various processes, including bacteria-mediated lithography, micro-contact stamping, and colloid imprints (Chen et al. 2016a; Pan et al. 2018).\nAs shown in Fig. 3b, Jafari et al. used imprinted organosilica sol-gel films of tetraethoxysilane and (3-mercaptopropyl)trimethoxysilane (MPTS) for selective detection of E. coli using an impedimetric method (Jafari et al. 2019). Similarly, Golabi et al. used imprinted poly(3-aminophenylboronic acid) films for detection of Staphylococcus epidermidis (S. epidermidis) (Golabi et al. 2017). Despite the absence of a highly selective molecular biorecognition element, CIPs and MIPs exhibit selectivity when exposed to samples that contain multiple analytes (i.e., non-target species) (Golabi et al. 2017; Jafari et al. 2019; Qi et al. 2013). MIPs and CIPs are also of interest with regard to opportunities in biosensor regeneration. Common adverse effects of regeneration on biosensors that employ molecular biorecognition elements, such as irreversible changes in structure, are less likely to affect MIPs and CIPs. However, it is generally accepted that current CIPs and MIPs exhibit lower selectivity to target species than antibodies and aptamers due to reduction of available chemical selectivity (Cheong et al. 2013; Kryscio and Peppas, 2012; Yáñez-Sedeño et al. 2017)."}
LitCovid-PD-HP
{"project":"LitCovid-PD-HP","denotations":[{"id":"T9","span":{"begin":7047,"end":7060},"obj":"Phenotype"}],"attributes":[{"id":"A9","pred":"hp_id","subj":"T9","obj":"http://purl.obolibrary.org/obo/HP_0003002"}],"text":"2.2 Biorecognition elements\nThe previous section discussed the transduction elements associated with pathogen detection using electrochemical biosensors. Given a biosensor is a device composed of integrated transducer and biorecognition elements, we next discuss the biorecognition elements used for selective detection of pathogens and corresponding immobilization techniques for their coupling to electrodes.\nBiorecognition elements for electrochemical biosensors can be defined as (1) biocatalytic or (2) biocomplexing. In the case of biocatalytic biorecognition elements, the biosensor response is based on a reaction catalyzed by macromolecules. Enzymes, whole cells, and tissues are the most commonly used biocatalytic biorecognition element. While enzyzmes provide biorecognition elements in various chemical sensing applications, they are often used as labels for pathogen detection applications and most commonly introduced via secondary binding steps. In the case of biocomplexing biorecognition elements, the biosensor response is based on the interaction of analytes with macromolecules or organized molecular assemblies. As shown in Table 1, Table 2, antibodies, peptides, and phages are the most commonly used biocomplexing biorecognition elements for pathogen detection. In addition to biomacromolecules, imprinted polymers have also been examined as biocomplexing biorecognition elements for pathogen detection using electrochemical biosensors.\n\n2.2.1 Antibodies and antibody fragments\nAntibodies and antibody fragments are among the most commonly utilized biorecognition elements for pathogen detection using electrochemical biosensors. Biosensors employing antibody-based biorecognition elements are commonly referred to as immunosensors. Given antibodies exhibit high selectivity and binding affinity for target species and can be generated for a wide range of infectious agents, antibodies are the gold-standard biorecognition element for pathogen detection. Antibodies contain recognition sites that selectively bind to antigens through a specific region of the antigen, referred to as an epitope (Patris et al. 2016). Antibodies can be labeled with fluorescent or enzymatic tags, which leads to the designation of the approach as label-based. While label-based approaches present measurement constraints associated with the use of additional reagents and processing steps (Cooper, 2009; Sang et al. 2016), antibody labeling may also alter the binding affinity to the antigen, which could affect the biosensor's selectivity. A detailed discussion of label-based biosensing approaches for pathogen detection has been reported elsewhere (Ahmed et al. 2014; Alahi and Mukhopadhyay, 2017; Bozal-Palabiyik et al. 2018; Leonard et al. 2003). A list of recent label-based approaches for pathogen detection using electrochemical biosensors, however, is provided in Table 2.\nWhile both monoclonal and polyclonal antibodies enable the selective detection of pathogens (Patris et al. 2016), they vary in terms of production method, selectivity, and binding affinity. Monoclonal antibodies are produced by hybridoma technology (Birch and Racher, 2006; James and Bell, 1987). Thus, monoclonal antibodies are highly selective and bind to a single epitope, making them less vulnerable to cross-reactivity. While monoclonal antibodies tend to have a higher degree of selectivity, they are more expensive and take longer to develop than polyclonal antibodies. Polyclonal antibodies are produced by separation of immunoglobulin proteins from the blood of an infected host (Birch and Racher, 2006). Polyclonal antibodies target different epitopes on a single antigen. While polyclonal antibodies exhibit increased variability between batches, they are relatively less expensive to produce than monoclonal antibodies and facilitate robust measurements in various settings (Byrne et al. 2009). Drawbacks to antibody use include high cost and stability challenges, such as the need for low-temperature storage. As shown in Table 1, Table 2, both monoclonal and polyclonal antibodies are used as biorecognition elements for pathogen detection. For assays involving secondary binding steps, monoclonal antibodies typically serve as the primary biorecognition element and are immobilized on the electrode, while polyclonal antibodies serve as the secondary biorecognition element and often facilitate target labeling. For assays that do not require secondary binding steps, polyclonal antibodies are also commonly used as immobilized biorecognition elements for pathogen detection. For example, Pandey et al. immobilized monoclonal anti-E. coli on a composite nanostructured electrode to detect E. coli across a wide dynamic range of 10 to 108 CFU/mL with a LOD of 3.8 CFU/mL (Pandey et al. 2017). Wu et al. used polyclonal anti-E. coli for detection of E. coli via amperometry that exhibited a LOD of 5 × 103 CFU/mL (Wu et al. 2016). Lin et al. used monoclonal antibodies for detection of avian influenza virus H5N1 in chicken swabs across a dynamic range of 2- 1 to 24 hemagglutination units (HAU)/50 μL using EIS and the ferri/ferrocyanide (Fe(CN)6 3 - /4-) couple as a redox probe (Lin et al. 2015). Luka et al. detected Cryptosporidium parvum (C. parvum) with a LOD of 40 cells/mm2 via capacitive sensing and Fe(CN)6 3 - /4- (Luka et al. 2019).\nAntibody fragments, such as single-chain variable fragments (scFvs), offer selectivity similar to antibodies, but they have the advantage of achieving relatively higher packing densities on electrode surfaces due to their relatively smaller size. For example, half-antibody fragments have been shown to improve biosensor sensitivity without the loss of selectivity, which warrants further investigation of reduced antibodies as biorecognition elements for pathogen detection applications (Sharma and Mutharasan, 2013). In addition to scFvs, Fabs, re-engineered IgGs, and dimers can also potentially be used as biorecognition elements for pathogen detection (Byrne et al. 2009).\n\n2.2.2 Carbohydrate-binding proteins\nCarbohydrate-binding proteins, such as lectins, also provide selective biorecognition elements for pathogen detection based on their ability to selectively bind ligands on target species. Peptide-based biorecognition elements are relatively low-cost, can be produced with high yield automated synthesis processes, and are modifiable (Pavan and Berti, 2012). For example, lectins have been investigated as biorecognition elements for pathogen detection through their ability to selectively bind glycosylated proteins on the surfaces of viruses and cells (Reina et al. 2008). Concanavalin A (ConA) lectin has been extensively investigated for E. coli detection (see Table 1) (Jantra et al. 2011; Saucedo et al. 2019; Xi et al. 2011; Yang et al. 2016b). While not yet widely investigated for pathogen detection using electrochemical biosensors, Etayash et al. recently showed that oligopeptides also provide attractive biorecognition elements for real-time biosensor-based detection of breast cancer cells (Etayash et al. 2015).\n\n2.2.3 Oligosaccharides\nTrisaccharides are carbohydrates that can selectively bind carbohydrate-specific receptors on pathogens. Thus, trisaccharide ligands have been used as biorecognition elements for pathogen detection using electrochemical biosensors. For example, Hai et al. used a hybrid E-QCM biosensor coated with hemagglutinin-specific trisaccharide ligands for the detection of human influenza A virus (H1N1) (Hai et al. 2017). The use of carbohydrates as biorecognition elements is limited in part due to the weak affinity of carbohydrate-protein interactions and low selectivity, which are currently mitigated through secondary interactions (Zeng et al. 2012).\n\n2.2.4 Oligonucleotides\nSingle-stranded DNA (ssDNA) is a useful biorecognition element for the detection of pathogens. While ssDNA is commonly used as a biorecognition element for DNA-based assays, ssDNA aptamers are commonly used for pathogen detection using electrochemical biosensors. Aptamers are single-stranded oligonucleotides capable of binding various molecules with high affinity and selectivity (Lakhin et al. 2013; Reverdatto et al. 2015). Aptamers are isolated from a large random sequence pool through a selection process that utilizes systematic evolution of ligands by exponential enrichment, also known as SELEX (Stoltenburg et al. 2007). Suitable binding sequences can be isolated from a large random oligonucleotide sequence pool and subsequently amplified for use. Thus, aptamers can exhibit high selectivity to target species (Stoltenburg et al. 2007). Aptamers can also be produced at a lower cost than alternative biorecognition elements, such as antibodies. Giamberardino et al. used SELEX to discover an aptamer for norovirus detection, which showed a million-fold higher binding affinity for the target than a random DNA strand that served as a negative control (Giamberardino et al. 2013). Iqbal et al. performed 10 rounds of SELEX to discover 14 aptamer clones with high affinities for C. parvum for detection in fruit samples (Iqbal et al. 2015). However, the use of aptamers as biorecognition elements has not yet replaced traditional biorecognition elements, such as antibodies, because of several challenges, such as aptamer stability, degradation, cross-reactivity, and reproducibility using alternative processing approaches (Lakhin et al. 2013).\n\n2.2.5 Phages\nPhages, also referred to as bacteriophages, are viruses that infect and replicate in bacteria through selective binding via tail-spike proteins (Haq et al. 2012). Thus, they have been examined as biorecognition elements for pathogen detection using electrochemical biosensors (Kutter and Sulakvelidze, 2004). Bacteriophages exhibit varying morphologies and are thus classified by selectivity and structure. A variety of bacteriophage-based electrochemical biosensors for pathogen detection can be found in Table 1. For example, Shabani et al. used E. coli-specific T4 bacteriophages for selective impedimetric detection studies (Shabani et al. 2008). Mejri et al. compared the use of bacteriophages to antibodies as biorecognition elements for E. coli detection (Mejri et al. 2010). In that study, they found that bacteriophages improved the water stability of the biosensor and increased the sensitivity by approximately a factor of four relative to the response obtained with antibodies based on EIS measurements (Mejri et al. 2010). In another study, Tolba et al. utilized immobilized bacteriophage-encoded peptidoglycan hydrolases on Au screen-printed electrodes for detection of L. innocua in pure milk with a LOD of 105 CFU/mL (Tolba et al. 2012). These results suggest that bacteriophages are potentially attractive biorecognition elements for water safety and environmental monitoring applications that require chronic monitoring of liquids.\n\n2.2.6 Cell- and molecularly-imprinted polymers\nGiven traditional biorecognition elements used in biosensing exhibit stability concerns, such as antibodies or aptamers, as discussed in Sections 2.2.1–2.2.4, there have been efforts to create engineered molecular biorecognition elements, such as scFvs. In contrast, materials-based biorecognition elements exploit the principle of target-specific morphology for selective capture (Pan et al. 2018; Zhou et al. 2019). The most common approach in materials-based biorecognition is based on cell- and molecularly-imprinted polymers (CIPs and MIPs, respectively) (Gui et al. 2018). CIPs and MIPs have been created using various processes, including bacteria-mediated lithography, micro-contact stamping, and colloid imprints (Chen et al. 2016a; Pan et al. 2018).\nAs shown in Fig. 3b, Jafari et al. used imprinted organosilica sol-gel films of tetraethoxysilane and (3-mercaptopropyl)trimethoxysilane (MPTS) for selective detection of E. coli using an impedimetric method (Jafari et al. 2019). Similarly, Golabi et al. used imprinted poly(3-aminophenylboronic acid) films for detection of Staphylococcus epidermidis (S. epidermidis) (Golabi et al. 2017). Despite the absence of a highly selective molecular biorecognition element, CIPs and MIPs exhibit selectivity when exposed to samples that contain multiple analytes (i.e., non-target species) (Golabi et al. 2017; Jafari et al. 2019; Qi et al. 2013). MIPs and CIPs are also of interest with regard to opportunities in biosensor regeneration. Common adverse effects of regeneration on biosensors that employ molecular biorecognition elements, such as irreversible changes in structure, are less likely to affect MIPs and CIPs. However, it is generally accepted that current CIPs and MIPs exhibit lower selectivity to target species than antibodies and aptamers due to reduction of available chemical selectivity (Cheong et al. 2013; Kryscio and Peppas, 2012; Yáñez-Sedeño et al. 2017)."}
LitCovid-sentences
{"project":"LitCovid-sentences","denotations":[{"id":"T477","span":{"begin":0,"end":28},"obj":"Sentence"},{"id":"T478","span":{"begin":29,"end":154},"obj":"Sentence"},{"id":"T479","span":{"begin":155,"end":411},"obj":"Sentence"},{"id":"T480","span":{"begin":412,"end":523},"obj":"Sentence"},{"id":"T481","span":{"begin":524,"end":651},"obj":"Sentence"},{"id":"T482","span":{"begin":652,"end":749},"obj":"Sentence"},{"id":"T483","span":{"begin":750,"end":962},"obj":"Sentence"},{"id":"T484","span":{"begin":963,"end":1134},"obj":"Sentence"},{"id":"T485","span":{"begin":1135,"end":1286},"obj":"Sentence"},{"id":"T486","span":{"begin":1287,"end":1461},"obj":"Sentence"},{"id":"T487","span":{"begin":1463,"end":1503},"obj":"Sentence"},{"id":"T488","span":{"begin":1504,"end":1655},"obj":"Sentence"},{"id":"T489","span":{"begin":1656,"end":1758},"obj":"Sentence"},{"id":"T490","span":{"begin":1759,"end":1980},"obj":"Sentence"},{"id":"T491","span":{"begin":1981,"end":2134},"obj":"Sentence"},{"id":"T492","span":{"begin":2135,"end":2141},"obj":"Sentence"},{"id":"T493","span":{"begin":2142,"end":2266},"obj":"Sentence"},{"id":"T494","span":{"begin":2267,"end":2422},"obj":"Sentence"},{"id":"T495","span":{"begin":2423,"end":2547},"obj":"Sentence"},{"id":"T496","span":{"begin":2548,"end":2671},"obj":"Sentence"},{"id":"T497","span":{"begin":2672,"end":2730},"obj":"Sentence"},{"id":"T498","span":{"begin":2731,"end":2751},"obj":"Sentence"},{"id":"T499","span":{"begin":2752,"end":2758},"obj":"Sentence"},{"id":"T500","span":{"begin":2759,"end":2888},"obj":"Sentence"},{"id":"T501","span":{"begin":2889,"end":2995},"obj":"Sentence"},{"id":"T502","span":{"begin":2996,"end":3078},"obj":"Sentence"},{"id":"T503","span":{"begin":3079,"end":3185},"obj":"Sentence"},{"id":"T504","span":{"begin":3186,"end":3313},"obj":"Sentence"},{"id":"T505","span":{"begin":3314,"end":3465},"obj":"Sentence"},{"id":"T506","span":{"begin":3466,"end":3602},"obj":"Sentence"},{"id":"T507","span":{"begin":3603,"end":3671},"obj":"Sentence"},{"id":"T508","span":{"begin":3672,"end":3888},"obj":"Sentence"},{"id":"T509","span":{"begin":3889,"end":3895},"obj":"Sentence"},{"id":"T510","span":{"begin":3896,"end":4011},"obj":"Sentence"},{"id":"T511","span":{"begin":4012,"end":4143},"obj":"Sentence"},{"id":"T512","span":{"begin":4144,"end":4415},"obj":"Sentence"},{"id":"T513","span":{"begin":4416,"end":4579},"obj":"Sentence"},{"id":"T514","span":{"begin":4580,"end":4788},"obj":"Sentence"},{"id":"T515","span":{"begin":4789,"end":4795},"obj":"Sentence"},{"id":"T516","span":{"begin":4796,"end":4925},"obj":"Sentence"},{"id":"T517","span":{"begin":4926,"end":4932},"obj":"Sentence"},{"id":"T518","span":{"begin":4933,"end":5194},"obj":"Sentence"},{"id":"T519","span":{"begin":5195,"end":5201},"obj":"Sentence"},{"id":"T520","span":{"begin":5202,"end":5340},"obj":"Sentence"},{"id":"T521","span":{"begin":5341,"end":5347},"obj":"Sentence"},{"id":"T522","span":{"begin":5348,"end":5594},"obj":"Sentence"},{"id":"T523","span":{"begin":5595,"end":5866},"obj":"Sentence"},{"id":"T524","span":{"begin":5867,"end":6018},"obj":"Sentence"},{"id":"T525","span":{"begin":6019,"end":6025},"obj":"Sentence"},{"id":"T526","span":{"begin":6027,"end":6063},"obj":"Sentence"},{"id":"T527","span":{"begin":6064,"end":6251},"obj":"Sentence"},{"id":"T528","span":{"begin":6252,"end":6421},"obj":"Sentence"},{"id":"T529","span":{"begin":6422,"end":6630},"obj":"Sentence"},{"id":"T530","span":{"begin":6631,"end":6637},"obj":"Sentence"},{"id":"T531","span":{"begin":6638,"end":6751},"obj":"Sentence"},{"id":"T532","span":{"begin":6752,"end":6772},"obj":"Sentence"},{"id":"T533","span":{"begin":6773,"end":6788},"obj":"Sentence"},{"id":"T534","span":{"begin":6789,"end":6806},"obj":"Sentence"},{"id":"T535","span":{"begin":6807,"end":6814},"obj":"Sentence"},{"id":"T536","span":{"begin":6815,"end":7082},"obj":"Sentence"},{"id":"T537","span":{"begin":7083,"end":7089},"obj":"Sentence"},{"id":"T538","span":{"begin":7091,"end":7114},"obj":"Sentence"},{"id":"T539","span":{"begin":7115,"end":7219},"obj":"Sentence"},{"id":"T540","span":{"begin":7220,"end":7346},"obj":"Sentence"},{"id":"T541","span":{"begin":7347,"end":7521},"obj":"Sentence"},{"id":"T542","span":{"begin":7522,"end":7528},"obj":"Sentence"},{"id":"T543","span":{"begin":7529,"end":7756},"obj":"Sentence"},{"id":"T544","span":{"begin":7757,"end":7763},"obj":"Sentence"},{"id":"T545","span":{"begin":7765,"end":7788},"obj":"Sentence"},{"id":"T546","span":{"begin":7789,"end":7883},"obj":"Sentence"},{"id":"T547","span":{"begin":7884,"end":8052},"obj":"Sentence"},{"id":"T548","span":{"begin":8053,"end":8185},"obj":"Sentence"},{"id":"T549","span":{"begin":8186,"end":8209},"obj":"Sentence"},{"id":"T550","span":{"begin":8210,"end":8216},"obj":"Sentence"},{"id":"T551","span":{"begin":8217,"end":8413},"obj":"Sentence"},{"id":"T552","span":{"begin":8414,"end":8420},"obj":"Sentence"},{"id":"T553","span":{"begin":8421,"end":8549},"obj":"Sentence"},{"id":"T554","span":{"begin":8550,"end":8631},"obj":"Sentence"},{"id":"T555","span":{"begin":8632,"end":8638},"obj":"Sentence"},{"id":"T556","span":{"begin":8639,"end":8746},"obj":"Sentence"},{"id":"T557","span":{"begin":8747,"end":8974},"obj":"Sentence"},{"id":"T558","span":{"begin":8975,"end":8981},"obj":"Sentence"},{"id":"T559","span":{"begin":8982,"end":9133},"obj":"Sentence"},{"id":"T560","span":{"begin":9134,"end":9140},"obj":"Sentence"},{"id":"T561","span":{"begin":9141,"end":9438},"obj":"Sentence"},{"id":"T562","span":{"begin":9439,"end":9445},"obj":"Sentence"},{"id":"T563","span":{"begin":9447,"end":9460},"obj":"Sentence"},{"id":"T564","span":{"begin":9461,"end":9616},"obj":"Sentence"},{"id":"T565","span":{"begin":9617,"end":9623},"obj":"Sentence"},{"id":"T566","span":{"begin":9624,"end":9769},"obj":"Sentence"},{"id":"T567","span":{"begin":9770,"end":9867},"obj":"Sentence"},{"id":"T568","span":{"begin":9868,"end":9975},"obj":"Sentence"},{"id":"T569","span":{"begin":9976,"end":10104},"obj":"Sentence"},{"id":"T570","span":{"begin":10105,"end":10111},"obj":"Sentence"},{"id":"T571","span":{"begin":10112,"end":10236},"obj":"Sentence"},{"id":"T572","span":{"begin":10237,"end":10243},"obj":"Sentence"},{"id":"T573","span":{"begin":10244,"end":10489},"obj":"Sentence"},{"id":"T574","span":{"begin":10490,"end":10496},"obj":"Sentence"},{"id":"T575","span":{"begin":10497,"end":10707},"obj":"Sentence"},{"id":"T576","span":{"begin":10708,"end":10714},"obj":"Sentence"},{"id":"T577","span":{"begin":10715,"end":10910},"obj":"Sentence"},{"id":"T578","span":{"begin":10912,"end":10959},"obj":"Sentence"},{"id":"T579","span":{"begin":10960,"end":11352},"obj":"Sentence"},{"id":"T580","span":{"begin":11353,"end":11370},"obj":"Sentence"},{"id":"T581","span":{"begin":11371,"end":11377},"obj":"Sentence"},{"id":"T582","span":{"begin":11378,"end":11531},"obj":"Sentence"},{"id":"T583","span":{"begin":11532,"end":11538},"obj":"Sentence"},{"id":"T584","span":{"begin":11539,"end":11694},"obj":"Sentence"},{"id":"T585","span":{"begin":11695,"end":11712},"obj":"Sentence"},{"id":"T586","span":{"begin":11713,"end":11719},"obj":"Sentence"},{"id":"T587","span":{"begin":11720,"end":11942},"obj":"Sentence"},{"id":"T588","span":{"begin":11943,"end":11949},"obj":"Sentence"},{"id":"T589","span":{"begin":11950,"end":12103},"obj":"Sentence"},{"id":"T590","span":{"begin":12104,"end":12110},"obj":"Sentence"},{"id":"T591","span":{"begin":12111,"end":12317},"obj":"Sentence"},{"id":"T592","span":{"begin":12318,"end":12337},"obj":"Sentence"},{"id":"T593","span":{"begin":12338,"end":12353},"obj":"Sentence"},{"id":"T594","span":{"begin":12354,"end":12360},"obj":"Sentence"},{"id":"T595","span":{"begin":12361,"end":12451},"obj":"Sentence"},{"id":"T596","span":{"begin":12452,"end":12635},"obj":"Sentence"},{"id":"T597","span":{"begin":12636,"end":12835},"obj":"Sentence"},{"id":"T598","span":{"begin":12836,"end":12887},"obj":"Sentence"},{"id":"T599","span":{"begin":12888,"end":12894},"obj":"Sentence"}],"namespaces":[{"prefix":"_base","uri":"http://pubannotation.org/ontology/tao.owl#"}],"text":"2.2 Biorecognition elements\nThe previous section discussed the transduction elements associated with pathogen detection using electrochemical biosensors. Given a biosensor is a device composed of integrated transducer and biorecognition elements, we next discuss the biorecognition elements used for selective detection of pathogens and corresponding immobilization techniques for their coupling to electrodes.\nBiorecognition elements for electrochemical biosensors can be defined as (1) biocatalytic or (2) biocomplexing. In the case of biocatalytic biorecognition elements, the biosensor response is based on a reaction catalyzed by macromolecules. Enzymes, whole cells, and tissues are the most commonly used biocatalytic biorecognition element. While enzyzmes provide biorecognition elements in various chemical sensing applications, they are often used as labels for pathogen detection applications and most commonly introduced via secondary binding steps. In the case of biocomplexing biorecognition elements, the biosensor response is based on the interaction of analytes with macromolecules or organized molecular assemblies. As shown in Table 1, Table 2, antibodies, peptides, and phages are the most commonly used biocomplexing biorecognition elements for pathogen detection. In addition to biomacromolecules, imprinted polymers have also been examined as biocomplexing biorecognition elements for pathogen detection using electrochemical biosensors.\n\n2.2.1 Antibodies and antibody fragments\nAntibodies and antibody fragments are among the most commonly utilized biorecognition elements for pathogen detection using electrochemical biosensors. Biosensors employing antibody-based biorecognition elements are commonly referred to as immunosensors. Given antibodies exhibit high selectivity and binding affinity for target species and can be generated for a wide range of infectious agents, antibodies are the gold-standard biorecognition element for pathogen detection. Antibodies contain recognition sites that selectively bind to antigens through a specific region of the antigen, referred to as an epitope (Patris et al. 2016). Antibodies can be labeled with fluorescent or enzymatic tags, which leads to the designation of the approach as label-based. While label-based approaches present measurement constraints associated with the use of additional reagents and processing steps (Cooper, 2009; Sang et al. 2016), antibody labeling may also alter the binding affinity to the antigen, which could affect the biosensor's selectivity. A detailed discussion of label-based biosensing approaches for pathogen detection has been reported elsewhere (Ahmed et al. 2014; Alahi and Mukhopadhyay, 2017; Bozal-Palabiyik et al. 2018; Leonard et al. 2003). A list of recent label-based approaches for pathogen detection using electrochemical biosensors, however, is provided in Table 2.\nWhile both monoclonal and polyclonal antibodies enable the selective detection of pathogens (Patris et al. 2016), they vary in terms of production method, selectivity, and binding affinity. Monoclonal antibodies are produced by hybridoma technology (Birch and Racher, 2006; James and Bell, 1987). Thus, monoclonal antibodies are highly selective and bind to a single epitope, making them less vulnerable to cross-reactivity. While monoclonal antibodies tend to have a higher degree of selectivity, they are more expensive and take longer to develop than polyclonal antibodies. Polyclonal antibodies are produced by separation of immunoglobulin proteins from the blood of an infected host (Birch and Racher, 2006). Polyclonal antibodies target different epitopes on a single antigen. While polyclonal antibodies exhibit increased variability between batches, they are relatively less expensive to produce than monoclonal antibodies and facilitate robust measurements in various settings (Byrne et al. 2009). Drawbacks to antibody use include high cost and stability challenges, such as the need for low-temperature storage. As shown in Table 1, Table 2, both monoclonal and polyclonal antibodies are used as biorecognition elements for pathogen detection. For assays involving secondary binding steps, monoclonal antibodies typically serve as the primary biorecognition element and are immobilized on the electrode, while polyclonal antibodies serve as the secondary biorecognition element and often facilitate target labeling. For assays that do not require secondary binding steps, polyclonal antibodies are also commonly used as immobilized biorecognition elements for pathogen detection. For example, Pandey et al. immobilized monoclonal anti-E. coli on a composite nanostructured electrode to detect E. coli across a wide dynamic range of 10 to 108 CFU/mL with a LOD of 3.8 CFU/mL (Pandey et al. 2017). Wu et al. used polyclonal anti-E. coli for detection of E. coli via amperometry that exhibited a LOD of 5 × 103 CFU/mL (Wu et al. 2016). Lin et al. used monoclonal antibodies for detection of avian influenza virus H5N1 in chicken swabs across a dynamic range of 2- 1 to 24 hemagglutination units (HAU)/50 μL using EIS and the ferri/ferrocyanide (Fe(CN)6 3 - /4-) couple as a redox probe (Lin et al. 2015). Luka et al. detected Cryptosporidium parvum (C. parvum) with a LOD of 40 cells/mm2 via capacitive sensing and Fe(CN)6 3 - /4- (Luka et al. 2019).\nAntibody fragments, such as single-chain variable fragments (scFvs), offer selectivity similar to antibodies, but they have the advantage of achieving relatively higher packing densities on electrode surfaces due to their relatively smaller size. For example, half-antibody fragments have been shown to improve biosensor sensitivity without the loss of selectivity, which warrants further investigation of reduced antibodies as biorecognition elements for pathogen detection applications (Sharma and Mutharasan, 2013). In addition to scFvs, Fabs, re-engineered IgGs, and dimers can also potentially be used as biorecognition elements for pathogen detection (Byrne et al. 2009).\n\n2.2.2 Carbohydrate-binding proteins\nCarbohydrate-binding proteins, such as lectins, also provide selective biorecognition elements for pathogen detection based on their ability to selectively bind ligands on target species. Peptide-based biorecognition elements are relatively low-cost, can be produced with high yield automated synthesis processes, and are modifiable (Pavan and Berti, 2012). For example, lectins have been investigated as biorecognition elements for pathogen detection through their ability to selectively bind glycosylated proteins on the surfaces of viruses and cells (Reina et al. 2008). Concanavalin A (ConA) lectin has been extensively investigated for E. coli detection (see Table 1) (Jantra et al. 2011; Saucedo et al. 2019; Xi et al. 2011; Yang et al. 2016b). While not yet widely investigated for pathogen detection using electrochemical biosensors, Etayash et al. recently showed that oligopeptides also provide attractive biorecognition elements for real-time biosensor-based detection of breast cancer cells (Etayash et al. 2015).\n\n2.2.3 Oligosaccharides\nTrisaccharides are carbohydrates that can selectively bind carbohydrate-specific receptors on pathogens. Thus, trisaccharide ligands have been used as biorecognition elements for pathogen detection using electrochemical biosensors. For example, Hai et al. used a hybrid E-QCM biosensor coated with hemagglutinin-specific trisaccharide ligands for the detection of human influenza A virus (H1N1) (Hai et al. 2017). The use of carbohydrates as biorecognition elements is limited in part due to the weak affinity of carbohydrate-protein interactions and low selectivity, which are currently mitigated through secondary interactions (Zeng et al. 2012).\n\n2.2.4 Oligonucleotides\nSingle-stranded DNA (ssDNA) is a useful biorecognition element for the detection of pathogens. While ssDNA is commonly used as a biorecognition element for DNA-based assays, ssDNA aptamers are commonly used for pathogen detection using electrochemical biosensors. Aptamers are single-stranded oligonucleotides capable of binding various molecules with high affinity and selectivity (Lakhin et al. 2013; Reverdatto et al. 2015). Aptamers are isolated from a large random sequence pool through a selection process that utilizes systematic evolution of ligands by exponential enrichment, also known as SELEX (Stoltenburg et al. 2007). Suitable binding sequences can be isolated from a large random oligonucleotide sequence pool and subsequently amplified for use. Thus, aptamers can exhibit high selectivity to target species (Stoltenburg et al. 2007). Aptamers can also be produced at a lower cost than alternative biorecognition elements, such as antibodies. Giamberardino et al. used SELEX to discover an aptamer for norovirus detection, which showed a million-fold higher binding affinity for the target than a random DNA strand that served as a negative control (Giamberardino et al. 2013). Iqbal et al. performed 10 rounds of SELEX to discover 14 aptamer clones with high affinities for C. parvum for detection in fruit samples (Iqbal et al. 2015). However, the use of aptamers as biorecognition elements has not yet replaced traditional biorecognition elements, such as antibodies, because of several challenges, such as aptamer stability, degradation, cross-reactivity, and reproducibility using alternative processing approaches (Lakhin et al. 2013).\n\n2.2.5 Phages\nPhages, also referred to as bacteriophages, are viruses that infect and replicate in bacteria through selective binding via tail-spike proteins (Haq et al. 2012). Thus, they have been examined as biorecognition elements for pathogen detection using electrochemical biosensors (Kutter and Sulakvelidze, 2004). Bacteriophages exhibit varying morphologies and are thus classified by selectivity and structure. A variety of bacteriophage-based electrochemical biosensors for pathogen detection can be found in Table 1. For example, Shabani et al. used E. coli-specific T4 bacteriophages for selective impedimetric detection studies (Shabani et al. 2008). Mejri et al. compared the use of bacteriophages to antibodies as biorecognition elements for E. coli detection (Mejri et al. 2010). In that study, they found that bacteriophages improved the water stability of the biosensor and increased the sensitivity by approximately a factor of four relative to the response obtained with antibodies based on EIS measurements (Mejri et al. 2010). In another study, Tolba et al. utilized immobilized bacteriophage-encoded peptidoglycan hydrolases on Au screen-printed electrodes for detection of L. innocua in pure milk with a LOD of 105 CFU/mL (Tolba et al. 2012). These results suggest that bacteriophages are potentially attractive biorecognition elements for water safety and environmental monitoring applications that require chronic monitoring of liquids.\n\n2.2.6 Cell- and molecularly-imprinted polymers\nGiven traditional biorecognition elements used in biosensing exhibit stability concerns, such as antibodies or aptamers, as discussed in Sections 2.2.1–2.2.4, there have been efforts to create engineered molecular biorecognition elements, such as scFvs. In contrast, materials-based biorecognition elements exploit the principle of target-specific morphology for selective capture (Pan et al. 2018; Zhou et al. 2019). The most common approach in materials-based biorecognition is based on cell- and molecularly-imprinted polymers (CIPs and MIPs, respectively) (Gui et al. 2018). CIPs and MIPs have been created using various processes, including bacteria-mediated lithography, micro-contact stamping, and colloid imprints (Chen et al. 2016a; Pan et al. 2018).\nAs shown in Fig. 3b, Jafari et al. used imprinted organosilica sol-gel films of tetraethoxysilane and (3-mercaptopropyl)trimethoxysilane (MPTS) for selective detection of E. coli using an impedimetric method (Jafari et al. 2019). Similarly, Golabi et al. used imprinted poly(3-aminophenylboronic acid) films for detection of Staphylococcus epidermidis (S. epidermidis) (Golabi et al. 2017). Despite the absence of a highly selective molecular biorecognition element, CIPs and MIPs exhibit selectivity when exposed to samples that contain multiple analytes (i.e., non-target species) (Golabi et al. 2017; Jafari et al. 2019; Qi et al. 2013). MIPs and CIPs are also of interest with regard to opportunities in biosensor regeneration. Common adverse effects of regeneration on biosensors that employ molecular biorecognition elements, such as irreversible changes in structure, are less likely to affect MIPs and CIPs. However, it is generally accepted that current CIPs and MIPs exhibit lower selectivity to target species than antibodies and aptamers due to reduction of available chemical selectivity (Cheong et al. 2013; Kryscio and Peppas, 2012; Yáñez-Sedeño et al. 2017)."}
2_test
{"project":"2_test","denotations":[{"id":"32364936-25608959-7713070","span":{"begin":2423,"end":2427},"obj":"25608959"},{"id":"32364936-24982325-7713071","span":{"begin":2672,"end":2676},"obj":"24982325"},{"id":"32364936-16822577-7713072","span":{"begin":3157,"end":3161},"obj":"16822577"},{"id":"32364936-3298441-7713073","span":{"begin":3179,"end":3183},"obj":"3298441"},{"id":"32364936-16822577-7713074","span":{"begin":3596,"end":3600},"obj":"16822577"},{"id":"32364936-22408533-7713075","span":{"begin":3889,"end":3893},"obj":"22408533"},{"id":"32364936-25263315-7713076","span":{"begin":5195,"end":5199},"obj":"25263315"},{"id":"32364936-23356211-7713077","span":{"begin":5860,"end":5864},"obj":"23356211"},{"id":"32364936-22408533-7713078","span":{"begin":6019,"end":6023},"obj":"22408533"},{"id":"32364936-22169951-7713079","span":{"begin":6415,"end":6419},"obj":"22169951"},{"id":"32364936-18633532-7713080","span":{"begin":6631,"end":6635},"obj":"18633532"},{"id":"32364936-26434765-7713081","span":{"begin":7083,"end":7087},"obj":"26434765"},{"id":"32364936-22200920-7713082","span":{"begin":7757,"end":7761},"obj":"22200920"},{"id":"32364936-24455181-7713083","span":{"begin":8186,"end":8190},"obj":"24455181"},{"id":"32364936-25866267-7713084","span":{"begin":8210,"end":8214},"obj":"25866267"},{"id":"32364936-17627883-7713085","span":{"begin":8414,"end":8418},"obj":"17627883"},{"id":"32364936-17627883-7713086","span":{"begin":8632,"end":8636},"obj":"17627883"},{"id":"32364936-24455181-7713087","span":{"begin":9439,"end":9443},"obj":"24455181"},{"id":"32364936-19072262-7713088","span":{"begin":10105,"end":10109},"obj":"19072262"},{"id":"32364936-20673624-7713089","span":{"begin":10237,"end":10241},"obj":"20673624"},{"id":"32364936-20673624-7713090","span":{"begin":10490,"end":10494},"obj":"20673624"},{"id":"32364936-23085745-7713091","span":{"begin":10708,"end":10712},"obj":"23085745"},{"id":"32364936-29876564-7713092","span":{"begin":11353,"end":11357},"obj":"29876564"},{"id":"32364936-28863325-7713093","span":{"begin":11532,"end":11536},"obj":"28863325"},{"id":"32364936-29876564-7713095","span":{"begin":11713,"end":11717},"obj":"29876564"},{"id":"32364936-27751788-7713096","span":{"begin":12104,"end":12108},"obj":"27751788"},{"id":"32364936-27751788-7713097","span":{"begin":12318,"end":12322},"obj":"27751788"},{"id":"32364936-22917919-7713098","span":{"begin":12354,"end":12358},"obj":"22917919"},{"id":"32364936-22100344-7713099","span":{"begin":12862,"end":12866},"obj":"22100344"},{"id":"32364936-28193351-7713100","span":{"begin":12888,"end":12892},"obj":"28193351"}],"text":"2.2 Biorecognition elements\nThe previous section discussed the transduction elements associated with pathogen detection using electrochemical biosensors. Given a biosensor is a device composed of integrated transducer and biorecognition elements, we next discuss the biorecognition elements used for selective detection of pathogens and corresponding immobilization techniques for their coupling to electrodes.\nBiorecognition elements for electrochemical biosensors can be defined as (1) biocatalytic or (2) biocomplexing. In the case of biocatalytic biorecognition elements, the biosensor response is based on a reaction catalyzed by macromolecules. Enzymes, whole cells, and tissues are the most commonly used biocatalytic biorecognition element. While enzyzmes provide biorecognition elements in various chemical sensing applications, they are often used as labels for pathogen detection applications and most commonly introduced via secondary binding steps. In the case of biocomplexing biorecognition elements, the biosensor response is based on the interaction of analytes with macromolecules or organized molecular assemblies. As shown in Table 1, Table 2, antibodies, peptides, and phages are the most commonly used biocomplexing biorecognition elements for pathogen detection. In addition to biomacromolecules, imprinted polymers have also been examined as biocomplexing biorecognition elements for pathogen detection using electrochemical biosensors.\n\n2.2.1 Antibodies and antibody fragments\nAntibodies and antibody fragments are among the most commonly utilized biorecognition elements for pathogen detection using electrochemical biosensors. Biosensors employing antibody-based biorecognition elements are commonly referred to as immunosensors. Given antibodies exhibit high selectivity and binding affinity for target species and can be generated for a wide range of infectious agents, antibodies are the gold-standard biorecognition element for pathogen detection. Antibodies contain recognition sites that selectively bind to antigens through a specific region of the antigen, referred to as an epitope (Patris et al. 2016). Antibodies can be labeled with fluorescent or enzymatic tags, which leads to the designation of the approach as label-based. While label-based approaches present measurement constraints associated with the use of additional reagents and processing steps (Cooper, 2009; Sang et al. 2016), antibody labeling may also alter the binding affinity to the antigen, which could affect the biosensor's selectivity. A detailed discussion of label-based biosensing approaches for pathogen detection has been reported elsewhere (Ahmed et al. 2014; Alahi and Mukhopadhyay, 2017; Bozal-Palabiyik et al. 2018; Leonard et al. 2003). A list of recent label-based approaches for pathogen detection using electrochemical biosensors, however, is provided in Table 2.\nWhile both monoclonal and polyclonal antibodies enable the selective detection of pathogens (Patris et al. 2016), they vary in terms of production method, selectivity, and binding affinity. Monoclonal antibodies are produced by hybridoma technology (Birch and Racher, 2006; James and Bell, 1987). Thus, monoclonal antibodies are highly selective and bind to a single epitope, making them less vulnerable to cross-reactivity. While monoclonal antibodies tend to have a higher degree of selectivity, they are more expensive and take longer to develop than polyclonal antibodies. Polyclonal antibodies are produced by separation of immunoglobulin proteins from the blood of an infected host (Birch and Racher, 2006). Polyclonal antibodies target different epitopes on a single antigen. While polyclonal antibodies exhibit increased variability between batches, they are relatively less expensive to produce than monoclonal antibodies and facilitate robust measurements in various settings (Byrne et al. 2009). Drawbacks to antibody use include high cost and stability challenges, such as the need for low-temperature storage. As shown in Table 1, Table 2, both monoclonal and polyclonal antibodies are used as biorecognition elements for pathogen detection. For assays involving secondary binding steps, monoclonal antibodies typically serve as the primary biorecognition element and are immobilized on the electrode, while polyclonal antibodies serve as the secondary biorecognition element and often facilitate target labeling. For assays that do not require secondary binding steps, polyclonal antibodies are also commonly used as immobilized biorecognition elements for pathogen detection. For example, Pandey et al. immobilized monoclonal anti-E. coli on a composite nanostructured electrode to detect E. coli across a wide dynamic range of 10 to 108 CFU/mL with a LOD of 3.8 CFU/mL (Pandey et al. 2017). Wu et al. used polyclonal anti-E. coli for detection of E. coli via amperometry that exhibited a LOD of 5 × 103 CFU/mL (Wu et al. 2016). Lin et al. used monoclonal antibodies for detection of avian influenza virus H5N1 in chicken swabs across a dynamic range of 2- 1 to 24 hemagglutination units (HAU)/50 μL using EIS and the ferri/ferrocyanide (Fe(CN)6 3 - /4-) couple as a redox probe (Lin et al. 2015). Luka et al. detected Cryptosporidium parvum (C. parvum) with a LOD of 40 cells/mm2 via capacitive sensing and Fe(CN)6 3 - /4- (Luka et al. 2019).\nAntibody fragments, such as single-chain variable fragments (scFvs), offer selectivity similar to antibodies, but they have the advantage of achieving relatively higher packing densities on electrode surfaces due to their relatively smaller size. For example, half-antibody fragments have been shown to improve biosensor sensitivity without the loss of selectivity, which warrants further investigation of reduced antibodies as biorecognition elements for pathogen detection applications (Sharma and Mutharasan, 2013). In addition to scFvs, Fabs, re-engineered IgGs, and dimers can also potentially be used as biorecognition elements for pathogen detection (Byrne et al. 2009).\n\n2.2.2 Carbohydrate-binding proteins\nCarbohydrate-binding proteins, such as lectins, also provide selective biorecognition elements for pathogen detection based on their ability to selectively bind ligands on target species. Peptide-based biorecognition elements are relatively low-cost, can be produced with high yield automated synthesis processes, and are modifiable (Pavan and Berti, 2012). For example, lectins have been investigated as biorecognition elements for pathogen detection through their ability to selectively bind glycosylated proteins on the surfaces of viruses and cells (Reina et al. 2008). Concanavalin A (ConA) lectin has been extensively investigated for E. coli detection (see Table 1) (Jantra et al. 2011; Saucedo et al. 2019; Xi et al. 2011; Yang et al. 2016b). While not yet widely investigated for pathogen detection using electrochemical biosensors, Etayash et al. recently showed that oligopeptides also provide attractive biorecognition elements for real-time biosensor-based detection of breast cancer cells (Etayash et al. 2015).\n\n2.2.3 Oligosaccharides\nTrisaccharides are carbohydrates that can selectively bind carbohydrate-specific receptors on pathogens. Thus, trisaccharide ligands have been used as biorecognition elements for pathogen detection using electrochemical biosensors. For example, Hai et al. used a hybrid E-QCM biosensor coated with hemagglutinin-specific trisaccharide ligands for the detection of human influenza A virus (H1N1) (Hai et al. 2017). The use of carbohydrates as biorecognition elements is limited in part due to the weak affinity of carbohydrate-protein interactions and low selectivity, which are currently mitigated through secondary interactions (Zeng et al. 2012).\n\n2.2.4 Oligonucleotides\nSingle-stranded DNA (ssDNA) is a useful biorecognition element for the detection of pathogens. While ssDNA is commonly used as a biorecognition element for DNA-based assays, ssDNA aptamers are commonly used for pathogen detection using electrochemical biosensors. Aptamers are single-stranded oligonucleotides capable of binding various molecules with high affinity and selectivity (Lakhin et al. 2013; Reverdatto et al. 2015). Aptamers are isolated from a large random sequence pool through a selection process that utilizes systematic evolution of ligands by exponential enrichment, also known as SELEX (Stoltenburg et al. 2007). Suitable binding sequences can be isolated from a large random oligonucleotide sequence pool and subsequently amplified for use. Thus, aptamers can exhibit high selectivity to target species (Stoltenburg et al. 2007). Aptamers can also be produced at a lower cost than alternative biorecognition elements, such as antibodies. Giamberardino et al. used SELEX to discover an aptamer for norovirus detection, which showed a million-fold higher binding affinity for the target than a random DNA strand that served as a negative control (Giamberardino et al. 2013). Iqbal et al. performed 10 rounds of SELEX to discover 14 aptamer clones with high affinities for C. parvum for detection in fruit samples (Iqbal et al. 2015). However, the use of aptamers as biorecognition elements has not yet replaced traditional biorecognition elements, such as antibodies, because of several challenges, such as aptamer stability, degradation, cross-reactivity, and reproducibility using alternative processing approaches (Lakhin et al. 2013).\n\n2.2.5 Phages\nPhages, also referred to as bacteriophages, are viruses that infect and replicate in bacteria through selective binding via tail-spike proteins (Haq et al. 2012). Thus, they have been examined as biorecognition elements for pathogen detection using electrochemical biosensors (Kutter and Sulakvelidze, 2004). Bacteriophages exhibit varying morphologies and are thus classified by selectivity and structure. A variety of bacteriophage-based electrochemical biosensors for pathogen detection can be found in Table 1. For example, Shabani et al. used E. coli-specific T4 bacteriophages for selective impedimetric detection studies (Shabani et al. 2008). Mejri et al. compared the use of bacteriophages to antibodies as biorecognition elements for E. coli detection (Mejri et al. 2010). In that study, they found that bacteriophages improved the water stability of the biosensor and increased the sensitivity by approximately a factor of four relative to the response obtained with antibodies based on EIS measurements (Mejri et al. 2010). In another study, Tolba et al. utilized immobilized bacteriophage-encoded peptidoglycan hydrolases on Au screen-printed electrodes for detection of L. innocua in pure milk with a LOD of 105 CFU/mL (Tolba et al. 2012). These results suggest that bacteriophages are potentially attractive biorecognition elements for water safety and environmental monitoring applications that require chronic monitoring of liquids.\n\n2.2.6 Cell- and molecularly-imprinted polymers\nGiven traditional biorecognition elements used in biosensing exhibit stability concerns, such as antibodies or aptamers, as discussed in Sections 2.2.1–2.2.4, there have been efforts to create engineered molecular biorecognition elements, such as scFvs. In contrast, materials-based biorecognition elements exploit the principle of target-specific morphology for selective capture (Pan et al. 2018; Zhou et al. 2019). The most common approach in materials-based biorecognition is based on cell- and molecularly-imprinted polymers (CIPs and MIPs, respectively) (Gui et al. 2018). CIPs and MIPs have been created using various processes, including bacteria-mediated lithography, micro-contact stamping, and colloid imprints (Chen et al. 2016a; Pan et al. 2018).\nAs shown in Fig. 3b, Jafari et al. used imprinted organosilica sol-gel films of tetraethoxysilane and (3-mercaptopropyl)trimethoxysilane (MPTS) for selective detection of E. coli using an impedimetric method (Jafari et al. 2019). Similarly, Golabi et al. used imprinted poly(3-aminophenylboronic acid) films for detection of Staphylococcus epidermidis (S. epidermidis) (Golabi et al. 2017). Despite the absence of a highly selective molecular biorecognition element, CIPs and MIPs exhibit selectivity when exposed to samples that contain multiple analytes (i.e., non-target species) (Golabi et al. 2017; Jafari et al. 2019; Qi et al. 2013). MIPs and CIPs are also of interest with regard to opportunities in biosensor regeneration. Common adverse effects of regeneration on biosensors that employ molecular biorecognition elements, such as irreversible changes in structure, are less likely to affect MIPs and CIPs. However, it is generally accepted that current CIPs and MIPs exhibit lower selectivity to target species than antibodies and aptamers due to reduction of available chemical selectivity (Cheong et al. 2013; Kryscio and Peppas, 2012; Yáñez-Sedeño et al. 2017)."}