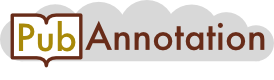
PMC:7152911 / 46421-54230
Annnotations
LitCovid-PubTator
{"project":"LitCovid-PubTator","denotations":[{"id":"1289","span":{"begin":1787,"end":1809},"obj":"Species"},{"id":"1290","span":{"begin":1811,"end":1827},"obj":"Species"},{"id":"1291","span":{"begin":1975,"end":1998},"obj":"Species"},{"id":"1292","span":{"begin":1698,"end":1702},"obj":"Chemical"},{"id":"1293","span":{"begin":1872,"end":1879},"obj":"Chemical"},{"id":"1297","span":{"begin":2732,"end":2753},"obj":"Species"},{"id":"1298","span":{"begin":2053,"end":2060},"obj":"Chemical"},{"id":"1299","span":{"begin":2252,"end":2259},"obj":"Chemical"},{"id":"1301","span":{"begin":3332,"end":3339},"obj":"Chemical"},{"id":"1306","span":{"begin":5339,"end":5347},"obj":"Gene"},{"id":"1307","span":{"begin":5272,"end":5279},"obj":"Species"},{"id":"1308","span":{"begin":5114,"end":5116},"obj":"Chemical"},{"id":"1309","span":{"begin":5208,"end":5211},"obj":"Disease"},{"id":"1317","span":{"begin":5764,"end":5772},"obj":"Gene"},{"id":"1318","span":{"begin":5594,"end":5608},"obj":"Species"},{"id":"1319","span":{"begin":5708,"end":5715},"obj":"Species"},{"id":"1320","span":{"begin":5720,"end":5731},"obj":"Species"},{"id":"1321","span":{"begin":5898,"end":5905},"obj":"Species"},{"id":"1322","span":{"begin":5910,"end":5919},"obj":"Species"},{"id":"1323","span":{"begin":5540,"end":5542},"obj":"Chemical"},{"id":"1328","span":{"begin":4939,"end":4966},"obj":"Species"},{"id":"1329","span":{"begin":4446,"end":4448},"obj":"Chemical"},{"id":"1330","span":{"begin":4788,"end":4794},"obj":"Chemical"},{"id":"1331","span":{"begin":4811,"end":4817},"obj":"Chemical"},{"id":"1337","span":{"begin":6761,"end":6776},"obj":"Species"},{"id":"1338","span":{"begin":6296,"end":6298},"obj":"Chemical"},{"id":"1339","span":{"begin":6343,"end":6362},"obj":"Chemical"},{"id":"1340","span":{"begin":6711,"end":6718},"obj":"Chemical"},{"id":"1341","span":{"begin":6726,"end":6728},"obj":"Chemical"}],"attributes":[{"id":"A1289","pred":"tao:has_database_id","subj":"1289","obj":"Tax:1639"},{"id":"A1290","pred":"tao:has_database_id","subj":"1290","obj":"Tax:1639"},{"id":"A1291","pred":"tao:has_database_id","subj":"1291","obj":"Tax:11320"},{"id":"A1293","pred":"tao:has_database_id","subj":"1293","obj":"MESH:D012825"},{"id":"A1297","pred":"tao:has_database_id","subj":"1297","obj":"Tax:12305"},{"id":"A1298","pred":"tao:has_database_id","subj":"1298","obj":"MESH:D011108"},{"id":"A1299","pred":"tao:has_database_id","subj":"1299","obj":"MESH:D011108"},{"id":"A1301","pred":"tao:has_database_id","subj":"1301","obj":"MESH:D012825"},{"id":"A1306","pred":"tao:has_database_id","subj":"1306","obj":"Gene:2960"},{"id":"A1307","pred":"tao:has_database_id","subj":"1307","obj":"Tax:562"},{"id":"A1308","pred":"tao:has_database_id","subj":"1308","obj":"MESH:D006046"},{"id":"A1309","pred":"tao:has_database_id","subj":"1309","obj":"MESH:D010259"},{"id":"A1317","pred":"tao:has_database_id","subj":"1317","obj":"Gene:2960"},{"id":"A1318","pred":"tao:has_database_id","subj":"1318","obj":"Tax:90371"},{"id":"A1319","pred":"tao:has_database_id","subj":"1319","obj":"Tax:562"},{"id":"A1320","pred":"tao:has_database_id","subj":"1320","obj":"Tax:666"},{"id":"A1321","pred":"tao:has_database_id","subj":"1321","obj":"Tax:562"},{"id":"A1322","pred":"tao:has_database_id","subj":"1322","obj":"Tax:1280"},{"id":"A1323","pred":"tao:has_database_id","subj":"1323","obj":"MESH:D006046"},{"id":"A1328","pred":"tao:has_database_id","subj":"1328","obj":"Tax:11072"},{"id":"A1329","pred":"tao:has_database_id","subj":"1329","obj":"MESH:D006046"},{"id":"A1330","pred":"tao:has_database_id","subj":"1330","obj":"MESH:D002244"},{"id":"A1331","pred":"tao:has_database_id","subj":"1331","obj":"MESH:D002244"},{"id":"A1337","pred":"tao:has_database_id","subj":"1337","obj":"Tax:11082"},{"id":"A1338","pred":"tao:has_database_id","subj":"1338","obj":"MESH:D006046"},{"id":"A1340","pred":"tao:has_database_id","subj":"1340","obj":"MESH:D000537"},{"id":"A1341","pred":"tao:has_database_id","subj":"1341","obj":"MESH:D010984"}],"namespaces":[{"prefix":"Tax","uri":"https://www.ncbi.nlm.nih.gov/taxonomy/"},{"prefix":"MESH","uri":"https://id.nlm.nih.gov/mesh/"},{"prefix":"Gene","uri":"https://www.ncbi.nlm.nih.gov/gene/"},{"prefix":"CVCL","uri":"https://web.expasy.org/cellosaurus/CVCL_"}],"text":"2.1.5 Electrode nanostructuring\nTransducers with physical dimensions comparable to the target species have been widely investigated as a means of creating sensitive biosensors (Gupta et al. 2004; Pumera et al. 2007; Singh et al. 2010; Wei et al. 2009). Thus, electrodes ranging from micrometers to nanometers have been investigated for pathogen detection. While nanoscale planar electrodes are among the most commonly examined for pathogen detection (Hong et al. 2015; Peh and Li, 2013), the fabrication of nanoscale structures of conducting and semiconducting materials using a wide range of bottom-up and top-down nanomanufacturing processes, such as nanowires, has led to the investigation of nanostructured electrodes for pathogen detection (Patolsky and Lieber, 2005). Nanostructuring can be performed simultaneously with bottom-up electrode fabrication processes or as a post-processing step with top-down electrode fabrication processes.\nNanowire-based electrodes have been fabricated using a variety of engineering materials using both bottom-up and top-down nanomanufacturing processes (Hu et al. 1999; Yogeswaran and Chen, 2008). A detailed review of nanomanufacturing processes for nanowire fabrication can be found elsewhere (Hu et al. 1999). Nanowires can exhibit circular, hexagonal, and even triangular cross-sections. The nanowire aspect ratio, defined as the ratio of the length to width, often ranges from 1 to greater than 10 (Hu et al. 1999; Vaseashta and Dimova-Malinovska, 2005; Wanekaya et al. 2006).\nAs shown in Table 1, metallic and ceramic microwire- and nanowire-based electrodes have been examined for pathogen detection. For example, Wang et al. used nanowire-bundled TiO2 electrodes synthesized using a bottom-up wet chemistry process for the detection of Listeria monocytogenes (L. monocytogenes) (Wang et al. 2008). Shen et al. fabricated silicon nanowire-based electrodes using a chemical vapor deposition process for the rapid detection of human influenza A virus in an array-based format (Shen et al. 2012).\nAlthough polymer nanowires have been relatively more applied to the detection of non-pathogenic species (Travas-Sejdic et al. 2014), there appears to be potential for their application to pathogen detection. Polymer nanowires are also synthesized via bottom-up and top-down nanomanufacturing processes, including hard template methods, soft template methods, or physical approaches, but efficient, large-scale synthesis remains a challenge (Xia et al. 2010). A comprehensive summary of studies using micro- and nano-wire electrodes for pathogen detection is shown in Table 1. For example, Chartuprayoon et al. used Au microelectrode arrays modified with polypyrrole nanoribbons to detect cucumber mosaic virus (Chartuprayoon et al. 2013).\nThe topographical modification of electrode surfaces with micro- and nano-structured features beyond wire-like structures has also been investigated for pathogen detection. Electrode nanostructuring increases the electrode surface area without significantly increasing the electrode volume, thereby increasing the ratio of electrode surface area to fluid volume analyzed (Soleymani et al. 2009). Topographical modification of electrodes can also affect their mechanical and electrical properties. For example, electrochemical deposition of PEDOT on silicon electrodes reduces the electrode electrical impedance across a wide frequency range, which offers measurement advantages for neural monitoring and recording applications (Ludwig et al. 2006).\nElectrode nanostructuring for pathogen detection beyond the fabrication of nanowire-based electrodes has been accomplished primarily using bottom-up wet chemistry approaches and electrochemical methods. Among the wet chemistry approaches for electrode nanostructuring (Eftekhari et al. 2008), nanostructured electrodes are often fabricated by the deposition or coupling of nanoparticles to planar electrodes. For example, AuNPs are commonly deposited on planar electrodes to provide a nanostructured surface for biorecognition element immobilization. In such studies, the particles are bound to the planar electrode via physical adsorption processes (Attar et al. 2016) or chemical methods (Wang et al. 2013). In addition to AuNPs, CNTs have also been extensively investigated as potentially useful nanomaterials for electrode nanostructuring (see Table 1).\nDe Luna et al. found that high-curvature nanostructured Au microelectrodes exhibited a reduced extent of biorecognition element aggregation relative to that found on planar electrodes in DNA sensing studies using a combination of experimental studies and molecular dynamics simulations (see Fig. 3 a) (De Luna et al. 2017; Mahshid et al. 2016). A study by Chin et al. found that nanostructuring of carbon electrodes with carbon nanoparticles enhanced the electron transfer kinetics and current intensity of the electrode by 63% for the detection of Japanese encephalitis virus (Chin et al. 2017).\nFig. 3 Emerging transduction approaches associated with electrochemical biosensors for pathogen detection. a) A nanostructured Au microelectrode array with high curvature (De Luna et al. 2017). b) Cell-imprinted polymer (CIP) with ‘artificial’ biorecognition elements for detection of E. coli using electrochemical impedance spectroscopy (EIS) and the Fe(CN)63-/4- redox probe (Jafari et al. 2019).\nFig. 4 Measurement settings associated with electrochemical biosensor-based multiplexed pathogen detection. a) Microfluidic device with an interdigitated Au microelectrode array for continuous measurement of S. typhimurium (Dastider et al. 2015). b) Conjugated nanoparticles with two different biorecognition elements for E. coli and V. cholerae detection via voltammetry using Fe(CN)63-/4- (Li et al. 2017). c) Schematic of a microfluidic device with two separate spatial regions of biorecognition elements for E. coli and S. aureus (Tian et al. 2016).\nIn addition to fabricating nanostructured electrodes by coupling already processed nanomaterials to planar electrodes, electrochemical methods are also commonly used for bottom-up electrode nanostructuring processes and have been leveraged to fabricate nanostructured electrodes for pathogen detection. For example, Hong et al. fabricated a nanostructured Au electrode via electrochemical deposition of gold (III) chloride hydrates for the detection of norovirus in lettuce extracts (Hong et al. 2015). While the physical or chemical deposition of materials on planar electrodes provides a useful nanostructuring approach, introducing porosity to the electrode, such as nanoporosity, also enables electrode nanostructuring. For example, Nguyen et al. utilized nanoporous alumina-coated Pt microwires for the detection of West Nile virus (Nguyen et al. 2009).\nWhile studies have reported improved biosensor performance using electrode nanostructuring, such as improved sensitivity and LOD, it is prudent to consider the effect of nanostructuring on biorecognition element immobilization and target binding. For example, nanostructured electrodes that exhibit high-aspect-ratio structures and other three-dimensional structures have also been shown to enhance biomolecular steric hindrance effects, which may have implications for pathogen detection applications (Hong et al. 2015; Lam et al. 2012; Mahshid et al. 2017). There also remains a need to understand device-to-device and batch-to-batch variation in electrode nanostructuring quality. For example, it is presently unclear how the structure (e.g., topography, crystal structure) and material properties (e.g., electrical properties) of nanostructured surfaces vary among mass-produced electrodes. It is also unclear how such variance in nanostructuring quality affects the repeatability of biosensor performance."}
LitCovid-PD-FMA-UBERON
{"project":"LitCovid-PD-FMA-UBERON","denotations":[{"id":"T112","span":{"begin":4577,"end":4580},"obj":"Body_part"},{"id":"T113","span":{"begin":4746,"end":4750},"obj":"Body_part"},{"id":"T114","span":{"begin":4968,"end":4972},"obj":"Body_part"},{"id":"T115","span":{"begin":5184,"end":5188},"obj":"Body_part"}],"attributes":[{"id":"A112","pred":"fma_id","subj":"T112","obj":"http://purl.org/sig/ont/fma/fma74412"},{"id":"A113","pred":"fma_id","subj":"T113","obj":"http://purl.org/sig/ont/fma/fma46495"},{"id":"A114","pred":"fma_id","subj":"T114","obj":"http://purl.org/sig/ont/fma/fma46495"},{"id":"A115","pred":"fma_id","subj":"T115","obj":"http://purl.org/sig/ont/fma/fma68646"}],"text":"2.1.5 Electrode nanostructuring\nTransducers with physical dimensions comparable to the target species have been widely investigated as a means of creating sensitive biosensors (Gupta et al. 2004; Pumera et al. 2007; Singh et al. 2010; Wei et al. 2009). Thus, electrodes ranging from micrometers to nanometers have been investigated for pathogen detection. While nanoscale planar electrodes are among the most commonly examined for pathogen detection (Hong et al. 2015; Peh and Li, 2013), the fabrication of nanoscale structures of conducting and semiconducting materials using a wide range of bottom-up and top-down nanomanufacturing processes, such as nanowires, has led to the investigation of nanostructured electrodes for pathogen detection (Patolsky and Lieber, 2005). Nanostructuring can be performed simultaneously with bottom-up electrode fabrication processes or as a post-processing step with top-down electrode fabrication processes.\nNanowire-based electrodes have been fabricated using a variety of engineering materials using both bottom-up and top-down nanomanufacturing processes (Hu et al. 1999; Yogeswaran and Chen, 2008). A detailed review of nanomanufacturing processes for nanowire fabrication can be found elsewhere (Hu et al. 1999). Nanowires can exhibit circular, hexagonal, and even triangular cross-sections. The nanowire aspect ratio, defined as the ratio of the length to width, often ranges from 1 to greater than 10 (Hu et al. 1999; Vaseashta and Dimova-Malinovska, 2005; Wanekaya et al. 2006).\nAs shown in Table 1, metallic and ceramic microwire- and nanowire-based electrodes have been examined for pathogen detection. For example, Wang et al. used nanowire-bundled TiO2 electrodes synthesized using a bottom-up wet chemistry process for the detection of Listeria monocytogenes (L. monocytogenes) (Wang et al. 2008). Shen et al. fabricated silicon nanowire-based electrodes using a chemical vapor deposition process for the rapid detection of human influenza A virus in an array-based format (Shen et al. 2012).\nAlthough polymer nanowires have been relatively more applied to the detection of non-pathogenic species (Travas-Sejdic et al. 2014), there appears to be potential for their application to pathogen detection. Polymer nanowires are also synthesized via bottom-up and top-down nanomanufacturing processes, including hard template methods, soft template methods, or physical approaches, but efficient, large-scale synthesis remains a challenge (Xia et al. 2010). A comprehensive summary of studies using micro- and nano-wire electrodes for pathogen detection is shown in Table 1. For example, Chartuprayoon et al. used Au microelectrode arrays modified with polypyrrole nanoribbons to detect cucumber mosaic virus (Chartuprayoon et al. 2013).\nThe topographical modification of electrode surfaces with micro- and nano-structured features beyond wire-like structures has also been investigated for pathogen detection. Electrode nanostructuring increases the electrode surface area without significantly increasing the electrode volume, thereby increasing the ratio of electrode surface area to fluid volume analyzed (Soleymani et al. 2009). Topographical modification of electrodes can also affect their mechanical and electrical properties. For example, electrochemical deposition of PEDOT on silicon electrodes reduces the electrode electrical impedance across a wide frequency range, which offers measurement advantages for neural monitoring and recording applications (Ludwig et al. 2006).\nElectrode nanostructuring for pathogen detection beyond the fabrication of nanowire-based electrodes has been accomplished primarily using bottom-up wet chemistry approaches and electrochemical methods. Among the wet chemistry approaches for electrode nanostructuring (Eftekhari et al. 2008), nanostructured electrodes are often fabricated by the deposition or coupling of nanoparticles to planar electrodes. For example, AuNPs are commonly deposited on planar electrodes to provide a nanostructured surface for biorecognition element immobilization. In such studies, the particles are bound to the planar electrode via physical adsorption processes (Attar et al. 2016) or chemical methods (Wang et al. 2013). In addition to AuNPs, CNTs have also been extensively investigated as potentially useful nanomaterials for electrode nanostructuring (see Table 1).\nDe Luna et al. found that high-curvature nanostructured Au microelectrodes exhibited a reduced extent of biorecognition element aggregation relative to that found on planar electrodes in DNA sensing studies using a combination of experimental studies and molecular dynamics simulations (see Fig. 3 a) (De Luna et al. 2017; Mahshid et al. 2016). A study by Chin et al. found that nanostructuring of carbon electrodes with carbon nanoparticles enhanced the electron transfer kinetics and current intensity of the electrode by 63% for the detection of Japanese encephalitis virus (Chin et al. 2017).\nFig. 3 Emerging transduction approaches associated with electrochemical biosensors for pathogen detection. a) A nanostructured Au microelectrode array with high curvature (De Luna et al. 2017). b) Cell-imprinted polymer (CIP) with ‘artificial’ biorecognition elements for detection of E. coli using electrochemical impedance spectroscopy (EIS) and the Fe(CN)63-/4- redox probe (Jafari et al. 2019).\nFig. 4 Measurement settings associated with electrochemical biosensor-based multiplexed pathogen detection. a) Microfluidic device with an interdigitated Au microelectrode array for continuous measurement of S. typhimurium (Dastider et al. 2015). b) Conjugated nanoparticles with two different biorecognition elements for E. coli and V. cholerae detection via voltammetry using Fe(CN)63-/4- (Li et al. 2017). c) Schematic of a microfluidic device with two separate spatial regions of biorecognition elements for E. coli and S. aureus (Tian et al. 2016).\nIn addition to fabricating nanostructured electrodes by coupling already processed nanomaterials to planar electrodes, electrochemical methods are also commonly used for bottom-up electrode nanostructuring processes and have been leveraged to fabricate nanostructured electrodes for pathogen detection. For example, Hong et al. fabricated a nanostructured Au electrode via electrochemical deposition of gold (III) chloride hydrates for the detection of norovirus in lettuce extracts (Hong et al. 2015). While the physical or chemical deposition of materials on planar electrodes provides a useful nanostructuring approach, introducing porosity to the electrode, such as nanoporosity, also enables electrode nanostructuring. For example, Nguyen et al. utilized nanoporous alumina-coated Pt microwires for the detection of West Nile virus (Nguyen et al. 2009).\nWhile studies have reported improved biosensor performance using electrode nanostructuring, such as improved sensitivity and LOD, it is prudent to consider the effect of nanostructuring on biorecognition element immobilization and target binding. For example, nanostructured electrodes that exhibit high-aspect-ratio structures and other three-dimensional structures have also been shown to enhance biomolecular steric hindrance effects, which may have implications for pathogen detection applications (Hong et al. 2015; Lam et al. 2012; Mahshid et al. 2017). There also remains a need to understand device-to-device and batch-to-batch variation in electrode nanostructuring quality. For example, it is presently unclear how the structure (e.g., topography, crystal structure) and material properties (e.g., electrical properties) of nanostructured surfaces vary among mass-produced electrodes. It is also unclear how such variance in nanostructuring quality affects the repeatability of biosensor performance."}
LitCovid-PD-UBERON
{"project":"LitCovid-PD-UBERON","denotations":[{"id":"T8","span":{"begin":2448,"end":2453},"obj":"Body_part"},{"id":"T9","span":{"begin":4746,"end":4750},"obj":"Body_part"},{"id":"T10","span":{"begin":4968,"end":4972},"obj":"Body_part"}],"attributes":[{"id":"A8","pred":"uberon_id","subj":"T8","obj":"http://purl.obolibrary.org/obo/UBERON_0002542"},{"id":"A9","pred":"uberon_id","subj":"T9","obj":"http://purl.obolibrary.org/obo/UBERON_0008199"},{"id":"A10","pred":"uberon_id","subj":"T10","obj":"http://purl.obolibrary.org/obo/UBERON_0008199"}],"text":"2.1.5 Electrode nanostructuring\nTransducers with physical dimensions comparable to the target species have been widely investigated as a means of creating sensitive biosensors (Gupta et al. 2004; Pumera et al. 2007; Singh et al. 2010; Wei et al. 2009). Thus, electrodes ranging from micrometers to nanometers have been investigated for pathogen detection. While nanoscale planar electrodes are among the most commonly examined for pathogen detection (Hong et al. 2015; Peh and Li, 2013), the fabrication of nanoscale structures of conducting and semiconducting materials using a wide range of bottom-up and top-down nanomanufacturing processes, such as nanowires, has led to the investigation of nanostructured electrodes for pathogen detection (Patolsky and Lieber, 2005). Nanostructuring can be performed simultaneously with bottom-up electrode fabrication processes or as a post-processing step with top-down electrode fabrication processes.\nNanowire-based electrodes have been fabricated using a variety of engineering materials using both bottom-up and top-down nanomanufacturing processes (Hu et al. 1999; Yogeswaran and Chen, 2008). A detailed review of nanomanufacturing processes for nanowire fabrication can be found elsewhere (Hu et al. 1999). Nanowires can exhibit circular, hexagonal, and even triangular cross-sections. The nanowire aspect ratio, defined as the ratio of the length to width, often ranges from 1 to greater than 10 (Hu et al. 1999; Vaseashta and Dimova-Malinovska, 2005; Wanekaya et al. 2006).\nAs shown in Table 1, metallic and ceramic microwire- and nanowire-based electrodes have been examined for pathogen detection. For example, Wang et al. used nanowire-bundled TiO2 electrodes synthesized using a bottom-up wet chemistry process for the detection of Listeria monocytogenes (L. monocytogenes) (Wang et al. 2008). Shen et al. fabricated silicon nanowire-based electrodes using a chemical vapor deposition process for the rapid detection of human influenza A virus in an array-based format (Shen et al. 2012).\nAlthough polymer nanowires have been relatively more applied to the detection of non-pathogenic species (Travas-Sejdic et al. 2014), there appears to be potential for their application to pathogen detection. Polymer nanowires are also synthesized via bottom-up and top-down nanomanufacturing processes, including hard template methods, soft template methods, or physical approaches, but efficient, large-scale synthesis remains a challenge (Xia et al. 2010). A comprehensive summary of studies using micro- and nano-wire electrodes for pathogen detection is shown in Table 1. For example, Chartuprayoon et al. used Au microelectrode arrays modified with polypyrrole nanoribbons to detect cucumber mosaic virus (Chartuprayoon et al. 2013).\nThe topographical modification of electrode surfaces with micro- and nano-structured features beyond wire-like structures has also been investigated for pathogen detection. Electrode nanostructuring increases the electrode surface area without significantly increasing the electrode volume, thereby increasing the ratio of electrode surface area to fluid volume analyzed (Soleymani et al. 2009). Topographical modification of electrodes can also affect their mechanical and electrical properties. For example, electrochemical deposition of PEDOT on silicon electrodes reduces the electrode electrical impedance across a wide frequency range, which offers measurement advantages for neural monitoring and recording applications (Ludwig et al. 2006).\nElectrode nanostructuring for pathogen detection beyond the fabrication of nanowire-based electrodes has been accomplished primarily using bottom-up wet chemistry approaches and electrochemical methods. Among the wet chemistry approaches for electrode nanostructuring (Eftekhari et al. 2008), nanostructured electrodes are often fabricated by the deposition or coupling of nanoparticles to planar electrodes. For example, AuNPs are commonly deposited on planar electrodes to provide a nanostructured surface for biorecognition element immobilization. In such studies, the particles are bound to the planar electrode via physical adsorption processes (Attar et al. 2016) or chemical methods (Wang et al. 2013). In addition to AuNPs, CNTs have also been extensively investigated as potentially useful nanomaterials for electrode nanostructuring (see Table 1).\nDe Luna et al. found that high-curvature nanostructured Au microelectrodes exhibited a reduced extent of biorecognition element aggregation relative to that found on planar electrodes in DNA sensing studies using a combination of experimental studies and molecular dynamics simulations (see Fig. 3 a) (De Luna et al. 2017; Mahshid et al. 2016). A study by Chin et al. found that nanostructuring of carbon electrodes with carbon nanoparticles enhanced the electron transfer kinetics and current intensity of the electrode by 63% for the detection of Japanese encephalitis virus (Chin et al. 2017).\nFig. 3 Emerging transduction approaches associated with electrochemical biosensors for pathogen detection. a) A nanostructured Au microelectrode array with high curvature (De Luna et al. 2017). b) Cell-imprinted polymer (CIP) with ‘artificial’ biorecognition elements for detection of E. coli using electrochemical impedance spectroscopy (EIS) and the Fe(CN)63-/4- redox probe (Jafari et al. 2019).\nFig. 4 Measurement settings associated with electrochemical biosensor-based multiplexed pathogen detection. a) Microfluidic device with an interdigitated Au microelectrode array for continuous measurement of S. typhimurium (Dastider et al. 2015). b) Conjugated nanoparticles with two different biorecognition elements for E. coli and V. cholerae detection via voltammetry using Fe(CN)63-/4- (Li et al. 2017). c) Schematic of a microfluidic device with two separate spatial regions of biorecognition elements for E. coli and S. aureus (Tian et al. 2016).\nIn addition to fabricating nanostructured electrodes by coupling already processed nanomaterials to planar electrodes, electrochemical methods are also commonly used for bottom-up electrode nanostructuring processes and have been leveraged to fabricate nanostructured electrodes for pathogen detection. For example, Hong et al. fabricated a nanostructured Au electrode via electrochemical deposition of gold (III) chloride hydrates for the detection of norovirus in lettuce extracts (Hong et al. 2015). While the physical or chemical deposition of materials on planar electrodes provides a useful nanostructuring approach, introducing porosity to the electrode, such as nanoporosity, also enables electrode nanostructuring. For example, Nguyen et al. utilized nanoporous alumina-coated Pt microwires for the detection of West Nile virus (Nguyen et al. 2009).\nWhile studies have reported improved biosensor performance using electrode nanostructuring, such as improved sensitivity and LOD, it is prudent to consider the effect of nanostructuring on biorecognition element immobilization and target binding. For example, nanostructured electrodes that exhibit high-aspect-ratio structures and other three-dimensional structures have also been shown to enhance biomolecular steric hindrance effects, which may have implications for pathogen detection applications (Hong et al. 2015; Lam et al. 2012; Mahshid et al. 2017). There also remains a need to understand device-to-device and batch-to-batch variation in electrode nanostructuring quality. For example, it is presently unclear how the structure (e.g., topography, crystal structure) and material properties (e.g., electrical properties) of nanostructured surfaces vary among mass-produced electrodes. It is also unclear how such variance in nanostructuring quality affects the repeatability of biosensor performance."}
LitCovid-PD-MONDO
{"project":"LitCovid-PD-MONDO","denotations":[{"id":"T58","span":{"begin":1981,"end":1990},"obj":"Disease"},{"id":"T59","span":{"begin":4939,"end":4960},"obj":"Disease"},{"id":"T60","span":{"begin":4948,"end":4960},"obj":"Disease"},{"id":"T61","span":{"begin":5208,"end":5211},"obj":"Disease"}],"attributes":[{"id":"A58","pred":"mondo_id","subj":"T58","obj":"http://purl.obolibrary.org/obo/MONDO_0005812"},{"id":"A59","pred":"mondo_id","subj":"T59","obj":"http://purl.obolibrary.org/obo/MONDO_0019209"},{"id":"A60","pred":"mondo_id","subj":"T60","obj":"http://purl.obolibrary.org/obo/MONDO_0019956"},{"id":"A61","pred":"mondo_id","subj":"T61","obj":"http://purl.obolibrary.org/obo/MONDO_0009459"},{"id":"A62","pred":"mondo_id","subj":"T61","obj":"http://purl.obolibrary.org/obo/MONDO_0015364"}],"text":"2.1.5 Electrode nanostructuring\nTransducers with physical dimensions comparable to the target species have been widely investigated as a means of creating sensitive biosensors (Gupta et al. 2004; Pumera et al. 2007; Singh et al. 2010; Wei et al. 2009). Thus, electrodes ranging from micrometers to nanometers have been investigated for pathogen detection. While nanoscale planar electrodes are among the most commonly examined for pathogen detection (Hong et al. 2015; Peh and Li, 2013), the fabrication of nanoscale structures of conducting and semiconducting materials using a wide range of bottom-up and top-down nanomanufacturing processes, such as nanowires, has led to the investigation of nanostructured electrodes for pathogen detection (Patolsky and Lieber, 2005). Nanostructuring can be performed simultaneously with bottom-up electrode fabrication processes or as a post-processing step with top-down electrode fabrication processes.\nNanowire-based electrodes have been fabricated using a variety of engineering materials using both bottom-up and top-down nanomanufacturing processes (Hu et al. 1999; Yogeswaran and Chen, 2008). A detailed review of nanomanufacturing processes for nanowire fabrication can be found elsewhere (Hu et al. 1999). Nanowires can exhibit circular, hexagonal, and even triangular cross-sections. The nanowire aspect ratio, defined as the ratio of the length to width, often ranges from 1 to greater than 10 (Hu et al. 1999; Vaseashta and Dimova-Malinovska, 2005; Wanekaya et al. 2006).\nAs shown in Table 1, metallic and ceramic microwire- and nanowire-based electrodes have been examined for pathogen detection. For example, Wang et al. used nanowire-bundled TiO2 electrodes synthesized using a bottom-up wet chemistry process for the detection of Listeria monocytogenes (L. monocytogenes) (Wang et al. 2008). Shen et al. fabricated silicon nanowire-based electrodes using a chemical vapor deposition process for the rapid detection of human influenza A virus in an array-based format (Shen et al. 2012).\nAlthough polymer nanowires have been relatively more applied to the detection of non-pathogenic species (Travas-Sejdic et al. 2014), there appears to be potential for their application to pathogen detection. Polymer nanowires are also synthesized via bottom-up and top-down nanomanufacturing processes, including hard template methods, soft template methods, or physical approaches, but efficient, large-scale synthesis remains a challenge (Xia et al. 2010). A comprehensive summary of studies using micro- and nano-wire electrodes for pathogen detection is shown in Table 1. For example, Chartuprayoon et al. used Au microelectrode arrays modified with polypyrrole nanoribbons to detect cucumber mosaic virus (Chartuprayoon et al. 2013).\nThe topographical modification of electrode surfaces with micro- and nano-structured features beyond wire-like structures has also been investigated for pathogen detection. Electrode nanostructuring increases the electrode surface area without significantly increasing the electrode volume, thereby increasing the ratio of electrode surface area to fluid volume analyzed (Soleymani et al. 2009). Topographical modification of electrodes can also affect their mechanical and electrical properties. For example, electrochemical deposition of PEDOT on silicon electrodes reduces the electrode electrical impedance across a wide frequency range, which offers measurement advantages for neural monitoring and recording applications (Ludwig et al. 2006).\nElectrode nanostructuring for pathogen detection beyond the fabrication of nanowire-based electrodes has been accomplished primarily using bottom-up wet chemistry approaches and electrochemical methods. Among the wet chemistry approaches for electrode nanostructuring (Eftekhari et al. 2008), nanostructured electrodes are often fabricated by the deposition or coupling of nanoparticles to planar electrodes. For example, AuNPs are commonly deposited on planar electrodes to provide a nanostructured surface for biorecognition element immobilization. In such studies, the particles are bound to the planar electrode via physical adsorption processes (Attar et al. 2016) or chemical methods (Wang et al. 2013). In addition to AuNPs, CNTs have also been extensively investigated as potentially useful nanomaterials for electrode nanostructuring (see Table 1).\nDe Luna et al. found that high-curvature nanostructured Au microelectrodes exhibited a reduced extent of biorecognition element aggregation relative to that found on planar electrodes in DNA sensing studies using a combination of experimental studies and molecular dynamics simulations (see Fig. 3 a) (De Luna et al. 2017; Mahshid et al. 2016). A study by Chin et al. found that nanostructuring of carbon electrodes with carbon nanoparticles enhanced the electron transfer kinetics and current intensity of the electrode by 63% for the detection of Japanese encephalitis virus (Chin et al. 2017).\nFig. 3 Emerging transduction approaches associated with electrochemical biosensors for pathogen detection. a) A nanostructured Au microelectrode array with high curvature (De Luna et al. 2017). b) Cell-imprinted polymer (CIP) with ‘artificial’ biorecognition elements for detection of E. coli using electrochemical impedance spectroscopy (EIS) and the Fe(CN)63-/4- redox probe (Jafari et al. 2019).\nFig. 4 Measurement settings associated with electrochemical biosensor-based multiplexed pathogen detection. a) Microfluidic device with an interdigitated Au microelectrode array for continuous measurement of S. typhimurium (Dastider et al. 2015). b) Conjugated nanoparticles with two different biorecognition elements for E. coli and V. cholerae detection via voltammetry using Fe(CN)63-/4- (Li et al. 2017). c) Schematic of a microfluidic device with two separate spatial regions of biorecognition elements for E. coli and S. aureus (Tian et al. 2016).\nIn addition to fabricating nanostructured electrodes by coupling already processed nanomaterials to planar electrodes, electrochemical methods are also commonly used for bottom-up electrode nanostructuring processes and have been leveraged to fabricate nanostructured electrodes for pathogen detection. For example, Hong et al. fabricated a nanostructured Au electrode via electrochemical deposition of gold (III) chloride hydrates for the detection of norovirus in lettuce extracts (Hong et al. 2015). While the physical or chemical deposition of materials on planar electrodes provides a useful nanostructuring approach, introducing porosity to the electrode, such as nanoporosity, also enables electrode nanostructuring. For example, Nguyen et al. utilized nanoporous alumina-coated Pt microwires for the detection of West Nile virus (Nguyen et al. 2009).\nWhile studies have reported improved biosensor performance using electrode nanostructuring, such as improved sensitivity and LOD, it is prudent to consider the effect of nanostructuring on biorecognition element immobilization and target binding. For example, nanostructured electrodes that exhibit high-aspect-ratio structures and other three-dimensional structures have also been shown to enhance biomolecular steric hindrance effects, which may have implications for pathogen detection applications (Hong et al. 2015; Lam et al. 2012; Mahshid et al. 2017). There also remains a need to understand device-to-device and batch-to-batch variation in electrode nanostructuring quality. For example, it is presently unclear how the structure (e.g., topography, crystal structure) and material properties (e.g., electrical properties) of nanostructured surfaces vary among mass-produced electrodes. It is also unclear how such variance in nanostructuring quality affects the repeatability of biosensor performance."}
LitCovid-PD-CLO
{"project":"LitCovid-PD-CLO","denotations":[{"id":"T336","span":{"begin":136,"end":137},"obj":"http://purl.obolibrary.org/obo/CLO_0001020"},{"id":"T337","span":{"begin":478,"end":480},"obj":"http://purl.obolibrary.org/obo/CLO_0001022"},{"id":"T338","span":{"begin":478,"end":480},"obj":"http://purl.obolibrary.org/obo/CLO_0007314"},{"id":"T339","span":{"begin":578,"end":579},"obj":"http://purl.obolibrary.org/obo/CLO_0001020"},{"id":"T340","span":{"begin":665,"end":668},"obj":"http://purl.obolibrary.org/obo/CLO_0051582"},{"id":"T341","span":{"begin":876,"end":877},"obj":"http://purl.obolibrary.org/obo/CLO_0001020"},{"id":"T342","span":{"begin":999,"end":1000},"obj":"http://purl.obolibrary.org/obo/CLO_0001020"},{"id":"T343","span":{"begin":1141,"end":1142},"obj":"http://purl.obolibrary.org/obo/CLO_0001020"},{"id":"T344","span":{"begin":1732,"end":1733},"obj":"http://purl.obolibrary.org/obo/CLO_0001020"},{"id":"T345","span":{"begin":1912,"end":1913},"obj":"http://purl.obolibrary.org/obo/CLO_0001020"},{"id":"T346","span":{"begin":1975,"end":1980},"obj":"http://purl.obolibrary.org/obo/NCBITaxon_9606"},{"id":"T347","span":{"begin":1991,"end":1992},"obj":"http://purl.obolibrary.org/obo/CLO_0001020"},{"id":"T348","span":{"begin":1993,"end":1998},"obj":"http://purl.obolibrary.org/obo/NCBITaxon_10239"},{"id":"T349","span":{"begin":2472,"end":2473},"obj":"http://purl.obolibrary.org/obo/CLO_0001020"},{"id":"T350","span":{"begin":2503,"end":2504},"obj":"http://purl.obolibrary.org/obo/CLO_0001020"},{"id":"T351","span":{"begin":2748,"end":2753},"obj":"http://purl.obolibrary.org/obo/NCBITaxon_10239"},{"id":"T352","span":{"begin":2905,"end":2908},"obj":"http://purl.obolibrary.org/obo/CLO_0051582"},{"id":"T353","span":{"begin":3401,"end":3402},"obj":"http://purl.obolibrary.org/obo/CLO_0001020"},{"id":"T354","span":{"begin":3633,"end":3636},"obj":"http://purl.obolibrary.org/obo/CLO_0051582"},{"id":"T355","span":{"begin":4015,"end":4016},"obj":"http://purl.obolibrary.org/obo/CLO_0001020"},{"id":"T356","span":{"begin":4475,"end":4476},"obj":"http://purl.obolibrary.org/obo/CLO_0001020"},{"id":"T357","span":{"begin":4603,"end":4604},"obj":"http://purl.obolibrary.org/obo/CLO_0001020"},{"id":"T358","span":{"begin":4688,"end":4689},"obj":"http://purl.obolibrary.org/obo/CLO_0001020"},{"id":"T359","span":{"begin":4735,"end":4736},"obj":"http://purl.obolibrary.org/obo/CLO_0001020"},{"id":"T360","span":{"begin":4961,"end":4966},"obj":"http://purl.obolibrary.org/obo/NCBITaxon_10239"},{"id":"T361","span":{"begin":5094,"end":5098},"obj":"http://purl.obolibrary.org/obo/CLO_0001627"},{"id":"T362","span":{"begin":5181,"end":5188},"obj":"http://purl.obolibrary.org/obo/CL_0000236"},{"id":"T363","span":{"begin":5494,"end":5495},"obj":"http://purl.obolibrary.org/obo/CLO_0001020"},{"id":"T364","span":{"begin":5510,"end":5516},"obj":"http://purl.obolibrary.org/obo/OBI_0000968"},{"id":"T365","span":{"begin":5633,"end":5634},"obj":"http://purl.obolibrary.org/obo/CLO_0001021"},{"id":"T366","span":{"begin":5778,"end":5780},"obj":"http://purl.obolibrary.org/obo/CLO_0001022"},{"id":"T367","span":{"begin":5778,"end":5780},"obj":"http://purl.obolibrary.org/obo/CLO_0007314"},{"id":"T368","span":{"begin":5811,"end":5812},"obj":"http://purl.obolibrary.org/obo/CLO_0001020"},{"id":"T369","span":{"begin":5826,"end":5832},"obj":"http://purl.obolibrary.org/obo/OBI_0000968"},{"id":"T370","span":{"begin":5851,"end":5866},"obj":"http://purl.obolibrary.org/obo/BFO_0000006"},{"id":"T371","span":{"begin":6279,"end":6280},"obj":"http://purl.obolibrary.org/obo/CLO_0001020"},{"id":"T372","span":{"begin":6528,"end":6529},"obj":"http://purl.obolibrary.org/obo/CLO_0001020"},{"id":"T373","span":{"begin":6771,"end":6776},"obj":"http://purl.obolibrary.org/obo/NCBITaxon_10239"},{"id":"T374","span":{"begin":7378,"end":7379},"obj":"http://purl.obolibrary.org/obo/CLO_0001020"},{"id":"T375","span":{"begin":7399,"end":7405},"obj":"http://purl.obolibrary.org/obo/OBI_0000968"},{"id":"T376","span":{"begin":7409,"end":7415},"obj":"http://purl.obolibrary.org/obo/OBI_0000968"}],"text":"2.1.5 Electrode nanostructuring\nTransducers with physical dimensions comparable to the target species have been widely investigated as a means of creating sensitive biosensors (Gupta et al. 2004; Pumera et al. 2007; Singh et al. 2010; Wei et al. 2009). Thus, electrodes ranging from micrometers to nanometers have been investigated for pathogen detection. While nanoscale planar electrodes are among the most commonly examined for pathogen detection (Hong et al. 2015; Peh and Li, 2013), the fabrication of nanoscale structures of conducting and semiconducting materials using a wide range of bottom-up and top-down nanomanufacturing processes, such as nanowires, has led to the investigation of nanostructured electrodes for pathogen detection (Patolsky and Lieber, 2005). Nanostructuring can be performed simultaneously with bottom-up electrode fabrication processes or as a post-processing step with top-down electrode fabrication processes.\nNanowire-based electrodes have been fabricated using a variety of engineering materials using both bottom-up and top-down nanomanufacturing processes (Hu et al. 1999; Yogeswaran and Chen, 2008). A detailed review of nanomanufacturing processes for nanowire fabrication can be found elsewhere (Hu et al. 1999). Nanowires can exhibit circular, hexagonal, and even triangular cross-sections. The nanowire aspect ratio, defined as the ratio of the length to width, often ranges from 1 to greater than 10 (Hu et al. 1999; Vaseashta and Dimova-Malinovska, 2005; Wanekaya et al. 2006).\nAs shown in Table 1, metallic and ceramic microwire- and nanowire-based electrodes have been examined for pathogen detection. For example, Wang et al. used nanowire-bundled TiO2 electrodes synthesized using a bottom-up wet chemistry process for the detection of Listeria monocytogenes (L. monocytogenes) (Wang et al. 2008). Shen et al. fabricated silicon nanowire-based electrodes using a chemical vapor deposition process for the rapid detection of human influenza A virus in an array-based format (Shen et al. 2012).\nAlthough polymer nanowires have been relatively more applied to the detection of non-pathogenic species (Travas-Sejdic et al. 2014), there appears to be potential for their application to pathogen detection. Polymer nanowires are also synthesized via bottom-up and top-down nanomanufacturing processes, including hard template methods, soft template methods, or physical approaches, but efficient, large-scale synthesis remains a challenge (Xia et al. 2010). A comprehensive summary of studies using micro- and nano-wire electrodes for pathogen detection is shown in Table 1. For example, Chartuprayoon et al. used Au microelectrode arrays modified with polypyrrole nanoribbons to detect cucumber mosaic virus (Chartuprayoon et al. 2013).\nThe topographical modification of electrode surfaces with micro- and nano-structured features beyond wire-like structures has also been investigated for pathogen detection. Electrode nanostructuring increases the electrode surface area without significantly increasing the electrode volume, thereby increasing the ratio of electrode surface area to fluid volume analyzed (Soleymani et al. 2009). Topographical modification of electrodes can also affect their mechanical and electrical properties. For example, electrochemical deposition of PEDOT on silicon electrodes reduces the electrode electrical impedance across a wide frequency range, which offers measurement advantages for neural monitoring and recording applications (Ludwig et al. 2006).\nElectrode nanostructuring for pathogen detection beyond the fabrication of nanowire-based electrodes has been accomplished primarily using bottom-up wet chemistry approaches and electrochemical methods. Among the wet chemistry approaches for electrode nanostructuring (Eftekhari et al. 2008), nanostructured electrodes are often fabricated by the deposition or coupling of nanoparticles to planar electrodes. For example, AuNPs are commonly deposited on planar electrodes to provide a nanostructured surface for biorecognition element immobilization. In such studies, the particles are bound to the planar electrode via physical adsorption processes (Attar et al. 2016) or chemical methods (Wang et al. 2013). In addition to AuNPs, CNTs have also been extensively investigated as potentially useful nanomaterials for electrode nanostructuring (see Table 1).\nDe Luna et al. found that high-curvature nanostructured Au microelectrodes exhibited a reduced extent of biorecognition element aggregation relative to that found on planar electrodes in DNA sensing studies using a combination of experimental studies and molecular dynamics simulations (see Fig. 3 a) (De Luna et al. 2017; Mahshid et al. 2016). A study by Chin et al. found that nanostructuring of carbon electrodes with carbon nanoparticles enhanced the electron transfer kinetics and current intensity of the electrode by 63% for the detection of Japanese encephalitis virus (Chin et al. 2017).\nFig. 3 Emerging transduction approaches associated with electrochemical biosensors for pathogen detection. a) A nanostructured Au microelectrode array with high curvature (De Luna et al. 2017). b) Cell-imprinted polymer (CIP) with ‘artificial’ biorecognition elements for detection of E. coli using electrochemical impedance spectroscopy (EIS) and the Fe(CN)63-/4- redox probe (Jafari et al. 2019).\nFig. 4 Measurement settings associated with electrochemical biosensor-based multiplexed pathogen detection. a) Microfluidic device with an interdigitated Au microelectrode array for continuous measurement of S. typhimurium (Dastider et al. 2015). b) Conjugated nanoparticles with two different biorecognition elements for E. coli and V. cholerae detection via voltammetry using Fe(CN)63-/4- (Li et al. 2017). c) Schematic of a microfluidic device with two separate spatial regions of biorecognition elements for E. coli and S. aureus (Tian et al. 2016).\nIn addition to fabricating nanostructured electrodes by coupling already processed nanomaterials to planar electrodes, electrochemical methods are also commonly used for bottom-up electrode nanostructuring processes and have been leveraged to fabricate nanostructured electrodes for pathogen detection. For example, Hong et al. fabricated a nanostructured Au electrode via electrochemical deposition of gold (III) chloride hydrates for the detection of norovirus in lettuce extracts (Hong et al. 2015). While the physical or chemical deposition of materials on planar electrodes provides a useful nanostructuring approach, introducing porosity to the electrode, such as nanoporosity, also enables electrode nanostructuring. For example, Nguyen et al. utilized nanoporous alumina-coated Pt microwires for the detection of West Nile virus (Nguyen et al. 2009).\nWhile studies have reported improved biosensor performance using electrode nanostructuring, such as improved sensitivity and LOD, it is prudent to consider the effect of nanostructuring on biorecognition element immobilization and target binding. For example, nanostructured electrodes that exhibit high-aspect-ratio structures and other three-dimensional structures have also been shown to enhance biomolecular steric hindrance effects, which may have implications for pathogen detection applications (Hong et al. 2015; Lam et al. 2012; Mahshid et al. 2017). There also remains a need to understand device-to-device and batch-to-batch variation in electrode nanostructuring quality. For example, it is presently unclear how the structure (e.g., topography, crystal structure) and material properties (e.g., electrical properties) of nanostructured surfaces vary among mass-produced electrodes. It is also unclear how such variance in nanostructuring quality affects the repeatability of biosensor performance."}
LitCovid-PD-CHEBI
{"project":"LitCovid-PD-CHEBI","denotations":[{"id":"T873","span":{"begin":478,"end":480},"obj":"Chemical"},{"id":"T874","span":{"begin":1194,"end":1202},"obj":"Chemical"},{"id":"T875","span":{"begin":1339,"end":1347},"obj":"Chemical"},{"id":"T876","span":{"begin":1582,"end":1590},"obj":"Chemical"},{"id":"T877","span":{"begin":1681,"end":1689},"obj":"Chemical"},{"id":"T878","span":{"begin":1698,"end":1702},"obj":"Chemical"},{"id":"T879","span":{"begin":1872,"end":1879},"obj":"Chemical"},{"id":"T880","span":{"begin":1880,"end":1888},"obj":"Chemical"},{"id":"T881","span":{"begin":2053,"end":2060},"obj":"Chemical"},{"id":"T883","span":{"begin":2217,"end":2228},"obj":"Chemical"},{"id":"T884","span":{"begin":2252,"end":2259},"obj":"Chemical"},{"id":"T885","span":{"begin":2659,"end":2661},"obj":"Chemical"},{"id":"T886","span":{"begin":2698,"end":2709},"obj":"Chemical"},{"id":"T888","span":{"begin":3323,"end":3328},"obj":"Chemical"},{"id":"T890","span":{"begin":3332,"end":3339},"obj":"Chemical"},{"id":"T8928","span":{"begin":3607,"end":3615},"obj":"Chemical"},{"id":"T10559","span":{"begin":3905,"end":3918},"obj":"Chemical"},{"id":"T35760","span":{"begin":4446,"end":4448},"obj":"Chemical"},{"id":"T53769","span":{"begin":4577,"end":4580},"obj":"Chemical"},{"id":"T37781","span":{"begin":4788,"end":4794},"obj":"Chemical"},{"id":"T56418","span":{"begin":4811,"end":4817},"obj":"Chemical"},{"id":"T32697","span":{"begin":4818,"end":4831},"obj":"Chemical"},{"id":"T26618","span":{"begin":4845,"end":4853},"obj":"Chemical"},{"id":"T5809","span":{"begin":5114,"end":5116},"obj":"Chemical"},{"id":"T47439","span":{"begin":5199,"end":5206},"obj":"Chemical"},{"id":"T81022","span":{"begin":5326,"end":5329},"obj":"Chemical"},{"id":"T16800","span":{"begin":5339,"end":5341},"obj":"Chemical"},{"id":"T75529","span":{"begin":5358,"end":5363},"obj":"Chemical"},{"id":"T57023","span":{"begin":5540,"end":5542},"obj":"Chemical"},{"id":"T53521","span":{"begin":5647,"end":5660},"obj":"Chemical"},{"id":"T23103","span":{"begin":5764,"end":5766},"obj":"Chemical"},{"id":"T20","span":{"begin":5778,"end":5780},"obj":"Chemical"},{"id":"T45528","span":{"begin":6296,"end":6298},"obj":"Chemical"},{"id":"T67953","span":{"begin":6343,"end":6362},"obj":"Chemical"},{"id":"T82273","span":{"begin":6343,"end":6347},"obj":"Chemical"},{"id":"T60058","span":{"begin":6354,"end":6362},"obj":"Chemical"},{"id":"T11281","span":{"begin":6363,"end":6371},"obj":"Chemical"},{"id":"T16339","span":{"begin":6711,"end":6718},"obj":"Chemical"},{"id":"T78755","span":{"begin":6726,"end":6728},"obj":"Chemical"}],"attributes":[{"id":"A873","pred":"chebi_id","subj":"T873","obj":"http://purl.obolibrary.org/obo/CHEBI_30145"},{"id":"A874","pred":"chebi_id","subj":"T874","obj":"http://purl.obolibrary.org/obo/CHEBI_52593"},{"id":"A875","pred":"chebi_id","subj":"T875","obj":"http://purl.obolibrary.org/obo/CHEBI_52593"},{"id":"A876","pred":"chebi_id","subj":"T876","obj":"http://purl.obolibrary.org/obo/CHEBI_52593"},{"id":"A877","pred":"chebi_id","subj":"T877","obj":"http://purl.obolibrary.org/obo/CHEBI_52593"},{"id":"A878","pred":"chebi_id","subj":"T878","obj":"http://purl.obolibrary.org/obo/CHEBI_32234"},{"id":"A879","pred":"chebi_id","subj":"T879","obj":"http://purl.obolibrary.org/obo/CHEBI_27573"},{"id":"A880","pred":"chebi_id","subj":"T880","obj":"http://purl.obolibrary.org/obo/CHEBI_52593"},{"id":"A881","pred":"chebi_id","subj":"T881","obj":"http://purl.obolibrary.org/obo/CHEBI_33839"},{"id":"A882","pred":"chebi_id","subj":"T881","obj":"http://purl.obolibrary.org/obo/CHEBI_60027"},{"id":"A883","pred":"chebi_id","subj":"T883","obj":"http://purl.obolibrary.org/obo/CHEBI_33232"},{"id":"A884","pred":"chebi_id","subj":"T884","obj":"http://purl.obolibrary.org/obo/CHEBI_60027"},{"id":"A885","pred":"chebi_id","subj":"T885","obj":"http://purl.obolibrary.org/obo/CHEBI_29287"},{"id":"A886","pred":"chebi_id","subj":"T886","obj":"http://purl.obolibrary.org/obo/CHEBI_38077"},{"id":"A887","pred":"chebi_id","subj":"T886","obj":"http://purl.obolibrary.org/obo/CHEBI_53263"},{"id":"A888","pred":"chebi_id","subj":"T888","obj":"http://purl.obolibrary.org/obo/CHEBI_53228"},{"id":"A889","pred":"chebi_id","subj":"T888","obj":"http://purl.obolibrary.org/obo/CHEBI_60622"},{"id":"A890","pred":"chebi_id","subj":"T890","obj":"http://purl.obolibrary.org/obo/CHEBI_27573"},{"id":"A69177","pred":"chebi_id","subj":"T8928","obj":"http://purl.obolibrary.org/obo/CHEBI_52593"},{"id":"A4079","pred":"chebi_id","subj":"T10559","obj":"http://purl.obolibrary.org/obo/CHEBI_50803"},{"id":"A20858","pred":"chebi_id","subj":"T35760","obj":"http://purl.obolibrary.org/obo/CHEBI_29287"},{"id":"A92058","pred":"chebi_id","subj":"T53769","obj":"http://purl.obolibrary.org/obo/CHEBI_16991"},{"id":"A10173","pred":"chebi_id","subj":"T37781","obj":"http://purl.obolibrary.org/obo/CHEBI_27594"},{"id":"A87902","pred":"chebi_id","subj":"T37781","obj":"http://purl.obolibrary.org/obo/CHEBI_33415"},{"id":"A10190","pred":"chebi_id","subj":"T56418","obj":"http://purl.obolibrary.org/obo/CHEBI_27594"},{"id":"A99954","pred":"chebi_id","subj":"T56418","obj":"http://purl.obolibrary.org/obo/CHEBI_33415"},{"id":"A42887","pred":"chebi_id","subj":"T32697","obj":"http://purl.obolibrary.org/obo/CHEBI_50803"},{"id":"A74550","pred":"chebi_id","subj":"T26618","obj":"http://purl.obolibrary.org/obo/CHEBI_10545"},{"id":"A83842","pred":"chebi_id","subj":"T5809","obj":"http://purl.obolibrary.org/obo/CHEBI_29287"},{"id":"A88034","pred":"chebi_id","subj":"T47439","obj":"http://purl.obolibrary.org/obo/CHEBI_33839"},{"id":"A43536","pred":"chebi_id","subj":"T47439","obj":"http://purl.obolibrary.org/obo/CHEBI_60027"},{"id":"A48064","pred":"chebi_id","subj":"T81022","obj":"http://purl.obolibrary.org/obo/CHEBI_73498"},{"id":"A64365","pred":"chebi_id","subj":"T16800","obj":"http://purl.obolibrary.org/obo/CHEBI_18248"},{"id":"A81225","pred":"chebi_id","subj":"T75529","obj":"http://purl.obolibrary.org/obo/CHEBI_50406"},{"id":"A94189","pred":"chebi_id","subj":"T57023","obj":"http://purl.obolibrary.org/obo/CHEBI_29287"},{"id":"A94923","pred":"chebi_id","subj":"T53521","obj":"http://purl.obolibrary.org/obo/CHEBI_50803"},{"id":"A97005","pred":"chebi_id","subj":"T23103","obj":"http://purl.obolibrary.org/obo/CHEBI_18248"},{"id":"A52321","pred":"chebi_id","subj":"T20","obj":"http://purl.obolibrary.org/obo/CHEBI_30145"},{"id":"A3457","pred":"chebi_id","subj":"T45528","obj":"http://purl.obolibrary.org/obo/CHEBI_29287"},{"id":"A23113","pred":"chebi_id","subj":"T67953","obj":"http://purl.obolibrary.org/obo/CHEBI_30076"},{"id":"A30644","pred":"chebi_id","subj":"T82273","obj":"http://purl.obolibrary.org/obo/CHEBI_29287"},{"id":"A70178","pred":"chebi_id","subj":"T82273","obj":"http://purl.obolibrary.org/obo/CHEBI_30050"},{"id":"A9877","pred":"chebi_id","subj":"T60058","obj":"http://purl.obolibrary.org/obo/CHEBI_17996"},{"id":"A60158","pred":"chebi_id","subj":"T11281","obj":"http://purl.obolibrary.org/obo/CHEBI_35505"},{"id":"A57508","pred":"chebi_id","subj":"T16339","obj":"http://purl.obolibrary.org/obo/CHEBI_30187"},{"id":"A6433","pred":"chebi_id","subj":"T78755","obj":"http://purl.obolibrary.org/obo/CHEBI_33364"},{"id":"A42504","pred":"chebi_id","subj":"T78755","obj":"http://purl.obolibrary.org/obo/CHEBI_75318"}],"text":"2.1.5 Electrode nanostructuring\nTransducers with physical dimensions comparable to the target species have been widely investigated as a means of creating sensitive biosensors (Gupta et al. 2004; Pumera et al. 2007; Singh et al. 2010; Wei et al. 2009). Thus, electrodes ranging from micrometers to nanometers have been investigated for pathogen detection. While nanoscale planar electrodes are among the most commonly examined for pathogen detection (Hong et al. 2015; Peh and Li, 2013), the fabrication of nanoscale structures of conducting and semiconducting materials using a wide range of bottom-up and top-down nanomanufacturing processes, such as nanowires, has led to the investigation of nanostructured electrodes for pathogen detection (Patolsky and Lieber, 2005). Nanostructuring can be performed simultaneously with bottom-up electrode fabrication processes or as a post-processing step with top-down electrode fabrication processes.\nNanowire-based electrodes have been fabricated using a variety of engineering materials using both bottom-up and top-down nanomanufacturing processes (Hu et al. 1999; Yogeswaran and Chen, 2008). A detailed review of nanomanufacturing processes for nanowire fabrication can be found elsewhere (Hu et al. 1999). Nanowires can exhibit circular, hexagonal, and even triangular cross-sections. The nanowire aspect ratio, defined as the ratio of the length to width, often ranges from 1 to greater than 10 (Hu et al. 1999; Vaseashta and Dimova-Malinovska, 2005; Wanekaya et al. 2006).\nAs shown in Table 1, metallic and ceramic microwire- and nanowire-based electrodes have been examined for pathogen detection. For example, Wang et al. used nanowire-bundled TiO2 electrodes synthesized using a bottom-up wet chemistry process for the detection of Listeria monocytogenes (L. monocytogenes) (Wang et al. 2008). Shen et al. fabricated silicon nanowire-based electrodes using a chemical vapor deposition process for the rapid detection of human influenza A virus in an array-based format (Shen et al. 2012).\nAlthough polymer nanowires have been relatively more applied to the detection of non-pathogenic species (Travas-Sejdic et al. 2014), there appears to be potential for their application to pathogen detection. Polymer nanowires are also synthesized via bottom-up and top-down nanomanufacturing processes, including hard template methods, soft template methods, or physical approaches, but efficient, large-scale synthesis remains a challenge (Xia et al. 2010). A comprehensive summary of studies using micro- and nano-wire electrodes for pathogen detection is shown in Table 1. For example, Chartuprayoon et al. used Au microelectrode arrays modified with polypyrrole nanoribbons to detect cucumber mosaic virus (Chartuprayoon et al. 2013).\nThe topographical modification of electrode surfaces with micro- and nano-structured features beyond wire-like structures has also been investigated for pathogen detection. Electrode nanostructuring increases the electrode surface area without significantly increasing the electrode volume, thereby increasing the ratio of electrode surface area to fluid volume analyzed (Soleymani et al. 2009). Topographical modification of electrodes can also affect their mechanical and electrical properties. For example, electrochemical deposition of PEDOT on silicon electrodes reduces the electrode electrical impedance across a wide frequency range, which offers measurement advantages for neural monitoring and recording applications (Ludwig et al. 2006).\nElectrode nanostructuring for pathogen detection beyond the fabrication of nanowire-based electrodes has been accomplished primarily using bottom-up wet chemistry approaches and electrochemical methods. Among the wet chemistry approaches for electrode nanostructuring (Eftekhari et al. 2008), nanostructured electrodes are often fabricated by the deposition or coupling of nanoparticles to planar electrodes. For example, AuNPs are commonly deposited on planar electrodes to provide a nanostructured surface for biorecognition element immobilization. In such studies, the particles are bound to the planar electrode via physical adsorption processes (Attar et al. 2016) or chemical methods (Wang et al. 2013). In addition to AuNPs, CNTs have also been extensively investigated as potentially useful nanomaterials for electrode nanostructuring (see Table 1).\nDe Luna et al. found that high-curvature nanostructured Au microelectrodes exhibited a reduced extent of biorecognition element aggregation relative to that found on planar electrodes in DNA sensing studies using a combination of experimental studies and molecular dynamics simulations (see Fig. 3 a) (De Luna et al. 2017; Mahshid et al. 2016). A study by Chin et al. found that nanostructuring of carbon electrodes with carbon nanoparticles enhanced the electron transfer kinetics and current intensity of the electrode by 63% for the detection of Japanese encephalitis virus (Chin et al. 2017).\nFig. 3 Emerging transduction approaches associated with electrochemical biosensors for pathogen detection. a) A nanostructured Au microelectrode array with high curvature (De Luna et al. 2017). b) Cell-imprinted polymer (CIP) with ‘artificial’ biorecognition elements for detection of E. coli using electrochemical impedance spectroscopy (EIS) and the Fe(CN)63-/4- redox probe (Jafari et al. 2019).\nFig. 4 Measurement settings associated with electrochemical biosensor-based multiplexed pathogen detection. a) Microfluidic device with an interdigitated Au microelectrode array for continuous measurement of S. typhimurium (Dastider et al. 2015). b) Conjugated nanoparticles with two different biorecognition elements for E. coli and V. cholerae detection via voltammetry using Fe(CN)63-/4- (Li et al. 2017). c) Schematic of a microfluidic device with two separate spatial regions of biorecognition elements for E. coli and S. aureus (Tian et al. 2016).\nIn addition to fabricating nanostructured electrodes by coupling already processed nanomaterials to planar electrodes, electrochemical methods are also commonly used for bottom-up electrode nanostructuring processes and have been leveraged to fabricate nanostructured electrodes for pathogen detection. For example, Hong et al. fabricated a nanostructured Au electrode via electrochemical deposition of gold (III) chloride hydrates for the detection of norovirus in lettuce extracts (Hong et al. 2015). While the physical or chemical deposition of materials on planar electrodes provides a useful nanostructuring approach, introducing porosity to the electrode, such as nanoporosity, also enables electrode nanostructuring. For example, Nguyen et al. utilized nanoporous alumina-coated Pt microwires for the detection of West Nile virus (Nguyen et al. 2009).\nWhile studies have reported improved biosensor performance using electrode nanostructuring, such as improved sensitivity and LOD, it is prudent to consider the effect of nanostructuring on biorecognition element immobilization and target binding. For example, nanostructured electrodes that exhibit high-aspect-ratio structures and other three-dimensional structures have also been shown to enhance biomolecular steric hindrance effects, which may have implications for pathogen detection applications (Hong et al. 2015; Lam et al. 2012; Mahshid et al. 2017). There also remains a need to understand device-to-device and batch-to-batch variation in electrode nanostructuring quality. For example, it is presently unclear how the structure (e.g., topography, crystal structure) and material properties (e.g., electrical properties) of nanostructured surfaces vary among mass-produced electrodes. It is also unclear how such variance in nanostructuring quality affects the repeatability of biosensor performance."}
LitCovid-PD-GO-BP
{"project":"LitCovid-PD-GO-BP","denotations":[{"id":"T47165","span":{"begin":2454,"end":2463},"obj":"http://purl.obolibrary.org/obo/GO_0009058"},{"id":"T48052","span":{"begin":4845,"end":4862},"obj":"http://purl.obolibrary.org/obo/GO_0022904"},{"id":"T32260","span":{"begin":5003,"end":5015},"obj":"http://purl.obolibrary.org/obo/GO_0009293"}],"text":"2.1.5 Electrode nanostructuring\nTransducers with physical dimensions comparable to the target species have been widely investigated as a means of creating sensitive biosensors (Gupta et al. 2004; Pumera et al. 2007; Singh et al. 2010; Wei et al. 2009). Thus, electrodes ranging from micrometers to nanometers have been investigated for pathogen detection. While nanoscale planar electrodes are among the most commonly examined for pathogen detection (Hong et al. 2015; Peh and Li, 2013), the fabrication of nanoscale structures of conducting and semiconducting materials using a wide range of bottom-up and top-down nanomanufacturing processes, such as nanowires, has led to the investigation of nanostructured electrodes for pathogen detection (Patolsky and Lieber, 2005). Nanostructuring can be performed simultaneously with bottom-up electrode fabrication processes or as a post-processing step with top-down electrode fabrication processes.\nNanowire-based electrodes have been fabricated using a variety of engineering materials using both bottom-up and top-down nanomanufacturing processes (Hu et al. 1999; Yogeswaran and Chen, 2008). A detailed review of nanomanufacturing processes for nanowire fabrication can be found elsewhere (Hu et al. 1999). Nanowires can exhibit circular, hexagonal, and even triangular cross-sections. The nanowire aspect ratio, defined as the ratio of the length to width, often ranges from 1 to greater than 10 (Hu et al. 1999; Vaseashta and Dimova-Malinovska, 2005; Wanekaya et al. 2006).\nAs shown in Table 1, metallic and ceramic microwire- and nanowire-based electrodes have been examined for pathogen detection. For example, Wang et al. used nanowire-bundled TiO2 electrodes synthesized using a bottom-up wet chemistry process for the detection of Listeria monocytogenes (L. monocytogenes) (Wang et al. 2008). Shen et al. fabricated silicon nanowire-based electrodes using a chemical vapor deposition process for the rapid detection of human influenza A virus in an array-based format (Shen et al. 2012).\nAlthough polymer nanowires have been relatively more applied to the detection of non-pathogenic species (Travas-Sejdic et al. 2014), there appears to be potential for their application to pathogen detection. Polymer nanowires are also synthesized via bottom-up and top-down nanomanufacturing processes, including hard template methods, soft template methods, or physical approaches, but efficient, large-scale synthesis remains a challenge (Xia et al. 2010). A comprehensive summary of studies using micro- and nano-wire electrodes for pathogen detection is shown in Table 1. For example, Chartuprayoon et al. used Au microelectrode arrays modified with polypyrrole nanoribbons to detect cucumber mosaic virus (Chartuprayoon et al. 2013).\nThe topographical modification of electrode surfaces with micro- and nano-structured features beyond wire-like structures has also been investigated for pathogen detection. Electrode nanostructuring increases the electrode surface area without significantly increasing the electrode volume, thereby increasing the ratio of electrode surface area to fluid volume analyzed (Soleymani et al. 2009). Topographical modification of electrodes can also affect their mechanical and electrical properties. For example, electrochemical deposition of PEDOT on silicon electrodes reduces the electrode electrical impedance across a wide frequency range, which offers measurement advantages for neural monitoring and recording applications (Ludwig et al. 2006).\nElectrode nanostructuring for pathogen detection beyond the fabrication of nanowire-based electrodes has been accomplished primarily using bottom-up wet chemistry approaches and electrochemical methods. Among the wet chemistry approaches for electrode nanostructuring (Eftekhari et al. 2008), nanostructured electrodes are often fabricated by the deposition or coupling of nanoparticles to planar electrodes. For example, AuNPs are commonly deposited on planar electrodes to provide a nanostructured surface for biorecognition element immobilization. In such studies, the particles are bound to the planar electrode via physical adsorption processes (Attar et al. 2016) or chemical methods (Wang et al. 2013). In addition to AuNPs, CNTs have also been extensively investigated as potentially useful nanomaterials for electrode nanostructuring (see Table 1).\nDe Luna et al. found that high-curvature nanostructured Au microelectrodes exhibited a reduced extent of biorecognition element aggregation relative to that found on planar electrodes in DNA sensing studies using a combination of experimental studies and molecular dynamics simulations (see Fig. 3 a) (De Luna et al. 2017; Mahshid et al. 2016). A study by Chin et al. found that nanostructuring of carbon electrodes with carbon nanoparticles enhanced the electron transfer kinetics and current intensity of the electrode by 63% for the detection of Japanese encephalitis virus (Chin et al. 2017).\nFig. 3 Emerging transduction approaches associated with electrochemical biosensors for pathogen detection. a) A nanostructured Au microelectrode array with high curvature (De Luna et al. 2017). b) Cell-imprinted polymer (CIP) with ‘artificial’ biorecognition elements for detection of E. coli using electrochemical impedance spectroscopy (EIS) and the Fe(CN)63-/4- redox probe (Jafari et al. 2019).\nFig. 4 Measurement settings associated with electrochemical biosensor-based multiplexed pathogen detection. a) Microfluidic device with an interdigitated Au microelectrode array for continuous measurement of S. typhimurium (Dastider et al. 2015). b) Conjugated nanoparticles with two different biorecognition elements for E. coli and V. cholerae detection via voltammetry using Fe(CN)63-/4- (Li et al. 2017). c) Schematic of a microfluidic device with two separate spatial regions of biorecognition elements for E. coli and S. aureus (Tian et al. 2016).\nIn addition to fabricating nanostructured electrodes by coupling already processed nanomaterials to planar electrodes, electrochemical methods are also commonly used for bottom-up electrode nanostructuring processes and have been leveraged to fabricate nanostructured electrodes for pathogen detection. For example, Hong et al. fabricated a nanostructured Au electrode via electrochemical deposition of gold (III) chloride hydrates for the detection of norovirus in lettuce extracts (Hong et al. 2015). While the physical or chemical deposition of materials on planar electrodes provides a useful nanostructuring approach, introducing porosity to the electrode, such as nanoporosity, also enables electrode nanostructuring. For example, Nguyen et al. utilized nanoporous alumina-coated Pt microwires for the detection of West Nile virus (Nguyen et al. 2009).\nWhile studies have reported improved biosensor performance using electrode nanostructuring, such as improved sensitivity and LOD, it is prudent to consider the effect of nanostructuring on biorecognition element immobilization and target binding. For example, nanostructured electrodes that exhibit high-aspect-ratio structures and other three-dimensional structures have also been shown to enhance biomolecular steric hindrance effects, which may have implications for pathogen detection applications (Hong et al. 2015; Lam et al. 2012; Mahshid et al. 2017). There also remains a need to understand device-to-device and batch-to-batch variation in electrode nanostructuring quality. For example, it is presently unclear how the structure (e.g., topography, crystal structure) and material properties (e.g., electrical properties) of nanostructured surfaces vary among mass-produced electrodes. It is also unclear how such variance in nanostructuring quality affects the repeatability of biosensor performance."}
LitCovid-PD-HP
{"project":"LitCovid-PD-HP","denotations":[{"id":"T8","span":{"begin":4948,"end":4960},"obj":"Phenotype"}],"attributes":[{"id":"A8","pred":"hp_id","subj":"T8","obj":"http://purl.obolibrary.org/obo/HP_0002383"}],"text":"2.1.5 Electrode nanostructuring\nTransducers with physical dimensions comparable to the target species have been widely investigated as a means of creating sensitive biosensors (Gupta et al. 2004; Pumera et al. 2007; Singh et al. 2010; Wei et al. 2009). Thus, electrodes ranging from micrometers to nanometers have been investigated for pathogen detection. While nanoscale planar electrodes are among the most commonly examined for pathogen detection (Hong et al. 2015; Peh and Li, 2013), the fabrication of nanoscale structures of conducting and semiconducting materials using a wide range of bottom-up and top-down nanomanufacturing processes, such as nanowires, has led to the investigation of nanostructured electrodes for pathogen detection (Patolsky and Lieber, 2005). Nanostructuring can be performed simultaneously with bottom-up electrode fabrication processes or as a post-processing step with top-down electrode fabrication processes.\nNanowire-based electrodes have been fabricated using a variety of engineering materials using both bottom-up and top-down nanomanufacturing processes (Hu et al. 1999; Yogeswaran and Chen, 2008). A detailed review of nanomanufacturing processes for nanowire fabrication can be found elsewhere (Hu et al. 1999). Nanowires can exhibit circular, hexagonal, and even triangular cross-sections. The nanowire aspect ratio, defined as the ratio of the length to width, often ranges from 1 to greater than 10 (Hu et al. 1999; Vaseashta and Dimova-Malinovska, 2005; Wanekaya et al. 2006).\nAs shown in Table 1, metallic and ceramic microwire- and nanowire-based electrodes have been examined for pathogen detection. For example, Wang et al. used nanowire-bundled TiO2 electrodes synthesized using a bottom-up wet chemistry process for the detection of Listeria monocytogenes (L. monocytogenes) (Wang et al. 2008). Shen et al. fabricated silicon nanowire-based electrodes using a chemical vapor deposition process for the rapid detection of human influenza A virus in an array-based format (Shen et al. 2012).\nAlthough polymer nanowires have been relatively more applied to the detection of non-pathogenic species (Travas-Sejdic et al. 2014), there appears to be potential for their application to pathogen detection. Polymer nanowires are also synthesized via bottom-up and top-down nanomanufacturing processes, including hard template methods, soft template methods, or physical approaches, but efficient, large-scale synthesis remains a challenge (Xia et al. 2010). A comprehensive summary of studies using micro- and nano-wire electrodes for pathogen detection is shown in Table 1. For example, Chartuprayoon et al. used Au microelectrode arrays modified with polypyrrole nanoribbons to detect cucumber mosaic virus (Chartuprayoon et al. 2013).\nThe topographical modification of electrode surfaces with micro- and nano-structured features beyond wire-like structures has also been investigated for pathogen detection. Electrode nanostructuring increases the electrode surface area without significantly increasing the electrode volume, thereby increasing the ratio of electrode surface area to fluid volume analyzed (Soleymani et al. 2009). Topographical modification of electrodes can also affect their mechanical and electrical properties. For example, electrochemical deposition of PEDOT on silicon electrodes reduces the electrode electrical impedance across a wide frequency range, which offers measurement advantages for neural monitoring and recording applications (Ludwig et al. 2006).\nElectrode nanostructuring for pathogen detection beyond the fabrication of nanowire-based electrodes has been accomplished primarily using bottom-up wet chemistry approaches and electrochemical methods. Among the wet chemistry approaches for electrode nanostructuring (Eftekhari et al. 2008), nanostructured electrodes are often fabricated by the deposition or coupling of nanoparticles to planar electrodes. For example, AuNPs are commonly deposited on planar electrodes to provide a nanostructured surface for biorecognition element immobilization. In such studies, the particles are bound to the planar electrode via physical adsorption processes (Attar et al. 2016) or chemical methods (Wang et al. 2013). In addition to AuNPs, CNTs have also been extensively investigated as potentially useful nanomaterials for electrode nanostructuring (see Table 1).\nDe Luna et al. found that high-curvature nanostructured Au microelectrodes exhibited a reduced extent of biorecognition element aggregation relative to that found on planar electrodes in DNA sensing studies using a combination of experimental studies and molecular dynamics simulations (see Fig. 3 a) (De Luna et al. 2017; Mahshid et al. 2016). A study by Chin et al. found that nanostructuring of carbon electrodes with carbon nanoparticles enhanced the electron transfer kinetics and current intensity of the electrode by 63% for the detection of Japanese encephalitis virus (Chin et al. 2017).\nFig. 3 Emerging transduction approaches associated with electrochemical biosensors for pathogen detection. a) A nanostructured Au microelectrode array with high curvature (De Luna et al. 2017). b) Cell-imprinted polymer (CIP) with ‘artificial’ biorecognition elements for detection of E. coli using electrochemical impedance spectroscopy (EIS) and the Fe(CN)63-/4- redox probe (Jafari et al. 2019).\nFig. 4 Measurement settings associated with electrochemical biosensor-based multiplexed pathogen detection. a) Microfluidic device with an interdigitated Au microelectrode array for continuous measurement of S. typhimurium (Dastider et al. 2015). b) Conjugated nanoparticles with two different biorecognition elements for E. coli and V. cholerae detection via voltammetry using Fe(CN)63-/4- (Li et al. 2017). c) Schematic of a microfluidic device with two separate spatial regions of biorecognition elements for E. coli and S. aureus (Tian et al. 2016).\nIn addition to fabricating nanostructured electrodes by coupling already processed nanomaterials to planar electrodes, electrochemical methods are also commonly used for bottom-up electrode nanostructuring processes and have been leveraged to fabricate nanostructured electrodes for pathogen detection. For example, Hong et al. fabricated a nanostructured Au electrode via electrochemical deposition of gold (III) chloride hydrates for the detection of norovirus in lettuce extracts (Hong et al. 2015). While the physical or chemical deposition of materials on planar electrodes provides a useful nanostructuring approach, introducing porosity to the electrode, such as nanoporosity, also enables electrode nanostructuring. For example, Nguyen et al. utilized nanoporous alumina-coated Pt microwires for the detection of West Nile virus (Nguyen et al. 2009).\nWhile studies have reported improved biosensor performance using electrode nanostructuring, such as improved sensitivity and LOD, it is prudent to consider the effect of nanostructuring on biorecognition element immobilization and target binding. For example, nanostructured electrodes that exhibit high-aspect-ratio structures and other three-dimensional structures have also been shown to enhance biomolecular steric hindrance effects, which may have implications for pathogen detection applications (Hong et al. 2015; Lam et al. 2012; Mahshid et al. 2017). There also remains a need to understand device-to-device and batch-to-batch variation in electrode nanostructuring quality. For example, it is presently unclear how the structure (e.g., topography, crystal structure) and material properties (e.g., electrical properties) of nanostructured surfaces vary among mass-produced electrodes. It is also unclear how such variance in nanostructuring quality affects the repeatability of biosensor performance."}
LitCovid-sentences
{"project":"LitCovid-sentences","denotations":[{"id":"T365","span":{"begin":0,"end":32},"obj":"Sentence"},{"id":"T366","span":{"begin":33,"end":190},"obj":"Sentence"},{"id":"T367","span":{"begin":191,"end":210},"obj":"Sentence"},{"id":"T368","span":{"begin":211,"end":229},"obj":"Sentence"},{"id":"T369","span":{"begin":230,"end":246},"obj":"Sentence"},{"id":"T370","span":{"begin":247,"end":253},"obj":"Sentence"},{"id":"T371","span":{"begin":254,"end":356},"obj":"Sentence"},{"id":"T372","span":{"begin":357,"end":463},"obj":"Sentence"},{"id":"T373","span":{"begin":464,"end":774},"obj":"Sentence"},{"id":"T374","span":{"begin":775,"end":945},"obj":"Sentence"},{"id":"T375","span":{"begin":946,"end":1106},"obj":"Sentence"},{"id":"T376","span":{"begin":1107,"end":1140},"obj":"Sentence"},{"id":"T377","span":{"begin":1141,"end":1248},"obj":"Sentence"},{"id":"T378","span":{"begin":1249,"end":1255},"obj":"Sentence"},{"id":"T379","span":{"begin":1256,"end":1334},"obj":"Sentence"},{"id":"T380","span":{"begin":1335,"end":1456},"obj":"Sentence"},{"id":"T381","span":{"begin":1457,"end":1517},"obj":"Sentence"},{"id":"T382","span":{"begin":1518,"end":1524},"obj":"Sentence"},{"id":"T383","span":{"begin":1525,"end":1650},"obj":"Sentence"},{"id":"T384","span":{"begin":1651,"end":1841},"obj":"Sentence"},{"id":"T385","span":{"begin":1842,"end":1848},"obj":"Sentence"},{"id":"T386","span":{"begin":1849,"end":2036},"obj":"Sentence"},{"id":"T387","span":{"begin":2037,"end":2043},"obj":"Sentence"},{"id":"T388","span":{"begin":2044,"end":2169},"obj":"Sentence"},{"id":"T389","span":{"begin":2170,"end":2251},"obj":"Sentence"},{"id":"T390","span":{"begin":2252,"end":2495},"obj":"Sentence"},{"id":"T391","span":{"begin":2496,"end":2502},"obj":"Sentence"},{"id":"T392","span":{"begin":2503,"end":2619},"obj":"Sentence"},{"id":"T393","span":{"begin":2620,"end":2775},"obj":"Sentence"},{"id":"T394","span":{"begin":2776,"end":2782},"obj":"Sentence"},{"id":"T395","span":{"begin":2783,"end":2955},"obj":"Sentence"},{"id":"T396","span":{"begin":2956,"end":3171},"obj":"Sentence"},{"id":"T397","span":{"begin":3172,"end":3178},"obj":"Sentence"},{"id":"T398","span":{"begin":3179,"end":3279},"obj":"Sentence"},{"id":"T399","span":{"begin":3280,"end":3524},"obj":"Sentence"},{"id":"T400","span":{"begin":3525,"end":3531},"obj":"Sentence"},{"id":"T401","span":{"begin":3532,"end":3734},"obj":"Sentence"},{"id":"T402","span":{"begin":3735,"end":3817},"obj":"Sentence"},{"id":"T403","span":{"begin":3818,"end":3940},"obj":"Sentence"},{"id":"T404","span":{"begin":3941,"end":4082},"obj":"Sentence"},{"id":"T405","span":{"begin":4083,"end":4195},"obj":"Sentence"},{"id":"T406","span":{"begin":4196,"end":4234},"obj":"Sentence"},{"id":"T407","span":{"begin":4235,"end":4241},"obj":"Sentence"},{"id":"T408","span":{"begin":4242,"end":4389},"obj":"Sentence"},{"id":"T409","span":{"begin":4390,"end":4706},"obj":"Sentence"},{"id":"T410","span":{"begin":4707,"end":4727},"obj":"Sentence"},{"id":"T411","span":{"begin":4728,"end":4734},"obj":"Sentence"},{"id":"T412","span":{"begin":4735,"end":4979},"obj":"Sentence"},{"id":"T413","span":{"begin":4980,"end":4986},"obj":"Sentence"},{"id":"T414","span":{"begin":4987,"end":5173},"obj":"Sentence"},{"id":"T415","span":{"begin":5174,"end":5378},"obj":"Sentence"},{"id":"T416","span":{"begin":5379,"end":5385},"obj":"Sentence"},{"id":"T417","span":{"begin":5386,"end":5625},"obj":"Sentence"},{"id":"T418","span":{"begin":5626,"end":5787},"obj":"Sentence"},{"id":"T419","span":{"begin":5788,"end":5932},"obj":"Sentence"},{"id":"T420","span":{"begin":5933,"end":5939},"obj":"Sentence"},{"id":"T421","span":{"begin":5940,"end":6242},"obj":"Sentence"},{"id":"T422","span":{"begin":6243,"end":6435},"obj":"Sentence"},{"id":"T423","span":{"begin":6436,"end":6442},"obj":"Sentence"},{"id":"T424","span":{"begin":6443,"end":6663},"obj":"Sentence"},{"id":"T425","span":{"begin":6664,"end":6791},"obj":"Sentence"},{"id":"T426","span":{"begin":6792,"end":6798},"obj":"Sentence"},{"id":"T427","span":{"begin":6799,"end":7045},"obj":"Sentence"},{"id":"T428","span":{"begin":7046,"end":7313},"obj":"Sentence"},{"id":"T429","span":{"begin":7314,"end":7330},"obj":"Sentence"},{"id":"T430","span":{"begin":7331,"end":7351},"obj":"Sentence"},{"id":"T431","span":{"begin":7352,"end":7358},"obj":"Sentence"},{"id":"T432","span":{"begin":7359,"end":7482},"obj":"Sentence"},{"id":"T433","span":{"begin":7483,"end":7693},"obj":"Sentence"},{"id":"T434","span":{"begin":7694,"end":7809},"obj":"Sentence"}],"namespaces":[{"prefix":"_base","uri":"http://pubannotation.org/ontology/tao.owl#"}],"text":"2.1.5 Electrode nanostructuring\nTransducers with physical dimensions comparable to the target species have been widely investigated as a means of creating sensitive biosensors (Gupta et al. 2004; Pumera et al. 2007; Singh et al. 2010; Wei et al. 2009). Thus, electrodes ranging from micrometers to nanometers have been investigated for pathogen detection. While nanoscale planar electrodes are among the most commonly examined for pathogen detection (Hong et al. 2015; Peh and Li, 2013), the fabrication of nanoscale structures of conducting and semiconducting materials using a wide range of bottom-up and top-down nanomanufacturing processes, such as nanowires, has led to the investigation of nanostructured electrodes for pathogen detection (Patolsky and Lieber, 2005). Nanostructuring can be performed simultaneously with bottom-up electrode fabrication processes or as a post-processing step with top-down electrode fabrication processes.\nNanowire-based electrodes have been fabricated using a variety of engineering materials using both bottom-up and top-down nanomanufacturing processes (Hu et al. 1999; Yogeswaran and Chen, 2008). A detailed review of nanomanufacturing processes for nanowire fabrication can be found elsewhere (Hu et al. 1999). Nanowires can exhibit circular, hexagonal, and even triangular cross-sections. The nanowire aspect ratio, defined as the ratio of the length to width, often ranges from 1 to greater than 10 (Hu et al. 1999; Vaseashta and Dimova-Malinovska, 2005; Wanekaya et al. 2006).\nAs shown in Table 1, metallic and ceramic microwire- and nanowire-based electrodes have been examined for pathogen detection. For example, Wang et al. used nanowire-bundled TiO2 electrodes synthesized using a bottom-up wet chemistry process for the detection of Listeria monocytogenes (L. monocytogenes) (Wang et al. 2008). Shen et al. fabricated silicon nanowire-based electrodes using a chemical vapor deposition process for the rapid detection of human influenza A virus in an array-based format (Shen et al. 2012).\nAlthough polymer nanowires have been relatively more applied to the detection of non-pathogenic species (Travas-Sejdic et al. 2014), there appears to be potential for their application to pathogen detection. Polymer nanowires are also synthesized via bottom-up and top-down nanomanufacturing processes, including hard template methods, soft template methods, or physical approaches, but efficient, large-scale synthesis remains a challenge (Xia et al. 2010). A comprehensive summary of studies using micro- and nano-wire electrodes for pathogen detection is shown in Table 1. For example, Chartuprayoon et al. used Au microelectrode arrays modified with polypyrrole nanoribbons to detect cucumber mosaic virus (Chartuprayoon et al. 2013).\nThe topographical modification of electrode surfaces with micro- and nano-structured features beyond wire-like structures has also been investigated for pathogen detection. Electrode nanostructuring increases the electrode surface area without significantly increasing the electrode volume, thereby increasing the ratio of electrode surface area to fluid volume analyzed (Soleymani et al. 2009). Topographical modification of electrodes can also affect their mechanical and electrical properties. For example, electrochemical deposition of PEDOT on silicon electrodes reduces the electrode electrical impedance across a wide frequency range, which offers measurement advantages for neural monitoring and recording applications (Ludwig et al. 2006).\nElectrode nanostructuring for pathogen detection beyond the fabrication of nanowire-based electrodes has been accomplished primarily using bottom-up wet chemistry approaches and electrochemical methods. Among the wet chemistry approaches for electrode nanostructuring (Eftekhari et al. 2008), nanostructured electrodes are often fabricated by the deposition or coupling of nanoparticles to planar electrodes. For example, AuNPs are commonly deposited on planar electrodes to provide a nanostructured surface for biorecognition element immobilization. In such studies, the particles are bound to the planar electrode via physical adsorption processes (Attar et al. 2016) or chemical methods (Wang et al. 2013). In addition to AuNPs, CNTs have also been extensively investigated as potentially useful nanomaterials for electrode nanostructuring (see Table 1).\nDe Luna et al. found that high-curvature nanostructured Au microelectrodes exhibited a reduced extent of biorecognition element aggregation relative to that found on planar electrodes in DNA sensing studies using a combination of experimental studies and molecular dynamics simulations (see Fig. 3 a) (De Luna et al. 2017; Mahshid et al. 2016). A study by Chin et al. found that nanostructuring of carbon electrodes with carbon nanoparticles enhanced the electron transfer kinetics and current intensity of the electrode by 63% for the detection of Japanese encephalitis virus (Chin et al. 2017).\nFig. 3 Emerging transduction approaches associated with electrochemical biosensors for pathogen detection. a) A nanostructured Au microelectrode array with high curvature (De Luna et al. 2017). b) Cell-imprinted polymer (CIP) with ‘artificial’ biorecognition elements for detection of E. coli using electrochemical impedance spectroscopy (EIS) and the Fe(CN)63-/4- redox probe (Jafari et al. 2019).\nFig. 4 Measurement settings associated with electrochemical biosensor-based multiplexed pathogen detection. a) Microfluidic device with an interdigitated Au microelectrode array for continuous measurement of S. typhimurium (Dastider et al. 2015). b) Conjugated nanoparticles with two different biorecognition elements for E. coli and V. cholerae detection via voltammetry using Fe(CN)63-/4- (Li et al. 2017). c) Schematic of a microfluidic device with two separate spatial regions of biorecognition elements for E. coli and S. aureus (Tian et al. 2016).\nIn addition to fabricating nanostructured electrodes by coupling already processed nanomaterials to planar electrodes, electrochemical methods are also commonly used for bottom-up electrode nanostructuring processes and have been leveraged to fabricate nanostructured electrodes for pathogen detection. For example, Hong et al. fabricated a nanostructured Au electrode via electrochemical deposition of gold (III) chloride hydrates for the detection of norovirus in lettuce extracts (Hong et al. 2015). While the physical or chemical deposition of materials on planar electrodes provides a useful nanostructuring approach, introducing porosity to the electrode, such as nanoporosity, also enables electrode nanostructuring. For example, Nguyen et al. utilized nanoporous alumina-coated Pt microwires for the detection of West Nile virus (Nguyen et al. 2009).\nWhile studies have reported improved biosensor performance using electrode nanostructuring, such as improved sensitivity and LOD, it is prudent to consider the effect of nanostructuring on biorecognition element immobilization and target binding. For example, nanostructured electrodes that exhibit high-aspect-ratio structures and other three-dimensional structures have also been shown to enhance biomolecular steric hindrance effects, which may have implications for pathogen detection applications (Hong et al. 2015; Lam et al. 2012; Mahshid et al. 2017). There also remains a need to understand device-to-device and batch-to-batch variation in electrode nanostructuring quality. For example, it is presently unclear how the structure (e.g., topography, crystal structure) and material properties (e.g., electrical properties) of nanostructured surfaces vary among mass-produced electrodes. It is also unclear how such variance in nanostructuring quality affects the repeatability of biosensor performance."}
2_test
{"project":"2_test","denotations":[{"id":"32364936-20419506-7713035","span":{"begin":230,"end":234},"obj":"20419506"},{"id":"32364936-19606287-7713036","span":{"begin":247,"end":251},"obj":"19606287"},{"id":"32364936-25254625-7713037","span":{"begin":464,"end":468},"obj":"25254625"},{"id":"32364936-23220066-7713038","span":{"begin":482,"end":486},"obj":"23220066"},{"id":"32364936-27879709-7713039","span":{"begin":1134,"end":1138},"obj":"27879709"},{"id":"32364936-18715043-7713040","span":{"begin":1842,"end":1846},"obj":"18715043"},{"id":"32364936-22731392-7713041","span":{"begin":2037,"end":2041},"obj":"22731392"},{"id":"32364936-32262272-7713042","span":{"begin":2170,"end":2174},"obj":"32262272"},{"id":"32364936-19837415-7713043","span":{"begin":2496,"end":2500},"obj":"19837415"},{"id":"32364936-19893517-7713044","span":{"begin":3172,"end":3176},"obj":"19893517"},{"id":"32364936-16510943-7713045","span":{"begin":3525,"end":3529},"obj":"16510943"},{"id":"32364936-23811484-7713046","span":{"begin":4235,"end":4239},"obj":"23811484"},{"id":"32364936-28075594-7713047","span":{"begin":4707,"end":4711},"obj":"28075594"},{"id":"32364936-28075594-7713048","span":{"begin":5174,"end":5178},"obj":"28075594"},{"id":"32364936-28382165-7713049","span":{"begin":5788,"end":5792},"obj":"28382165"},{"id":"32364936-25254625-7713050","span":{"begin":6436,"end":6440},"obj":"25254625"},{"id":"32364936-19663392-7713051","span":{"begin":6792,"end":6796},"obj":"19663392"},{"id":"32364936-25254625-7713052","span":{"begin":7314,"end":7318},"obj":"25254625"},{"id":"32364936-22142422-7713053","span":{"begin":7331,"end":7335},"obj":"22142422"},{"id":"32364936-28829912-7713054","span":{"begin":7352,"end":7356},"obj":"28829912"}],"text":"2.1.5 Electrode nanostructuring\nTransducers with physical dimensions comparable to the target species have been widely investigated as a means of creating sensitive biosensors (Gupta et al. 2004; Pumera et al. 2007; Singh et al. 2010; Wei et al. 2009). Thus, electrodes ranging from micrometers to nanometers have been investigated for pathogen detection. While nanoscale planar electrodes are among the most commonly examined for pathogen detection (Hong et al. 2015; Peh and Li, 2013), the fabrication of nanoscale structures of conducting and semiconducting materials using a wide range of bottom-up and top-down nanomanufacturing processes, such as nanowires, has led to the investigation of nanostructured electrodes for pathogen detection (Patolsky and Lieber, 2005). Nanostructuring can be performed simultaneously with bottom-up electrode fabrication processes or as a post-processing step with top-down electrode fabrication processes.\nNanowire-based electrodes have been fabricated using a variety of engineering materials using both bottom-up and top-down nanomanufacturing processes (Hu et al. 1999; Yogeswaran and Chen, 2008). A detailed review of nanomanufacturing processes for nanowire fabrication can be found elsewhere (Hu et al. 1999). Nanowires can exhibit circular, hexagonal, and even triangular cross-sections. The nanowire aspect ratio, defined as the ratio of the length to width, often ranges from 1 to greater than 10 (Hu et al. 1999; Vaseashta and Dimova-Malinovska, 2005; Wanekaya et al. 2006).\nAs shown in Table 1, metallic and ceramic microwire- and nanowire-based electrodes have been examined for pathogen detection. For example, Wang et al. used nanowire-bundled TiO2 electrodes synthesized using a bottom-up wet chemistry process for the detection of Listeria monocytogenes (L. monocytogenes) (Wang et al. 2008). Shen et al. fabricated silicon nanowire-based electrodes using a chemical vapor deposition process for the rapid detection of human influenza A virus in an array-based format (Shen et al. 2012).\nAlthough polymer nanowires have been relatively more applied to the detection of non-pathogenic species (Travas-Sejdic et al. 2014), there appears to be potential for their application to pathogen detection. Polymer nanowires are also synthesized via bottom-up and top-down nanomanufacturing processes, including hard template methods, soft template methods, or physical approaches, but efficient, large-scale synthesis remains a challenge (Xia et al. 2010). A comprehensive summary of studies using micro- and nano-wire electrodes for pathogen detection is shown in Table 1. For example, Chartuprayoon et al. used Au microelectrode arrays modified with polypyrrole nanoribbons to detect cucumber mosaic virus (Chartuprayoon et al. 2013).\nThe topographical modification of electrode surfaces with micro- and nano-structured features beyond wire-like structures has also been investigated for pathogen detection. Electrode nanostructuring increases the electrode surface area without significantly increasing the electrode volume, thereby increasing the ratio of electrode surface area to fluid volume analyzed (Soleymani et al. 2009). Topographical modification of electrodes can also affect their mechanical and electrical properties. For example, electrochemical deposition of PEDOT on silicon electrodes reduces the electrode electrical impedance across a wide frequency range, which offers measurement advantages for neural monitoring and recording applications (Ludwig et al. 2006).\nElectrode nanostructuring for pathogen detection beyond the fabrication of nanowire-based electrodes has been accomplished primarily using bottom-up wet chemistry approaches and electrochemical methods. Among the wet chemistry approaches for electrode nanostructuring (Eftekhari et al. 2008), nanostructured electrodes are often fabricated by the deposition or coupling of nanoparticles to planar electrodes. For example, AuNPs are commonly deposited on planar electrodes to provide a nanostructured surface for biorecognition element immobilization. In such studies, the particles are bound to the planar electrode via physical adsorption processes (Attar et al. 2016) or chemical methods (Wang et al. 2013). In addition to AuNPs, CNTs have also been extensively investigated as potentially useful nanomaterials for electrode nanostructuring (see Table 1).\nDe Luna et al. found that high-curvature nanostructured Au microelectrodes exhibited a reduced extent of biorecognition element aggregation relative to that found on planar electrodes in DNA sensing studies using a combination of experimental studies and molecular dynamics simulations (see Fig. 3 a) (De Luna et al. 2017; Mahshid et al. 2016). A study by Chin et al. found that nanostructuring of carbon electrodes with carbon nanoparticles enhanced the electron transfer kinetics and current intensity of the electrode by 63% for the detection of Japanese encephalitis virus (Chin et al. 2017).\nFig. 3 Emerging transduction approaches associated with electrochemical biosensors for pathogen detection. a) A nanostructured Au microelectrode array with high curvature (De Luna et al. 2017). b) Cell-imprinted polymer (CIP) with ‘artificial’ biorecognition elements for detection of E. coli using electrochemical impedance spectroscopy (EIS) and the Fe(CN)63-/4- redox probe (Jafari et al. 2019).\nFig. 4 Measurement settings associated with electrochemical biosensor-based multiplexed pathogen detection. a) Microfluidic device with an interdigitated Au microelectrode array for continuous measurement of S. typhimurium (Dastider et al. 2015). b) Conjugated nanoparticles with two different biorecognition elements for E. coli and V. cholerae detection via voltammetry using Fe(CN)63-/4- (Li et al. 2017). c) Schematic of a microfluidic device with two separate spatial regions of biorecognition elements for E. coli and S. aureus (Tian et al. 2016).\nIn addition to fabricating nanostructured electrodes by coupling already processed nanomaterials to planar electrodes, electrochemical methods are also commonly used for bottom-up electrode nanostructuring processes and have been leveraged to fabricate nanostructured electrodes for pathogen detection. For example, Hong et al. fabricated a nanostructured Au electrode via electrochemical deposition of gold (III) chloride hydrates for the detection of norovirus in lettuce extracts (Hong et al. 2015). While the physical or chemical deposition of materials on planar electrodes provides a useful nanostructuring approach, introducing porosity to the electrode, such as nanoporosity, also enables electrode nanostructuring. For example, Nguyen et al. utilized nanoporous alumina-coated Pt microwires for the detection of West Nile virus (Nguyen et al. 2009).\nWhile studies have reported improved biosensor performance using electrode nanostructuring, such as improved sensitivity and LOD, it is prudent to consider the effect of nanostructuring on biorecognition element immobilization and target binding. For example, nanostructured electrodes that exhibit high-aspect-ratio structures and other three-dimensional structures have also been shown to enhance biomolecular steric hindrance effects, which may have implications for pathogen detection applications (Hong et al. 2015; Lam et al. 2012; Mahshid et al. 2017). There also remains a need to understand device-to-device and batch-to-batch variation in electrode nanostructuring quality. For example, it is presently unclear how the structure (e.g., topography, crystal structure) and material properties (e.g., electrical properties) of nanostructured surfaces vary among mass-produced electrodes. It is also unclear how such variance in nanostructuring quality affects the repeatability of biosensor performance."}