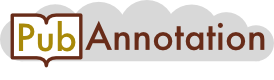
PMC:7152911 / 1695-10682
Annnotations
LitCovid-PubTator
{"project":"LitCovid-PubTator","denotations":[{"id":"11","span":{"begin":455,"end":470},"obj":"Species"},{"id":"12","span":{"begin":494,"end":501},"obj":"Species"},{"id":"13","span":{"begin":506,"end":515},"obj":"Species"},{"id":"14","span":{"begin":708,"end":722},"obj":"Species"},{"id":"15","span":{"begin":293,"end":302},"obj":"Disease"},{"id":"16","span":{"begin":343,"end":349},"obj":"Disease"},{"id":"17","span":{"begin":951,"end":970},"obj":"Disease"},{"id":"23","span":{"begin":1814,"end":1821},"obj":"Species"},{"id":"24","span":{"begin":1806,"end":1813},"obj":"Species"},{"id":"25","span":{"begin":1233,"end":1242},"obj":"Disease"},{"id":"26","span":{"begin":1611,"end":1620},"obj":"Disease"},{"id":"27","span":{"begin":2148,"end":2158},"obj":"Disease"},{"id":"29","span":{"begin":5925,"end":5937},"obj":"Disease"},{"id":"31","span":{"begin":7986,"end":7991},"obj":"Chemical"}],"attributes":[{"id":"A11","pred":"tao:has_database_id","subj":"11","obj":"Tax:11309"},{"id":"A12","pred":"tao:has_database_id","subj":"12","obj":"Tax:562"},{"id":"A13","pred":"tao:has_database_id","subj":"13","obj":"Tax:1280"},{"id":"A14","pred":"tao:has_database_id","subj":"14","obj":"Tax:2697049"},{"id":"A15","pred":"tao:has_database_id","subj":"15","obj":"MESH:D007239"},{"id":"A16","pred":"tao:has_database_id","subj":"16","obj":"MESH:D003643"},{"id":"A17","pred":"tao:has_database_id","subj":"17","obj":"MESH:D003141"},{"id":"A23","pred":"tao:has_database_id","subj":"23","obj":"Tax:83334"},{"id":"A24","pred":"tao:has_database_id","subj":"24","obj":"Tax:562"},{"id":"A25","pred":"tao:has_database_id","subj":"25","obj":"MESH:D007239"},{"id":"A26","pred":"tao:has_database_id","subj":"26","obj":"MESH:D007239"},{"id":"A27","pred":"tao:has_database_id","subj":"27","obj":"MESH:D007239"},{"id":"A29","pred":"tao:has_database_id","subj":"29","obj":"MESH:D019965"},{"id":"A31","pred":"tao:has_database_id","subj":"31","obj":"MESH:D014867"}],"namespaces":[{"prefix":"Tax","uri":"https://www.ncbi.nlm.nih.gov/taxonomy/"},{"prefix":"MESH","uri":"https://id.nlm.nih.gov/mesh/"},{"prefix":"Gene","uri":"https://www.ncbi.nlm.nih.gov/gene/"},{"prefix":"CVCL","uri":"https://web.expasy.org/cellosaurus/CVCL_"}],"text":"1 Introduction\nPathogens are infectious agents that cause disease. They include microorganisms, such as fungi, protozoans, and bacteria, and molecular-scale infectious agents, including viruses and prions. Foodborne, waterborne, and airborne pathogens enter the body through various modes of infection and are responsible for over 15 million deaths annually worldwide (Dye, 2014). Some of the most common pathogens include viruses, such as norovirus and influenza virus, and bacteria, such as E. coli and S. aureus. Pathogens vary in many regards, such as virulence, contagiousness, mode of transmission, and infectious dose. For example, the world is currently facing a global pandemic associated with the COVID-19 virus, for which virulence and infectious dose data are still emerging. Techniques for sensitive and rapid detection of pathogens in complex matrices, such as body fluids and aerosols, and on surfaces are critical to the treatment of infectious diseases and controlling the spread of disease.\nThe techniques used to identify and quantify pathogens can be broadly distinguished as immunoassays or DNA-based assays. The use of immunoassays versus DNA-based assays depends on various factors, including the stage of an infection and the availability of antibodies and DNA sequence data, such as viral DNA, toxin-producing genes, as well as species- and strain-selective genes. Immunoassays are ubiquitous across medical diagnostics and food safety applications. Pathogens can be identified through the presence of generated antibodies in an organism, which may be present both during and after an infection (i.e., after the pathogen is no longer present). In such assays, both the biorecognition element and the target are antibodies. If antibodies are available for the pathogen (e.g., anti-E. coli O157:H7), one can also directly detect the pathogen using immunoassays. The ability to indirectly and directly detect pathogens via generated antibodies and pathogen epitopes, respectively, makes immunoassays flexible techniques for pathogen detection. In cases of limited antibody availability, need for highly sensitive results, or infections that do not generate a significant level of antibody production in the organism although the pathogen is present, DNA-based assays are commonly employed. DNA-based assays require the pathogen to be present in the sample or to have been recently present. In addition to detection of pathogens using antibodies or toxin-producing genes, pathogens can also be detected based on their expression of toxins. Thus, targets associated with pathogen detection include toxins, nucleic acids, viruses, cells, and oocysts. As a result, biorecognition elements widely vary, including antibodies, aptamers, and imprinted polymers. Several comprehensive reviews have been written on pathogen detection using high-throughput, well plate-based bioanalytical techniques (Alahi and Mukhopadhyay, 2017; Lazcka et al. 2007; Zourob et al. 2008), such as enzyme-linked immunosorbent assay (ELISA) (Law et al. 2015) and polymerase chain reaction (PCR) (Klein, 2002; Malorny et al. 2003), which remain the gold standards for pathogen detection. Few reviews, however, have focused on emerging label-free biosensors for pathogen detection, which provide useful characteristics for applications in process monitoring (e.g., of biomanufacturing processes), environmental monitoring, and precision agriculture.\nBioanalytical techniques utilize a selective biorecognition element, often called a molecular probe, in combination with an analytical system, such as a plate reader or PCR analyzer, to quantify one or more components of a sample. While capable of being highly sensitive and robust, they are destructive testing methods and require the addition of reagents to the sample and extensive sample preparation steps, which increase the time-to-results (TTR). Bioanalytical techniques, such as PCR, may also encounter inhibition effects caused by background species in the sample (Justino et al. 2017; Scognamiglio et al. 2016; Sin et al. 2014), which introduce measurement bias and increase measurement uncertainty (Clark et al. 2016; Silverman et al. 2019). Considering such limitations of traditional plate-based bioanalytical techniques and the need for real-time continuous monitoring capabilities among various applications, there is a need to examine alternative bioanalytical techniques.\nOver the past twenty-five years, biosensors have emerged to complement PCR and ELISA for pathogen detection. Biosensors are based on the direct integration of a selective biorecognition element and a sensitive transducer element and provide complementary platforms to PCR and ELISA for pathogen identification and quantification. According to the International Union of Pure and Applied Chemistry (IUPAC), a biosensor must contain a biorecognition element in direct spatial contact with a transduction element (Thévenot et al. 2001). In addition, a biosensor should provide quantitative or semi-quantitative analytical information and measurement without the requirement of additional processing steps or reagents. While a biosensor should also be a self-contained, integrated device, the measurement approach can vary from droplet formats to continuous flow formats that require associated fluid handling systems. Biosensors have achieved sensitive and selective real-time detection of pathogens in various environments without the need for sample preparation. For example, biosensors have enabled the detection of an abundance of pathogens in various matrices and environments, including foods, body fluids, and object surfaces. In addition to sample preparation-free protocols, biosensors are compatible with label-free protocols (Daniels and Pourmand, 2007; Rapp et al. 2010; Sang et al. 2016; Vestergaard et al. 2007). Labels, often referred to as reporters, are molecular species, such as organic dyes or quantum dots (Resch-Genger et al. 2008), that are attached to the target, either directly or through a biorecognition element, using a series of sample preparation steps or secondary binding steps to facilitate detection through the properties of the label. Thus, label-free biosensors avoid the use of a reporter species to detect the target species (Cooper, 2009; Syahir et al. 2015). Label-free assays often have fewer sample preparation steps due to the elimination of procedures associated with target labeling and lower cost than label-based assays, which are important considerations for applications in which preparation facilities or trained personnel are either limited or unavailable (Cooper, 2009; Syahir et al. 2015).\nWhile various types of transducers have been investigated for pathogen biosensing (Lazcka et al. 2007; Singh et al. 2014; Yoo and Lee, 2016), including mechanical and optical transducers, such as cantilever biosensors or surface plasmon resonance (SPR)-based biosensors, electrochemical biosensors have been extensively applied to pathogen detection (Felix and Angnes, 2018; Pereira da Silva Neves et al. 2018; Saucedo et al. 2019). Electrochemical biosensors for pathogen detection utilize conducting and semiconducting materials as the transducer, which is commonly referred to as an electrode. The chemical energy associated with binding between target pathogens and electrode-immobilized biorecognition elements is converted into electrical energy through an electrochemical method that involves the electrode and a pathogen-containing electrolyte solution. To date, electrochemical biosensors have enabled sample preparation-free detection of pathogens in various matrices, in situ detection of pathogens on surfaces, rapid pathogen detection using low-cost platforms, multiplexed detection of pathogens in practical matrices, and detection of pathogens via wireless actuation and data acquisition formats. As a result, electrochemical biosensors for pathogen detection have been widely examined for food and water safety, medical diagnostic, environmental monitoring, and bio-threat applications (Amiri et al. 2018; Duffy and Moore, 2017; Felix and Angnes, 2018; Furst and Francis, 2019; Mishra et al. 2018; Monzó et al. 2015; Rastogi and Singh, 2019).\nHere, we critically review electrochemical biosensors for pathogen detection. To gain insight into the trajectory of the field, electrochemical biosensors for pathogen detection reported since 2005 are critically reviewed and classified with respect to IUPAC-recommended definitions and classifications (Thévenot et al. 2001). Applications of electrochemical biosensors for pathogen detection are critically reviewed with respect to the target pathogen, sample matrix, biosensor design, fabrication method, measurement format, and biosensor performance. We also discuss future directions of electrochemical biosensors for pathogen detection, which includes a discussion of present technological and methodological challenges and emerging application areas."}
LitCovid-PD-FMA-UBERON
{"project":"LitCovid-PD-FMA-UBERON","denotations":[{"id":"T22063","span":{"begin":263,"end":267},"obj":"Body_part"},{"id":"T33096","span":{"begin":876,"end":887},"obj":"Body_part"},{"id":"T10582","span":{"begin":876,"end":880},"obj":"Body_part"},{"id":"T19835","span":{"begin":1113,"end":1116},"obj":"Body_part"},{"id":"T55984","span":{"begin":1162,"end":1165},"obj":"Body_part"},{"id":"T20931","span":{"begin":1282,"end":1285},"obj":"Body_part"},{"id":"T41986","span":{"begin":1315,"end":1318},"obj":"Body_part"},{"id":"T2928","span":{"begin":2087,"end":2095},"obj":"Body_part"},{"id":"T4405","span":{"begin":2203,"end":2211},"obj":"Body_part"},{"id":"T32223","span":{"begin":2273,"end":2276},"obj":"Body_part"},{"id":"T61707","span":{"begin":2313,"end":2316},"obj":"Body_part"},{"id":"T51330","span":{"begin":2651,"end":2656},"obj":"Body_part"},{"id":"T19578","span":{"begin":5627,"end":5638},"obj":"Body_part"},{"id":"T23928","span":{"begin":5627,"end":5631},"obj":"Body_part"}],"attributes":[{"id":"A77238","pred":"fma_id","subj":"T22063","obj":"http://purl.org/sig/ont/fma/fma256135"},{"id":"A93903","pred":"fma_id","subj":"T33096","obj":"http://purl.org/sig/ont/fma/fma280556"},{"id":"A83207","pred":"fma_id","subj":"T10582","obj":"http://purl.org/sig/ont/fma/fma256135"},{"id":"A86288","pred":"fma_id","subj":"T19835","obj":"http://purl.org/sig/ont/fma/fma74412"},{"id":"A12665","pred":"fma_id","subj":"T55984","obj":"http://purl.org/sig/ont/fma/fma74412"},{"id":"A6183","pred":"fma_id","subj":"T20931","obj":"http://purl.org/sig/ont/fma/fma74412"},{"id":"A6414","pred":"fma_id","subj":"T41986","obj":"http://purl.org/sig/ont/fma/fma74412"},{"id":"A38311","pred":"fma_id","subj":"T2928","obj":"http://purl.org/sig/ont/fma/fma62871"},{"id":"A23588","pred":"fma_id","subj":"T4405","obj":"http://purl.org/sig/ont/fma/fma62871"},{"id":"A13343","pred":"fma_id","subj":"T32223","obj":"http://purl.org/sig/ont/fma/fma74412"},{"id":"A9814","pred":"fma_id","subj":"T61707","obj":"http://purl.org/sig/ont/fma/fma74412"},{"id":"A15342","pred":"fma_id","subj":"T51330","obj":"http://purl.org/sig/ont/fma/fma68646"},{"id":"A91539","pred":"fma_id","subj":"T19578","obj":"http://purl.org/sig/ont/fma/fma280556"},{"id":"A56795","pred":"fma_id","subj":"T23928","obj":"http://purl.org/sig/ont/fma/fma256135"}],"text":"1 Introduction\nPathogens are infectious agents that cause disease. They include microorganisms, such as fungi, protozoans, and bacteria, and molecular-scale infectious agents, including viruses and prions. Foodborne, waterborne, and airborne pathogens enter the body through various modes of infection and are responsible for over 15 million deaths annually worldwide (Dye, 2014). Some of the most common pathogens include viruses, such as norovirus and influenza virus, and bacteria, such as E. coli and S. aureus. Pathogens vary in many regards, such as virulence, contagiousness, mode of transmission, and infectious dose. For example, the world is currently facing a global pandemic associated with the COVID-19 virus, for which virulence and infectious dose data are still emerging. Techniques for sensitive and rapid detection of pathogens in complex matrices, such as body fluids and aerosols, and on surfaces are critical to the treatment of infectious diseases and controlling the spread of disease.\nThe techniques used to identify and quantify pathogens can be broadly distinguished as immunoassays or DNA-based assays. The use of immunoassays versus DNA-based assays depends on various factors, including the stage of an infection and the availability of antibodies and DNA sequence data, such as viral DNA, toxin-producing genes, as well as species- and strain-selective genes. Immunoassays are ubiquitous across medical diagnostics and food safety applications. Pathogens can be identified through the presence of generated antibodies in an organism, which may be present both during and after an infection (i.e., after the pathogen is no longer present). In such assays, both the biorecognition element and the target are antibodies. If antibodies are available for the pathogen (e.g., anti-E. coli O157:H7), one can also directly detect the pathogen using immunoassays. The ability to indirectly and directly detect pathogens via generated antibodies and pathogen epitopes, respectively, makes immunoassays flexible techniques for pathogen detection. In cases of limited antibody availability, need for highly sensitive results, or infections that do not generate a significant level of antibody production in the organism although the pathogen is present, DNA-based assays are commonly employed. DNA-based assays require the pathogen to be present in the sample or to have been recently present. In addition to detection of pathogens using antibodies or toxin-producing genes, pathogens can also be detected based on their expression of toxins. Thus, targets associated with pathogen detection include toxins, nucleic acids, viruses, cells, and oocysts. As a result, biorecognition elements widely vary, including antibodies, aptamers, and imprinted polymers. Several comprehensive reviews have been written on pathogen detection using high-throughput, well plate-based bioanalytical techniques (Alahi and Mukhopadhyay, 2017; Lazcka et al. 2007; Zourob et al. 2008), such as enzyme-linked immunosorbent assay (ELISA) (Law et al. 2015) and polymerase chain reaction (PCR) (Klein, 2002; Malorny et al. 2003), which remain the gold standards for pathogen detection. Few reviews, however, have focused on emerging label-free biosensors for pathogen detection, which provide useful characteristics for applications in process monitoring (e.g., of biomanufacturing processes), environmental monitoring, and precision agriculture.\nBioanalytical techniques utilize a selective biorecognition element, often called a molecular probe, in combination with an analytical system, such as a plate reader or PCR analyzer, to quantify one or more components of a sample. While capable of being highly sensitive and robust, they are destructive testing methods and require the addition of reagents to the sample and extensive sample preparation steps, which increase the time-to-results (TTR). Bioanalytical techniques, such as PCR, may also encounter inhibition effects caused by background species in the sample (Justino et al. 2017; Scognamiglio et al. 2016; Sin et al. 2014), which introduce measurement bias and increase measurement uncertainty (Clark et al. 2016; Silverman et al. 2019). Considering such limitations of traditional plate-based bioanalytical techniques and the need for real-time continuous monitoring capabilities among various applications, there is a need to examine alternative bioanalytical techniques.\nOver the past twenty-five years, biosensors have emerged to complement PCR and ELISA for pathogen detection. Biosensors are based on the direct integration of a selective biorecognition element and a sensitive transducer element and provide complementary platforms to PCR and ELISA for pathogen identification and quantification. According to the International Union of Pure and Applied Chemistry (IUPAC), a biosensor must contain a biorecognition element in direct spatial contact with a transduction element (Thévenot et al. 2001). In addition, a biosensor should provide quantitative or semi-quantitative analytical information and measurement without the requirement of additional processing steps or reagents. While a biosensor should also be a self-contained, integrated device, the measurement approach can vary from droplet formats to continuous flow formats that require associated fluid handling systems. Biosensors have achieved sensitive and selective real-time detection of pathogens in various environments without the need for sample preparation. For example, biosensors have enabled the detection of an abundance of pathogens in various matrices and environments, including foods, body fluids, and object surfaces. In addition to sample preparation-free protocols, biosensors are compatible with label-free protocols (Daniels and Pourmand, 2007; Rapp et al. 2010; Sang et al. 2016; Vestergaard et al. 2007). Labels, often referred to as reporters, are molecular species, such as organic dyes or quantum dots (Resch-Genger et al. 2008), that are attached to the target, either directly or through a biorecognition element, using a series of sample preparation steps or secondary binding steps to facilitate detection through the properties of the label. Thus, label-free biosensors avoid the use of a reporter species to detect the target species (Cooper, 2009; Syahir et al. 2015). Label-free assays often have fewer sample preparation steps due to the elimination of procedures associated with target labeling and lower cost than label-based assays, which are important considerations for applications in which preparation facilities or trained personnel are either limited or unavailable (Cooper, 2009; Syahir et al. 2015).\nWhile various types of transducers have been investigated for pathogen biosensing (Lazcka et al. 2007; Singh et al. 2014; Yoo and Lee, 2016), including mechanical and optical transducers, such as cantilever biosensors or surface plasmon resonance (SPR)-based biosensors, electrochemical biosensors have been extensively applied to pathogen detection (Felix and Angnes, 2018; Pereira da Silva Neves et al. 2018; Saucedo et al. 2019). Electrochemical biosensors for pathogen detection utilize conducting and semiconducting materials as the transducer, which is commonly referred to as an electrode. The chemical energy associated with binding between target pathogens and electrode-immobilized biorecognition elements is converted into electrical energy through an electrochemical method that involves the electrode and a pathogen-containing electrolyte solution. To date, electrochemical biosensors have enabled sample preparation-free detection of pathogens in various matrices, in situ detection of pathogens on surfaces, rapid pathogen detection using low-cost platforms, multiplexed detection of pathogens in practical matrices, and detection of pathogens via wireless actuation and data acquisition formats. As a result, electrochemical biosensors for pathogen detection have been widely examined for food and water safety, medical diagnostic, environmental monitoring, and bio-threat applications (Amiri et al. 2018; Duffy and Moore, 2017; Felix and Angnes, 2018; Furst and Francis, 2019; Mishra et al. 2018; Monzó et al. 2015; Rastogi and Singh, 2019).\nHere, we critically review electrochemical biosensors for pathogen detection. To gain insight into the trajectory of the field, electrochemical biosensors for pathogen detection reported since 2005 are critically reviewed and classified with respect to IUPAC-recommended definitions and classifications (Thévenot et al. 2001). Applications of electrochemical biosensors for pathogen detection are critically reviewed with respect to the target pathogen, sample matrix, biosensor design, fabrication method, measurement format, and biosensor performance. We also discuss future directions of electrochemical biosensors for pathogen detection, which includes a discussion of present technological and methodological challenges and emerging application areas."}
LitCovid-PD-UBERON
{"project":"LitCovid-PD-UBERON","denotations":[{"id":"T1","span":{"begin":152,"end":157},"obj":"Body_part"}],"attributes":[{"id":"A1","pred":"uberon_id","subj":"T1","obj":"http://purl.obolibrary.org/obo/UBERON_0002542"}],"text":"1 Introduction\nPathogens are infectious agents that cause disease. They include microorganisms, such as fungi, protozoans, and bacteria, and molecular-scale infectious agents, including viruses and prions. Foodborne, waterborne, and airborne pathogens enter the body through various modes of infection and are responsible for over 15 million deaths annually worldwide (Dye, 2014). Some of the most common pathogens include viruses, such as norovirus and influenza virus, and bacteria, such as E. coli and S. aureus. Pathogens vary in many regards, such as virulence, contagiousness, mode of transmission, and infectious dose. For example, the world is currently facing a global pandemic associated with the COVID-19 virus, for which virulence and infectious dose data are still emerging. Techniques for sensitive and rapid detection of pathogens in complex matrices, such as body fluids and aerosols, and on surfaces are critical to the treatment of infectious diseases and controlling the spread of disease.\nThe techniques used to identify and quantify pathogens can be broadly distinguished as immunoassays or DNA-based assays. The use of immunoassays versus DNA-based assays depends on various factors, including the stage of an infection and the availability of antibodies and DNA sequence data, such as viral DNA, toxin-producing genes, as well as species- and strain-selective genes. Immunoassays are ubiquitous across medical diagnostics and food safety applications. Pathogens can be identified through the presence of generated antibodies in an organism, which may be present both during and after an infection (i.e., after the pathogen is no longer present). In such assays, both the biorecognition element and the target are antibodies. If antibodies are available for the pathogen (e.g., anti-E. coli O157:H7), one can also directly detect the pathogen using immunoassays. The ability to indirectly and directly detect pathogens via generated antibodies and pathogen epitopes, respectively, makes immunoassays flexible techniques for pathogen detection. In cases of limited antibody availability, need for highly sensitive results, or infections that do not generate a significant level of antibody production in the organism although the pathogen is present, DNA-based assays are commonly employed. DNA-based assays require the pathogen to be present in the sample or to have been recently present. In addition to detection of pathogens using antibodies or toxin-producing genes, pathogens can also be detected based on their expression of toxins. Thus, targets associated with pathogen detection include toxins, nucleic acids, viruses, cells, and oocysts. As a result, biorecognition elements widely vary, including antibodies, aptamers, and imprinted polymers. Several comprehensive reviews have been written on pathogen detection using high-throughput, well plate-based bioanalytical techniques (Alahi and Mukhopadhyay, 2017; Lazcka et al. 2007; Zourob et al. 2008), such as enzyme-linked immunosorbent assay (ELISA) (Law et al. 2015) and polymerase chain reaction (PCR) (Klein, 2002; Malorny et al. 2003), which remain the gold standards for pathogen detection. Few reviews, however, have focused on emerging label-free biosensors for pathogen detection, which provide useful characteristics for applications in process monitoring (e.g., of biomanufacturing processes), environmental monitoring, and precision agriculture.\nBioanalytical techniques utilize a selective biorecognition element, often called a molecular probe, in combination with an analytical system, such as a plate reader or PCR analyzer, to quantify one or more components of a sample. While capable of being highly sensitive and robust, they are destructive testing methods and require the addition of reagents to the sample and extensive sample preparation steps, which increase the time-to-results (TTR). Bioanalytical techniques, such as PCR, may also encounter inhibition effects caused by background species in the sample (Justino et al. 2017; Scognamiglio et al. 2016; Sin et al. 2014), which introduce measurement bias and increase measurement uncertainty (Clark et al. 2016; Silverman et al. 2019). Considering such limitations of traditional plate-based bioanalytical techniques and the need for real-time continuous monitoring capabilities among various applications, there is a need to examine alternative bioanalytical techniques.\nOver the past twenty-five years, biosensors have emerged to complement PCR and ELISA for pathogen detection. Biosensors are based on the direct integration of a selective biorecognition element and a sensitive transducer element and provide complementary platforms to PCR and ELISA for pathogen identification and quantification. According to the International Union of Pure and Applied Chemistry (IUPAC), a biosensor must contain a biorecognition element in direct spatial contact with a transduction element (Thévenot et al. 2001). In addition, a biosensor should provide quantitative or semi-quantitative analytical information and measurement without the requirement of additional processing steps or reagents. While a biosensor should also be a self-contained, integrated device, the measurement approach can vary from droplet formats to continuous flow formats that require associated fluid handling systems. Biosensors have achieved sensitive and selective real-time detection of pathogens in various environments without the need for sample preparation. For example, biosensors have enabled the detection of an abundance of pathogens in various matrices and environments, including foods, body fluids, and object surfaces. In addition to sample preparation-free protocols, biosensors are compatible with label-free protocols (Daniels and Pourmand, 2007; Rapp et al. 2010; Sang et al. 2016; Vestergaard et al. 2007). Labels, often referred to as reporters, are molecular species, such as organic dyes or quantum dots (Resch-Genger et al. 2008), that are attached to the target, either directly or through a biorecognition element, using a series of sample preparation steps or secondary binding steps to facilitate detection through the properties of the label. Thus, label-free biosensors avoid the use of a reporter species to detect the target species (Cooper, 2009; Syahir et al. 2015). Label-free assays often have fewer sample preparation steps due to the elimination of procedures associated with target labeling and lower cost than label-based assays, which are important considerations for applications in which preparation facilities or trained personnel are either limited or unavailable (Cooper, 2009; Syahir et al. 2015).\nWhile various types of transducers have been investigated for pathogen biosensing (Lazcka et al. 2007; Singh et al. 2014; Yoo and Lee, 2016), including mechanical and optical transducers, such as cantilever biosensors or surface plasmon resonance (SPR)-based biosensors, electrochemical biosensors have been extensively applied to pathogen detection (Felix and Angnes, 2018; Pereira da Silva Neves et al. 2018; Saucedo et al. 2019). Electrochemical biosensors for pathogen detection utilize conducting and semiconducting materials as the transducer, which is commonly referred to as an electrode. The chemical energy associated with binding between target pathogens and electrode-immobilized biorecognition elements is converted into electrical energy through an electrochemical method that involves the electrode and a pathogen-containing electrolyte solution. To date, electrochemical biosensors have enabled sample preparation-free detection of pathogens in various matrices, in situ detection of pathogens on surfaces, rapid pathogen detection using low-cost platforms, multiplexed detection of pathogens in practical matrices, and detection of pathogens via wireless actuation and data acquisition formats. As a result, electrochemical biosensors for pathogen detection have been widely examined for food and water safety, medical diagnostic, environmental monitoring, and bio-threat applications (Amiri et al. 2018; Duffy and Moore, 2017; Felix and Angnes, 2018; Furst and Francis, 2019; Mishra et al. 2018; Monzó et al. 2015; Rastogi and Singh, 2019).\nHere, we critically review electrochemical biosensors for pathogen detection. To gain insight into the trajectory of the field, electrochemical biosensors for pathogen detection reported since 2005 are critically reviewed and classified with respect to IUPAC-recommended definitions and classifications (Thévenot et al. 2001). Applications of electrochemical biosensors for pathogen detection are critically reviewed with respect to the target pathogen, sample matrix, biosensor design, fabrication method, measurement format, and biosensor performance. We also discuss future directions of electrochemical biosensors for pathogen detection, which includes a discussion of present technological and methodological challenges and emerging application areas."}
LitCovid-PD-MONDO
{"project":"LitCovid-PD-MONDO","denotations":[{"id":"T1","span":{"begin":30,"end":40},"obj":"Disease"},{"id":"T2","span":{"begin":158,"end":168},"obj":"Disease"},{"id":"T3","span":{"begin":293,"end":302},"obj":"Disease"},{"id":"T4","span":{"begin":455,"end":464},"obj":"Disease"},{"id":"T5","span":{"begin":610,"end":620},"obj":"Disease"},{"id":"T6","span":{"begin":708,"end":716},"obj":"Disease"},{"id":"T7","span":{"begin":748,"end":758},"obj":"Disease"},{"id":"T8","span":{"begin":951,"end":961},"obj":"Disease"},{"id":"T9","span":{"begin":1233,"end":1242},"obj":"Disease"},{"id":"T10","span":{"begin":1611,"end":1620},"obj":"Disease"},{"id":"T11","span":{"begin":2148,"end":2158},"obj":"Disease"}],"attributes":[{"id":"A1","pred":"mondo_id","subj":"T1","obj":"http://purl.obolibrary.org/obo/MONDO_0005550"},{"id":"A2","pred":"mondo_id","subj":"T2","obj":"http://purl.obolibrary.org/obo/MONDO_0005550"},{"id":"A3","pred":"mondo_id","subj":"T3","obj":"http://purl.obolibrary.org/obo/MONDO_0005550"},{"id":"A4","pred":"mondo_id","subj":"T4","obj":"http://purl.obolibrary.org/obo/MONDO_0005812"},{"id":"A5","pred":"mondo_id","subj":"T5","obj":"http://purl.obolibrary.org/obo/MONDO_0005550"},{"id":"A6","pred":"mondo_id","subj":"T6","obj":"http://purl.obolibrary.org/obo/MONDO_0100096"},{"id":"A7","pred":"mondo_id","subj":"T7","obj":"http://purl.obolibrary.org/obo/MONDO_0005550"},{"id":"A8","pred":"mondo_id","subj":"T8","obj":"http://purl.obolibrary.org/obo/MONDO_0005550"},{"id":"A9","pred":"mondo_id","subj":"T9","obj":"http://purl.obolibrary.org/obo/MONDO_0005550"},{"id":"A10","pred":"mondo_id","subj":"T10","obj":"http://purl.obolibrary.org/obo/MONDO_0005550"},{"id":"A11","pred":"mondo_id","subj":"T11","obj":"http://purl.obolibrary.org/obo/MONDO_0005550"}],"text":"1 Introduction\nPathogens are infectious agents that cause disease. They include microorganisms, such as fungi, protozoans, and bacteria, and molecular-scale infectious agents, including viruses and prions. Foodborne, waterborne, and airborne pathogens enter the body through various modes of infection and are responsible for over 15 million deaths annually worldwide (Dye, 2014). Some of the most common pathogens include viruses, such as norovirus and influenza virus, and bacteria, such as E. coli and S. aureus. Pathogens vary in many regards, such as virulence, contagiousness, mode of transmission, and infectious dose. For example, the world is currently facing a global pandemic associated with the COVID-19 virus, for which virulence and infectious dose data are still emerging. Techniques for sensitive and rapid detection of pathogens in complex matrices, such as body fluids and aerosols, and on surfaces are critical to the treatment of infectious diseases and controlling the spread of disease.\nThe techniques used to identify and quantify pathogens can be broadly distinguished as immunoassays or DNA-based assays. The use of immunoassays versus DNA-based assays depends on various factors, including the stage of an infection and the availability of antibodies and DNA sequence data, such as viral DNA, toxin-producing genes, as well as species- and strain-selective genes. Immunoassays are ubiquitous across medical diagnostics and food safety applications. Pathogens can be identified through the presence of generated antibodies in an organism, which may be present both during and after an infection (i.e., after the pathogen is no longer present). In such assays, both the biorecognition element and the target are antibodies. If antibodies are available for the pathogen (e.g., anti-E. coli O157:H7), one can also directly detect the pathogen using immunoassays. The ability to indirectly and directly detect pathogens via generated antibodies and pathogen epitopes, respectively, makes immunoassays flexible techniques for pathogen detection. In cases of limited antibody availability, need for highly sensitive results, or infections that do not generate a significant level of antibody production in the organism although the pathogen is present, DNA-based assays are commonly employed. DNA-based assays require the pathogen to be present in the sample or to have been recently present. In addition to detection of pathogens using antibodies or toxin-producing genes, pathogens can also be detected based on their expression of toxins. Thus, targets associated with pathogen detection include toxins, nucleic acids, viruses, cells, and oocysts. As a result, biorecognition elements widely vary, including antibodies, aptamers, and imprinted polymers. Several comprehensive reviews have been written on pathogen detection using high-throughput, well plate-based bioanalytical techniques (Alahi and Mukhopadhyay, 2017; Lazcka et al. 2007; Zourob et al. 2008), such as enzyme-linked immunosorbent assay (ELISA) (Law et al. 2015) and polymerase chain reaction (PCR) (Klein, 2002; Malorny et al. 2003), which remain the gold standards for pathogen detection. Few reviews, however, have focused on emerging label-free biosensors for pathogen detection, which provide useful characteristics for applications in process monitoring (e.g., of biomanufacturing processes), environmental monitoring, and precision agriculture.\nBioanalytical techniques utilize a selective biorecognition element, often called a molecular probe, in combination with an analytical system, such as a plate reader or PCR analyzer, to quantify one or more components of a sample. While capable of being highly sensitive and robust, they are destructive testing methods and require the addition of reagents to the sample and extensive sample preparation steps, which increase the time-to-results (TTR). Bioanalytical techniques, such as PCR, may also encounter inhibition effects caused by background species in the sample (Justino et al. 2017; Scognamiglio et al. 2016; Sin et al. 2014), which introduce measurement bias and increase measurement uncertainty (Clark et al. 2016; Silverman et al. 2019). Considering such limitations of traditional plate-based bioanalytical techniques and the need for real-time continuous monitoring capabilities among various applications, there is a need to examine alternative bioanalytical techniques.\nOver the past twenty-five years, biosensors have emerged to complement PCR and ELISA for pathogen detection. Biosensors are based on the direct integration of a selective biorecognition element and a sensitive transducer element and provide complementary platforms to PCR and ELISA for pathogen identification and quantification. According to the International Union of Pure and Applied Chemistry (IUPAC), a biosensor must contain a biorecognition element in direct spatial contact with a transduction element (Thévenot et al. 2001). In addition, a biosensor should provide quantitative or semi-quantitative analytical information and measurement without the requirement of additional processing steps or reagents. While a biosensor should also be a self-contained, integrated device, the measurement approach can vary from droplet formats to continuous flow formats that require associated fluid handling systems. Biosensors have achieved sensitive and selective real-time detection of pathogens in various environments without the need for sample preparation. For example, biosensors have enabled the detection of an abundance of pathogens in various matrices and environments, including foods, body fluids, and object surfaces. In addition to sample preparation-free protocols, biosensors are compatible with label-free protocols (Daniels and Pourmand, 2007; Rapp et al. 2010; Sang et al. 2016; Vestergaard et al. 2007). Labels, often referred to as reporters, are molecular species, such as organic dyes or quantum dots (Resch-Genger et al. 2008), that are attached to the target, either directly or through a biorecognition element, using a series of sample preparation steps or secondary binding steps to facilitate detection through the properties of the label. Thus, label-free biosensors avoid the use of a reporter species to detect the target species (Cooper, 2009; Syahir et al. 2015). Label-free assays often have fewer sample preparation steps due to the elimination of procedures associated with target labeling and lower cost than label-based assays, which are important considerations for applications in which preparation facilities or trained personnel are either limited or unavailable (Cooper, 2009; Syahir et al. 2015).\nWhile various types of transducers have been investigated for pathogen biosensing (Lazcka et al. 2007; Singh et al. 2014; Yoo and Lee, 2016), including mechanical and optical transducers, such as cantilever biosensors or surface plasmon resonance (SPR)-based biosensors, electrochemical biosensors have been extensively applied to pathogen detection (Felix and Angnes, 2018; Pereira da Silva Neves et al. 2018; Saucedo et al. 2019). Electrochemical biosensors for pathogen detection utilize conducting and semiconducting materials as the transducer, which is commonly referred to as an electrode. The chemical energy associated with binding between target pathogens and electrode-immobilized biorecognition elements is converted into electrical energy through an electrochemical method that involves the electrode and a pathogen-containing electrolyte solution. To date, electrochemical biosensors have enabled sample preparation-free detection of pathogens in various matrices, in situ detection of pathogens on surfaces, rapid pathogen detection using low-cost platforms, multiplexed detection of pathogens in practical matrices, and detection of pathogens via wireless actuation and data acquisition formats. As a result, electrochemical biosensors for pathogen detection have been widely examined for food and water safety, medical diagnostic, environmental monitoring, and bio-threat applications (Amiri et al. 2018; Duffy and Moore, 2017; Felix and Angnes, 2018; Furst and Francis, 2019; Mishra et al. 2018; Monzó et al. 2015; Rastogi and Singh, 2019).\nHere, we critically review electrochemical biosensors for pathogen detection. To gain insight into the trajectory of the field, electrochemical biosensors for pathogen detection reported since 2005 are critically reviewed and classified with respect to IUPAC-recommended definitions and classifications (Thévenot et al. 2001). Applications of electrochemical biosensors for pathogen detection are critically reviewed with respect to the target pathogen, sample matrix, biosensor design, fabrication method, measurement format, and biosensor performance. We also discuss future directions of electrochemical biosensors for pathogen detection, which includes a discussion of present technological and methodological challenges and emerging application areas."}
LitCovid-PD-CLO
{"project":"LitCovid-PD-CLO","denotations":[{"id":"T14476","span":{"begin":128,"end":136},"obj":"http://purl.obolibrary.org/obo/NCBITaxon_2"},{"id":"T80625","span":{"begin":187,"end":194},"obj":"http://purl.obolibrary.org/obo/NCBITaxon_10239"},{"id":"T63352","span":{"begin":424,"end":431},"obj":"http://purl.obolibrary.org/obo/NCBITaxon_10239"},{"id":"T73125","span":{"begin":465,"end":470},"obj":"http://purl.obolibrary.org/obo/NCBITaxon_10239"},{"id":"T61249","span":{"begin":476,"end":484},"obj":"http://purl.obolibrary.org/obo/NCBITaxon_2"},{"id":"T28402","span":{"begin":663,"end":669},"obj":"http://purl.obolibrary.org/obo/UBERON_0001456"},{"id":"T8155","span":{"begin":670,"end":671},"obj":"http://purl.obolibrary.org/obo/CLO_0001020"},{"id":"T36574","span":{"begin":717,"end":722},"obj":"http://purl.obolibrary.org/obo/NCBITaxon_10239"},{"id":"T3431","span":{"begin":876,"end":887},"obj":"http://purl.obolibrary.org/obo/UBERON_0006314"},{"id":"T91690","span":{"begin":1336,"end":1341},"obj":"http://purl.obolibrary.org/obo/OGG_0000000002"},{"id":"T24843","span":{"begin":1384,"end":1389},"obj":"http://purl.obolibrary.org/obo/OGG_0000000002"},{"id":"T36131","span":{"begin":1555,"end":1563},"obj":"http://purl.obolibrary.org/obo/OBI_0100026"},{"id":"T85202","span":{"begin":1555,"end":1563},"obj":"http://purl.obolibrary.org/obo/UBERON_0000468"},{"id":"T8720","span":{"begin":2180,"end":2181},"obj":"http://purl.obolibrary.org/obo/CLO_0001020"},{"id":"T80278","span":{"begin":2230,"end":2238},"obj":"http://purl.obolibrary.org/obo/OBI_0100026"},{"id":"T41563","span":{"begin":2230,"end":2238},"obj":"http://purl.obolibrary.org/obo/UBERON_0000468"},{"id":"T50996","span":{"begin":2487,"end":2492},"obj":"http://purl.obolibrary.org/obo/OGG_0000000002"},{"id":"T38879","span":{"begin":2642,"end":2649},"obj":"http://purl.obolibrary.org/obo/NCBITaxon_10239"},{"id":"T82236","span":{"begin":2651,"end":2656},"obj":"http://purl.obolibrary.org/obo/GO_0005623"},{"id":"T65256","span":{"begin":2674,"end":2675},"obj":"http://purl.obolibrary.org/obo/CLO_0001020"},{"id":"T92248","span":{"begin":3207,"end":3214},"obj":"http://purl.obolibrary.org/obo/CLO_0009985"},{"id":"T37566","span":{"begin":3227,"end":3232},"obj":"http://purl.obolibrary.org/obo/CLO_0007225"},{"id":"T50432","span":{"begin":3474,"end":3475},"obj":"http://purl.obolibrary.org/obo/CLO_0001020"},{"id":"T51725","span":{"begin":3516,"end":3524},"obj":"http://purl.obolibrary.org/obo/PR_000001898"},{"id":"T3158","span":{"begin":3592,"end":3593},"obj":"http://purl.obolibrary.org/obo/CLO_0001020"},{"id":"T61528","span":{"begin":3662,"end":3663},"obj":"http://purl.obolibrary.org/obo/CLO_0001020"},{"id":"T42866","span":{"begin":3745,"end":3752},"obj":"http://purl.obolibrary.org/obo/UBERON_0000473"},{"id":"T73209","span":{"begin":4374,"end":4375},"obj":"http://purl.obolibrary.org/obo/CLO_0001020"},{"id":"T71756","span":{"begin":4589,"end":4590},"obj":"http://purl.obolibrary.org/obo/CLO_0001020"},{"id":"T88152","span":{"begin":4628,"end":4629},"obj":"http://purl.obolibrary.org/obo/CLO_0001020"},{"id":"T43393","span":{"begin":4836,"end":4837},"obj":"http://purl.obolibrary.org/obo/CLO_0001020"},{"id":"T67322","span":{"begin":4861,"end":4862},"obj":"http://purl.obolibrary.org/obo/CLO_0001020"},{"id":"T62612","span":{"begin":4917,"end":4918},"obj":"http://purl.obolibrary.org/obo/CLO_0001020"},{"id":"T45685","span":{"begin":4977,"end":4978},"obj":"http://purl.obolibrary.org/obo/CLO_0001020"},{"id":"T64650","span":{"begin":5151,"end":5152},"obj":"http://purl.obolibrary.org/obo/CLO_0001020"},{"id":"T47476","span":{"begin":5178,"end":5179},"obj":"http://purl.obolibrary.org/obo/CLO_0001020"},{"id":"T30180","span":{"begin":5207,"end":5213},"obj":"http://purl.obolibrary.org/obo/OBI_0000968"},{"id":"T64360","span":{"begin":5627,"end":5638},"obj":"http://purl.obolibrary.org/obo/UBERON_0006314"},{"id":"T67174","span":{"begin":5644,"end":5650},"obj":"http://purl.obolibrary.org/obo/BFO_0000030"},{"id":"T57506","span":{"begin":5742,"end":5747},"obj":"http://purl.obolibrary.org/obo/CLO_0007225"},{"id":"T64576","span":{"begin":5854,"end":5860},"obj":"http://purl.obolibrary.org/obo/CLO_0007225"},{"id":"T5259","span":{"begin":5925,"end":5932},"obj":"http://purl.obolibrary.org/obo/OBI_0100026"},{"id":"T67349","span":{"begin":5925,"end":5932},"obj":"http://purl.obolibrary.org/obo/UBERON_0000468"},{"id":"T43939","span":{"begin":6042,"end":6043},"obj":"http://purl.obolibrary.org/obo/CLO_0001020"},{"id":"T68512","span":{"begin":6074,"end":6075},"obj":"http://purl.obolibrary.org/obo/CLO_0001020"},{"id":"T34652","span":{"begin":6192,"end":6197},"obj":"http://purl.obolibrary.org/obo/CLO_0007225"},{"id":"T93702","span":{"begin":6205,"end":6210},"obj":"http://purl.obolibrary.org/obo/CLO_0007225"},{"id":"T95045","span":{"begin":6244,"end":6245},"obj":"http://purl.obolibrary.org/obo/CLO_0001020"},{"id":"T20027","span":{"begin":6328,"end":6333},"obj":"http://purl.obolibrary.org/obo/CLO_0007225"},{"id":"T56574","span":{"begin":6448,"end":6456},"obj":"http://purl.obolibrary.org/obo/CLO_0007225"},{"id":"T61655","span":{"begin":6477,"end":6482},"obj":"http://purl.obolibrary.org/obo/CLO_0007225"},{"id":"T74784","span":{"begin":7041,"end":7045},"obj":"http://purl.obolibrary.org/obo/CLO_0001185"},{"id":"T91038","span":{"begin":7055,"end":7057},"obj":"http://www.ebi.ac.uk/efo/EFO_0000265"},{"id":"T60304","span":{"begin":7077,"end":7081},"obj":"http://purl.obolibrary.org/obo/CLO_0001185"},{"id":"T27628","span":{"begin":7490,"end":7491},"obj":"http://purl.obolibrary.org/obo/CLO_0001020"},{"id":"T93259","span":{"begin":7887,"end":7888},"obj":"http://purl.obolibrary.org/obo/CLO_0001020"},{"id":"T17235","span":{"begin":8088,"end":8092},"obj":"http://purl.obolibrary.org/obo/CLO_0001185"},{"id":"T5167","span":{"begin":8135,"end":8139},"obj":"http://purl.obolibrary.org/obo/CLO_0001185"},{"id":"T39108","span":{"begin":8180,"end":8184},"obj":"http://purl.obolibrary.org/obo/CLO_0001185"},{"id":"T17345","span":{"begin":8352,"end":8357},"obj":"http://purl.obolibrary.org/obo/UBERON_0007688"},{"id":"T36712","span":{"begin":8888,"end":8889},"obj":"http://purl.obolibrary.org/obo/CLO_0001020"}],"text":"1 Introduction\nPathogens are infectious agents that cause disease. They include microorganisms, such as fungi, protozoans, and bacteria, and molecular-scale infectious agents, including viruses and prions. Foodborne, waterborne, and airborne pathogens enter the body through various modes of infection and are responsible for over 15 million deaths annually worldwide (Dye, 2014). Some of the most common pathogens include viruses, such as norovirus and influenza virus, and bacteria, such as E. coli and S. aureus. Pathogens vary in many regards, such as virulence, contagiousness, mode of transmission, and infectious dose. For example, the world is currently facing a global pandemic associated with the COVID-19 virus, for which virulence and infectious dose data are still emerging. Techniques for sensitive and rapid detection of pathogens in complex matrices, such as body fluids and aerosols, and on surfaces are critical to the treatment of infectious diseases and controlling the spread of disease.\nThe techniques used to identify and quantify pathogens can be broadly distinguished as immunoassays or DNA-based assays. The use of immunoassays versus DNA-based assays depends on various factors, including the stage of an infection and the availability of antibodies and DNA sequence data, such as viral DNA, toxin-producing genes, as well as species- and strain-selective genes. Immunoassays are ubiquitous across medical diagnostics and food safety applications. Pathogens can be identified through the presence of generated antibodies in an organism, which may be present both during and after an infection (i.e., after the pathogen is no longer present). In such assays, both the biorecognition element and the target are antibodies. If antibodies are available for the pathogen (e.g., anti-E. coli O157:H7), one can also directly detect the pathogen using immunoassays. The ability to indirectly and directly detect pathogens via generated antibodies and pathogen epitopes, respectively, makes immunoassays flexible techniques for pathogen detection. In cases of limited antibody availability, need for highly sensitive results, or infections that do not generate a significant level of antibody production in the organism although the pathogen is present, DNA-based assays are commonly employed. DNA-based assays require the pathogen to be present in the sample or to have been recently present. In addition to detection of pathogens using antibodies or toxin-producing genes, pathogens can also be detected based on their expression of toxins. Thus, targets associated with pathogen detection include toxins, nucleic acids, viruses, cells, and oocysts. As a result, biorecognition elements widely vary, including antibodies, aptamers, and imprinted polymers. Several comprehensive reviews have been written on pathogen detection using high-throughput, well plate-based bioanalytical techniques (Alahi and Mukhopadhyay, 2017; Lazcka et al. 2007; Zourob et al. 2008), such as enzyme-linked immunosorbent assay (ELISA) (Law et al. 2015) and polymerase chain reaction (PCR) (Klein, 2002; Malorny et al. 2003), which remain the gold standards for pathogen detection. Few reviews, however, have focused on emerging label-free biosensors for pathogen detection, which provide useful characteristics for applications in process monitoring (e.g., of biomanufacturing processes), environmental monitoring, and precision agriculture.\nBioanalytical techniques utilize a selective biorecognition element, often called a molecular probe, in combination with an analytical system, such as a plate reader or PCR analyzer, to quantify one or more components of a sample. While capable of being highly sensitive and robust, they are destructive testing methods and require the addition of reagents to the sample and extensive sample preparation steps, which increase the time-to-results (TTR). Bioanalytical techniques, such as PCR, may also encounter inhibition effects caused by background species in the sample (Justino et al. 2017; Scognamiglio et al. 2016; Sin et al. 2014), which introduce measurement bias and increase measurement uncertainty (Clark et al. 2016; Silverman et al. 2019). Considering such limitations of traditional plate-based bioanalytical techniques and the need for real-time continuous monitoring capabilities among various applications, there is a need to examine alternative bioanalytical techniques.\nOver the past twenty-five years, biosensors have emerged to complement PCR and ELISA for pathogen detection. Biosensors are based on the direct integration of a selective biorecognition element and a sensitive transducer element and provide complementary platforms to PCR and ELISA for pathogen identification and quantification. According to the International Union of Pure and Applied Chemistry (IUPAC), a biosensor must contain a biorecognition element in direct spatial contact with a transduction element (Thévenot et al. 2001). In addition, a biosensor should provide quantitative or semi-quantitative analytical information and measurement without the requirement of additional processing steps or reagents. While a biosensor should also be a self-contained, integrated device, the measurement approach can vary from droplet formats to continuous flow formats that require associated fluid handling systems. Biosensors have achieved sensitive and selective real-time detection of pathogens in various environments without the need for sample preparation. For example, biosensors have enabled the detection of an abundance of pathogens in various matrices and environments, including foods, body fluids, and object surfaces. In addition to sample preparation-free protocols, biosensors are compatible with label-free protocols (Daniels and Pourmand, 2007; Rapp et al. 2010; Sang et al. 2016; Vestergaard et al. 2007). Labels, often referred to as reporters, are molecular species, such as organic dyes or quantum dots (Resch-Genger et al. 2008), that are attached to the target, either directly or through a biorecognition element, using a series of sample preparation steps or secondary binding steps to facilitate detection through the properties of the label. Thus, label-free biosensors avoid the use of a reporter species to detect the target species (Cooper, 2009; Syahir et al. 2015). Label-free assays often have fewer sample preparation steps due to the elimination of procedures associated with target labeling and lower cost than label-based assays, which are important considerations for applications in which preparation facilities or trained personnel are either limited or unavailable (Cooper, 2009; Syahir et al. 2015).\nWhile various types of transducers have been investigated for pathogen biosensing (Lazcka et al. 2007; Singh et al. 2014; Yoo and Lee, 2016), including mechanical and optical transducers, such as cantilever biosensors or surface plasmon resonance (SPR)-based biosensors, electrochemical biosensors have been extensively applied to pathogen detection (Felix and Angnes, 2018; Pereira da Silva Neves et al. 2018; Saucedo et al. 2019). Electrochemical biosensors for pathogen detection utilize conducting and semiconducting materials as the transducer, which is commonly referred to as an electrode. The chemical energy associated with binding between target pathogens and electrode-immobilized biorecognition elements is converted into electrical energy through an electrochemical method that involves the electrode and a pathogen-containing electrolyte solution. To date, electrochemical biosensors have enabled sample preparation-free detection of pathogens in various matrices, in situ detection of pathogens on surfaces, rapid pathogen detection using low-cost platforms, multiplexed detection of pathogens in practical matrices, and detection of pathogens via wireless actuation and data acquisition formats. As a result, electrochemical biosensors for pathogen detection have been widely examined for food and water safety, medical diagnostic, environmental monitoring, and bio-threat applications (Amiri et al. 2018; Duffy and Moore, 2017; Felix and Angnes, 2018; Furst and Francis, 2019; Mishra et al. 2018; Monzó et al. 2015; Rastogi and Singh, 2019).\nHere, we critically review electrochemical biosensors for pathogen detection. To gain insight into the trajectory of the field, electrochemical biosensors for pathogen detection reported since 2005 are critically reviewed and classified with respect to IUPAC-recommended definitions and classifications (Thévenot et al. 2001). Applications of electrochemical biosensors for pathogen detection are critically reviewed with respect to the target pathogen, sample matrix, biosensor design, fabrication method, measurement format, and biosensor performance. We also discuss future directions of electrochemical biosensors for pathogen detection, which includes a discussion of present technological and methodological challenges and emerging application areas."}
LitCovid-PD-CHEBI
{"project":"LitCovid-PD-CHEBI","denotations":[{"id":"T4","span":{"begin":1113,"end":1116},"obj":"Chemical"},{"id":"T5","span":{"begin":1162,"end":1165},"obj":"Chemical"},{"id":"T6","span":{"begin":1282,"end":1285},"obj":"Chemical"},{"id":"T7","span":{"begin":1315,"end":1318},"obj":"Chemical"},{"id":"T8","span":{"begin":1320,"end":1325},"obj":"Chemical"},{"id":"T9","span":{"begin":1819,"end":1821},"obj":"Chemical"},{"id":"T10","span":{"begin":2273,"end":2276},"obj":"Chemical"},{"id":"T11","span":{"begin":2313,"end":2316},"obj":"Chemical"},{"id":"T12","span":{"begin":2471,"end":2476},"obj":"Chemical"},{"id":"T13","span":{"begin":2554,"end":2560},"obj":"Chemical"},{"id":"T14","span":{"begin":2619,"end":2625},"obj":"Chemical"},{"id":"T15","span":{"begin":2627,"end":2640},"obj":"Chemical"},{"id":"T16","span":{"begin":2635,"end":2640},"obj":"Chemical"},{"id":"T17","span":{"begin":2743,"end":2751},"obj":"Chemical"},{"id":"T18","span":{"begin":2767,"end":2775},"obj":"Chemical"},{"id":"T19","span":{"begin":3141,"end":3145},"obj":"Chemical"},{"id":"T21","span":{"begin":3227,"end":3232},"obj":"Chemical"},{"id":"T22","span":{"begin":3535,"end":3540},"obj":"Chemical"},{"id":"T23","span":{"begin":3789,"end":3797},"obj":"Chemical"},{"id":"T24","span":{"begin":5135,"end":5143},"obj":"Chemical"},{"id":"T25","span":{"begin":5742,"end":5747},"obj":"Chemical"},{"id":"T26","span":{"begin":5933,"end":5937},"obj":"Chemical"},{"id":"T27","span":{"begin":6192,"end":6197},"obj":"Chemical"},{"id":"T28","span":{"begin":6205,"end":6210},"obj":"Chemical"},{"id":"T29","span":{"begin":6477,"end":6482},"obj":"Chemical"},{"id":"T30","span":{"begin":7524,"end":7532},"obj":"Chemical"},{"id":"T31","span":{"begin":7986,"end":7991},"obj":"Chemical"},{"id":"T32","span":{"begin":8969,"end":8980},"obj":"Chemical"}],"attributes":[{"id":"A4","pred":"chebi_id","subj":"T4","obj":"http://purl.obolibrary.org/obo/CHEBI_16991"},{"id":"A5","pred":"chebi_id","subj":"T5","obj":"http://purl.obolibrary.org/obo/CHEBI_16991"},{"id":"A6","pred":"chebi_id","subj":"T6","obj":"http://purl.obolibrary.org/obo/CHEBI_16991"},{"id":"A7","pred":"chebi_id","subj":"T7","obj":"http://purl.obolibrary.org/obo/CHEBI_16991"},{"id":"A8","pred":"chebi_id","subj":"T8","obj":"http://purl.obolibrary.org/obo/CHEBI_27026"},{"id":"A9","pred":"chebi_id","subj":"T9","obj":"http://purl.obolibrary.org/obo/CHEBI_83438"},{"id":"A10","pred":"chebi_id","subj":"T10","obj":"http://purl.obolibrary.org/obo/CHEBI_16991"},{"id":"A11","pred":"chebi_id","subj":"T11","obj":"http://purl.obolibrary.org/obo/CHEBI_16991"},{"id":"A12","pred":"chebi_id","subj":"T12","obj":"http://purl.obolibrary.org/obo/CHEBI_27026"},{"id":"A13","pred":"chebi_id","subj":"T13","obj":"http://purl.obolibrary.org/obo/CHEBI_27026"},{"id":"A14","pred":"chebi_id","subj":"T14","obj":"http://purl.obolibrary.org/obo/CHEBI_27026"},{"id":"A15","pred":"chebi_id","subj":"T15","obj":"http://purl.obolibrary.org/obo/CHEBI_33696"},{"id":"A16","pred":"chebi_id","subj":"T16","obj":"http://purl.obolibrary.org/obo/CHEBI_37527"},{"id":"A17","pred":"chebi_id","subj":"T17","obj":"http://purl.obolibrary.org/obo/CHEBI_140488"},{"id":"A18","pred":"chebi_id","subj":"T18","obj":"http://purl.obolibrary.org/obo/CHEBI_33839"},{"id":"A19","pred":"chebi_id","subj":"T19","obj":"http://purl.obolibrary.org/obo/CHEBI_29287"},{"id":"A20","pred":"chebi_id","subj":"T19","obj":"http://purl.obolibrary.org/obo/CHEBI_30050"},{"id":"A21","pred":"chebi_id","subj":"T21","obj":"http://purl.obolibrary.org/obo/CHEBI_35209"},{"id":"A22","pred":"chebi_id","subj":"T22","obj":"http://purl.obolibrary.org/obo/CHEBI_50406"},{"id":"A23","pred":"chebi_id","subj":"T23","obj":"http://purl.obolibrary.org/obo/CHEBI_33893"},{"id":"A24","pred":"chebi_id","subj":"T24","obj":"http://purl.obolibrary.org/obo/CHEBI_33893"},{"id":"A25","pred":"chebi_id","subj":"T25","obj":"http://purl.obolibrary.org/obo/CHEBI_35209"},{"id":"A26","pred":"chebi_id","subj":"T26","obj":"http://purl.obolibrary.org/obo/CHEBI_37958"},{"id":"A27","pred":"chebi_id","subj":"T27","obj":"http://purl.obolibrary.org/obo/CHEBI_35209"},{"id":"A28","pred":"chebi_id","subj":"T28","obj":"http://purl.obolibrary.org/obo/CHEBI_35209"},{"id":"A29","pred":"chebi_id","subj":"T29","obj":"http://purl.obolibrary.org/obo/CHEBI_35209"},{"id":"A30","pred":"chebi_id","subj":"T30","obj":"http://purl.obolibrary.org/obo/CHEBI_75958"},{"id":"A31","pred":"chebi_id","subj":"T31","obj":"http://purl.obolibrary.org/obo/CHEBI_15377"},{"id":"A32","pred":"chebi_id","subj":"T32","obj":"http://purl.obolibrary.org/obo/CHEBI_33232"}],"text":"1 Introduction\nPathogens are infectious agents that cause disease. They include microorganisms, such as fungi, protozoans, and bacteria, and molecular-scale infectious agents, including viruses and prions. Foodborne, waterborne, and airborne pathogens enter the body through various modes of infection and are responsible for over 15 million deaths annually worldwide (Dye, 2014). Some of the most common pathogens include viruses, such as norovirus and influenza virus, and bacteria, such as E. coli and S. aureus. Pathogens vary in many regards, such as virulence, contagiousness, mode of transmission, and infectious dose. For example, the world is currently facing a global pandemic associated with the COVID-19 virus, for which virulence and infectious dose data are still emerging. Techniques for sensitive and rapid detection of pathogens in complex matrices, such as body fluids and aerosols, and on surfaces are critical to the treatment of infectious diseases and controlling the spread of disease.\nThe techniques used to identify and quantify pathogens can be broadly distinguished as immunoassays or DNA-based assays. The use of immunoassays versus DNA-based assays depends on various factors, including the stage of an infection and the availability of antibodies and DNA sequence data, such as viral DNA, toxin-producing genes, as well as species- and strain-selective genes. Immunoassays are ubiquitous across medical diagnostics and food safety applications. Pathogens can be identified through the presence of generated antibodies in an organism, which may be present both during and after an infection (i.e., after the pathogen is no longer present). In such assays, both the biorecognition element and the target are antibodies. If antibodies are available for the pathogen (e.g., anti-E. coli O157:H7), one can also directly detect the pathogen using immunoassays. The ability to indirectly and directly detect pathogens via generated antibodies and pathogen epitopes, respectively, makes immunoassays flexible techniques for pathogen detection. In cases of limited antibody availability, need for highly sensitive results, or infections that do not generate a significant level of antibody production in the organism although the pathogen is present, DNA-based assays are commonly employed. DNA-based assays require the pathogen to be present in the sample or to have been recently present. In addition to detection of pathogens using antibodies or toxin-producing genes, pathogens can also be detected based on their expression of toxins. Thus, targets associated with pathogen detection include toxins, nucleic acids, viruses, cells, and oocysts. As a result, biorecognition elements widely vary, including antibodies, aptamers, and imprinted polymers. Several comprehensive reviews have been written on pathogen detection using high-throughput, well plate-based bioanalytical techniques (Alahi and Mukhopadhyay, 2017; Lazcka et al. 2007; Zourob et al. 2008), such as enzyme-linked immunosorbent assay (ELISA) (Law et al. 2015) and polymerase chain reaction (PCR) (Klein, 2002; Malorny et al. 2003), which remain the gold standards for pathogen detection. Few reviews, however, have focused on emerging label-free biosensors for pathogen detection, which provide useful characteristics for applications in process monitoring (e.g., of biomanufacturing processes), environmental monitoring, and precision agriculture.\nBioanalytical techniques utilize a selective biorecognition element, often called a molecular probe, in combination with an analytical system, such as a plate reader or PCR analyzer, to quantify one or more components of a sample. While capable of being highly sensitive and robust, they are destructive testing methods and require the addition of reagents to the sample and extensive sample preparation steps, which increase the time-to-results (TTR). Bioanalytical techniques, such as PCR, may also encounter inhibition effects caused by background species in the sample (Justino et al. 2017; Scognamiglio et al. 2016; Sin et al. 2014), which introduce measurement bias and increase measurement uncertainty (Clark et al. 2016; Silverman et al. 2019). Considering such limitations of traditional plate-based bioanalytical techniques and the need for real-time continuous monitoring capabilities among various applications, there is a need to examine alternative bioanalytical techniques.\nOver the past twenty-five years, biosensors have emerged to complement PCR and ELISA for pathogen detection. Biosensors are based on the direct integration of a selective biorecognition element and a sensitive transducer element and provide complementary platforms to PCR and ELISA for pathogen identification and quantification. According to the International Union of Pure and Applied Chemistry (IUPAC), a biosensor must contain a biorecognition element in direct spatial contact with a transduction element (Thévenot et al. 2001). In addition, a biosensor should provide quantitative or semi-quantitative analytical information and measurement without the requirement of additional processing steps or reagents. While a biosensor should also be a self-contained, integrated device, the measurement approach can vary from droplet formats to continuous flow formats that require associated fluid handling systems. Biosensors have achieved sensitive and selective real-time detection of pathogens in various environments without the need for sample preparation. For example, biosensors have enabled the detection of an abundance of pathogens in various matrices and environments, including foods, body fluids, and object surfaces. In addition to sample preparation-free protocols, biosensors are compatible with label-free protocols (Daniels and Pourmand, 2007; Rapp et al. 2010; Sang et al. 2016; Vestergaard et al. 2007). Labels, often referred to as reporters, are molecular species, such as organic dyes or quantum dots (Resch-Genger et al. 2008), that are attached to the target, either directly or through a biorecognition element, using a series of sample preparation steps or secondary binding steps to facilitate detection through the properties of the label. Thus, label-free biosensors avoid the use of a reporter species to detect the target species (Cooper, 2009; Syahir et al. 2015). Label-free assays often have fewer sample preparation steps due to the elimination of procedures associated with target labeling and lower cost than label-based assays, which are important considerations for applications in which preparation facilities or trained personnel are either limited or unavailable (Cooper, 2009; Syahir et al. 2015).\nWhile various types of transducers have been investigated for pathogen biosensing (Lazcka et al. 2007; Singh et al. 2014; Yoo and Lee, 2016), including mechanical and optical transducers, such as cantilever biosensors or surface plasmon resonance (SPR)-based biosensors, electrochemical biosensors have been extensively applied to pathogen detection (Felix and Angnes, 2018; Pereira da Silva Neves et al. 2018; Saucedo et al. 2019). Electrochemical biosensors for pathogen detection utilize conducting and semiconducting materials as the transducer, which is commonly referred to as an electrode. The chemical energy associated with binding between target pathogens and electrode-immobilized biorecognition elements is converted into electrical energy through an electrochemical method that involves the electrode and a pathogen-containing electrolyte solution. To date, electrochemical biosensors have enabled sample preparation-free detection of pathogens in various matrices, in situ detection of pathogens on surfaces, rapid pathogen detection using low-cost platforms, multiplexed detection of pathogens in practical matrices, and detection of pathogens via wireless actuation and data acquisition formats. As a result, electrochemical biosensors for pathogen detection have been widely examined for food and water safety, medical diagnostic, environmental monitoring, and bio-threat applications (Amiri et al. 2018; Duffy and Moore, 2017; Felix and Angnes, 2018; Furst and Francis, 2019; Mishra et al. 2018; Monzó et al. 2015; Rastogi and Singh, 2019).\nHere, we critically review electrochemical biosensors for pathogen detection. To gain insight into the trajectory of the field, electrochemical biosensors for pathogen detection reported since 2005 are critically reviewed and classified with respect to IUPAC-recommended definitions and classifications (Thévenot et al. 2001). Applications of electrochemical biosensors for pathogen detection are critically reviewed with respect to the target pathogen, sample matrix, biosensor design, fabrication method, measurement format, and biosensor performance. We also discuss future directions of electrochemical biosensors for pathogen detection, which includes a discussion of present technological and methodological challenges and emerging application areas."}
LitCovid-PD-GO-BP
{"project":"LitCovid-PD-GO-BP","denotations":[{"id":"T24194","span":{"begin":557,"end":566},"obj":"http://purl.obolibrary.org/obo/GO_0016032"},{"id":"T43287","span":{"begin":557,"end":566},"obj":"http://purl.obolibrary.org/obo/GO_0009405"},{"id":"T54619","span":{"begin":734,"end":743},"obj":"http://purl.obolibrary.org/obo/GO_0016032"},{"id":"T91958","span":{"begin":734,"end":743},"obj":"http://purl.obolibrary.org/obo/GO_0009405"},{"id":"T8583","span":{"begin":2203,"end":2222},"obj":"http://purl.obolibrary.org/obo/GO_0002377"},{"id":"T1220","span":{"begin":4919,"end":4931},"obj":"http://purl.obolibrary.org/obo/GO_0009293"}],"text":"1 Introduction\nPathogens are infectious agents that cause disease. They include microorganisms, such as fungi, protozoans, and bacteria, and molecular-scale infectious agents, including viruses and prions. Foodborne, waterborne, and airborne pathogens enter the body through various modes of infection and are responsible for over 15 million deaths annually worldwide (Dye, 2014). Some of the most common pathogens include viruses, such as norovirus and influenza virus, and bacteria, such as E. coli and S. aureus. Pathogens vary in many regards, such as virulence, contagiousness, mode of transmission, and infectious dose. For example, the world is currently facing a global pandemic associated with the COVID-19 virus, for which virulence and infectious dose data are still emerging. Techniques for sensitive and rapid detection of pathogens in complex matrices, such as body fluids and aerosols, and on surfaces are critical to the treatment of infectious diseases and controlling the spread of disease.\nThe techniques used to identify and quantify pathogens can be broadly distinguished as immunoassays or DNA-based assays. The use of immunoassays versus DNA-based assays depends on various factors, including the stage of an infection and the availability of antibodies and DNA sequence data, such as viral DNA, toxin-producing genes, as well as species- and strain-selective genes. Immunoassays are ubiquitous across medical diagnostics and food safety applications. Pathogens can be identified through the presence of generated antibodies in an organism, which may be present both during and after an infection (i.e., after the pathogen is no longer present). In such assays, both the biorecognition element and the target are antibodies. If antibodies are available for the pathogen (e.g., anti-E. coli O157:H7), one can also directly detect the pathogen using immunoassays. The ability to indirectly and directly detect pathogens via generated antibodies and pathogen epitopes, respectively, makes immunoassays flexible techniques for pathogen detection. In cases of limited antibody availability, need for highly sensitive results, or infections that do not generate a significant level of antibody production in the organism although the pathogen is present, DNA-based assays are commonly employed. DNA-based assays require the pathogen to be present in the sample or to have been recently present. In addition to detection of pathogens using antibodies or toxin-producing genes, pathogens can also be detected based on their expression of toxins. Thus, targets associated with pathogen detection include toxins, nucleic acids, viruses, cells, and oocysts. As a result, biorecognition elements widely vary, including antibodies, aptamers, and imprinted polymers. Several comprehensive reviews have been written on pathogen detection using high-throughput, well plate-based bioanalytical techniques (Alahi and Mukhopadhyay, 2017; Lazcka et al. 2007; Zourob et al. 2008), such as enzyme-linked immunosorbent assay (ELISA) (Law et al. 2015) and polymerase chain reaction (PCR) (Klein, 2002; Malorny et al. 2003), which remain the gold standards for pathogen detection. Few reviews, however, have focused on emerging label-free biosensors for pathogen detection, which provide useful characteristics for applications in process monitoring (e.g., of biomanufacturing processes), environmental monitoring, and precision agriculture.\nBioanalytical techniques utilize a selective biorecognition element, often called a molecular probe, in combination with an analytical system, such as a plate reader or PCR analyzer, to quantify one or more components of a sample. While capable of being highly sensitive and robust, they are destructive testing methods and require the addition of reagents to the sample and extensive sample preparation steps, which increase the time-to-results (TTR). Bioanalytical techniques, such as PCR, may also encounter inhibition effects caused by background species in the sample (Justino et al. 2017; Scognamiglio et al. 2016; Sin et al. 2014), which introduce measurement bias and increase measurement uncertainty (Clark et al. 2016; Silverman et al. 2019). Considering such limitations of traditional plate-based bioanalytical techniques and the need for real-time continuous monitoring capabilities among various applications, there is a need to examine alternative bioanalytical techniques.\nOver the past twenty-five years, biosensors have emerged to complement PCR and ELISA for pathogen detection. Biosensors are based on the direct integration of a selective biorecognition element and a sensitive transducer element and provide complementary platforms to PCR and ELISA for pathogen identification and quantification. According to the International Union of Pure and Applied Chemistry (IUPAC), a biosensor must contain a biorecognition element in direct spatial contact with a transduction element (Thévenot et al. 2001). In addition, a biosensor should provide quantitative or semi-quantitative analytical information and measurement without the requirement of additional processing steps or reagents. While a biosensor should also be a self-contained, integrated device, the measurement approach can vary from droplet formats to continuous flow formats that require associated fluid handling systems. Biosensors have achieved sensitive and selective real-time detection of pathogens in various environments without the need for sample preparation. For example, biosensors have enabled the detection of an abundance of pathogens in various matrices and environments, including foods, body fluids, and object surfaces. In addition to sample preparation-free protocols, biosensors are compatible with label-free protocols (Daniels and Pourmand, 2007; Rapp et al. 2010; Sang et al. 2016; Vestergaard et al. 2007). Labels, often referred to as reporters, are molecular species, such as organic dyes or quantum dots (Resch-Genger et al. 2008), that are attached to the target, either directly or through a biorecognition element, using a series of sample preparation steps or secondary binding steps to facilitate detection through the properties of the label. Thus, label-free biosensors avoid the use of a reporter species to detect the target species (Cooper, 2009; Syahir et al. 2015). Label-free assays often have fewer sample preparation steps due to the elimination of procedures associated with target labeling and lower cost than label-based assays, which are important considerations for applications in which preparation facilities or trained personnel are either limited or unavailable (Cooper, 2009; Syahir et al. 2015).\nWhile various types of transducers have been investigated for pathogen biosensing (Lazcka et al. 2007; Singh et al. 2014; Yoo and Lee, 2016), including mechanical and optical transducers, such as cantilever biosensors or surface plasmon resonance (SPR)-based biosensors, electrochemical biosensors have been extensively applied to pathogen detection (Felix and Angnes, 2018; Pereira da Silva Neves et al. 2018; Saucedo et al. 2019). Electrochemical biosensors for pathogen detection utilize conducting and semiconducting materials as the transducer, which is commonly referred to as an electrode. The chemical energy associated with binding between target pathogens and electrode-immobilized biorecognition elements is converted into electrical energy through an electrochemical method that involves the electrode and a pathogen-containing electrolyte solution. To date, electrochemical biosensors have enabled sample preparation-free detection of pathogens in various matrices, in situ detection of pathogens on surfaces, rapid pathogen detection using low-cost platforms, multiplexed detection of pathogens in practical matrices, and detection of pathogens via wireless actuation and data acquisition formats. As a result, electrochemical biosensors for pathogen detection have been widely examined for food and water safety, medical diagnostic, environmental monitoring, and bio-threat applications (Amiri et al. 2018; Duffy and Moore, 2017; Felix and Angnes, 2018; Furst and Francis, 2019; Mishra et al. 2018; Monzó et al. 2015; Rastogi and Singh, 2019).\nHere, we critically review electrochemical biosensors for pathogen detection. To gain insight into the trajectory of the field, electrochemical biosensors for pathogen detection reported since 2005 are critically reviewed and classified with respect to IUPAC-recommended definitions and classifications (Thévenot et al. 2001). Applications of electrochemical biosensors for pathogen detection are critically reviewed with respect to the target pathogen, sample matrix, biosensor design, fabrication method, measurement format, and biosensor performance. We also discuss future directions of electrochemical biosensors for pathogen detection, which includes a discussion of present technological and methodological challenges and emerging application areas."}
LitCovid-PD-HP
{"project":"LitCovid-PD-HP","denotations":[{"id":"T1","span":{"begin":2119,"end":2135},"obj":"Phenotype"},{"id":"T2","span":{"begin":3695,"end":3711},"obj":"Phenotype"}],"attributes":[{"id":"A1","pred":"hp_id","subj":"T1","obj":"http://purl.obolibrary.org/obo/HP_0041092"},{"id":"A2","pred":"hp_id","subj":"T2","obj":"http://purl.obolibrary.org/obo/HP_0041092"}],"text":"1 Introduction\nPathogens are infectious agents that cause disease. They include microorganisms, such as fungi, protozoans, and bacteria, and molecular-scale infectious agents, including viruses and prions. Foodborne, waterborne, and airborne pathogens enter the body through various modes of infection and are responsible for over 15 million deaths annually worldwide (Dye, 2014). Some of the most common pathogens include viruses, such as norovirus and influenza virus, and bacteria, such as E. coli and S. aureus. Pathogens vary in many regards, such as virulence, contagiousness, mode of transmission, and infectious dose. For example, the world is currently facing a global pandemic associated with the COVID-19 virus, for which virulence and infectious dose data are still emerging. Techniques for sensitive and rapid detection of pathogens in complex matrices, such as body fluids and aerosols, and on surfaces are critical to the treatment of infectious diseases and controlling the spread of disease.\nThe techniques used to identify and quantify pathogens can be broadly distinguished as immunoassays or DNA-based assays. The use of immunoassays versus DNA-based assays depends on various factors, including the stage of an infection and the availability of antibodies and DNA sequence data, such as viral DNA, toxin-producing genes, as well as species- and strain-selective genes. Immunoassays are ubiquitous across medical diagnostics and food safety applications. Pathogens can be identified through the presence of generated antibodies in an organism, which may be present both during and after an infection (i.e., after the pathogen is no longer present). In such assays, both the biorecognition element and the target are antibodies. If antibodies are available for the pathogen (e.g., anti-E. coli O157:H7), one can also directly detect the pathogen using immunoassays. The ability to indirectly and directly detect pathogens via generated antibodies and pathogen epitopes, respectively, makes immunoassays flexible techniques for pathogen detection. In cases of limited antibody availability, need for highly sensitive results, or infections that do not generate a significant level of antibody production in the organism although the pathogen is present, DNA-based assays are commonly employed. DNA-based assays require the pathogen to be present in the sample or to have been recently present. In addition to detection of pathogens using antibodies or toxin-producing genes, pathogens can also be detected based on their expression of toxins. Thus, targets associated with pathogen detection include toxins, nucleic acids, viruses, cells, and oocysts. As a result, biorecognition elements widely vary, including antibodies, aptamers, and imprinted polymers. Several comprehensive reviews have been written on pathogen detection using high-throughput, well plate-based bioanalytical techniques (Alahi and Mukhopadhyay, 2017; Lazcka et al. 2007; Zourob et al. 2008), such as enzyme-linked immunosorbent assay (ELISA) (Law et al. 2015) and polymerase chain reaction (PCR) (Klein, 2002; Malorny et al. 2003), which remain the gold standards for pathogen detection. Few reviews, however, have focused on emerging label-free biosensors for pathogen detection, which provide useful characteristics for applications in process monitoring (e.g., of biomanufacturing processes), environmental monitoring, and precision agriculture.\nBioanalytical techniques utilize a selective biorecognition element, often called a molecular probe, in combination with an analytical system, such as a plate reader or PCR analyzer, to quantify one or more components of a sample. While capable of being highly sensitive and robust, they are destructive testing methods and require the addition of reagents to the sample and extensive sample preparation steps, which increase the time-to-results (TTR). Bioanalytical techniques, such as PCR, may also encounter inhibition effects caused by background species in the sample (Justino et al. 2017; Scognamiglio et al. 2016; Sin et al. 2014), which introduce measurement bias and increase measurement uncertainty (Clark et al. 2016; Silverman et al. 2019). Considering such limitations of traditional plate-based bioanalytical techniques and the need for real-time continuous monitoring capabilities among various applications, there is a need to examine alternative bioanalytical techniques.\nOver the past twenty-five years, biosensors have emerged to complement PCR and ELISA for pathogen detection. Biosensors are based on the direct integration of a selective biorecognition element and a sensitive transducer element and provide complementary platforms to PCR and ELISA for pathogen identification and quantification. According to the International Union of Pure and Applied Chemistry (IUPAC), a biosensor must contain a biorecognition element in direct spatial contact with a transduction element (Thévenot et al. 2001). In addition, a biosensor should provide quantitative or semi-quantitative analytical information and measurement without the requirement of additional processing steps or reagents. While a biosensor should also be a self-contained, integrated device, the measurement approach can vary from droplet formats to continuous flow formats that require associated fluid handling systems. Biosensors have achieved sensitive and selective real-time detection of pathogens in various environments without the need for sample preparation. For example, biosensors have enabled the detection of an abundance of pathogens in various matrices and environments, including foods, body fluids, and object surfaces. In addition to sample preparation-free protocols, biosensors are compatible with label-free protocols (Daniels and Pourmand, 2007; Rapp et al. 2010; Sang et al. 2016; Vestergaard et al. 2007). Labels, often referred to as reporters, are molecular species, such as organic dyes or quantum dots (Resch-Genger et al. 2008), that are attached to the target, either directly or through a biorecognition element, using a series of sample preparation steps or secondary binding steps to facilitate detection through the properties of the label. Thus, label-free biosensors avoid the use of a reporter species to detect the target species (Cooper, 2009; Syahir et al. 2015). Label-free assays often have fewer sample preparation steps due to the elimination of procedures associated with target labeling and lower cost than label-based assays, which are important considerations for applications in which preparation facilities or trained personnel are either limited or unavailable (Cooper, 2009; Syahir et al. 2015).\nWhile various types of transducers have been investigated for pathogen biosensing (Lazcka et al. 2007; Singh et al. 2014; Yoo and Lee, 2016), including mechanical and optical transducers, such as cantilever biosensors or surface plasmon resonance (SPR)-based biosensors, electrochemical biosensors have been extensively applied to pathogen detection (Felix and Angnes, 2018; Pereira da Silva Neves et al. 2018; Saucedo et al. 2019). Electrochemical biosensors for pathogen detection utilize conducting and semiconducting materials as the transducer, which is commonly referred to as an electrode. The chemical energy associated with binding between target pathogens and electrode-immobilized biorecognition elements is converted into electrical energy through an electrochemical method that involves the electrode and a pathogen-containing electrolyte solution. To date, electrochemical biosensors have enabled sample preparation-free detection of pathogens in various matrices, in situ detection of pathogens on surfaces, rapid pathogen detection using low-cost platforms, multiplexed detection of pathogens in practical matrices, and detection of pathogens via wireless actuation and data acquisition formats. As a result, electrochemical biosensors for pathogen detection have been widely examined for food and water safety, medical diagnostic, environmental monitoring, and bio-threat applications (Amiri et al. 2018; Duffy and Moore, 2017; Felix and Angnes, 2018; Furst and Francis, 2019; Mishra et al. 2018; Monzó et al. 2015; Rastogi and Singh, 2019).\nHere, we critically review electrochemical biosensors for pathogen detection. To gain insight into the trajectory of the field, electrochemical biosensors for pathogen detection reported since 2005 are critically reviewed and classified with respect to IUPAC-recommended definitions and classifications (Thévenot et al. 2001). Applications of electrochemical biosensors for pathogen detection are critically reviewed with respect to the target pathogen, sample matrix, biosensor design, fabrication method, measurement format, and biosensor performance. We also discuss future directions of electrochemical biosensors for pathogen detection, which includes a discussion of present technological and methodological challenges and emerging application areas."}
LitCovid-sentences
{"project":"LitCovid-sentences","denotations":[{"id":"T16","span":{"begin":0,"end":15},"obj":"Sentence"},{"id":"T17","span":{"begin":16,"end":67},"obj":"Sentence"},{"id":"T18","span":{"begin":68,"end":206},"obj":"Sentence"},{"id":"T19","span":{"begin":207,"end":381},"obj":"Sentence"},{"id":"T20","span":{"begin":382,"end":516},"obj":"Sentence"},{"id":"T21","span":{"begin":517,"end":626},"obj":"Sentence"},{"id":"T22","span":{"begin":627,"end":788},"obj":"Sentence"},{"id":"T23","span":{"begin":789,"end":1009},"obj":"Sentence"},{"id":"T24","span":{"begin":1010,"end":1130},"obj":"Sentence"},{"id":"T25","span":{"begin":1131,"end":1390},"obj":"Sentence"},{"id":"T26","span":{"begin":1391,"end":1475},"obj":"Sentence"},{"id":"T27","span":{"begin":1476,"end":1669},"obj":"Sentence"},{"id":"T28","span":{"begin":1670,"end":1748},"obj":"Sentence"},{"id":"T29","span":{"begin":1749,"end":1885},"obj":"Sentence"},{"id":"T30","span":{"begin":1886,"end":2066},"obj":"Sentence"},{"id":"T31","span":{"begin":2067,"end":2312},"obj":"Sentence"},{"id":"T32","span":{"begin":2313,"end":2412},"obj":"Sentence"},{"id":"T33","span":{"begin":2413,"end":2561},"obj":"Sentence"},{"id":"T34","span":{"begin":2562,"end":2670},"obj":"Sentence"},{"id":"T35","span":{"begin":2671,"end":2776},"obj":"Sentence"},{"id":"T36","span":{"begin":2777,"end":2956},"obj":"Sentence"},{"id":"T37","span":{"begin":2957,"end":2976},"obj":"Sentence"},{"id":"T38","span":{"begin":2977,"end":3045},"obj":"Sentence"},{"id":"T39","span":{"begin":3046,"end":3116},"obj":"Sentence"},{"id":"T40","span":{"begin":3117,"end":3179},"obj":"Sentence"},{"id":"T41","span":{"begin":3180,"end":3440},"obj":"Sentence"},{"id":"T42","span":{"begin":3441,"end":3671},"obj":"Sentence"},{"id":"T43","span":{"begin":3672,"end":3893},"obj":"Sentence"},{"id":"T44","span":{"begin":3894,"end":4029},"obj":"Sentence"},{"id":"T45","span":{"begin":4030,"end":4055},"obj":"Sentence"},{"id":"T46","span":{"begin":4056,"end":4072},"obj":"Sentence"},{"id":"T47","span":{"begin":4073,"end":4163},"obj":"Sentence"},{"id":"T48","span":{"begin":4164,"end":4186},"obj":"Sentence"},{"id":"T49","span":{"begin":4187,"end":4193},"obj":"Sentence"},{"id":"T50","span":{"begin":4194,"end":4429},"obj":"Sentence"},{"id":"T51","span":{"begin":4430,"end":4538},"obj":"Sentence"},{"id":"T52","span":{"begin":4539,"end":4759},"obj":"Sentence"},{"id":"T53","span":{"begin":4760,"end":4956},"obj":"Sentence"},{"id":"T54","span":{"begin":4957,"end":4963},"obj":"Sentence"},{"id":"T55","span":{"begin":4964,"end":5144},"obj":"Sentence"},{"id":"T56","span":{"begin":5145,"end":5344},"obj":"Sentence"},{"id":"T57","span":{"begin":5345,"end":5491},"obj":"Sentence"},{"id":"T58","span":{"begin":5492,"end":5660},"obj":"Sentence"},{"id":"T59","span":{"begin":5661,"end":5803},"obj":"Sentence"},{"id":"T60","span":{"begin":5804,"end":5821},"obj":"Sentence"},{"id":"T61","span":{"begin":5822,"end":5846},"obj":"Sentence"},{"id":"T62","span":{"begin":5847,"end":5853},"obj":"Sentence"},{"id":"T63","span":{"begin":5854,"end":5974},"obj":"Sentence"},{"id":"T64","span":{"begin":5975,"end":6198},"obj":"Sentence"},{"id":"T65","span":{"begin":6199,"end":6320},"obj":"Sentence"},{"id":"T66","span":{"begin":6321,"end":6327},"obj":"Sentence"},{"id":"T67","span":{"begin":6328,"end":6664},"obj":"Sentence"},{"id":"T68","span":{"begin":6665,"end":6671},"obj":"Sentence"},{"id":"T69","span":{"begin":6672,"end":6768},"obj":"Sentence"},{"id":"T70","span":{"begin":6769,"end":6787},"obj":"Sentence"},{"id":"T71","span":{"begin":6788,"end":7076},"obj":"Sentence"},{"id":"T72","span":{"begin":7077,"end":7097},"obj":"Sentence"},{"id":"T73","span":{"begin":7098,"end":7104},"obj":"Sentence"},{"id":"T74","span":{"begin":7105,"end":7268},"obj":"Sentence"},{"id":"T75","span":{"begin":7269,"end":7533},"obj":"Sentence"},{"id":"T76","span":{"begin":7534,"end":7883},"obj":"Sentence"},{"id":"T77","span":{"begin":7884,"end":8087},"obj":"Sentence"},{"id":"T78","span":{"begin":8088,"end":8179},"obj":"Sentence"},{"id":"T79","span":{"begin":8180,"end":8198},"obj":"Sentence"},{"id":"T80","span":{"begin":8199,"end":8230},"obj":"Sentence"},{"id":"T81","span":{"begin":8231,"end":8308},"obj":"Sentence"},{"id":"T82","span":{"begin":8309,"end":8550},"obj":"Sentence"},{"id":"T83","span":{"begin":8551,"end":8557},"obj":"Sentence"},{"id":"T84","span":{"begin":8558,"end":8784},"obj":"Sentence"},{"id":"T85","span":{"begin":8785,"end":8987},"obj":"Sentence"}],"namespaces":[{"prefix":"_base","uri":"http://pubannotation.org/ontology/tao.owl#"}],"text":"1 Introduction\nPathogens are infectious agents that cause disease. They include microorganisms, such as fungi, protozoans, and bacteria, and molecular-scale infectious agents, including viruses and prions. Foodborne, waterborne, and airborne pathogens enter the body through various modes of infection and are responsible for over 15 million deaths annually worldwide (Dye, 2014). Some of the most common pathogens include viruses, such as norovirus and influenza virus, and bacteria, such as E. coli and S. aureus. Pathogens vary in many regards, such as virulence, contagiousness, mode of transmission, and infectious dose. For example, the world is currently facing a global pandemic associated with the COVID-19 virus, for which virulence and infectious dose data are still emerging. Techniques for sensitive and rapid detection of pathogens in complex matrices, such as body fluids and aerosols, and on surfaces are critical to the treatment of infectious diseases and controlling the spread of disease.\nThe techniques used to identify and quantify pathogens can be broadly distinguished as immunoassays or DNA-based assays. The use of immunoassays versus DNA-based assays depends on various factors, including the stage of an infection and the availability of antibodies and DNA sequence data, such as viral DNA, toxin-producing genes, as well as species- and strain-selective genes. Immunoassays are ubiquitous across medical diagnostics and food safety applications. Pathogens can be identified through the presence of generated antibodies in an organism, which may be present both during and after an infection (i.e., after the pathogen is no longer present). In such assays, both the biorecognition element and the target are antibodies. If antibodies are available for the pathogen (e.g., anti-E. coli O157:H7), one can also directly detect the pathogen using immunoassays. The ability to indirectly and directly detect pathogens via generated antibodies and pathogen epitopes, respectively, makes immunoassays flexible techniques for pathogen detection. In cases of limited antibody availability, need for highly sensitive results, or infections that do not generate a significant level of antibody production in the organism although the pathogen is present, DNA-based assays are commonly employed. DNA-based assays require the pathogen to be present in the sample or to have been recently present. In addition to detection of pathogens using antibodies or toxin-producing genes, pathogens can also be detected based on their expression of toxins. Thus, targets associated with pathogen detection include toxins, nucleic acids, viruses, cells, and oocysts. As a result, biorecognition elements widely vary, including antibodies, aptamers, and imprinted polymers. Several comprehensive reviews have been written on pathogen detection using high-throughput, well plate-based bioanalytical techniques (Alahi and Mukhopadhyay, 2017; Lazcka et al. 2007; Zourob et al. 2008), such as enzyme-linked immunosorbent assay (ELISA) (Law et al. 2015) and polymerase chain reaction (PCR) (Klein, 2002; Malorny et al. 2003), which remain the gold standards for pathogen detection. Few reviews, however, have focused on emerging label-free biosensors for pathogen detection, which provide useful characteristics for applications in process monitoring (e.g., of biomanufacturing processes), environmental monitoring, and precision agriculture.\nBioanalytical techniques utilize a selective biorecognition element, often called a molecular probe, in combination with an analytical system, such as a plate reader or PCR analyzer, to quantify one or more components of a sample. While capable of being highly sensitive and robust, they are destructive testing methods and require the addition of reagents to the sample and extensive sample preparation steps, which increase the time-to-results (TTR). Bioanalytical techniques, such as PCR, may also encounter inhibition effects caused by background species in the sample (Justino et al. 2017; Scognamiglio et al. 2016; Sin et al. 2014), which introduce measurement bias and increase measurement uncertainty (Clark et al. 2016; Silverman et al. 2019). Considering such limitations of traditional plate-based bioanalytical techniques and the need for real-time continuous monitoring capabilities among various applications, there is a need to examine alternative bioanalytical techniques.\nOver the past twenty-five years, biosensors have emerged to complement PCR and ELISA for pathogen detection. Biosensors are based on the direct integration of a selective biorecognition element and a sensitive transducer element and provide complementary platforms to PCR and ELISA for pathogen identification and quantification. According to the International Union of Pure and Applied Chemistry (IUPAC), a biosensor must contain a biorecognition element in direct spatial contact with a transduction element (Thévenot et al. 2001). In addition, a biosensor should provide quantitative or semi-quantitative analytical information and measurement without the requirement of additional processing steps or reagents. While a biosensor should also be a self-contained, integrated device, the measurement approach can vary from droplet formats to continuous flow formats that require associated fluid handling systems. Biosensors have achieved sensitive and selective real-time detection of pathogens in various environments without the need for sample preparation. For example, biosensors have enabled the detection of an abundance of pathogens in various matrices and environments, including foods, body fluids, and object surfaces. In addition to sample preparation-free protocols, biosensors are compatible with label-free protocols (Daniels and Pourmand, 2007; Rapp et al. 2010; Sang et al. 2016; Vestergaard et al. 2007). Labels, often referred to as reporters, are molecular species, such as organic dyes or quantum dots (Resch-Genger et al. 2008), that are attached to the target, either directly or through a biorecognition element, using a series of sample preparation steps or secondary binding steps to facilitate detection through the properties of the label. Thus, label-free biosensors avoid the use of a reporter species to detect the target species (Cooper, 2009; Syahir et al. 2015). Label-free assays often have fewer sample preparation steps due to the elimination of procedures associated with target labeling and lower cost than label-based assays, which are important considerations for applications in which preparation facilities or trained personnel are either limited or unavailable (Cooper, 2009; Syahir et al. 2015).\nWhile various types of transducers have been investigated for pathogen biosensing (Lazcka et al. 2007; Singh et al. 2014; Yoo and Lee, 2016), including mechanical and optical transducers, such as cantilever biosensors or surface plasmon resonance (SPR)-based biosensors, electrochemical biosensors have been extensively applied to pathogen detection (Felix and Angnes, 2018; Pereira da Silva Neves et al. 2018; Saucedo et al. 2019). Electrochemical biosensors for pathogen detection utilize conducting and semiconducting materials as the transducer, which is commonly referred to as an electrode. The chemical energy associated with binding between target pathogens and electrode-immobilized biorecognition elements is converted into electrical energy through an electrochemical method that involves the electrode and a pathogen-containing electrolyte solution. To date, electrochemical biosensors have enabled sample preparation-free detection of pathogens in various matrices, in situ detection of pathogens on surfaces, rapid pathogen detection using low-cost platforms, multiplexed detection of pathogens in practical matrices, and detection of pathogens via wireless actuation and data acquisition formats. As a result, electrochemical biosensors for pathogen detection have been widely examined for food and water safety, medical diagnostic, environmental monitoring, and bio-threat applications (Amiri et al. 2018; Duffy and Moore, 2017; Felix and Angnes, 2018; Furst and Francis, 2019; Mishra et al. 2018; Monzó et al. 2015; Rastogi and Singh, 2019).\nHere, we critically review electrochemical biosensors for pathogen detection. To gain insight into the trajectory of the field, electrochemical biosensors for pathogen detection reported since 2005 are critically reviewed and classified with respect to IUPAC-recommended definitions and classifications (Thévenot et al. 2001). Applications of electrochemical biosensors for pathogen detection are critically reviewed with respect to the target pathogen, sample matrix, biosensor design, fabrication method, measurement format, and biosensor performance. We also discuss future directions of electrochemical biosensors for pathogen detection, which includes a discussion of present technological and methodological challenges and emerging application areas."}
2_test
{"project":"2_test","denotations":[{"id":"32364936-16934970-7712879","span":{"begin":2957,"end":2961},"obj":"16934970"},{"id":"32364936-25628612-7712880","span":{"begin":3046,"end":3050},"obj":"25628612"},{"id":"32364936-12067606-7712881","span":{"begin":3096,"end":3100},"obj":"12067606"},{"id":"32364936-12672591-7712882","span":{"begin":3117,"end":3121},"obj":"12672591"},{"id":"32364936-24524681-7712883","span":{"begin":4073,"end":4077},"obj":"24524681"},{"id":"32364936-27779849-7712884","span":{"begin":4164,"end":4168},"obj":"27779849"},{"id":"32364936-11261847-7712885","span":{"begin":4957,"end":4961},"obj":"11261847"},{"id":"32364936-18176631-7712886","span":{"begin":5786,"end":5790},"obj":"18176631"},{"id":"32364936-20563563-7712887","span":{"begin":5804,"end":5808},"obj":"20563563"},{"id":"32364936-25608959-7712888","span":{"begin":5822,"end":5826},"obj":"25608959"},{"id":"32364936-28903304-7712889","span":{"begin":5847,"end":5851},"obj":"28903304"},{"id":"32364936-18756197-7712890","span":{"begin":5975,"end":5979},"obj":"18756197"},{"id":"32364936-27600222-7712891","span":{"begin":6321,"end":6325},"obj":"27600222"},{"id":"32364936-27600222-7712892","span":{"begin":6665,"end":6669},"obj":"27600222"},{"id":"32364936-16934970-7712893","span":{"begin":6769,"end":6773},"obj":"16934970"},{"id":"32364936-26506111-7712894","span":{"begin":6807,"end":6811},"obj":"26506111"},{"id":"32364936-29182930-7712895","span":{"begin":7041,"end":7045},"obj":"29182930"},{"id":"32364936-29756447-7712896","span":{"begin":8088,"end":8092},"obj":"29756447"},{"id":"32364936-29182930-7712897","span":{"begin":8135,"end":8139},"obj":"29182930"},{"id":"32364936-30557008-7712898","span":{"begin":8160,"end":8164},"obj":"30557008"},{"id":"32364936-26339688-7712899","span":{"begin":8199,"end":8203},"obj":"26339688"},{"id":"32364936-11261847-7712900","span":{"begin":8551,"end":8555},"obj":"11261847"}],"text":"1 Introduction\nPathogens are infectious agents that cause disease. They include microorganisms, such as fungi, protozoans, and bacteria, and molecular-scale infectious agents, including viruses and prions. Foodborne, waterborne, and airborne pathogens enter the body through various modes of infection and are responsible for over 15 million deaths annually worldwide (Dye, 2014). Some of the most common pathogens include viruses, such as norovirus and influenza virus, and bacteria, such as E. coli and S. aureus. Pathogens vary in many regards, such as virulence, contagiousness, mode of transmission, and infectious dose. For example, the world is currently facing a global pandemic associated with the COVID-19 virus, for which virulence and infectious dose data are still emerging. Techniques for sensitive and rapid detection of pathogens in complex matrices, such as body fluids and aerosols, and on surfaces are critical to the treatment of infectious diseases and controlling the spread of disease.\nThe techniques used to identify and quantify pathogens can be broadly distinguished as immunoassays or DNA-based assays. The use of immunoassays versus DNA-based assays depends on various factors, including the stage of an infection and the availability of antibodies and DNA sequence data, such as viral DNA, toxin-producing genes, as well as species- and strain-selective genes. Immunoassays are ubiquitous across medical diagnostics and food safety applications. Pathogens can be identified through the presence of generated antibodies in an organism, which may be present both during and after an infection (i.e., after the pathogen is no longer present). In such assays, both the biorecognition element and the target are antibodies. If antibodies are available for the pathogen (e.g., anti-E. coli O157:H7), one can also directly detect the pathogen using immunoassays. The ability to indirectly and directly detect pathogens via generated antibodies and pathogen epitopes, respectively, makes immunoassays flexible techniques for pathogen detection. In cases of limited antibody availability, need for highly sensitive results, or infections that do not generate a significant level of antibody production in the organism although the pathogen is present, DNA-based assays are commonly employed. DNA-based assays require the pathogen to be present in the sample or to have been recently present. In addition to detection of pathogens using antibodies or toxin-producing genes, pathogens can also be detected based on their expression of toxins. Thus, targets associated with pathogen detection include toxins, nucleic acids, viruses, cells, and oocysts. As a result, biorecognition elements widely vary, including antibodies, aptamers, and imprinted polymers. Several comprehensive reviews have been written on pathogen detection using high-throughput, well plate-based bioanalytical techniques (Alahi and Mukhopadhyay, 2017; Lazcka et al. 2007; Zourob et al. 2008), such as enzyme-linked immunosorbent assay (ELISA) (Law et al. 2015) and polymerase chain reaction (PCR) (Klein, 2002; Malorny et al. 2003), which remain the gold standards for pathogen detection. Few reviews, however, have focused on emerging label-free biosensors for pathogen detection, which provide useful characteristics for applications in process monitoring (e.g., of biomanufacturing processes), environmental monitoring, and precision agriculture.\nBioanalytical techniques utilize a selective biorecognition element, often called a molecular probe, in combination with an analytical system, such as a plate reader or PCR analyzer, to quantify one or more components of a sample. While capable of being highly sensitive and robust, they are destructive testing methods and require the addition of reagents to the sample and extensive sample preparation steps, which increase the time-to-results (TTR). Bioanalytical techniques, such as PCR, may also encounter inhibition effects caused by background species in the sample (Justino et al. 2017; Scognamiglio et al. 2016; Sin et al. 2014), which introduce measurement bias and increase measurement uncertainty (Clark et al. 2016; Silverman et al. 2019). Considering such limitations of traditional plate-based bioanalytical techniques and the need for real-time continuous monitoring capabilities among various applications, there is a need to examine alternative bioanalytical techniques.\nOver the past twenty-five years, biosensors have emerged to complement PCR and ELISA for pathogen detection. Biosensors are based on the direct integration of a selective biorecognition element and a sensitive transducer element and provide complementary platforms to PCR and ELISA for pathogen identification and quantification. According to the International Union of Pure and Applied Chemistry (IUPAC), a biosensor must contain a biorecognition element in direct spatial contact with a transduction element (Thévenot et al. 2001). In addition, a biosensor should provide quantitative or semi-quantitative analytical information and measurement without the requirement of additional processing steps or reagents. While a biosensor should also be a self-contained, integrated device, the measurement approach can vary from droplet formats to continuous flow formats that require associated fluid handling systems. Biosensors have achieved sensitive and selective real-time detection of pathogens in various environments without the need for sample preparation. For example, biosensors have enabled the detection of an abundance of pathogens in various matrices and environments, including foods, body fluids, and object surfaces. In addition to sample preparation-free protocols, biosensors are compatible with label-free protocols (Daniels and Pourmand, 2007; Rapp et al. 2010; Sang et al. 2016; Vestergaard et al. 2007). Labels, often referred to as reporters, are molecular species, such as organic dyes or quantum dots (Resch-Genger et al. 2008), that are attached to the target, either directly or through a biorecognition element, using a series of sample preparation steps or secondary binding steps to facilitate detection through the properties of the label. Thus, label-free biosensors avoid the use of a reporter species to detect the target species (Cooper, 2009; Syahir et al. 2015). Label-free assays often have fewer sample preparation steps due to the elimination of procedures associated with target labeling and lower cost than label-based assays, which are important considerations for applications in which preparation facilities or trained personnel are either limited or unavailable (Cooper, 2009; Syahir et al. 2015).\nWhile various types of transducers have been investigated for pathogen biosensing (Lazcka et al. 2007; Singh et al. 2014; Yoo and Lee, 2016), including mechanical and optical transducers, such as cantilever biosensors or surface plasmon resonance (SPR)-based biosensors, electrochemical biosensors have been extensively applied to pathogen detection (Felix and Angnes, 2018; Pereira da Silva Neves et al. 2018; Saucedo et al. 2019). Electrochemical biosensors for pathogen detection utilize conducting and semiconducting materials as the transducer, which is commonly referred to as an electrode. The chemical energy associated with binding between target pathogens and electrode-immobilized biorecognition elements is converted into electrical energy through an electrochemical method that involves the electrode and a pathogen-containing electrolyte solution. To date, electrochemical biosensors have enabled sample preparation-free detection of pathogens in various matrices, in situ detection of pathogens on surfaces, rapid pathogen detection using low-cost platforms, multiplexed detection of pathogens in practical matrices, and detection of pathogens via wireless actuation and data acquisition formats. As a result, electrochemical biosensors for pathogen detection have been widely examined for food and water safety, medical diagnostic, environmental monitoring, and bio-threat applications (Amiri et al. 2018; Duffy and Moore, 2017; Felix and Angnes, 2018; Furst and Francis, 2019; Mishra et al. 2018; Monzó et al. 2015; Rastogi and Singh, 2019).\nHere, we critically review electrochemical biosensors for pathogen detection. To gain insight into the trajectory of the field, electrochemical biosensors for pathogen detection reported since 2005 are critically reviewed and classified with respect to IUPAC-recommended definitions and classifications (Thévenot et al. 2001). Applications of electrochemical biosensors for pathogen detection are critically reviewed with respect to the target pathogen, sample matrix, biosensor design, fabrication method, measurement format, and biosensor performance. We also discuss future directions of electrochemical biosensors for pathogen detection, which includes a discussion of present technological and methodological challenges and emerging application areas."}