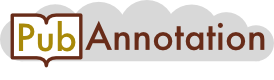
PMC:7152911 / 108682-111303
Annnotations
LitCovid-PubTator
{"project":"LitCovid-PubTator","denotations":[{"id":"1731","span":{"begin":2594,"end":2601},"obj":"Species"},{"id":"1732","span":{"begin":2410,"end":2417},"obj":"Chemical"},{"id":"1733","span":{"begin":2436,"end":2453},"obj":"Chemical"},{"id":"1744","span":{"begin":1901,"end":1903},"obj":"Gene"},{"id":"1745","span":{"begin":1576,"end":1578},"obj":"Gene"},{"id":"1746","span":{"begin":1553,"end":1560},"obj":"Species"},{"id":"1747","span":{"begin":1769,"end":1783},"obj":"Species"},{"id":"1748","span":{"begin":1684,"end":1698},"obj":"Species"},{"id":"1749","span":{"begin":1323,"end":1325},"obj":"Chemical"},{"id":"1750","span":{"begin":1491,"end":1499},"obj":"Chemical"},{"id":"1751","span":{"begin":1524,"end":1528},"obj":"Chemical"},{"id":"1752","span":{"begin":1648,"end":1655},"obj":"Chemical"},{"id":"1753","span":{"begin":1812,"end":1819},"obj":"Chemical"}],"attributes":[{"id":"A1731","pred":"tao:has_database_id","subj":"1731","obj":"Tax:562"},{"id":"A1732","pred":"tao:has_database_id","subj":"1732","obj":"MESH:D005947"},{"id":"A1733","pred":"tao:has_database_id","subj":"1733","obj":"MESH:D006861"},{"id":"A1744","pred":"tao:has_database_id","subj":"1744","obj":"Gene:21832"},{"id":"A1745","pred":"tao:has_database_id","subj":"1745","obj":"Gene:21832"},{"id":"A1746","pred":"tao:has_database_id","subj":"1746","obj":"Tax:562"},{"id":"A1747","pred":"tao:has_database_id","subj":"1747","obj":"Tax:90371"},{"id":"A1748","pred":"tao:has_database_id","subj":"1748","obj":"Tax:90371"},{"id":"A1749","pred":"tao:has_database_id","subj":"1749","obj":"MESH:D006046"},{"id":"A1750","pred":"tao:has_database_id","subj":"1750","obj":"MESH:C009469"},{"id":"A1751","pred":"tao:has_database_id","subj":"1751","obj":"MESH:D006861"},{"id":"A1752","pred":"tao:has_database_id","subj":"1752","obj":"MESH:D005947"},{"id":"A1753","pred":"tao:has_database_id","subj":"1753","obj":"MESH:D005947"}],"namespaces":[{"prefix":"Tax","uri":"https://www.ncbi.nlm.nih.gov/taxonomy/"},{"prefix":"MESH","uri":"https://id.nlm.nih.gov/mesh/"},{"prefix":"Gene","uri":"https://www.ncbi.nlm.nih.gov/gene/"},{"prefix":"CVCL","uri":"https://web.expasy.org/cellosaurus/CVCL_"}],"text":"Here, we classify assays that use secondary binding steps as labeled approaches in Table 1, Table 2 regardless of if the primary binding step produced a response. There is, however, a more subtle distinction if binding of the secondary species is used for amplification or verification purposes as previously discussed. Labels often include a biorecognition element-enzyme or -nanoparticle conjugate. In electrochemical biosensing applications, such labels often serve the purpose of altering the material properties or transport processes of the electrode-electrolyte interface, often by inducing a secondary reaction. Secondary binding of optically-active nanomaterials to captured targets can also enable the use of optical transducers for simultaneous detection or bioanalysis. Enzymes are among the most commonly used secondary binding species for label-based pathogen detection. As shown in Table 2, electrochemical biosensors for pathogen detection that employ enzymes are commonly performed as a sandwich assay format. A schematic of secondary binding steps for biosensor amplification based on the binding of HRP-antibody conjugates is shown in Fig. 6 a (Kokkinos et al. 2016). Hong et al. used HRP-labeled secondary antibodies to amplify the CV and EIS responses of a concanavalin A-functionalized nanostructured Au electrode to detect norovirus (Hong et al. 2015). Gayathri et al. used an HRP-antibody conjugate to induce an enzyme-assisted reduction reaction with an immobilized thionine-antibody receptor in an H2O2 system for detection of E. coli down to 50 CFU/mL using a sandwich assay format (Gayathri et al. 2016). Xu et al. used glucose oxidase and monoclonal anti-S. typhimurium to functionalize magnetic bead labels for separation and detection of S. typhimurium on an Au IDAM using EIS and glucose to catalyze the reaction that exhibited a linear working range of 102 to 106 CFU/mL (Xu et al. 2016b).\nFig. 6 Highlight of secondary binding and signal amplification approaches utilized in electrochemical biosensor-based pathogen detection. a) Four amplification approaches associated with the secondary binding of enzyme-labeled secondary antibodies: (A) electron transfer mediation; (B) nanostructuring of surface for increased rate of charge transfer kinetics; (C) conversion of electrochemically inactive substrate into a detectable electroactive product; (D) catalysis of oxidation of glucose for production of hydrogen peroxide for electrochemical detection (Kokkinos et al. 2016). b) Signal amplification via non-selective binding of AuNPs to bound bacterial target (E. coli) (Wan et al. 2016)."}
LitCovid-PD-FMA-UBERON
{"project":"LitCovid-PD-FMA-UBERON","denotations":[{"id":"T5","span":{"begin":1122,"end":1130},"obj":"Body_part"},{"id":"T6","span":{"begin":1404,"end":1412},"obj":"Body_part"},{"id":"T7","span":{"begin":1500,"end":1508},"obj":"Body_part"},{"id":"T8","span":{"begin":1648,"end":1655},"obj":"Body_part"},{"id":"T9","span":{"begin":1812,"end":1819},"obj":"Body_part"},{"id":"T10","span":{"begin":2410,"end":2417},"obj":"Body_part"}],"attributes":[{"id":"A5","pred":"fma_id","subj":"T5","obj":"http://purl.org/sig/ont/fma/fma62871"},{"id":"A6","pred":"fma_id","subj":"T6","obj":"http://purl.org/sig/ont/fma/fma62871"},{"id":"A7","pred":"fma_id","subj":"T7","obj":"http://purl.org/sig/ont/fma/fma62871"},{"id":"A8","pred":"fma_id","subj":"T8","obj":"http://purl.org/sig/ont/fma/fma82743"},{"id":"A9","pred":"fma_id","subj":"T9","obj":"http://purl.org/sig/ont/fma/fma82743"},{"id":"A10","pred":"fma_id","subj":"T10","obj":"http://purl.org/sig/ont/fma/fma82743"}],"text":"Here, we classify assays that use secondary binding steps as labeled approaches in Table 1, Table 2 regardless of if the primary binding step produced a response. There is, however, a more subtle distinction if binding of the secondary species is used for amplification or verification purposes as previously discussed. Labels often include a biorecognition element-enzyme or -nanoparticle conjugate. In electrochemical biosensing applications, such labels often serve the purpose of altering the material properties or transport processes of the electrode-electrolyte interface, often by inducing a secondary reaction. Secondary binding of optically-active nanomaterials to captured targets can also enable the use of optical transducers for simultaneous detection or bioanalysis. Enzymes are among the most commonly used secondary binding species for label-based pathogen detection. As shown in Table 2, electrochemical biosensors for pathogen detection that employ enzymes are commonly performed as a sandwich assay format. A schematic of secondary binding steps for biosensor amplification based on the binding of HRP-antibody conjugates is shown in Fig. 6 a (Kokkinos et al. 2016). Hong et al. used HRP-labeled secondary antibodies to amplify the CV and EIS responses of a concanavalin A-functionalized nanostructured Au electrode to detect norovirus (Hong et al. 2015). Gayathri et al. used an HRP-antibody conjugate to induce an enzyme-assisted reduction reaction with an immobilized thionine-antibody receptor in an H2O2 system for detection of E. coli down to 50 CFU/mL using a sandwich assay format (Gayathri et al. 2016). Xu et al. used glucose oxidase and monoclonal anti-S. typhimurium to functionalize magnetic bead labels for separation and detection of S. typhimurium on an Au IDAM using EIS and glucose to catalyze the reaction that exhibited a linear working range of 102 to 106 CFU/mL (Xu et al. 2016b).\nFig. 6 Highlight of secondary binding and signal amplification approaches utilized in electrochemical biosensor-based pathogen detection. a) Four amplification approaches associated with the secondary binding of enzyme-labeled secondary antibodies: (A) electron transfer mediation; (B) nanostructuring of surface for increased rate of charge transfer kinetics; (C) conversion of electrochemically inactive substrate into a detectable electroactive product; (D) catalysis of oxidation of glucose for production of hydrogen peroxide for electrochemical detection (Kokkinos et al. 2016). b) Signal amplification via non-selective binding of AuNPs to bound bacterial target (E. coli) (Wan et al. 2016)."}
LitCovid-PD-CLO
{"project":"LitCovid-PD-CLO","denotations":[{"id":"T55","span":{"begin":61,"end":68},"obj":"http://purl.obolibrary.org/obo/CLO_0007225"},{"id":"T56","span":{"begin":151,"end":152},"obj":"http://purl.obolibrary.org/obo/CLO_0001020"},{"id":"T57","span":{"begin":182,"end":183},"obj":"http://purl.obolibrary.org/obo/CLO_0001020"},{"id":"T58","span":{"begin":320,"end":326},"obj":"http://purl.obolibrary.org/obo/CLO_0007225"},{"id":"T59","span":{"begin":341,"end":342},"obj":"http://purl.obolibrary.org/obo/CLO_0001020"},{"id":"T60","span":{"begin":450,"end":456},"obj":"http://purl.obolibrary.org/obo/CLO_0007225"},{"id":"T61","span":{"begin":598,"end":599},"obj":"http://purl.obolibrary.org/obo/CLO_0001020"},{"id":"T62","span":{"begin":651,"end":657},"obj":"http://purl.obolibrary.org/obo/CLO_0001658"},{"id":"T63","span":{"begin":853,"end":858},"obj":"http://purl.obolibrary.org/obo/CLO_0007225"},{"id":"T64","span":{"begin":1002,"end":1003},"obj":"http://purl.obolibrary.org/obo/CLO_0001020"},{"id":"T65","span":{"begin":1027,"end":1028},"obj":"http://purl.obolibrary.org/obo/CLO_0001020"},{"id":"T66","span":{"begin":1161,"end":1162},"obj":"http://purl.obolibrary.org/obo/CLO_0001020"},{"id":"T67","span":{"begin":1208,"end":1215},"obj":"http://purl.obolibrary.org/obo/CLO_0007225"},{"id":"T68","span":{"begin":1276,"end":1277},"obj":"http://purl.obolibrary.org/obo/CLO_0001020"},{"id":"T69","span":{"begin":1291,"end":1292},"obj":"http://purl.obolibrary.org/obo/CLO_0001020"},{"id":"T70","span":{"begin":1585,"end":1586},"obj":"http://purl.obolibrary.org/obo/CLO_0001020"},{"id":"T71","span":{"begin":1730,"end":1736},"obj":"http://purl.obolibrary.org/obo/CLO_0007225"},{"id":"T72","span":{"begin":1860,"end":1861},"obj":"http://purl.obolibrary.org/obo/CLO_0001020"},{"id":"T73","span":{"begin":1886,"end":1889},"obj":"http://purl.obolibrary.org/obo/CLO_0054060"},{"id":"T74","span":{"begin":1965,"end":1971},"obj":"http://purl.obolibrary.org/obo/SO_0000418"},{"id":"T75","span":{"begin":2061,"end":2062},"obj":"http://purl.obolibrary.org/obo/CLO_0001020"},{"id":"T76","span":{"begin":2142,"end":2149},"obj":"http://purl.obolibrary.org/obo/CLO_0007225"},{"id":"T77","span":{"begin":2173,"end":2174},"obj":"http://purl.obolibrary.org/obo/CLO_0001020"},{"id":"T78","span":{"begin":2206,"end":2207},"obj":"http://purl.obolibrary.org/obo/CLO_0001021"},{"id":"T79","span":{"begin":2344,"end":2345},"obj":"http://purl.obolibrary.org/obo/CLO_0001020"},{"id":"T80","span":{"begin":2508,"end":2509},"obj":"http://purl.obolibrary.org/obo/CLO_0001021"},{"id":"T81","span":{"begin":2511,"end":2517},"obj":"http://purl.obolibrary.org/obo/SO_0000418"}],"text":"Here, we classify assays that use secondary binding steps as labeled approaches in Table 1, Table 2 regardless of if the primary binding step produced a response. There is, however, a more subtle distinction if binding of the secondary species is used for amplification or verification purposes as previously discussed. Labels often include a biorecognition element-enzyme or -nanoparticle conjugate. In electrochemical biosensing applications, such labels often serve the purpose of altering the material properties or transport processes of the electrode-electrolyte interface, often by inducing a secondary reaction. Secondary binding of optically-active nanomaterials to captured targets can also enable the use of optical transducers for simultaneous detection or bioanalysis. Enzymes are among the most commonly used secondary binding species for label-based pathogen detection. As shown in Table 2, electrochemical biosensors for pathogen detection that employ enzymes are commonly performed as a sandwich assay format. A schematic of secondary binding steps for biosensor amplification based on the binding of HRP-antibody conjugates is shown in Fig. 6 a (Kokkinos et al. 2016). Hong et al. used HRP-labeled secondary antibodies to amplify the CV and EIS responses of a concanavalin A-functionalized nanostructured Au electrode to detect norovirus (Hong et al. 2015). Gayathri et al. used an HRP-antibody conjugate to induce an enzyme-assisted reduction reaction with an immobilized thionine-antibody receptor in an H2O2 system for detection of E. coli down to 50 CFU/mL using a sandwich assay format (Gayathri et al. 2016). Xu et al. used glucose oxidase and monoclonal anti-S. typhimurium to functionalize magnetic bead labels for separation and detection of S. typhimurium on an Au IDAM using EIS and glucose to catalyze the reaction that exhibited a linear working range of 102 to 106 CFU/mL (Xu et al. 2016b).\nFig. 6 Highlight of secondary binding and signal amplification approaches utilized in electrochemical biosensor-based pathogen detection. a) Four amplification approaches associated with the secondary binding of enzyme-labeled secondary antibodies: (A) electron transfer mediation; (B) nanostructuring of surface for increased rate of charge transfer kinetics; (C) conversion of electrochemically inactive substrate into a detectable electroactive product; (D) catalysis of oxidation of glucose for production of hydrogen peroxide for electrochemical detection (Kokkinos et al. 2016). b) Signal amplification via non-selective binding of AuNPs to bound bacterial target (E. coli) (Wan et al. 2016)."}
LitCovid-PD-CHEBI
{"project":"LitCovid-PD-CHEBI","denotations":[{"id":"T85992","span":{"begin":377,"end":389},"obj":"Chemical"},{"id":"T79871","span":{"begin":853,"end":858},"obj":"Chemical"},{"id":"T38535","span":{"begin":1259,"end":1262},"obj":"Chemical"},{"id":"T3489","span":{"begin":1323,"end":1325},"obj":"Chemical"},{"id":"T53259","span":{"begin":1491,"end":1499},"obj":"Chemical"},{"id":"T27782","span":{"begin":1524,"end":1528},"obj":"Chemical"},{"id":"T3704","span":{"begin":1648,"end":1655},"obj":"Chemical"},{"id":"T88418","span":{"begin":1790,"end":1792},"obj":"Chemical"},{"id":"T37293","span":{"begin":1804,"end":1807},"obj":"Chemical"},{"id":"T37013","span":{"begin":1812,"end":1819},"obj":"Chemical"},{"id":"T63449","span":{"begin":2176,"end":2184},"obj":"Chemical"},{"id":"T53377","span":{"begin":2410,"end":2417},"obj":"Chemical"},{"id":"T87981","span":{"begin":2436,"end":2453},"obj":"Chemical"},{"id":"T8061","span":{"begin":2436,"end":2444},"obj":"Chemical"},{"id":"T99997","span":{"begin":2445,"end":2453},"obj":"Chemical"}],"attributes":[{"id":"A25631","pred":"chebi_id","subj":"T85992","obj":"http://purl.obolibrary.org/obo/CHEBI_50803"},{"id":"A31111","pred":"chebi_id","subj":"T79871","obj":"http://purl.obolibrary.org/obo/CHEBI_35209"},{"id":"A68607","pred":"chebi_id","subj":"T38535","obj":"http://purl.obolibrary.org/obo/CHEBI_73498"},{"id":"A10382","pred":"chebi_id","subj":"T3489","obj":"http://purl.obolibrary.org/obo/CHEBI_29287"},{"id":"A42819","pred":"chebi_id","subj":"T53259","obj":"http://purl.obolibrary.org/obo/CHEBI_52295"},{"id":"A87863","pred":"chebi_id","subj":"T27782","obj":"http://purl.obolibrary.org/obo/CHEBI_16240"},{"id":"A18008","pred":"chebi_id","subj":"T3704","obj":"http://purl.obolibrary.org/obo/CHEBI_17234"},{"id":"A47314","pred":"chebi_id","subj":"T3704","obj":"http://purl.obolibrary.org/obo/CHEBI_4167"},{"id":"A68157","pred":"chebi_id","subj":"T88418","obj":"http://purl.obolibrary.org/obo/CHEBI_29287"},{"id":"A51043","pred":"chebi_id","subj":"T37293","obj":"http://purl.obolibrary.org/obo/CHEBI_73498"},{"id":"A69207","pred":"chebi_id","subj":"T37013","obj":"http://purl.obolibrary.org/obo/CHEBI_17234"},{"id":"A61560","pred":"chebi_id","subj":"T37013","obj":"http://purl.obolibrary.org/obo/CHEBI_4167"},{"id":"A86942","pred":"chebi_id","subj":"T63449","obj":"http://purl.obolibrary.org/obo/CHEBI_10545"},{"id":"A30259","pred":"chebi_id","subj":"T53377","obj":"http://purl.obolibrary.org/obo/CHEBI_17234"},{"id":"A82684","pred":"chebi_id","subj":"T53377","obj":"http://purl.obolibrary.org/obo/CHEBI_4167"},{"id":"A49303","pred":"chebi_id","subj":"T87981","obj":"http://purl.obolibrary.org/obo/CHEBI_16240"},{"id":"A26576","pred":"chebi_id","subj":"T8061","obj":"http://purl.obolibrary.org/obo/CHEBI_49637"},{"id":"A24995","pred":"chebi_id","subj":"T99997","obj":"http://purl.obolibrary.org/obo/CHEBI_44785"}],"text":"Here, we classify assays that use secondary binding steps as labeled approaches in Table 1, Table 2 regardless of if the primary binding step produced a response. There is, however, a more subtle distinction if binding of the secondary species is used for amplification or verification purposes as previously discussed. Labels often include a biorecognition element-enzyme or -nanoparticle conjugate. In electrochemical biosensing applications, such labels often serve the purpose of altering the material properties or transport processes of the electrode-electrolyte interface, often by inducing a secondary reaction. Secondary binding of optically-active nanomaterials to captured targets can also enable the use of optical transducers for simultaneous detection or bioanalysis. Enzymes are among the most commonly used secondary binding species for label-based pathogen detection. As shown in Table 2, electrochemical biosensors for pathogen detection that employ enzymes are commonly performed as a sandwich assay format. A schematic of secondary binding steps for biosensor amplification based on the binding of HRP-antibody conjugates is shown in Fig. 6 a (Kokkinos et al. 2016). Hong et al. used HRP-labeled secondary antibodies to amplify the CV and EIS responses of a concanavalin A-functionalized nanostructured Au electrode to detect norovirus (Hong et al. 2015). Gayathri et al. used an HRP-antibody conjugate to induce an enzyme-assisted reduction reaction with an immobilized thionine-antibody receptor in an H2O2 system for detection of E. coli down to 50 CFU/mL using a sandwich assay format (Gayathri et al. 2016). Xu et al. used glucose oxidase and monoclonal anti-S. typhimurium to functionalize magnetic bead labels for separation and detection of S. typhimurium on an Au IDAM using EIS and glucose to catalyze the reaction that exhibited a linear working range of 102 to 106 CFU/mL (Xu et al. 2016b).\nFig. 6 Highlight of secondary binding and signal amplification approaches utilized in electrochemical biosensor-based pathogen detection. a) Four amplification approaches associated with the secondary binding of enzyme-labeled secondary antibodies: (A) electron transfer mediation; (B) nanostructuring of surface for increased rate of charge transfer kinetics; (C) conversion of electrochemically inactive substrate into a detectable electroactive product; (D) catalysis of oxidation of glucose for production of hydrogen peroxide for electrochemical detection (Kokkinos et al. 2016). b) Signal amplification via non-selective binding of AuNPs to bound bacterial target (E. coli) (Wan et al. 2016)."}
LitCovid-PD-GO-BP
{"project":"LitCovid-PD-GO-BP","denotations":[{"id":"T2","span":{"begin":520,"end":529},"obj":"http://purl.obolibrary.org/obo/GO_0006810"},{"id":"T3","span":{"begin":2176,"end":2193},"obj":"http://purl.obolibrary.org/obo/GO_0022904"}],"text":"Here, we classify assays that use secondary binding steps as labeled approaches in Table 1, Table 2 regardless of if the primary binding step produced a response. There is, however, a more subtle distinction if binding of the secondary species is used for amplification or verification purposes as previously discussed. Labels often include a biorecognition element-enzyme or -nanoparticle conjugate. In electrochemical biosensing applications, such labels often serve the purpose of altering the material properties or transport processes of the electrode-electrolyte interface, often by inducing a secondary reaction. Secondary binding of optically-active nanomaterials to captured targets can also enable the use of optical transducers for simultaneous detection or bioanalysis. Enzymes are among the most commonly used secondary binding species for label-based pathogen detection. As shown in Table 2, electrochemical biosensors for pathogen detection that employ enzymes are commonly performed as a sandwich assay format. A schematic of secondary binding steps for biosensor amplification based on the binding of HRP-antibody conjugates is shown in Fig. 6 a (Kokkinos et al. 2016). Hong et al. used HRP-labeled secondary antibodies to amplify the CV and EIS responses of a concanavalin A-functionalized nanostructured Au electrode to detect norovirus (Hong et al. 2015). Gayathri et al. used an HRP-antibody conjugate to induce an enzyme-assisted reduction reaction with an immobilized thionine-antibody receptor in an H2O2 system for detection of E. coli down to 50 CFU/mL using a sandwich assay format (Gayathri et al. 2016). Xu et al. used glucose oxidase and monoclonal anti-S. typhimurium to functionalize magnetic bead labels for separation and detection of S. typhimurium on an Au IDAM using EIS and glucose to catalyze the reaction that exhibited a linear working range of 102 to 106 CFU/mL (Xu et al. 2016b).\nFig. 6 Highlight of secondary binding and signal amplification approaches utilized in electrochemical biosensor-based pathogen detection. a) Four amplification approaches associated with the secondary binding of enzyme-labeled secondary antibodies: (A) electron transfer mediation; (B) nanostructuring of surface for increased rate of charge transfer kinetics; (C) conversion of electrochemically inactive substrate into a detectable electroactive product; (D) catalysis of oxidation of glucose for production of hydrogen peroxide for electrochemical detection (Kokkinos et al. 2016). b) Signal amplification via non-selective binding of AuNPs to bound bacterial target (E. coli) (Wan et al. 2016)."}
LitCovid-sentences
{"project":"LitCovid-sentences","denotations":[{"id":"T891","span":{"begin":0,"end":162},"obj":"Sentence"},{"id":"T892","span":{"begin":163,"end":319},"obj":"Sentence"},{"id":"T893","span":{"begin":320,"end":400},"obj":"Sentence"},{"id":"T894","span":{"begin":401,"end":619},"obj":"Sentence"},{"id":"T895","span":{"begin":620,"end":781},"obj":"Sentence"},{"id":"T896","span":{"begin":782,"end":884},"obj":"Sentence"},{"id":"T897","span":{"begin":885,"end":1026},"obj":"Sentence"},{"id":"T898","span":{"begin":1027,"end":1179},"obj":"Sentence"},{"id":"T899","span":{"begin":1180,"end":1186},"obj":"Sentence"},{"id":"T900","span":{"begin":1187,"end":1368},"obj":"Sentence"},{"id":"T901","span":{"begin":1369,"end":1375},"obj":"Sentence"},{"id":"T902","span":{"begin":1376,"end":1625},"obj":"Sentence"},{"id":"T903","span":{"begin":1626,"end":1632},"obj":"Sentence"},{"id":"T904","span":{"begin":1633,"end":1914},"obj":"Sentence"},{"id":"T905","span":{"begin":1915,"end":1922},"obj":"Sentence"},{"id":"T906","span":{"begin":1923,"end":2500},"obj":"Sentence"},{"id":"T907","span":{"begin":2501,"end":2614},"obj":"Sentence"},{"id":"T908","span":{"begin":2615,"end":2621},"obj":"Sentence"}],"namespaces":[{"prefix":"_base","uri":"http://pubannotation.org/ontology/tao.owl#"}],"text":"Here, we classify assays that use secondary binding steps as labeled approaches in Table 1, Table 2 regardless of if the primary binding step produced a response. There is, however, a more subtle distinction if binding of the secondary species is used for amplification or verification purposes as previously discussed. Labels often include a biorecognition element-enzyme or -nanoparticle conjugate. In electrochemical biosensing applications, such labels often serve the purpose of altering the material properties or transport processes of the electrode-electrolyte interface, often by inducing a secondary reaction. Secondary binding of optically-active nanomaterials to captured targets can also enable the use of optical transducers for simultaneous detection or bioanalysis. Enzymes are among the most commonly used secondary binding species for label-based pathogen detection. As shown in Table 2, electrochemical biosensors for pathogen detection that employ enzymes are commonly performed as a sandwich assay format. A schematic of secondary binding steps for biosensor amplification based on the binding of HRP-antibody conjugates is shown in Fig. 6 a (Kokkinos et al. 2016). Hong et al. used HRP-labeled secondary antibodies to amplify the CV and EIS responses of a concanavalin A-functionalized nanostructured Au electrode to detect norovirus (Hong et al. 2015). Gayathri et al. used an HRP-antibody conjugate to induce an enzyme-assisted reduction reaction with an immobilized thionine-antibody receptor in an H2O2 system for detection of E. coli down to 50 CFU/mL using a sandwich assay format (Gayathri et al. 2016). Xu et al. used glucose oxidase and monoclonal anti-S. typhimurium to functionalize magnetic bead labels for separation and detection of S. typhimurium on an Au IDAM using EIS and glucose to catalyze the reaction that exhibited a linear working range of 102 to 106 CFU/mL (Xu et al. 2016b).\nFig. 6 Highlight of secondary binding and signal amplification approaches utilized in electrochemical biosensor-based pathogen detection. a) Four amplification approaches associated with the secondary binding of enzyme-labeled secondary antibodies: (A) electron transfer mediation; (B) nanostructuring of surface for increased rate of charge transfer kinetics; (C) conversion of electrochemically inactive substrate into a detectable electroactive product; (D) catalysis of oxidation of glucose for production of hydrogen peroxide for electrochemical detection (Kokkinos et al. 2016). b) Signal amplification via non-selective binding of AuNPs to bound bacterial target (E. coli) (Wan et al. 2016)."}
2_test
{"project":"2_test","denotations":[{"id":"32364936-25254625-7713148","span":{"begin":1369,"end":1373},"obj":"25254625"},{"id":"32364936-27040944-7713149","span":{"begin":1626,"end":1630},"obj":"27040944"},{"id":"32364936-26796138-7713151","span":{"begin":2615,"end":2619},"obj":"26796138"}],"text":"Here, we classify assays that use secondary binding steps as labeled approaches in Table 1, Table 2 regardless of if the primary binding step produced a response. There is, however, a more subtle distinction if binding of the secondary species is used for amplification or verification purposes as previously discussed. Labels often include a biorecognition element-enzyme or -nanoparticle conjugate. In electrochemical biosensing applications, such labels often serve the purpose of altering the material properties or transport processes of the electrode-electrolyte interface, often by inducing a secondary reaction. Secondary binding of optically-active nanomaterials to captured targets can also enable the use of optical transducers for simultaneous detection or bioanalysis. Enzymes are among the most commonly used secondary binding species for label-based pathogen detection. As shown in Table 2, electrochemical biosensors for pathogen detection that employ enzymes are commonly performed as a sandwich assay format. A schematic of secondary binding steps for biosensor amplification based on the binding of HRP-antibody conjugates is shown in Fig. 6 a (Kokkinos et al. 2016). Hong et al. used HRP-labeled secondary antibodies to amplify the CV and EIS responses of a concanavalin A-functionalized nanostructured Au electrode to detect norovirus (Hong et al. 2015). Gayathri et al. used an HRP-antibody conjugate to induce an enzyme-assisted reduction reaction with an immobilized thionine-antibody receptor in an H2O2 system for detection of E. coli down to 50 CFU/mL using a sandwich assay format (Gayathri et al. 2016). Xu et al. used glucose oxidase and monoclonal anti-S. typhimurium to functionalize magnetic bead labels for separation and detection of S. typhimurium on an Au IDAM using EIS and glucose to catalyze the reaction that exhibited a linear working range of 102 to 106 CFU/mL (Xu et al. 2016b).\nFig. 6 Highlight of secondary binding and signal amplification approaches utilized in electrochemical biosensor-based pathogen detection. a) Four amplification approaches associated with the secondary binding of enzyme-labeled secondary antibodies: (A) electron transfer mediation; (B) nanostructuring of surface for increased rate of charge transfer kinetics; (C) conversion of electrochemically inactive substrate into a detectable electroactive product; (D) catalysis of oxidation of glucose for production of hydrogen peroxide for electrochemical detection (Kokkinos et al. 2016). b) Signal amplification via non-selective binding of AuNPs to bound bacterial target (E. coli) (Wan et al. 2016)."}