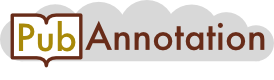
PMC:3543922 / 4495-8829
Annnotations
2_test
TEs Recently Inserted into the Human Genome
Comparative genomics allows us to investigate species-specific TEs. Through a combinational method of computational data mining and experimental verification, species-specific TEs have been well studied in various primate genomes, including human, chimpanzee, and rhesus macaque. During the past 6 million years, more than 10,000 human-specific TEs were newly inserted in the human [21]. The majority of the identified elements belong to Alu, L1, and SVA retrotransposons. These elements have contributed to the genomic difference between human and non-human primates through insertion and post-insertion recombination between the elements [8, 22].
Alu elements are most ubiquitous in the human genome. A master gene model has been generally accepted to explain the amplification of Alu elements. In the model, only limited numbers of hyperactive "source" or "master" genes are able to produce a high number of Alu copies over evolutionary time. One of the previous studies about the AluYb subfamily, one of the most active Alu subfamilies in the human genome, found that the copy number of AluYb elements is very different between human and non-human primates; a high copy number of AluYb elements exists in the human, but a very small number of the elements exists in non-human primates [23]. The oldest AluYb element resides at its orthologous position in all hominid primate genomes, demonstrating that the AluYb subfamily emerged 18 to 25 million years ago. Then, after approximately 20 million years of retrotranspositional quiescence, a major expansion of the subfamily occurred only in the human genome within the past several million years. To explain their successful proliferation in the human genome, a new model, "stealth driver," was introduced. In this model, a high copy of the Alu elements could be driven at least in part by "stealth driver" elements, which maintain low retrotransposition activity over extended periods of time. Although this element has low retrotransposition activity, they have the potential to produce short-lived hyperactive copies responsible for the remarkable expansion of AluYb elements within the human genome [24].
L1 is another successful element, occupying ~17% of the human genome. The copy number of Alu elements is much higher than that of L1 (Alu, ~1.2 million copies; L1, ~520,000 copies). Nonetheless, Alu elements are responsible for ~11% of the human genome, because the Alu element is much shorter than L1 (Alu average length, 300 bp; full-length of LINE, 6 kb) [25]. Through characterization of sequence diversity of chimpanzee-specific L1 subfamilies as compared to their human-specific counterparts, it was concluded that L1s experienced different evolutionary fates between humans and chimpanzees within the past ~6 million years. Although the species-specific L1 copy numbers were on the same order in the two species (1,800 human-specific L1s vs. 1,200 chimpanzee-specific L1s), the number of retrotransposition-competent elements was much higher in the human genome than in the chimpanzee genome. The species-specific L1s were grouped into several L1 subfamilies. All human L1 subfamilies belonged to a single lineage, but two distinct L1 lineages were identified in the chimpanzee genome [15].
SVA is shared only in human and apes. Fewer than 1,000 copy numbers were detected in orangutan (~15 million years ago), and no SVA detected in Old World monkeys indicating SVA is a hominid-specific element. Like Alu elements, SVA elements retrotranspose to another locus in trans by using reverse-transcriptase encoded by L1s [8]. Due to the limited mobilization and short evolutionary time, the copy number of SVA is very small compared to Alu and L1 elements [15, 26, 27]. There are six SVA subfamilies (SVA_A to SVA_F) in the human genome. The older, SVA_A to SVA_D, evolved in a single lineage, whereas human-specific SVA_E and SVA_F were derived independently from their ancestral sequences. The copy number of SVA was estimated throughout the primate genomes of human, chimpanzee, and gorilla, and there was no significant difference among them. The two elements, Alu and L1, showed a huge expansion at a specific evolutionary time along the primate lineage, but SVA still did not show any burst in its copy number [26, 27].