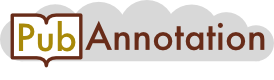
PMC:7643666 / 11719-19888
Annnotations
Zoonoses
{"project":"Zoonoses","denotations":[{"id":"T1","span":{"begin":2131,"end":2143},"obj":"Gene"},{"id":"T10","span":{"begin":449,"end":461},"obj":"Gene"},{"id":"T11","span":{"begin":707,"end":719},"obj":"Gene"},{"id":"T12","span":{"begin":1156,"end":1168},"obj":"Gene"},{"id":"T13","span":{"begin":1384,"end":1396},"obj":"Gene"},{"id":"T14","span":{"begin":2216,"end":2228},"obj":"Gene"},{"id":"T15","span":{"begin":2576,"end":2588},"obj":"Gene"},{"id":"T16","span":{"begin":2776,"end":2788},"obj":"Gene"},{"id":"T17","span":{"begin":5975,"end":5987},"obj":"Gene"},{"id":"T19","span":{"begin":2144,"end":2147},"obj":"Phenomena"},{"id":"T23","span":{"begin":2229,"end":2232},"obj":"Phenomena"},{"id":"T24","span":{"begin":7043,"end":7046},"obj":"Phenomena"},{"id":"T25","span":{"begin":7736,"end":7739},"obj":"Phenomena"},{"id":"T26","span":{"begin":8002,"end":8005},"obj":"Phenomena"},{"id":"T27","span":{"begin":8064,"end":8067},"obj":"Phenomena"},{"id":"T8","span":{"begin":38,"end":50},"obj":"Gene"},{"id":"T9","span":{"begin":342,"end":354},"obj":"Gene"}],"attributes":[{"id":"A17","pred":"tao:has_database_id","subj":"T17","obj":"Gene:43740568"},{"id":"A19","pred":"tao:has_database_id","subj":"T19","obj":"MESH:D000072417"},{"id":"A23","pred":"tao:has_database_id","subj":"T23","obj":"MESH:D000072417"},{"id":"A11","pred":"tao:has_database_id","subj":"T11","obj":"Gene:43740568"},{"id":"A13","pred":"tao:has_database_id","subj":"T13","obj":"Gene:43740568"},{"id":"A26","pred":"tao:has_database_id","subj":"T26","obj":"MESH:D000072417"},{"id":"A27","pred":"tao:has_database_id","subj":"T27","obj":"MESH:D000072417"},{"id":"A15","pred":"tao:has_database_id","subj":"T15","obj":"Gene:43740568"},{"id":"A9","pred":"tao:has_database_id","subj":"T9","obj":"Gene:43740568"},{"id":"A25","pred":"tao:has_database_id","subj":"T25","obj":"MESH:D000072417"},{"id":"A12","pred":"tao:has_database_id","subj":"T12","obj":"Gene:43740568"},{"id":"A16","pred":"tao:has_database_id","subj":"T16","obj":"Gene:43740568"},{"id":"A10","pred":"tao:has_database_id","subj":"T10","obj":"Gene:43740568"},{"id":"A8","pred":"tao:has_database_id","subj":"T8","obj":"Gene:43740568"},{"id":"A1","pred":"tao:has_database_id","subj":"T1","obj":"Gene:43740568"},{"id":"A24","pred":"tao:has_database_id","subj":"T24","obj":"MESH:D000072417"},{"id":"A14","pred":"tao:has_database_id","subj":"T14","obj":"Gene:43740568"}],"text":"3 Results\nBased on the evidence that SARS-CoV-2 S derives from the bat [19], the first step of our study was aimed to identify Chiroptera living in Italy that may be new reservoirs of SARS-CoV-2 and, as such, responsible for new outbreaks in Italy.\nhACE2 receptor is essential for infection of SARS-CoV-2 in humans. The crystal structure of SARS-CoV-2 S receptor bound to ACE2 has been recently solved [9]. In a preliminary step, we analyzed hACE2/SARS-CoV-2 S complex and identified in hACE2 the residues K31, H34, E37, D38, Q42, K353, and N330 as those critical for the interaction with S protein; among them, K31 and K353, revealed to be critical for S recognition [1].\nThe study of the binding mode of SARS-CoV-2 S protein in complex with ACE2 of Chiroptera may offer important information on the possibility that these species could be a reservoir for the virus. Using ExPASy SWISS-MODEL and having as a template the crystallographic coordinates of hACE2 (PDB ID: 6M0J) [9] we calculated the homology models of the ACE2 proteins presenting not less than 70% sequence homology with hACE2 (Figure 2B). These were subjected to molecular docking against SARS-CoV-2 S protein, imposing as restraints the distances with K31 and K353, that are the most critical residues for S recognition. The data resulting from molecular docking indicates similar binding stability for all the ACE2/SARS-CoV-2 S protein complexes (see Supplementary Table S2). Analysis of the structural models evidences the conservation of the residues within the binding site, highlighting a critical similarity of hACE2 with the ACE2 of the Chiroptera species. In particular, we focused on ACE2 of Myotis daubentonii and Rhinolophus ferrumequinum. These are the solely ACE2 sequences retrieved from the UniProt database that belong to Chiroptera species living in Italy. The homology models of ACE2 belonging to Chiroptera species in complex with S protein were subjected to 1 ns molecular dynamics simulations in water using protein S/hACE2 complex (PDB ID: 6M0J) as control.\nFigure 2 (A) Rhinolophus ferrumequinum and Myotis daubentonii ACE2 in complex with SARS-CoV-2 S RBD. The structures are superimposed to hACE2 structure in complex with SARS-CoV-2 S RBD. The data, extracted from the last step of molecular dynamics, are relative to 1–358 ACE2 residues. (B) Multiple sequence alignment including hACE2 and ACE2 of Rhinolophus ferrumequinum and Myotis daubentonii. Residues involved in the interaction with S protein are shown: the residue numbers in red represent the residues essential for hACE2/SARS-CoV-2 S binding. Conserved residues are highlighted in blue, while similar residues are highlighted in green. Bold black residue numbers indicate amino acids essential for the stability of hACE2/SARS-CoV-2 S interaction.\nAnalysis of molecular dynamics considering the variation of RMSD during the time indicated each complex reaching a conformational steady-state, as observed from the presence of RMSD plateau (see Supplementary Figure S1) [35]. In Figure 2A the structural coordinates of Myotis daubentonii and Rhinolophus ferrumequinum ACE2 derived from the last step of molecular dynamics are superimposed to the crystal structure of hACE2. The poses are very similar (RMSD on backbone heavy atoms: 5.83 Å) and the residues defining the protein-protein interaction framework are almost conserved (see Supplementary Tables S3–S7, Figures S2–S6).\nFigure 2B shows the sequence alignment of 1–358 residues of hACE2 and ACE2 of Myotis daubentonii and Rhinolophus ferrumequinum (Omega Clustal). 78.77% sequence identity is observable for Myotis daubentonii, 77.93% for Rhinolophus ferrumequinum (UniProt ID: E2DHI2), and 81.24% for Rhinolophus ferrumequinum (UniProt ID: B6ZGN7). The identity refers to all the residues ranging from 1 to 358. Most of the residues essential for the binding with S protein are conserved. In particular, Rhinolophus ferrumequinum preserves the residues E37, Q42, N330, and K353, with D38 (Homo sapiens) replaced by N38 (Rhinolophus ferrumequinum), which conserves the physical-chemical properties of the amino acid. Myotis daubentonii preserved H34, E37, D38, and K353, with K31 and Q42 (Homo sapiens) replaced by N31 and E42 (Myotis daubentonii), respectively. Considering these results together with the data deriving from in silico calculations, Rhinolophus ferrumequinum is to be considered a potential primary animal reservoir within the Italian distribution area [37].\nOnce we identified Rhinolophus ferrumequinum as a potential primary reservoir of SARS-CoV-2 in Italy [19], the objective of our study was to identify a hypothetical secondary animal reservoir in the Italian territory. If confirmed by experimental data, this information is critical for predicting a possible contagion line in the wild and domestic fauna present in Italy.\nResidues 1–358 of ACE2 sequences of Rhinolophus ferrumequinum (UniProt ID: E2DHI2 and B6ZGN7), Myotis daubentonii (UniProt ID: E2DHI8) and Homo sapiens (UniProt ID: Q9BYF1) were used as a template in a similarity search (UniProt database) to identify ACE2 sequences of wild and domestic animals present in the Italian region. As a result, ACE2 sequences of 10 wild and domestic animal species present in Italy were selected, showing no less than 70% identity on 1–358 residues (Figure 3).\nFigure 3 Multiple sequence alignments including ACE2 amino acid sequences of Rhinolophus ferrumequinum (UniProt ID: E2DHI2 and B6ZGN7) (A–B), Myotis daubentonii (UniProt ID: E2DHI8) (C), with ACE2 amino acid sequences of wild and domestic animal species present in Italy. The residues in blue are preserved, the residues in green have similar chemical characteristics.\nFigure 3B indicates that the binding sites of ACE2 sequences in domestic and wild animal species show the highest number of conserved residues if considered in comparison to ACE2 of Rhinolophus ferrumequinum: the binding site of Rhinolophus ferrumequinum ACE2/SARS-CoV-2 S complex has \u003e80% sequence identity with the binding site of domestic and wild animal species present in Italy.\nTo evaluate the possibility that the selected wild and domestic animals may be the second reservoir of a mutated form of coronavirus infection, we simulated the interaction between ACE2 of domestic and wild animal species present in Italy (Figure 3B) and Bat-CoV spike protein.\nPreliminarily, homology models of the SARS-like bat coronavirus (Bat-CoV/Rp3/2004) S protein and ACE2 proteins of domestic and wild animals were built (see Supplementary Table S8). After, molecular docking calculations were carried out imposing intermolecular distance restraints to preserve the binding poses: 5 Å distance was imposed between ACE2 E37 and Bat-CoV S protein Y477. 1 ns molecular dynamics performed on all the structural models revealed conformational stability consistent with RMSD plateau in RMSD vs time plot. (see Supplementary Figure S7, Table S9).\nIn Figure 4A, the structure of Rhinolophus ferrumequinum ACE2 in complex with Bt-CoV/Rp3/2004 S RBD is superimposed to ACE2 structures (residues 1–358) of domestic and wild animal species as derived from the last step of 1 ns molecular dynamics. The ACE2 structures are very similar (RMSD on backbone heavy atoms: 4.76 Å), suggesting that the BtCoV/Rp3/2004 S protein is potentially adapt to undertake efficacious interaction with ACE2 of domestic and wild animals. In Figure 4B multiple sequence alignments indicate that ACE2 residues involved in the interaction with BtCoV/Rp3/2004 S protein are conserved in all domestic and wild species except for Canis lupus familiaris and Vulpes vulpes.\nFigure 4 (A) The structure of Rhinolophus ferrumequinum ACE2 in complex with Bt-CoV/Rp3/2004 S RBD is superimposed to ACE2 (residues 1–358) of domestic and wild animal species as derived from the last step of 1 ns molecular dynamics ribbon representation. (B) Residues of ACE2 (belonging to Italian domestic and wild animals) interacting with Bt-CoV/Rp3/2004 S RBD, compared to residues of hACE2 interacting with S protein RBD. Residues highlighted in blue are preserved; residues in green have similar chemical characteristics."}
Covid19_manual_annotation_v2
{"project":"Covid19_manual_annotation_v2","denotations":[{"id":"T1","span":{"begin":2131,"end":2143},"obj":"Gene"},{"id":"T8","span":{"begin":38,"end":50},"obj":"Gene"},{"id":"T9","span":{"begin":342,"end":354},"obj":"Gene"},{"id":"T10","span":{"begin":449,"end":461},"obj":"Gene"},{"id":"T11","span":{"begin":707,"end":719},"obj":"Gene"},{"id":"T12","span":{"begin":1156,"end":1168},"obj":"Gene"},{"id":"T13","span":{"begin":1384,"end":1396},"obj":"Gene"},{"id":"T14","span":{"begin":2216,"end":2228},"obj":"Gene"},{"id":"T15","span":{"begin":2576,"end":2588},"obj":"Gene"},{"id":"T16","span":{"begin":2776,"end":2788},"obj":"Gene"},{"id":"T17","span":{"begin":5975,"end":5987},"obj":"Gene"},{"id":"T19","span":{"begin":2144,"end":2147},"obj":"Phenomena"},{"id":"T23","span":{"begin":2229,"end":2232},"obj":"Phenomena"},{"id":"T24","span":{"begin":7043,"end":7046},"obj":"Phenomena"},{"id":"T25","span":{"begin":7736,"end":7739},"obj":"Phenomena"},{"id":"T26","span":{"begin":8002,"end":8005},"obj":"Phenomena"},{"id":"T27","span":{"begin":8064,"end":8067},"obj":"Phenomena"}],"attributes":[{"id":"A8","pred":"tao:has_database_id","subj":"T8","obj":"Gene:43740568"},{"id":"A17","pred":"tao:has_database_id","subj":"T17","obj":"Gene:43740568"},{"id":"A19","pred":"tao:has_database_id","subj":"T19","obj":"MESH:D000072417"},{"id":"A1","pred":"tao:has_database_id","subj":"T1","obj":"Gene:43740568"},{"id":"A15","pred":"tao:has_database_id","subj":"T15","obj":"Gene:43740568"},{"id":"A24","pred":"tao:has_database_id","subj":"T24","obj":"MESH:D000072417"},{"id":"A9","pred":"tao:has_database_id","subj":"T9","obj":"Gene:43740568"},{"id":"A12","pred":"tao:has_database_id","subj":"T12","obj":"Gene:43740568"},{"id":"A16","pred":"tao:has_database_id","subj":"T16","obj":"Gene:43740568"},{"id":"A26","pred":"tao:has_database_id","subj":"T26","obj":"MESH:D000072417"},{"id":"A11","pred":"tao:has_database_id","subj":"T11","obj":"Gene:43740568"},{"id":"A14","pred":"tao:has_database_id","subj":"T14","obj":"Gene:43740568"},{"id":"A13","pred":"tao:has_database_id","subj":"T13","obj":"Gene:43740568"},{"id":"A25","pred":"tao:has_database_id","subj":"T25","obj":"MESH:D000072417"},{"id":"A27","pred":"tao:has_database_id","subj":"T27","obj":"MESH:D000072417"},{"id":"A10","pred":"tao:has_database_id","subj":"T10","obj":"Gene:43740568"},{"id":"A23","pred":"tao:has_database_id","subj":"T23","obj":"MESH:D000072417"}],"namespaces":[{"prefix":"Tax","uri":"https://www.ncbi.nlm.nih.gov/taxonomy/"},{"prefix":"MESH","uri":"https://id.nlm.nih.gov/mesh/"},{"prefix":"Gene","uri":"https://www.ncbi.nlm.nih.gov/gene/"},{"prefix":"BioProject","uri":"https://www.ncbi.nlm.nih.gov/bioproject/"},{"prefix":"CVCL","uri":"https://web.expasy.org/cellosaurus/CVCL_"},{"prefix":"HP","uri":"https://hpo.jax.org/app/browse/term/HP:"},{"prefix":"BioSample","uri":"https://www.ncbi.nlm.nih.gov/biosample/"}],"text":"3 Results\nBased on the evidence that SARS-CoV-2 S derives from the bat [19], the first step of our study was aimed to identify Chiroptera living in Italy that may be new reservoirs of SARS-CoV-2 and, as such, responsible for new outbreaks in Italy.\nhACE2 receptor is essential for infection of SARS-CoV-2 in humans. The crystal structure of SARS-CoV-2 S receptor bound to ACE2 has been recently solved [9]. In a preliminary step, we analyzed hACE2/SARS-CoV-2 S complex and identified in hACE2 the residues K31, H34, E37, D38, Q42, K353, and N330 as those critical for the interaction with S protein; among them, K31 and K353, revealed to be critical for S recognition [1].\nThe study of the binding mode of SARS-CoV-2 S protein in complex with ACE2 of Chiroptera may offer important information on the possibility that these species could be a reservoir for the virus. Using ExPASy SWISS-MODEL and having as a template the crystallographic coordinates of hACE2 (PDB ID: 6M0J) [9] we calculated the homology models of the ACE2 proteins presenting not less than 70% sequence homology with hACE2 (Figure 2B). These were subjected to molecular docking against SARS-CoV-2 S protein, imposing as restraints the distances with K31 and K353, that are the most critical residues for S recognition. The data resulting from molecular docking indicates similar binding stability for all the ACE2/SARS-CoV-2 S protein complexes (see Supplementary Table S2). Analysis of the structural models evidences the conservation of the residues within the binding site, highlighting a critical similarity of hACE2 with the ACE2 of the Chiroptera species. In particular, we focused on ACE2 of Myotis daubentonii and Rhinolophus ferrumequinum. These are the solely ACE2 sequences retrieved from the UniProt database that belong to Chiroptera species living in Italy. The homology models of ACE2 belonging to Chiroptera species in complex with S protein were subjected to 1 ns molecular dynamics simulations in water using protein S/hACE2 complex (PDB ID: 6M0J) as control.\nFigure 2 (A) Rhinolophus ferrumequinum and Myotis daubentonii ACE2 in complex with SARS-CoV-2 S RBD. The structures are superimposed to hACE2 structure in complex with SARS-CoV-2 S RBD. The data, extracted from the last step of molecular dynamics, are relative to 1–358 ACE2 residues. (B) Multiple sequence alignment including hACE2 and ACE2 of Rhinolophus ferrumequinum and Myotis daubentonii. Residues involved in the interaction with S protein are shown: the residue numbers in red represent the residues essential for hACE2/SARS-CoV-2 S binding. Conserved residues are highlighted in blue, while similar residues are highlighted in green. Bold black residue numbers indicate amino acids essential for the stability of hACE2/SARS-CoV-2 S interaction.\nAnalysis of molecular dynamics considering the variation of RMSD during the time indicated each complex reaching a conformational steady-state, as observed from the presence of RMSD plateau (see Supplementary Figure S1) [35]. In Figure 2A the structural coordinates of Myotis daubentonii and Rhinolophus ferrumequinum ACE2 derived from the last step of molecular dynamics are superimposed to the crystal structure of hACE2. The poses are very similar (RMSD on backbone heavy atoms: 5.83 Å) and the residues defining the protein-protein interaction framework are almost conserved (see Supplementary Tables S3–S7, Figures S2–S6).\nFigure 2B shows the sequence alignment of 1–358 residues of hACE2 and ACE2 of Myotis daubentonii and Rhinolophus ferrumequinum (Omega Clustal). 78.77% sequence identity is observable for Myotis daubentonii, 77.93% for Rhinolophus ferrumequinum (UniProt ID: E2DHI2), and 81.24% for Rhinolophus ferrumequinum (UniProt ID: B6ZGN7). The identity refers to all the residues ranging from 1 to 358. Most of the residues essential for the binding with S protein are conserved. In particular, Rhinolophus ferrumequinum preserves the residues E37, Q42, N330, and K353, with D38 (Homo sapiens) replaced by N38 (Rhinolophus ferrumequinum), which conserves the physical-chemical properties of the amino acid. Myotis daubentonii preserved H34, E37, D38, and K353, with K31 and Q42 (Homo sapiens) replaced by N31 and E42 (Myotis daubentonii), respectively. Considering these results together with the data deriving from in silico calculations, Rhinolophus ferrumequinum is to be considered a potential primary animal reservoir within the Italian distribution area [37].\nOnce we identified Rhinolophus ferrumequinum as a potential primary reservoir of SARS-CoV-2 in Italy [19], the objective of our study was to identify a hypothetical secondary animal reservoir in the Italian territory. If confirmed by experimental data, this information is critical for predicting a possible contagion line in the wild and domestic fauna present in Italy.\nResidues 1–358 of ACE2 sequences of Rhinolophus ferrumequinum (UniProt ID: E2DHI2 and B6ZGN7), Myotis daubentonii (UniProt ID: E2DHI8) and Homo sapiens (UniProt ID: Q9BYF1) were used as a template in a similarity search (UniProt database) to identify ACE2 sequences of wild and domestic animals present in the Italian region. As a result, ACE2 sequences of 10 wild and domestic animal species present in Italy were selected, showing no less than 70% identity on 1–358 residues (Figure 3).\nFigure 3 Multiple sequence alignments including ACE2 amino acid sequences of Rhinolophus ferrumequinum (UniProt ID: E2DHI2 and B6ZGN7) (A–B), Myotis daubentonii (UniProt ID: E2DHI8) (C), with ACE2 amino acid sequences of wild and domestic animal species present in Italy. The residues in blue are preserved, the residues in green have similar chemical characteristics.\nFigure 3B indicates that the binding sites of ACE2 sequences in domestic and wild animal species show the highest number of conserved residues if considered in comparison to ACE2 of Rhinolophus ferrumequinum: the binding site of Rhinolophus ferrumequinum ACE2/SARS-CoV-2 S complex has \u003e80% sequence identity with the binding site of domestic and wild animal species present in Italy.\nTo evaluate the possibility that the selected wild and domestic animals may be the second reservoir of a mutated form of coronavirus infection, we simulated the interaction between ACE2 of domestic and wild animal species present in Italy (Figure 3B) and Bat-CoV spike protein.\nPreliminarily, homology models of the SARS-like bat coronavirus (Bat-CoV/Rp3/2004) S protein and ACE2 proteins of domestic and wild animals were built (see Supplementary Table S8). After, molecular docking calculations were carried out imposing intermolecular distance restraints to preserve the binding poses: 5 Å distance was imposed between ACE2 E37 and Bat-CoV S protein Y477. 1 ns molecular dynamics performed on all the structural models revealed conformational stability consistent with RMSD plateau in RMSD vs time plot. (see Supplementary Figure S7, Table S9).\nIn Figure 4A, the structure of Rhinolophus ferrumequinum ACE2 in complex with Bt-CoV/Rp3/2004 S RBD is superimposed to ACE2 structures (residues 1–358) of domestic and wild animal species as derived from the last step of 1 ns molecular dynamics. The ACE2 structures are very similar (RMSD on backbone heavy atoms: 4.76 Å), suggesting that the BtCoV/Rp3/2004 S protein is potentially adapt to undertake efficacious interaction with ACE2 of domestic and wild animals. In Figure 4B multiple sequence alignments indicate that ACE2 residues involved in the interaction with BtCoV/Rp3/2004 S protein are conserved in all domestic and wild species except for Canis lupus familiaris and Vulpes vulpes.\nFigure 4 (A) The structure of Rhinolophus ferrumequinum ACE2 in complex with Bt-CoV/Rp3/2004 S RBD is superimposed to ACE2 (residues 1–358) of domestic and wild animal species as derived from the last step of 1 ns molecular dynamics ribbon representation. (B) Residues of ACE2 (belonging to Italian domestic and wild animals) interacting with Bt-CoV/Rp3/2004 S RBD, compared to residues of hACE2 interacting with S protein RBD. Residues highlighted in blue are preserved; residues in green have similar chemical characteristics."}