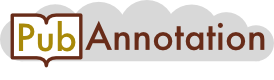
PMC:7565482 / 1731-7436
Annnotations
LitCovid-PD-FMA-UBERON
{"project":"LitCovid-PD-FMA-UBERON","denotations":[{"id":"T7","span":{"begin":904,"end":909},"obj":"Body_part"},{"id":"T8","span":{"begin":1107,"end":1111},"obj":"Body_part"},{"id":"T9","span":{"begin":1266,"end":1270},"obj":"Body_part"},{"id":"T10","span":{"begin":1486,"end":1490},"obj":"Body_part"},{"id":"T11","span":{"begin":1888,"end":1899},"obj":"Body_part"},{"id":"T12","span":{"begin":1933,"end":1937},"obj":"Body_part"},{"id":"T13","span":{"begin":2193,"end":2201},"obj":"Body_part"},{"id":"T14","span":{"begin":2445,"end":2454},"obj":"Body_part"},{"id":"T15","span":{"begin":2464,"end":2467},"obj":"Body_part"},{"id":"T16","span":{"begin":2699,"end":2707},"obj":"Body_part"},{"id":"T17","span":{"begin":2851,"end":2855},"obj":"Body_part"},{"id":"T18","span":{"begin":2914,"end":2918},"obj":"Body_part"},{"id":"T19","span":{"begin":2967,"end":2975},"obj":"Body_part"},{"id":"T20","span":{"begin":3082,"end":3086},"obj":"Body_part"},{"id":"T21","span":{"begin":3275,"end":3279},"obj":"Body_part"},{"id":"T22","span":{"begin":3453,"end":3457},"obj":"Body_part"},{"id":"T23","span":{"begin":4407,"end":4410},"obj":"Body_part"},{"id":"T24","span":{"begin":4563,"end":4567},"obj":"Body_part"},{"id":"T25","span":{"begin":4651,"end":4656},"obj":"Body_part"},{"id":"T26","span":{"begin":4814,"end":4818},"obj":"Body_part"},{"id":"T27","span":{"begin":4860,"end":4864},"obj":"Body_part"},{"id":"T28","span":{"begin":5350,"end":5356},"obj":"Body_part"},{"id":"T29","span":{"begin":5435,"end":5439},"obj":"Body_part"},{"id":"T30","span":{"begin":5579,"end":5583},"obj":"Body_part"}],"attributes":[{"id":"A7","pred":"fma_id","subj":"T7","obj":"http://purl.org/sig/ont/fma/fma68646"},{"id":"A8","pred":"fma_id","subj":"T8","obj":"http://purl.org/sig/ont/fma/fma68646"},{"id":"A9","pred":"fma_id","subj":"T9","obj":"http://purl.org/sig/ont/fma/fma68646"},{"id":"A10","pred":"fma_id","subj":"T10","obj":"http://purl.org/sig/ont/fma/fma68646"},{"id":"A11","pred":"fma_id","subj":"T11","obj":"http://purl.org/sig/ont/fma/fma82739"},{"id":"A12","pred":"fma_id","subj":"T12","obj":"http://purl.org/sig/ont/fma/fma68646"},{"id":"A13","pred":"fma_id","subj":"T13","obj":"http://purl.org/sig/ont/fma/fma67257"},{"id":"A14","pred":"fma_id","subj":"T14","obj":"http://purl.org/sig/ont/fma/fma62852"},{"id":"A15","pred":"fma_id","subj":"T15","obj":"http://purl.org/sig/ont/fma/fma84795"},{"id":"A16","pred":"fma_id","subj":"T16","obj":"http://purl.org/sig/ont/fma/fma67257"},{"id":"A17","pred":"fma_id","subj":"T17","obj":"http://purl.org/sig/ont/fma/fma68646"},{"id":"A18","pred":"fma_id","subj":"T18","obj":"http://purl.org/sig/ont/fma/fma68646"},{"id":"A19","pred":"fma_id","subj":"T19","obj":"http://purl.org/sig/ont/fma/fma67257"},{"id":"A20","pred":"fma_id","subj":"T20","obj":"http://purl.org/sig/ont/fma/fma68646"},{"id":"A21","pred":"fma_id","subj":"T21","obj":"http://purl.org/sig/ont/fma/fma68646"},{"id":"A22","pred":"fma_id","subj":"T22","obj":"http://purl.org/sig/ont/fma/fma68646"},{"id":"A23","pred":"fma_id","subj":"T23","obj":"http://purl.org/sig/ont/fma/fma278683"},{"id":"A24","pred":"fma_id","subj":"T24","obj":"http://purl.org/sig/ont/fma/fma68646"},{"id":"A25","pred":"fma_id","subj":"T25","obj":"http://purl.org/sig/ont/fma/fma68646"},{"id":"A26","pred":"fma_id","subj":"T26","obj":"http://purl.org/sig/ont/fma/fma68646"},{"id":"A27","pred":"fma_id","subj":"T27","obj":"http://purl.org/sig/ont/fma/fma68646"},{"id":"A28","pred":"fma_id","subj":"T28","obj":"http://purl.org/sig/ont/fma/fma84116"},{"id":"A29","pred":"fma_id","subj":"T29","obj":"http://purl.org/sig/ont/fma/fma68646"},{"id":"A30","pred":"fma_id","subj":"T30","obj":"http://purl.org/sig/ont/fma/fma68646"}],"text":"1. Introduction\nSince the start of the COVID-19 pandemic in December 2019, researchers around the world have put major efforts towards a better understanding of the immune response to its causative agent, the SARS-CoV-2. Although an impressive amount of scientific information has been generated in a very short period of time, there remain significant gaps in our understanding of SARS-CoV-2 immune control. In particular, it remains unclear what kind of adaptive immunity should be triggered by vaccination in order to achieve sterile immunity, or at least lead to an ameliorated disease course, in cases where vaccination cannot provide absolute protection from infection. We know from the available literature on other coronaviruses (mainly SARS-CoV-1 and MERS) that antibodies can neutralize the infection, although these humoral responses are short lived in many individuals, and that long-lived T cells responses are present in people with less severe disease outcomes [1,2,3,4,5]. The emerging data on the immune response to SARS-CoV-2 demonstrate the essential contribution of the virus-specific T-cell responses, possibly in addition to the action of neutralizing antibodies, in viral control [3,6,7,8,9,10,11,12,13]. Thus, improved tools to assess host T cell immunity in detail are urgently needed to better identify these responses and to define their role in the outcome of SARS-CoV-2 infection.\nEx-vivo immune analyses of samples from infected individuals can identify T cell responses to specific pathogens like viruses. Such analyses can help to better understand the role of host immunity in virus control and to guide successful vaccine development. However, they rely on the use of the correct recall antigens that can elicit specific responses in vitro. The urgency of the current SARS-CoV-2 pandemic has led researchers to tackle the problem of screening the 10,000 amino acids of the SARS-CoV-2 proteome for T cell responses by selecting viral sequences based on different criteria: (i) bioinformatically predicted epitopes, (ii) homology of SARS-CoV-2 sequences with epitopes defined in other coronaviruses (mainly SARS-CoV) or (iii) selecting some specific SARS-CoV-2 proteins over others [5,7,9,11,14,15,16,17,18,19]. However, all these approaches have intrinsic limitations. Bioinformatic prediction tools are trained on sets of previously described epitopes, but since the available epitope repertoire for many human leukocyte antigen (HLA) alleles is limited, its prediction capacity is also limited [20,21]. Inferences based on epitope sequence homology with other coronaviruses are hampered because past studies on SARS-CoV-1 and MERS only included few selected viral proteins. This is of concern, since screening only a part of the SARS-CoV-2 proteome will potentially miss an important portion of the virus-specific T cell response. Indeed, recent data indicate the existence of T cell responses against structural and non-structural proteins [5,9] for SARS-CoV-2 and other viral infections [22]. Finally, no study has considered the existence of T cell responses to epitopes encoded by open-reading frames (ORF) in alternative frames, as reported for other viral infections [23,24,25,26].\nIn order to reliably measure total virus-specific T cell immunity, the recall antigens used need to be as representative as possible of the worldwide viral sequences, even for genetically more stable viruses like coronaviruses. T cell recognition of epitopes is very sensitive to mismatches and not matching the recall antigen with the autologous virus can lead to missed responses [27]. For this reason, different test antigen design strategies, trying to cope with the diversity of circulating viral isolates in a single sequence, have been developed in the past. These strategies include central sequence designs such as Center of Tree (COT) [28,29,30,31,32], Ancestral [33,34,35,36] or Consensus sequences [29,30,31,32,35,37,38,39,40,41,42,43]; which may (Ancestral, COT) or may not (Consensus) represent naturally occurring sequences of replication competent viruses. All these designs are sensitive to the underlying sequence database and may change over time as new sequence information on additional isolates becomes available. Direct comparisons of these different central sequence approaches have been performed for a highly variable pathogen (human immunodeficiency virus, HIV) and shown that the different designs yielded comparable results when synthetic peptides covering these sequences were used to measure virus-specific T cell responses [42,43]. However, the additional costs in terms of peptide synthesis and cells needed for ex-vivo experiments, may not warrant inclusion of all the different variants into a single test set.\nThus, the characterization of the complete T cell responses to SARS-CoV-2 urgently needs T cell antigens that cover the whole SARS-CoV-2 proteome while covering sequence diversity, and which can be combined in different experimental set-ups and immune assays. To this end, we created a consensus sequence to cover the genetic diversity of SARS-CoV-2 (CoV-2-cons) for all ORF, including those described in alternative open reading frames. Given the computational ease for its initial generation and periodic updates, we designed a consensus sequence using more than 1700 CoV-2 full-genome sequences and designed overlapping peptide (OLP) sets as recall antigens in T cell assays. The CoV-2-cons OLP sets are presented here in different designs, balancing costs for synthesis with the sensitivity of detecting T cell responses and with the intention to provide a common test antigen that will allow data comparability across laboratories."}
LitCovid-PD-MONDO
{"project":"LitCovid-PD-MONDO","denotations":[{"id":"T10","span":{"begin":39,"end":47},"obj":"Disease"},{"id":"T11","span":{"begin":209,"end":217},"obj":"Disease"},{"id":"T12","span":{"begin":382,"end":390},"obj":"Disease"},{"id":"T13","span":{"begin":529,"end":536},"obj":"Disease"},{"id":"T14","span":{"begin":665,"end":674},"obj":"Disease"},{"id":"T15","span":{"begin":745,"end":753},"obj":"Disease"},{"id":"T16","span":{"begin":801,"end":810},"obj":"Disease"},{"id":"T17","span":{"begin":1033,"end":1041},"obj":"Disease"},{"id":"T18","span":{"begin":1388,"end":1396},"obj":"Disease"},{"id":"T19","span":{"begin":1399,"end":1408},"obj":"Disease"},{"id":"T20","span":{"begin":1802,"end":1810},"obj":"Disease"},{"id":"T21","span":{"begin":1907,"end":1915},"obj":"Disease"},{"id":"T22","span":{"begin":2065,"end":2073},"obj":"Disease"},{"id":"T23","span":{"begin":2139,"end":2147},"obj":"Disease"},{"id":"T24","span":{"begin":2182,"end":2190},"obj":"Disease"},{"id":"T25","span":{"begin":2646,"end":2654},"obj":"Disease"},{"id":"T26","span":{"begin":2764,"end":2772},"obj":"Disease"},{"id":"T27","span":{"begin":2986,"end":2994},"obj":"Disease"},{"id":"T28","span":{"begin":3007,"end":3023},"obj":"Disease"},{"id":"T29","span":{"begin":3191,"end":3207},"obj":"Disease"},{"id":"T30","span":{"begin":4383,"end":4399},"obj":"Disease"},{"id":"T31","span":{"begin":4832,"end":4840},"obj":"Disease"},{"id":"T32","span":{"begin":4895,"end":4903},"obj":"Disease"},{"id":"T33","span":{"begin":5108,"end":5116},"obj":"Disease"}],"attributes":[{"id":"A10","pred":"mondo_id","subj":"T10","obj":"http://purl.obolibrary.org/obo/MONDO_0100096"},{"id":"A11","pred":"mondo_id","subj":"T11","obj":"http://purl.obolibrary.org/obo/MONDO_0005091"},{"id":"A12","pred":"mondo_id","subj":"T12","obj":"http://purl.obolibrary.org/obo/MONDO_0005091"},{"id":"A13","pred":"mondo_id","subj":"T13","obj":"http://purl.obolibrary.org/obo/MONDO_0005047"},{"id":"A14","pred":"mondo_id","subj":"T14","obj":"http://purl.obolibrary.org/obo/MONDO_0005550"},{"id":"A15","pred":"mondo_id","subj":"T15","obj":"http://purl.obolibrary.org/obo/MONDO_0005091"},{"id":"A16","pred":"mondo_id","subj":"T16","obj":"http://purl.obolibrary.org/obo/MONDO_0005550"},{"id":"A17","pred":"mondo_id","subj":"T17","obj":"http://purl.obolibrary.org/obo/MONDO_0005091"},{"id":"A18","pred":"mondo_id","subj":"T18","obj":"http://purl.obolibrary.org/obo/MONDO_0005091"},{"id":"A19","pred":"mondo_id","subj":"T19","obj":"http://purl.obolibrary.org/obo/MONDO_0005550"},{"id":"A20","pred":"mondo_id","subj":"T20","obj":"http://purl.obolibrary.org/obo/MONDO_0005091"},{"id":"A21","pred":"mondo_id","subj":"T21","obj":"http://purl.obolibrary.org/obo/MONDO_0005091"},{"id":"A22","pred":"mondo_id","subj":"T22","obj":"http://purl.obolibrary.org/obo/MONDO_0005091"},{"id":"A23","pred":"mondo_id","subj":"T23","obj":"http://purl.obolibrary.org/obo/MONDO_0005091"},{"id":"A24","pred":"mondo_id","subj":"T24","obj":"http://purl.obolibrary.org/obo/MONDO_0005091"},{"id":"A25","pred":"mondo_id","subj":"T25","obj":"http://purl.obolibrary.org/obo/MONDO_0005091"},{"id":"A26","pred":"mondo_id","subj":"T26","obj":"http://purl.obolibrary.org/obo/MONDO_0005091"},{"id":"A27","pred":"mondo_id","subj":"T27","obj":"http://purl.obolibrary.org/obo/MONDO_0005091"},{"id":"A28","pred":"mondo_id","subj":"T28","obj":"http://purl.obolibrary.org/obo/MONDO_0005108"},{"id":"A29","pred":"mondo_id","subj":"T29","obj":"http://purl.obolibrary.org/obo/MONDO_0005108"},{"id":"A30","pred":"mondo_id","subj":"T30","obj":"http://purl.obolibrary.org/obo/MONDO_0021094"},{"id":"A31","pred":"mondo_id","subj":"T31","obj":"http://purl.obolibrary.org/obo/MONDO_0005091"},{"id":"A32","pred":"mondo_id","subj":"T32","obj":"http://purl.obolibrary.org/obo/MONDO_0005091"},{"id":"A33","pred":"mondo_id","subj":"T33","obj":"http://purl.obolibrary.org/obo/MONDO_0005091"}],"text":"1. Introduction\nSince the start of the COVID-19 pandemic in December 2019, researchers around the world have put major efforts towards a better understanding of the immune response to its causative agent, the SARS-CoV-2. Although an impressive amount of scientific information has been generated in a very short period of time, there remain significant gaps in our understanding of SARS-CoV-2 immune control. In particular, it remains unclear what kind of adaptive immunity should be triggered by vaccination in order to achieve sterile immunity, or at least lead to an ameliorated disease course, in cases where vaccination cannot provide absolute protection from infection. We know from the available literature on other coronaviruses (mainly SARS-CoV-1 and MERS) that antibodies can neutralize the infection, although these humoral responses are short lived in many individuals, and that long-lived T cells responses are present in people with less severe disease outcomes [1,2,3,4,5]. The emerging data on the immune response to SARS-CoV-2 demonstrate the essential contribution of the virus-specific T-cell responses, possibly in addition to the action of neutralizing antibodies, in viral control [3,6,7,8,9,10,11,12,13]. Thus, improved tools to assess host T cell immunity in detail are urgently needed to better identify these responses and to define their role in the outcome of SARS-CoV-2 infection.\nEx-vivo immune analyses of samples from infected individuals can identify T cell responses to specific pathogens like viruses. Such analyses can help to better understand the role of host immunity in virus control and to guide successful vaccine development. However, they rely on the use of the correct recall antigens that can elicit specific responses in vitro. The urgency of the current SARS-CoV-2 pandemic has led researchers to tackle the problem of screening the 10,000 amino acids of the SARS-CoV-2 proteome for T cell responses by selecting viral sequences based on different criteria: (i) bioinformatically predicted epitopes, (ii) homology of SARS-CoV-2 sequences with epitopes defined in other coronaviruses (mainly SARS-CoV) or (iii) selecting some specific SARS-CoV-2 proteins over others [5,7,9,11,14,15,16,17,18,19]. However, all these approaches have intrinsic limitations. Bioinformatic prediction tools are trained on sets of previously described epitopes, but since the available epitope repertoire for many human leukocyte antigen (HLA) alleles is limited, its prediction capacity is also limited [20,21]. Inferences based on epitope sequence homology with other coronaviruses are hampered because past studies on SARS-CoV-1 and MERS only included few selected viral proteins. This is of concern, since screening only a part of the SARS-CoV-2 proteome will potentially miss an important portion of the virus-specific T cell response. Indeed, recent data indicate the existence of T cell responses against structural and non-structural proteins [5,9] for SARS-CoV-2 and other viral infections [22]. Finally, no study has considered the existence of T cell responses to epitopes encoded by open-reading frames (ORF) in alternative frames, as reported for other viral infections [23,24,25,26].\nIn order to reliably measure total virus-specific T cell immunity, the recall antigens used need to be as representative as possible of the worldwide viral sequences, even for genetically more stable viruses like coronaviruses. T cell recognition of epitopes is very sensitive to mismatches and not matching the recall antigen with the autologous virus can lead to missed responses [27]. For this reason, different test antigen design strategies, trying to cope with the diversity of circulating viral isolates in a single sequence, have been developed in the past. These strategies include central sequence designs such as Center of Tree (COT) [28,29,30,31,32], Ancestral [33,34,35,36] or Consensus sequences [29,30,31,32,35,37,38,39,40,41,42,43]; which may (Ancestral, COT) or may not (Consensus) represent naturally occurring sequences of replication competent viruses. All these designs are sensitive to the underlying sequence database and may change over time as new sequence information on additional isolates becomes available. Direct comparisons of these different central sequence approaches have been performed for a highly variable pathogen (human immunodeficiency virus, HIV) and shown that the different designs yielded comparable results when synthetic peptides covering these sequences were used to measure virus-specific T cell responses [42,43]. However, the additional costs in terms of peptide synthesis and cells needed for ex-vivo experiments, may not warrant inclusion of all the different variants into a single test set.\nThus, the characterization of the complete T cell responses to SARS-CoV-2 urgently needs T cell antigens that cover the whole SARS-CoV-2 proteome while covering sequence diversity, and which can be combined in different experimental set-ups and immune assays. To this end, we created a consensus sequence to cover the genetic diversity of SARS-CoV-2 (CoV-2-cons) for all ORF, including those described in alternative open reading frames. Given the computational ease for its initial generation and periodic updates, we designed a consensus sequence using more than 1700 CoV-2 full-genome sequences and designed overlapping peptide (OLP) sets as recall antigens in T cell assays. The CoV-2-cons OLP sets are presented here in different designs, balancing costs for synthesis with the sensitivity of detecting T cell responses and with the intention to provide a common test antigen that will allow data comparability across laboratories."}
LitCovid-PD-CLO
{"project":"LitCovid-PD-CLO","denotations":[{"id":"T13","span":{"begin":135,"end":136},"obj":"http://purl.obolibrary.org/obo/CLO_0001020"},{"id":"T14","span":{"begin":277,"end":280},"obj":"http://purl.obolibrary.org/obo/CLO_0051582"},{"id":"T15","span":{"begin":299,"end":300},"obj":"http://purl.obolibrary.org/obo/CLO_0001020"},{"id":"T16","span":{"begin":902,"end":909},"obj":"http://purl.obolibrary.org/obo/CL_0000084"},{"id":"T17","span":{"begin":1090,"end":1095},"obj":"http://purl.obolibrary.org/obo/NCBITaxon_10239"},{"id":"T18","span":{"begin":1105,"end":1111},"obj":"http://purl.obolibrary.org/obo/CL_0000084"},{"id":"T19","span":{"begin":1264,"end":1270},"obj":"http://purl.obolibrary.org/obo/CL_0000084"},{"id":"T20","span":{"begin":1484,"end":1490},"obj":"http://purl.obolibrary.org/obo/CL_0000084"},{"id":"T21","span":{"begin":1528,"end":1535},"obj":"http://purl.obolibrary.org/obo/NCBITaxon_10239"},{"id":"T22","span":{"begin":1610,"end":1615},"obj":"http://purl.obolibrary.org/obo/NCBITaxon_10239"},{"id":"T23","span":{"begin":1822,"end":1825},"obj":"http://purl.obolibrary.org/obo/CLO_0051582"},{"id":"T24","span":{"begin":1931,"end":1937},"obj":"http://purl.obolibrary.org/obo/CL_0000084"},{"id":"T25","span":{"begin":2439,"end":2444},"obj":"http://purl.obolibrary.org/obo/NCBITaxon_9606"},{"id":"T26","span":{"begin":2750,"end":2751},"obj":"http://purl.obolibrary.org/obo/CLO_0001020"},{"id":"T27","span":{"begin":2834,"end":2839},"obj":"http://purl.obolibrary.org/obo/NCBITaxon_10239"},{"id":"T28","span":{"begin":2849,"end":2855},"obj":"http://purl.obolibrary.org/obo/CL_0000084"},{"id":"T29","span":{"begin":2912,"end":2918},"obj":"http://purl.obolibrary.org/obo/CL_0000084"},{"id":"T30","span":{"begin":3025,"end":3027},"obj":"http://purl.obolibrary.org/obo/CLO_0050507"},{"id":"T31","span":{"begin":3048,"end":3051},"obj":"http://purl.obolibrary.org/obo/CLO_0051582"},{"id":"T32","span":{"begin":3080,"end":3086},"obj":"http://purl.obolibrary.org/obo/CL_0000084"},{"id":"T33","span":{"begin":3258,"end":3263},"obj":"http://purl.obolibrary.org/obo/NCBITaxon_10239"},{"id":"T34","span":{"begin":3273,"end":3279},"obj":"http://purl.obolibrary.org/obo/CL_0000084"},{"id":"T35","span":{"begin":3423,"end":3430},"obj":"http://purl.obolibrary.org/obo/NCBITaxon_10239"},{"id":"T36","span":{"begin":3451,"end":3457},"obj":"http://purl.obolibrary.org/obo/CL_0000084"},{"id":"T37","span":{"begin":3570,"end":3575},"obj":"http://purl.obolibrary.org/obo/NCBITaxon_10239"},{"id":"T38","span":{"begin":3606,"end":3608},"obj":"http://purl.obolibrary.org/obo/CLO_0050509"},{"id":"T39","span":{"begin":3638,"end":3642},"obj":"http://purl.obolibrary.org/obo/UBERON_0000473"},{"id":"T40","span":{"begin":3737,"end":3738},"obj":"http://purl.obolibrary.org/obo/CLO_0001020"},{"id":"T41","span":{"begin":4087,"end":4094},"obj":"http://purl.obolibrary.org/obo/NCBITaxon_10239"},{"id":"T42","span":{"begin":4349,"end":4350},"obj":"http://purl.obolibrary.org/obo/CLO_0001020"},{"id":"T43","span":{"begin":4377,"end":4382},"obj":"http://purl.obolibrary.org/obo/NCBITaxon_9606"},{"id":"T44","span":{"begin":4400,"end":4405},"obj":"http://purl.obolibrary.org/obo/NCBITaxon_10239"},{"id":"T45","span":{"begin":4491,"end":4499},"obj":"http://purl.obolibrary.org/obo/PR_000018263"},{"id":"T46","span":{"begin":4546,"end":4551},"obj":"http://purl.obolibrary.org/obo/NCBITaxon_10239"},{"id":"T47","span":{"begin":4561,"end":4567},"obj":"http://purl.obolibrary.org/obo/CL_0000084"},{"id":"T48","span":{"begin":4629,"end":4636},"obj":"http://purl.obolibrary.org/obo/PR_000018263"},{"id":"T49","span":{"begin":4651,"end":4656},"obj":"http://purl.obolibrary.org/obo/GO_0005623"},{"id":"T50","span":{"begin":4750,"end":4751},"obj":"http://purl.obolibrary.org/obo/CLO_0001020"},{"id":"T51","span":{"begin":4759,"end":4763},"obj":"http://purl.obolibrary.org/obo/UBERON_0000473"},{"id":"T52","span":{"begin":4812,"end":4818},"obj":"http://purl.obolibrary.org/obo/CL_0000084"},{"id":"T53","span":{"begin":4858,"end":4864},"obj":"http://purl.obolibrary.org/obo/CL_0000084"},{"id":"T54","span":{"begin":5053,"end":5054},"obj":"http://purl.obolibrary.org/obo/CLO_0001020"},{"id":"T55","span":{"begin":5297,"end":5298},"obj":"http://purl.obolibrary.org/obo/CLO_0001020"},{"id":"T56","span":{"begin":5392,"end":5399},"obj":"http://purl.obolibrary.org/obo/PR_000018263"},{"id":"T57","span":{"begin":5433,"end":5439},"obj":"http://purl.obolibrary.org/obo/CL_0000084"},{"id":"T58","span":{"begin":5577,"end":5583},"obj":"http://purl.obolibrary.org/obo/CL_0000084"},{"id":"T59","span":{"begin":5628,"end":5629},"obj":"http://purl.obolibrary.org/obo/CLO_0001020"},{"id":"T60","span":{"begin":5637,"end":5641},"obj":"http://purl.obolibrary.org/obo/UBERON_0000473"}],"text":"1. Introduction\nSince the start of the COVID-19 pandemic in December 2019, researchers around the world have put major efforts towards a better understanding of the immune response to its causative agent, the SARS-CoV-2. Although an impressive amount of scientific information has been generated in a very short period of time, there remain significant gaps in our understanding of SARS-CoV-2 immune control. In particular, it remains unclear what kind of adaptive immunity should be triggered by vaccination in order to achieve sterile immunity, or at least lead to an ameliorated disease course, in cases where vaccination cannot provide absolute protection from infection. We know from the available literature on other coronaviruses (mainly SARS-CoV-1 and MERS) that antibodies can neutralize the infection, although these humoral responses are short lived in many individuals, and that long-lived T cells responses are present in people with less severe disease outcomes [1,2,3,4,5]. The emerging data on the immune response to SARS-CoV-2 demonstrate the essential contribution of the virus-specific T-cell responses, possibly in addition to the action of neutralizing antibodies, in viral control [3,6,7,8,9,10,11,12,13]. Thus, improved tools to assess host T cell immunity in detail are urgently needed to better identify these responses and to define their role in the outcome of SARS-CoV-2 infection.\nEx-vivo immune analyses of samples from infected individuals can identify T cell responses to specific pathogens like viruses. Such analyses can help to better understand the role of host immunity in virus control and to guide successful vaccine development. However, they rely on the use of the correct recall antigens that can elicit specific responses in vitro. The urgency of the current SARS-CoV-2 pandemic has led researchers to tackle the problem of screening the 10,000 amino acids of the SARS-CoV-2 proteome for T cell responses by selecting viral sequences based on different criteria: (i) bioinformatically predicted epitopes, (ii) homology of SARS-CoV-2 sequences with epitopes defined in other coronaviruses (mainly SARS-CoV) or (iii) selecting some specific SARS-CoV-2 proteins over others [5,7,9,11,14,15,16,17,18,19]. However, all these approaches have intrinsic limitations. Bioinformatic prediction tools are trained on sets of previously described epitopes, but since the available epitope repertoire for many human leukocyte antigen (HLA) alleles is limited, its prediction capacity is also limited [20,21]. Inferences based on epitope sequence homology with other coronaviruses are hampered because past studies on SARS-CoV-1 and MERS only included few selected viral proteins. This is of concern, since screening only a part of the SARS-CoV-2 proteome will potentially miss an important portion of the virus-specific T cell response. Indeed, recent data indicate the existence of T cell responses against structural and non-structural proteins [5,9] for SARS-CoV-2 and other viral infections [22]. Finally, no study has considered the existence of T cell responses to epitopes encoded by open-reading frames (ORF) in alternative frames, as reported for other viral infections [23,24,25,26].\nIn order to reliably measure total virus-specific T cell immunity, the recall antigens used need to be as representative as possible of the worldwide viral sequences, even for genetically more stable viruses like coronaviruses. T cell recognition of epitopes is very sensitive to mismatches and not matching the recall antigen with the autologous virus can lead to missed responses [27]. For this reason, different test antigen design strategies, trying to cope with the diversity of circulating viral isolates in a single sequence, have been developed in the past. These strategies include central sequence designs such as Center of Tree (COT) [28,29,30,31,32], Ancestral [33,34,35,36] or Consensus sequences [29,30,31,32,35,37,38,39,40,41,42,43]; which may (Ancestral, COT) or may not (Consensus) represent naturally occurring sequences of replication competent viruses. All these designs are sensitive to the underlying sequence database and may change over time as new sequence information on additional isolates becomes available. Direct comparisons of these different central sequence approaches have been performed for a highly variable pathogen (human immunodeficiency virus, HIV) and shown that the different designs yielded comparable results when synthetic peptides covering these sequences were used to measure virus-specific T cell responses [42,43]. However, the additional costs in terms of peptide synthesis and cells needed for ex-vivo experiments, may not warrant inclusion of all the different variants into a single test set.\nThus, the characterization of the complete T cell responses to SARS-CoV-2 urgently needs T cell antigens that cover the whole SARS-CoV-2 proteome while covering sequence diversity, and which can be combined in different experimental set-ups and immune assays. To this end, we created a consensus sequence to cover the genetic diversity of SARS-CoV-2 (CoV-2-cons) for all ORF, including those described in alternative open reading frames. Given the computational ease for its initial generation and periodic updates, we designed a consensus sequence using more than 1700 CoV-2 full-genome sequences and designed overlapping peptide (OLP) sets as recall antigens in T cell assays. The CoV-2-cons OLP sets are presented here in different designs, balancing costs for synthesis with the sensitivity of detecting T cell responses and with the intention to provide a common test antigen that will allow data comparability across laboratories."}
LitCovid-PubTator
{"project":"LitCovid-PubTator","denotations":[{"id":"37","span":{"begin":209,"end":219},"obj":"Species"},{"id":"38","span":{"begin":382,"end":392},"obj":"Species"},{"id":"39","span":{"begin":723,"end":736},"obj":"Species"},{"id":"40","span":{"begin":745,"end":753},"obj":"Species"},{"id":"41","span":{"begin":935,"end":941},"obj":"Species"},{"id":"42","span":{"begin":1033,"end":1043},"obj":"Species"},{"id":"43","span":{"begin":39,"end":47},"obj":"Disease"},{"id":"44","span":{"begin":665,"end":674},"obj":"Disease"},{"id":"45","span":{"begin":760,"end":764},"obj":"Disease"},{"id":"46","span":{"begin":801,"end":810},"obj":"Disease"},{"id":"47","span":{"begin":1388,"end":1408},"obj":"Disease"},{"id":"63","span":{"begin":1802,"end":1812},"obj":"Species"},{"id":"64","span":{"begin":1907,"end":1917},"obj":"Species"},{"id":"65","span":{"begin":2065,"end":2075},"obj":"Species"},{"id":"66","span":{"begin":2117,"end":2130},"obj":"Species"},{"id":"67","span":{"begin":2139,"end":2147},"obj":"Species"},{"id":"68","span":{"begin":2182,"end":2192},"obj":"Species"},{"id":"69","span":{"begin":2595,"end":2608},"obj":"Species"},{"id":"70","span":{"begin":2646,"end":2654},"obj":"Species"},{"id":"71","span":{"begin":2764,"end":2774},"obj":"Species"},{"id":"72","span":{"begin":2986,"end":2996},"obj":"Species"},{"id":"73","span":{"begin":2439,"end":2444},"obj":"Species"},{"id":"74","span":{"begin":1450,"end":1458},"obj":"Disease"},{"id":"75","span":{"begin":2661,"end":2665},"obj":"Disease"},{"id":"76","span":{"begin":3007,"end":3023},"obj":"Disease"},{"id":"77","span":{"begin":3191,"end":3207},"obj":"Disease"},{"id":"81","span":{"begin":3436,"end":3449},"obj":"Species"},{"id":"82","span":{"begin":4065,"end":4094},"obj":"Species"},{"id":"83","span":{"begin":4377,"end":4405},"obj":"Species"},{"id":"90","span":{"begin":4832,"end":4842},"obj":"Species"},{"id":"91","span":{"begin":4895,"end":4905},"obj":"Species"},{"id":"92","span":{"begin":5108,"end":5118},"obj":"Species"},{"id":"93","span":{"begin":5120,"end":5125},"obj":"Species"},{"id":"94","span":{"begin":5339,"end":5344},"obj":"Species"},{"id":"95","span":{"begin":5452,"end":5457},"obj":"Species"}],"attributes":[{"id":"A37","pred":"tao:has_database_id","subj":"37","obj":"Tax:2697049"},{"id":"A38","pred":"tao:has_database_id","subj":"38","obj":"Tax:2697049"},{"id":"A39","pred":"tao:has_database_id","subj":"39","obj":"Tax:11118"},{"id":"A40","pred":"tao:has_database_id","subj":"40","obj":"Tax:694009"},{"id":"A41","pred":"tao:has_database_id","subj":"41","obj":"Tax:9606"},{"id":"A42","pred":"tao:has_database_id","subj":"42","obj":"Tax:2697049"},{"id":"A43","pred":"tao:has_database_id","subj":"43","obj":"MESH:C000657245"},{"id":"A44","pred":"tao:has_database_id","subj":"44","obj":"MESH:D007239"},{"id":"A45","pred":"tao:has_database_id","subj":"45","obj":"MESH:D018352"},{"id":"A46","pred":"tao:has_database_id","subj":"46","obj":"MESH:D007239"},{"id":"A47","pred":"tao:has_database_id","subj":"47","obj":"MESH:C000657245"},{"id":"A63","pred":"tao:has_database_id","subj":"63","obj":"Tax:2697049"},{"id":"A64","pred":"tao:has_database_id","subj":"64","obj":"Tax:2697049"},{"id":"A65","pred":"tao:has_database_id","subj":"65","obj":"Tax:2697049"},{"id":"A66","pred":"tao:has_database_id","subj":"66","obj":"Tax:11118"},{"id":"A67","pred":"tao:has_database_id","subj":"67","obj":"Tax:694009"},{"id":"A68","pred":"tao:has_database_id","subj":"68","obj":"Tax:2697049"},{"id":"A69","pred":"tao:has_database_id","subj":"69","obj":"Tax:11118"},{"id":"A70","pred":"tao:has_database_id","subj":"70","obj":"Tax:694009"},{"id":"A71","pred":"tao:has_database_id","subj":"71","obj":"Tax:2697049"},{"id":"A72","pred":"tao:has_database_id","subj":"72","obj":"Tax:2697049"},{"id":"A73","pred":"tao:has_database_id","subj":"73","obj":"Tax:9606"},{"id":"A74","pred":"tao:has_database_id","subj":"74","obj":"MESH:D007239"},{"id":"A75","pred":"tao:has_database_id","subj":"75","obj":"MESH:D018352"},{"id":"A76","pred":"tao:has_database_id","subj":"76","obj":"MESH:D001102"},{"id":"A77","pred":"tao:has_database_id","subj":"77","obj":"MESH:D001102"},{"id":"A81","pred":"tao:has_database_id","subj":"81","obj":"Tax:11118"},{"id":"A82","pred":"tao:has_database_id","subj":"82","obj":"Tax:353210"},{"id":"A83","pred":"tao:has_database_id","subj":"83","obj":"Tax:12721"},{"id":"A90","pred":"tao:has_database_id","subj":"90","obj":"Tax:2697049"},{"id":"A91","pred":"tao:has_database_id","subj":"91","obj":"Tax:2697049"},{"id":"A92","pred":"tao:has_database_id","subj":"92","obj":"Tax:2697049"},{"id":"A93","pred":"tao:has_database_id","subj":"93","obj":"Tax:2697049"},{"id":"A94","pred":"tao:has_database_id","subj":"94","obj":"Tax:2697049"},{"id":"A95","pred":"tao:has_database_id","subj":"95","obj":"Tax:2697049"}],"namespaces":[{"prefix":"Tax","uri":"https://www.ncbi.nlm.nih.gov/taxonomy/"},{"prefix":"MESH","uri":"https://id.nlm.nih.gov/mesh/"},{"prefix":"Gene","uri":"https://www.ncbi.nlm.nih.gov/gene/"},{"prefix":"CVCL","uri":"https://web.expasy.org/cellosaurus/CVCL_"}],"text":"1. Introduction\nSince the start of the COVID-19 pandemic in December 2019, researchers around the world have put major efforts towards a better understanding of the immune response to its causative agent, the SARS-CoV-2. Although an impressive amount of scientific information has been generated in a very short period of time, there remain significant gaps in our understanding of SARS-CoV-2 immune control. In particular, it remains unclear what kind of adaptive immunity should be triggered by vaccination in order to achieve sterile immunity, or at least lead to an ameliorated disease course, in cases where vaccination cannot provide absolute protection from infection. We know from the available literature on other coronaviruses (mainly SARS-CoV-1 and MERS) that antibodies can neutralize the infection, although these humoral responses are short lived in many individuals, and that long-lived T cells responses are present in people with less severe disease outcomes [1,2,3,4,5]. The emerging data on the immune response to SARS-CoV-2 demonstrate the essential contribution of the virus-specific T-cell responses, possibly in addition to the action of neutralizing antibodies, in viral control [3,6,7,8,9,10,11,12,13]. Thus, improved tools to assess host T cell immunity in detail are urgently needed to better identify these responses and to define their role in the outcome of SARS-CoV-2 infection.\nEx-vivo immune analyses of samples from infected individuals can identify T cell responses to specific pathogens like viruses. Such analyses can help to better understand the role of host immunity in virus control and to guide successful vaccine development. However, they rely on the use of the correct recall antigens that can elicit specific responses in vitro. The urgency of the current SARS-CoV-2 pandemic has led researchers to tackle the problem of screening the 10,000 amino acids of the SARS-CoV-2 proteome for T cell responses by selecting viral sequences based on different criteria: (i) bioinformatically predicted epitopes, (ii) homology of SARS-CoV-2 sequences with epitopes defined in other coronaviruses (mainly SARS-CoV) or (iii) selecting some specific SARS-CoV-2 proteins over others [5,7,9,11,14,15,16,17,18,19]. However, all these approaches have intrinsic limitations. Bioinformatic prediction tools are trained on sets of previously described epitopes, but since the available epitope repertoire for many human leukocyte antigen (HLA) alleles is limited, its prediction capacity is also limited [20,21]. Inferences based on epitope sequence homology with other coronaviruses are hampered because past studies on SARS-CoV-1 and MERS only included few selected viral proteins. This is of concern, since screening only a part of the SARS-CoV-2 proteome will potentially miss an important portion of the virus-specific T cell response. Indeed, recent data indicate the existence of T cell responses against structural and non-structural proteins [5,9] for SARS-CoV-2 and other viral infections [22]. Finally, no study has considered the existence of T cell responses to epitopes encoded by open-reading frames (ORF) in alternative frames, as reported for other viral infections [23,24,25,26].\nIn order to reliably measure total virus-specific T cell immunity, the recall antigens used need to be as representative as possible of the worldwide viral sequences, even for genetically more stable viruses like coronaviruses. T cell recognition of epitopes is very sensitive to mismatches and not matching the recall antigen with the autologous virus can lead to missed responses [27]. For this reason, different test antigen design strategies, trying to cope with the diversity of circulating viral isolates in a single sequence, have been developed in the past. These strategies include central sequence designs such as Center of Tree (COT) [28,29,30,31,32], Ancestral [33,34,35,36] or Consensus sequences [29,30,31,32,35,37,38,39,40,41,42,43]; which may (Ancestral, COT) or may not (Consensus) represent naturally occurring sequences of replication competent viruses. All these designs are sensitive to the underlying sequence database and may change over time as new sequence information on additional isolates becomes available. Direct comparisons of these different central sequence approaches have been performed for a highly variable pathogen (human immunodeficiency virus, HIV) and shown that the different designs yielded comparable results when synthetic peptides covering these sequences were used to measure virus-specific T cell responses [42,43]. However, the additional costs in terms of peptide synthesis and cells needed for ex-vivo experiments, may not warrant inclusion of all the different variants into a single test set.\nThus, the characterization of the complete T cell responses to SARS-CoV-2 urgently needs T cell antigens that cover the whole SARS-CoV-2 proteome while covering sequence diversity, and which can be combined in different experimental set-ups and immune assays. To this end, we created a consensus sequence to cover the genetic diversity of SARS-CoV-2 (CoV-2-cons) for all ORF, including those described in alternative open reading frames. Given the computational ease for its initial generation and periodic updates, we designed a consensus sequence using more than 1700 CoV-2 full-genome sequences and designed overlapping peptide (OLP) sets as recall antigens in T cell assays. The CoV-2-cons OLP sets are presented here in different designs, balancing costs for synthesis with the sensitivity of detecting T cell responses and with the intention to provide a common test antigen that will allow data comparability across laboratories."}
LitCovid-PD-HP
{"project":"LitCovid-PD-HP","denotations":[{"id":null,"span":{"begin":4383,"end":4399},"obj":"Phenotype"}],"text":"1. Introduction\nSince the start of the COVID-19 pandemic in December 2019, researchers around the world have put major efforts towards a better understanding of the immune response to its causative agent, the SARS-CoV-2. Although an impressive amount of scientific information has been generated in a very short period of time, there remain significant gaps in our understanding of SARS-CoV-2 immune control. In particular, it remains unclear what kind of adaptive immunity should be triggered by vaccination in order to achieve sterile immunity, or at least lead to an ameliorated disease course, in cases where vaccination cannot provide absolute protection from infection. We know from the available literature on other coronaviruses (mainly SARS-CoV-1 and MERS) that antibodies can neutralize the infection, although these humoral responses are short lived in many individuals, and that long-lived T cells responses are present in people with less severe disease outcomes [1,2,3,4,5]. The emerging data on the immune response to SARS-CoV-2 demonstrate the essential contribution of the virus-specific T-cell responses, possibly in addition to the action of neutralizing antibodies, in viral control [3,6,7,8,9,10,11,12,13]. Thus, improved tools to assess host T cell immunity in detail are urgently needed to better identify these responses and to define their role in the outcome of SARS-CoV-2 infection.\nEx-vivo immune analyses of samples from infected individuals can identify T cell responses to specific pathogens like viruses. Such analyses can help to better understand the role of host immunity in virus control and to guide successful vaccine development. However, they rely on the use of the correct recall antigens that can elicit specific responses in vitro. The urgency of the current SARS-CoV-2 pandemic has led researchers to tackle the problem of screening the 10,000 amino acids of the SARS-CoV-2 proteome for T cell responses by selecting viral sequences based on different criteria: (i) bioinformatically predicted epitopes, (ii) homology of SARS-CoV-2 sequences with epitopes defined in other coronaviruses (mainly SARS-CoV) or (iii) selecting some specific SARS-CoV-2 proteins over others [5,7,9,11,14,15,16,17,18,19]. However, all these approaches have intrinsic limitations. Bioinformatic prediction tools are trained on sets of previously described epitopes, but since the available epitope repertoire for many human leukocyte antigen (HLA) alleles is limited, its prediction capacity is also limited [20,21]. Inferences based on epitope sequence homology with other coronaviruses are hampered because past studies on SARS-CoV-1 and MERS only included few selected viral proteins. This is of concern, since screening only a part of the SARS-CoV-2 proteome will potentially miss an important portion of the virus-specific T cell response. Indeed, recent data indicate the existence of T cell responses against structural and non-structural proteins [5,9] for SARS-CoV-2 and other viral infections [22]. Finally, no study has considered the existence of T cell responses to epitopes encoded by open-reading frames (ORF) in alternative frames, as reported for other viral infections [23,24,25,26].\nIn order to reliably measure total virus-specific T cell immunity, the recall antigens used need to be as representative as possible of the worldwide viral sequences, even for genetically more stable viruses like coronaviruses. T cell recognition of epitopes is very sensitive to mismatches and not matching the recall antigen with the autologous virus can lead to missed responses [27]. For this reason, different test antigen design strategies, trying to cope with the diversity of circulating viral isolates in a single sequence, have been developed in the past. These strategies include central sequence designs such as Center of Tree (COT) [28,29,30,31,32], Ancestral [33,34,35,36] or Consensus sequences [29,30,31,32,35,37,38,39,40,41,42,43]; which may (Ancestral, COT) or may not (Consensus) represent naturally occurring sequences of replication competent viruses. All these designs are sensitive to the underlying sequence database and may change over time as new sequence information on additional isolates becomes available. Direct comparisons of these different central sequence approaches have been performed for a highly variable pathogen (human immunodeficiency virus, HIV) and shown that the different designs yielded comparable results when synthetic peptides covering these sequences were used to measure virus-specific T cell responses [42,43]. However, the additional costs in terms of peptide synthesis and cells needed for ex-vivo experiments, may not warrant inclusion of all the different variants into a single test set.\nThus, the characterization of the complete T cell responses to SARS-CoV-2 urgently needs T cell antigens that cover the whole SARS-CoV-2 proteome while covering sequence diversity, and which can be combined in different experimental set-ups and immune assays. To this end, we created a consensus sequence to cover the genetic diversity of SARS-CoV-2 (CoV-2-cons) for all ORF, including those described in alternative open reading frames. Given the computational ease for its initial generation and periodic updates, we designed a consensus sequence using more than 1700 CoV-2 full-genome sequences and designed overlapping peptide (OLP) sets as recall antigens in T cell assays. The CoV-2-cons OLP sets are presented here in different designs, balancing costs for synthesis with the sensitivity of detecting T cell responses and with the intention to provide a common test antigen that will allow data comparability across laboratories."}
LitCovid-PD-GO-BP
{"project":"LitCovid-PD-GO-BP","denotations":[{"id":"T3","span":{"begin":165,"end":180},"obj":"http://purl.obolibrary.org/obo/GO_0006955"},{"id":"T4","span":{"begin":1014,"end":1029},"obj":"http://purl.obolibrary.org/obo/GO_0006955"},{"id":"T5","span":{"begin":3007,"end":3023},"obj":"http://purl.obolibrary.org/obo/GO_0016032"},{"id":"T6","span":{"begin":3191,"end":3207},"obj":"http://purl.obolibrary.org/obo/GO_0016032"},{"id":"T7","span":{"begin":3453,"end":3469},"obj":"http://purl.obolibrary.org/obo/GO_0008037"},{"id":"T8","span":{"begin":4629,"end":4646},"obj":"http://purl.obolibrary.org/obo/GO_0043043"},{"id":"T9","span":{"begin":4637,"end":4646},"obj":"http://purl.obolibrary.org/obo/GO_0009058"},{"id":"T10","span":{"begin":5533,"end":5542},"obj":"http://purl.obolibrary.org/obo/GO_0009058"}],"text":"1. Introduction\nSince the start of the COVID-19 pandemic in December 2019, researchers around the world have put major efforts towards a better understanding of the immune response to its causative agent, the SARS-CoV-2. Although an impressive amount of scientific information has been generated in a very short period of time, there remain significant gaps in our understanding of SARS-CoV-2 immune control. In particular, it remains unclear what kind of adaptive immunity should be triggered by vaccination in order to achieve sterile immunity, or at least lead to an ameliorated disease course, in cases where vaccination cannot provide absolute protection from infection. We know from the available literature on other coronaviruses (mainly SARS-CoV-1 and MERS) that antibodies can neutralize the infection, although these humoral responses are short lived in many individuals, and that long-lived T cells responses are present in people with less severe disease outcomes [1,2,3,4,5]. The emerging data on the immune response to SARS-CoV-2 demonstrate the essential contribution of the virus-specific T-cell responses, possibly in addition to the action of neutralizing antibodies, in viral control [3,6,7,8,9,10,11,12,13]. Thus, improved tools to assess host T cell immunity in detail are urgently needed to better identify these responses and to define their role in the outcome of SARS-CoV-2 infection.\nEx-vivo immune analyses of samples from infected individuals can identify T cell responses to specific pathogens like viruses. Such analyses can help to better understand the role of host immunity in virus control and to guide successful vaccine development. However, they rely on the use of the correct recall antigens that can elicit specific responses in vitro. The urgency of the current SARS-CoV-2 pandemic has led researchers to tackle the problem of screening the 10,000 amino acids of the SARS-CoV-2 proteome for T cell responses by selecting viral sequences based on different criteria: (i) bioinformatically predicted epitopes, (ii) homology of SARS-CoV-2 sequences with epitopes defined in other coronaviruses (mainly SARS-CoV) or (iii) selecting some specific SARS-CoV-2 proteins over others [5,7,9,11,14,15,16,17,18,19]. However, all these approaches have intrinsic limitations. Bioinformatic prediction tools are trained on sets of previously described epitopes, but since the available epitope repertoire for many human leukocyte antigen (HLA) alleles is limited, its prediction capacity is also limited [20,21]. Inferences based on epitope sequence homology with other coronaviruses are hampered because past studies on SARS-CoV-1 and MERS only included few selected viral proteins. This is of concern, since screening only a part of the SARS-CoV-2 proteome will potentially miss an important portion of the virus-specific T cell response. Indeed, recent data indicate the existence of T cell responses against structural and non-structural proteins [5,9] for SARS-CoV-2 and other viral infections [22]. Finally, no study has considered the existence of T cell responses to epitopes encoded by open-reading frames (ORF) in alternative frames, as reported for other viral infections [23,24,25,26].\nIn order to reliably measure total virus-specific T cell immunity, the recall antigens used need to be as representative as possible of the worldwide viral sequences, even for genetically more stable viruses like coronaviruses. T cell recognition of epitopes is very sensitive to mismatches and not matching the recall antigen with the autologous virus can lead to missed responses [27]. For this reason, different test antigen design strategies, trying to cope with the diversity of circulating viral isolates in a single sequence, have been developed in the past. These strategies include central sequence designs such as Center of Tree (COT) [28,29,30,31,32], Ancestral [33,34,35,36] or Consensus sequences [29,30,31,32,35,37,38,39,40,41,42,43]; which may (Ancestral, COT) or may not (Consensus) represent naturally occurring sequences of replication competent viruses. All these designs are sensitive to the underlying sequence database and may change over time as new sequence information on additional isolates becomes available. Direct comparisons of these different central sequence approaches have been performed for a highly variable pathogen (human immunodeficiency virus, HIV) and shown that the different designs yielded comparable results when synthetic peptides covering these sequences were used to measure virus-specific T cell responses [42,43]. However, the additional costs in terms of peptide synthesis and cells needed for ex-vivo experiments, may not warrant inclusion of all the different variants into a single test set.\nThus, the characterization of the complete T cell responses to SARS-CoV-2 urgently needs T cell antigens that cover the whole SARS-CoV-2 proteome while covering sequence diversity, and which can be combined in different experimental set-ups and immune assays. To this end, we created a consensus sequence to cover the genetic diversity of SARS-CoV-2 (CoV-2-cons) for all ORF, including those described in alternative open reading frames. Given the computational ease for its initial generation and periodic updates, we designed a consensus sequence using more than 1700 CoV-2 full-genome sequences and designed overlapping peptide (OLP) sets as recall antigens in T cell assays. The CoV-2-cons OLP sets are presented here in different designs, balancing costs for synthesis with the sensitivity of detecting T cell responses and with the intention to provide a common test antigen that will allow data comparability across laboratories."}
LitCovid-sentences
{"project":"LitCovid-sentences","denotations":[{"id":"T10","span":{"begin":0,"end":2},"obj":"Sentence"},{"id":"T11","span":{"begin":3,"end":15},"obj":"Sentence"},{"id":"T12","span":{"begin":16,"end":220},"obj":"Sentence"},{"id":"T13","span":{"begin":221,"end":408},"obj":"Sentence"},{"id":"T14","span":{"begin":409,"end":675},"obj":"Sentence"},{"id":"T15","span":{"begin":676,"end":988},"obj":"Sentence"},{"id":"T16","span":{"begin":989,"end":1227},"obj":"Sentence"},{"id":"T17","span":{"begin":1228,"end":1409},"obj":"Sentence"},{"id":"T18","span":{"begin":1410,"end":1536},"obj":"Sentence"},{"id":"T19","span":{"begin":1537,"end":1668},"obj":"Sentence"},{"id":"T20","span":{"begin":1669,"end":1774},"obj":"Sentence"},{"id":"T21","span":{"begin":1775,"end":2243},"obj":"Sentence"},{"id":"T22","span":{"begin":2244,"end":2301},"obj":"Sentence"},{"id":"T23","span":{"begin":2302,"end":2537},"obj":"Sentence"},{"id":"T24","span":{"begin":2538,"end":2708},"obj":"Sentence"},{"id":"T25","span":{"begin":2709,"end":2865},"obj":"Sentence"},{"id":"T26","span":{"begin":2866,"end":3029},"obj":"Sentence"},{"id":"T27","span":{"begin":3030,"end":3222},"obj":"Sentence"},{"id":"T28","span":{"begin":3223,"end":3450},"obj":"Sentence"},{"id":"T29","span":{"begin":3451,"end":3610},"obj":"Sentence"},{"id":"T30","span":{"begin":3611,"end":3788},"obj":"Sentence"},{"id":"T31","span":{"begin":3789,"end":4095},"obj":"Sentence"},{"id":"T32","span":{"begin":4096,"end":4258},"obj":"Sentence"},{"id":"T33","span":{"begin":4259,"end":4586},"obj":"Sentence"},{"id":"T34","span":{"begin":4587,"end":4768},"obj":"Sentence"},{"id":"T35","span":{"begin":4769,"end":5028},"obj":"Sentence"},{"id":"T36","span":{"begin":5029,"end":5206},"obj":"Sentence"},{"id":"T37","span":{"begin":5207,"end":5447},"obj":"Sentence"},{"id":"T38","span":{"begin":5448,"end":5705},"obj":"Sentence"}],"namespaces":[{"prefix":"_base","uri":"http://pubannotation.org/ontology/tao.owl#"}],"text":"1. Introduction\nSince the start of the COVID-19 pandemic in December 2019, researchers around the world have put major efforts towards a better understanding of the immune response to its causative agent, the SARS-CoV-2. Although an impressive amount of scientific information has been generated in a very short period of time, there remain significant gaps in our understanding of SARS-CoV-2 immune control. In particular, it remains unclear what kind of adaptive immunity should be triggered by vaccination in order to achieve sterile immunity, or at least lead to an ameliorated disease course, in cases where vaccination cannot provide absolute protection from infection. We know from the available literature on other coronaviruses (mainly SARS-CoV-1 and MERS) that antibodies can neutralize the infection, although these humoral responses are short lived in many individuals, and that long-lived T cells responses are present in people with less severe disease outcomes [1,2,3,4,5]. The emerging data on the immune response to SARS-CoV-2 demonstrate the essential contribution of the virus-specific T-cell responses, possibly in addition to the action of neutralizing antibodies, in viral control [3,6,7,8,9,10,11,12,13]. Thus, improved tools to assess host T cell immunity in detail are urgently needed to better identify these responses and to define their role in the outcome of SARS-CoV-2 infection.\nEx-vivo immune analyses of samples from infected individuals can identify T cell responses to specific pathogens like viruses. Such analyses can help to better understand the role of host immunity in virus control and to guide successful vaccine development. However, they rely on the use of the correct recall antigens that can elicit specific responses in vitro. The urgency of the current SARS-CoV-2 pandemic has led researchers to tackle the problem of screening the 10,000 amino acids of the SARS-CoV-2 proteome for T cell responses by selecting viral sequences based on different criteria: (i) bioinformatically predicted epitopes, (ii) homology of SARS-CoV-2 sequences with epitopes defined in other coronaviruses (mainly SARS-CoV) or (iii) selecting some specific SARS-CoV-2 proteins over others [5,7,9,11,14,15,16,17,18,19]. However, all these approaches have intrinsic limitations. Bioinformatic prediction tools are trained on sets of previously described epitopes, but since the available epitope repertoire for many human leukocyte antigen (HLA) alleles is limited, its prediction capacity is also limited [20,21]. Inferences based on epitope sequence homology with other coronaviruses are hampered because past studies on SARS-CoV-1 and MERS only included few selected viral proteins. This is of concern, since screening only a part of the SARS-CoV-2 proteome will potentially miss an important portion of the virus-specific T cell response. Indeed, recent data indicate the existence of T cell responses against structural and non-structural proteins [5,9] for SARS-CoV-2 and other viral infections [22]. Finally, no study has considered the existence of T cell responses to epitopes encoded by open-reading frames (ORF) in alternative frames, as reported for other viral infections [23,24,25,26].\nIn order to reliably measure total virus-specific T cell immunity, the recall antigens used need to be as representative as possible of the worldwide viral sequences, even for genetically more stable viruses like coronaviruses. T cell recognition of epitopes is very sensitive to mismatches and not matching the recall antigen with the autologous virus can lead to missed responses [27]. For this reason, different test antigen design strategies, trying to cope with the diversity of circulating viral isolates in a single sequence, have been developed in the past. These strategies include central sequence designs such as Center of Tree (COT) [28,29,30,31,32], Ancestral [33,34,35,36] or Consensus sequences [29,30,31,32,35,37,38,39,40,41,42,43]; which may (Ancestral, COT) or may not (Consensus) represent naturally occurring sequences of replication competent viruses. All these designs are sensitive to the underlying sequence database and may change over time as new sequence information on additional isolates becomes available. Direct comparisons of these different central sequence approaches have been performed for a highly variable pathogen (human immunodeficiency virus, HIV) and shown that the different designs yielded comparable results when synthetic peptides covering these sequences were used to measure virus-specific T cell responses [42,43]. However, the additional costs in terms of peptide synthesis and cells needed for ex-vivo experiments, may not warrant inclusion of all the different variants into a single test set.\nThus, the characterization of the complete T cell responses to SARS-CoV-2 urgently needs T cell antigens that cover the whole SARS-CoV-2 proteome while covering sequence diversity, and which can be combined in different experimental set-ups and immune assays. To this end, we created a consensus sequence to cover the genetic diversity of SARS-CoV-2 (CoV-2-cons) for all ORF, including those described in alternative open reading frames. Given the computational ease for its initial generation and periodic updates, we designed a consensus sequence using more than 1700 CoV-2 full-genome sequences and designed overlapping peptide (OLP) sets as recall antigens in T cell assays. The CoV-2-cons OLP sets are presented here in different designs, balancing costs for synthesis with the sensitivity of detecting T cell responses and with the intention to provide a common test antigen that will allow data comparability across laboratories."}