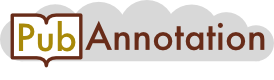
PMC:7556614 / 32913-43645
Annnotations
2_test
{"project":"2_test","denotations":[{"id":"33057878-28717504-28018","span":{"begin":593,"end":595},"obj":"28717504"},{"id":"33057878-30951793-28019","span":{"begin":2154,"end":2156},"obj":"30951793"},{"id":"33057878-24350328-28020","span":{"begin":4789,"end":4791},"obj":"24350328"},{"id":"33057878-24350328-28021","span":{"begin":5744,"end":5746},"obj":"24350328"}],"text":"Characterisation of Optimised Formulation\n\nScanning Electron Microscopy\nThe SEM images revealed a round and spherical shape morphology with a smooth surface of the nanoparticles (Fig. 4a, b) (34).\n\nHigh-Resolution Transmission Electron Microscopy\nFigure 4 c shows the range of particle size from 172 to 198 nm. The HRTEM measurement was in the agreement with dynamic light scattering (DLS) measurement of PALN1 particle size of 187.7 ± 3.75 nm. Also, the figure shows a two-layer structure of nanoparticles, which corresponds to the formation of chitosan shell layer around the lecithin core (35).\n\nDifferential Scanning Calorimetry\nFigure 5 a lecithin showed a low-intensity endothermic peak at 47.2°C, which was the chain melting transition point where it transformed into a liquid crystalline form from a gel form with enhanced ability to act as a drug carrier (36). Two endotherms were observed for chitosan, where 83.8°C was attributed to water loss and polymeric chain decomposition at 303.7°C (37). PEG 2000 endothermic peaks at 53.5°C, indicating melting process of the sample (36).\nFig. 5 Characterisation of PALN nanoparticles. a DSC thermogram of PALNs, physical mixture, ACV, lecithin, PEG 2000, chitosan; b XRD Diffractogram for PALNs\nACV had four thermal effects shown in Fig. 5 a. The analysis for ACV used was ACV polymorph form VI, with 1:2 ratio of ACV to water. The first endothermic peak at 116.5°C was assigned to the dehydration process. It leads to recrystallisation of ACV form VI to ACV form V, which undergoes a phase transition to IV at 174.6°C. Further heating leads to endotherm at 255.7°C, indicating ACV melting point (MP). Last exothermic peak at 259.1°C was assigned to the exothermic decomposition of the ACV (38).\nThe physical mixture for the first endotherm was assigned to the lecithin and PEG2000. ACV endothermic peak diminished but a new peak emerged at 151°C. It might be due to molecular interaction between drug, lecithin and chitosan. The interaction causes ACV to have decreased crystallinity and increased amorphous properties of acyclovir, or turned into semi-solid form, or dissolution in the matrix melt (39). For PALN1, peak diminishments of lecithin, chitosan, and PEG 2000 were observed. An endotherm observed at 242.3°C was assigned to the left shift of the ACV MP. Although the left shift was evidence for crystallinity changes, the amplitude of shifting was abnormal. Table III showed that heating rate for acyclovir and PM was higher than PALN1. Higher heating rate results in MP shifted to a higher temperature which is in agreement from the previous report of acyclovir MP (40).\n\nX-Ray Diffraction\nFigure 5 b showed the ACV peaks at 2θ of 7.12°, 10.66°, 13.22°, 16.19°, 23.56°, 24.10°, 26.33°, and 29.44°, indicating crystalline nature of the drug. Crystallinity index was calculated based on (12) with the aids of OriginPro Graphing \u0026 Analysis 2020 software. The ACV crystalline and total peak area were found to be 53,181.75 and 56,443.52 unit area, respectively, with crystallinity index calculated to be 0.9422, which was very crystalline12 CrystallinityIndex=AreaCrystalline/AreaTotalwhere AreaCrystalline and AreaTotal were integrated areas for crystalline structure and total integrated area, respectively.\nACV peak was diminished from PM diffractogram. This suggested possibility of ACV turned into the disordered crystalline state due to the molecular interaction with lecithin and chitosan. By comparing ACV and PALN1 diffractogram, a broad peak emerged with sharp peak diminishment indicating ACV entrapped in LPNPs system in the amorphous state of the nanoparticles (41).\n\nAttenuated Total Reflection-Fourier Transform Infrared\nFigure 6 showed PEG 2000 characteristic peaks at 3409 cm−1 indicating intermolecular hydrogen-bonded hydroxyl group (O–H) asymmetric stretching. Peaks at 2945 cm−1, 2884 cm−1, and 2860 cm−1 are assigned to C–H symmetrical stretching. C–H bending is observed at 2740 cm−1 (42). Peaks at 1240 cm−1, 1146 cm−1, 1099 cm−1, and 1059 cm−1 are assigned to C–O–C stretching (43,44). Chitosan’s broad peak at 3354 cm−1 and 3291 cm−1 are indexed to NH2 stretching vibration. Peaks at 1651 cm−1 and 1585 cm−1 are assigned to N–H bending of primary amine. Peaks 1199 cm−1 and 1150 cm−1 are attributed to C–O stretching of C–O–C. Two intense peaks at 1058 cm−1 and 1026 cm−1 indicate the presence of primary aliphatic amine stretching of C–N overlapping with secondary cyclic alcohol stretching of O–H 1 (42,43,45). A peak at 894 cm−1 is attributed to the pyranoid ring of chitosan (46).\nFig. 6 Stacked spectra of ATR-FTIR from 4000 to 600 cm−1 for PALNs, physical mixture, acyclovir, lecithin, chitosan, and PEG 2000\nLecithin’ IR spectrum displays characteristic N–H group stretching at peak 3379 cm−1 (47). Symmetry stretching of C–H for the cis-double bond is depicted at 3009 cm−1. Saturated aliphatic ester carbonyl stretching (–C=O) is represented at peak 1735 cm−1 (48). A strong, sharp peak at 1087 cm−1 shows a symmetric stretching of phosphate groups (42). The asymmetric stretchings of P=O which overlapped with P–O where both co-exist in phosphodiester group, together with P–O–C stretching, were at peaks 1238 cm−1 and 1062 cm−1 respectively. The peak at 968 cm−1 is attributed to N+–(CH3)3 asymmetric stretching overlapped with O–P–O deformation (49). Out of plane bending of HC–CN is depicted at 818 cm−1 (50).\nAcyclovir IR spectrum shows intermolecular and intra-molecular hydrogen-bonded hydroxyl group (O–H) stretchings at 3515 cm−1 and 3470 cm−1 (51), while NH2 symmetrical stretching is at 3438 cm−1 (52). Secondary amine stretching is at 3290 cm−1 (42). A peak at 3177 cm−1 is attributed to N–H stretching overlapped with O–H stretching (47). C–H and H–C–H stretchings are represented at 2927 cm−1 and 2854 cm−1 (42). The presence of C=O at 1708 cm−1, presence of C8–H together with ring vibration of guanine, was shown at 1483 cm−1, while the presence of guanine structure was inferred at 1370 cm−1 (42).\nThe IR spectra of PM represent the combination of all characteristic peaks, such as 2884 cm−1 and 2860 cm−1 (PEG 2000); 3354 cm−1 and 3291 cm−1 (chitosan); 3379 cm−1 and 3009 cm−1 (lecithin); and 3470 cm−1, 3438 cm−1, and 3177 cm−1(ACV). The peaks showed very slight shifting (± 5 cm−1), indicating no bonding interaction between drug and other formulation ingredients. PALN1 was observed that none of the ACV characteristic peaks emerged, indicating encapsulation of the drug.\n\nStability Study of PALN1\nTable VI displays the particle size of PALN1 at different sampling time. The t tests were conducted with a p value higher than 0.05 for all tests suggested that there was an insignificant variation in PALN1 particle size on each sampling time as compared with 0th day’s measurement. The obtained results were in agreement with a PALN1 zeta potential of 37.70 ± 1.16 mV which render the suspension stable by avoiding particle aggregation due to strong surface charge that repels each other. In brief, optimised formulations exhibit good physical stability for up to 60 days. There was no significant difference was observed p \u003c 0.05.\nTable 6 Particle Size and Zeta Potential of PALNs at Each Sampling Time\nDay Particle size (nm) Zeta potential (mV)\n0th 187.70 ± 3.75 37.94 ± 0.33\n15th 193.80 ± 5.73 38.26 ± 0.40\n30th 194.77 ± 2.05 38.22 ± 0.33\n45th 184.37 ± 4.00 37.83 ± 0.26\n60th 183.47 ± 4.47 37.32 ± 0.29\n\nIn Vitro Drug Release\nFigure 7 a and b showed ACV release profile in pH 1.2 (SGF) and pH 6.4 (SIF) respectively. Various kinetic models were employed for a comprehensive understanding of drug release mechanisms from the optimised formulation. Table VII shows the values of correlation coefficient (r2) and releasing model constant (k). Higuchi model showed the best fit with the highest r2 value and can be concluded that the PALN formulation showed modified release. This model corresponds to the sustained release properties of lecithin-chitosan nanoparticle. The drug release mechanism was identified based on the diffusion constant, n, from Korsmeyer-Peppas equation, as shown in Eq. (11). The mathematical adjustment of Eqs. (13) and (14) was obtained:13 Qt/QCR=KKPtn14 log10Qt/QCR=log10KKPnlog10twhere Qt and QCR indicate the amount of drug being released at time t and after time ∞ respectively. n represents the diffusional exponent and Kkp gives Korsmeyer release rate constant. Based on the equation, the slope of Korsemeyer-Peppas graph was the mechanism constant for Higuchi model. With n values reported to be 0.6185 and 0.6103 for SGF and SIF, respectively, it was concluded that PALN1 was following Higuchi drug releasing model with non-Fickian transport.\nFig. 7 ACV in vitro drug release profile in a pH 1.2, b pH 6.4, and c ACV intestinal permeation profile\nTable 7 Coefficient of Determination r2 and Releasing Model Constant K for Drug Releasing Models\nModel pH 1.2 pH 6.4\nCoefficient of determination, r2 Release model constant, K Coefficient of determination, r2 Release model constant, K\nZero order 0.6184 3.1424 0.5605 3.0729\nFirst order 0.9339 0.2588 0.7999 1.3440\nHiguchi model 0.9341 25.4563 0.9199 26.5229\nKorsmeyer-Peppas model 0.3101 18.6351 0.2953 19.8312\nHixson-Crowell model 0.0053 0.0131 0.0032 0.0100\nThe italicized is done to show the Nanoparticle release model is folloing Higuchi release model. It is done just to make it more visible\n\nStudy of Ex Vivo Drug Permeability\nFigure 7 c shows the cumulative percentage of drug permeated against time. Table VIII shows that at the 10th hour, only 58% of the drug was permeated to the releasing media. The drug permeation profile had been prolonged due to the smaller porosity and thicker intestinal membrane compared with the dialysis membrane. The surface area of the intestine accommodating formulation was 5 cm2.15 Pappcms−1=1.1626mg10×60×60s×15cm2×1mg/cm3=6.4589×10−6cms−116 Jssmgcm−2s−1=6.4589×10−6cms−1×1mg/cm3=6.4589×10−6mgcm−2s−1\nTable 8 Amount, Percentage of Acyclovir Permeated, and Apparent Permeability at Each Time Point\nt Amount permeated % permeated Apparent permeability (mg/cm2)\n0 0.0000 ± 0.0000 00.00 ± 0.00 0.0000 ± 0.0000\n0.5 0.5902 ± 0.0009 29.51 ± 0.05 0.1180 ± 0.0002\n1 0.8525 ± 0.0003 42.63 ± 0.01 0.1705 ± 0.0001\n2 1.0565 ± 0.0010 52.83 ± 0.05 0.2113 ± 0.0002\n4 1.0806 ± 0.0003 54.03 ± 0.01 0.2161 ± 0.0001\n6 1.0937 ± 0.0001 54.69 ± 0.00 0.2187 ± 0.0000\n8 1.1074 ± 0.0002 55.37 ± 0.01 0.2215 ± 0.0000\n10 1.1626 ± 0.0016 58.13 ± 0.08 0.2325 ± 0.0003\nPapp of 6.4589 × 10−6 cm/s was a 3.2-fold improvement as compared with reported value of 0.12 × 10−6 to 2.0 × 10−6 cm s−1 (8). The optimised PALN formulation had the potential to be used in tablet formulation with improved drug intestinal permeability."}