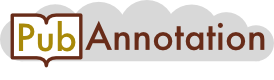
PMC:7473770 / 1329-9777
Annnotations
LitCovid-PD-FMA-UBERON
{"project":"LitCovid-PD-FMA-UBERON","denotations":[{"id":"T12","span":{"begin":188,"end":193},"obj":"Body_part"},{"id":"T13","span":{"begin":261,"end":265},"obj":"Body_part"},{"id":"T14","span":{"begin":334,"end":339},"obj":"Body_part"},{"id":"T15","span":{"begin":542,"end":546},"obj":"Body_part"},{"id":"T16","span":{"begin":675,"end":683},"obj":"Body_part"},{"id":"T17","span":{"begin":1104,"end":1122},"obj":"Body_part"},{"id":"T18","span":{"begin":1218,"end":1226},"obj":"Body_part"},{"id":"T19","span":{"begin":1227,"end":1236},"obj":"Body_part"},{"id":"T20","span":{"begin":1442,"end":1447},"obj":"Body_part"},{"id":"T21","span":{"begin":1589,"end":1594},"obj":"Body_part"},{"id":"T22","span":{"begin":1704,"end":1710},"obj":"Body_part"},{"id":"T23","span":{"begin":1828,"end":1832},"obj":"Body_part"},{"id":"T24","span":{"begin":1947,"end":1952},"obj":"Body_part"},{"id":"T25","span":{"begin":2224,"end":2230},"obj":"Body_part"},{"id":"T26","span":{"begin":2251,"end":2255},"obj":"Body_part"},{"id":"T27","span":{"begin":2966,"end":2970},"obj":"Body_part"},{"id":"T28","span":{"begin":3064,"end":3077},"obj":"Body_part"},{"id":"T29","span":{"begin":3064,"end":3069},"obj":"Body_part"},{"id":"T30","span":{"begin":3333,"end":3337},"obj":"Body_part"},{"id":"T31","span":{"begin":3588,"end":3592},"obj":"Body_part"},{"id":"T32","span":{"begin":3673,"end":3679},"obj":"Body_part"},{"id":"T33","span":{"begin":3708,"end":3719},"obj":"Body_part"},{"id":"T34","span":{"begin":4338,"end":4345},"obj":"Body_part"},{"id":"T35","span":{"begin":4922,"end":4926},"obj":"Body_part"},{"id":"T36","span":{"begin":5538,"end":5544},"obj":"Body_part"},{"id":"T37","span":{"begin":6093,"end":6099},"obj":"Body_part"},{"id":"T38","span":{"begin":6781,"end":6786},"obj":"Body_part"},{"id":"T39","span":{"begin":6800,"end":6806},"obj":"Body_part"},{"id":"T40","span":{"begin":7599,"end":7605},"obj":"Body_part"},{"id":"T41","span":{"begin":7928,"end":7934},"obj":"Body_part"},{"id":"T42","span":{"begin":8155,"end":8159},"obj":"Body_part"},{"id":"T43","span":{"begin":8160,"end":8167},"obj":"Body_part"}],"attributes":[{"id":"A12","pred":"fma_id","subj":"T12","obj":"http://purl.org/sig/ont/fma/fma67498"},{"id":"A13","pred":"fma_id","subj":"T13","obj":"http://purl.org/sig/ont/fma/fma7195"},{"id":"A14","pred":"fma_id","subj":"T14","obj":"http://purl.org/sig/ont/fma/fma9576"},{"id":"A15","pred":"fma_id","subj":"T15","obj":"http://purl.org/sig/ont/fma/fma7195"},{"id":"A16","pred":"fma_id","subj":"T16","obj":"http://purl.org/sig/ont/fma/fma264783"},{"id":"A17","pred":"fma_id","subj":"T17","obj":"http://purl.org/sig/ont/fma/fma63841"},{"id":"A18","pred":"fma_id","subj":"T18","obj":"http://purl.org/sig/ont/fma/fma264783"},{"id":"A19","pred":"fma_id","subj":"T19","obj":"http://purl.org/sig/ont/fma/fma63194"},{"id":"A20","pred":"fma_id","subj":"T20","obj":"http://purl.org/sig/ont/fma/fma68877"},{"id":"A21","pred":"fma_id","subj":"T21","obj":"http://purl.org/sig/ont/fma/fma68877"},{"id":"A22","pred":"fma_id","subj":"T22","obj":"http://purl.org/sig/ont/fma/fma9637"},{"id":"A23","pred":"fma_id","subj":"T23","obj":"http://purl.org/sig/ont/fma/fma7195"},{"id":"A24","pred":"fma_id","subj":"T24","obj":"http://purl.org/sig/ont/fma/fma68877"},{"id":"A25","pred":"fma_id","subj":"T25","obj":"http://purl.org/sig/ont/fma/fma9637"},{"id":"A26","pred":"fma_id","subj":"T26","obj":"http://purl.org/sig/ont/fma/fma7195"},{"id":"A27","pred":"fma_id","subj":"T27","obj":"http://purl.org/sig/ont/fma/fma74402"},{"id":"A28","pred":"fma_id","subj":"T28","obj":"http://purl.org/sig/ont/fma/fma63183"},{"id":"A29","pred":"fma_id","subj":"T29","obj":"http://purl.org/sig/ont/fma/fma9670"},{"id":"A30","pred":"fma_id","subj":"T30","obj":"http://purl.org/sig/ont/fma/fma7195"},{"id":"A31","pred":"fma_id","subj":"T31","obj":"http://purl.org/sig/ont/fma/fma68646"},{"id":"A32","pred":"fma_id","subj":"T32","obj":"http://purl.org/sig/ont/fma/fma9637"},{"id":"A33","pred":"fma_id","subj":"T33","obj":"http://purl.org/sig/ont/fma/fma76577"},{"id":"A34","pred":"fma_id","subj":"T34","obj":"http://purl.org/sig/ont/fma/fma9637"},{"id":"A35","pred":"fma_id","subj":"T35","obj":"http://purl.org/sig/ont/fma/fma7195"},{"id":"A36","pred":"fma_id","subj":"T36","obj":"http://purl.org/sig/ont/fma/fma9637"},{"id":"A37","pred":"fma_id","subj":"T37","obj":"http://purl.org/sig/ont/fma/fma9637"},{"id":"A38","pred":"fma_id","subj":"T38","obj":"http://purl.org/sig/ont/fma/fma68646"},{"id":"A39","pred":"fma_id","subj":"T39","obj":"http://purl.org/sig/ont/fma/fma9637"},{"id":"A40","pred":"fma_id","subj":"T40","obj":"http://purl.org/sig/ont/fma/fma9637"},{"id":"A41","pred":"fma_id","subj":"T41","obj":"http://purl.org/sig/ont/fma/fma9637"},{"id":"A42","pred":"fma_id","subj":"T42","obj":"http://purl.org/sig/ont/fma/fma7195"},{"id":"A43","pred":"fma_id","subj":"T43","obj":"http://purl.org/sig/ont/fma/fma9637"}],"text":"Introduction\nSevere progression of the 2019 coronavirus disease (Covid-19) is frequently accompanied by the clinical acute respiratory distress syndrome (ARDS) and respiratory failure, an organ manifestation responsible for the majority of Covid-19 fatalities. Lung injury associated with ARDS can be readily detected by radiographic chest imaging and clinical computed tomography (CT), which have assisted the diagnosis and management of Covid-19 patients (Lee et al., 2020; Shi et al., 2020; Chung et al., 2020). Here, so-called peripheral lung ground-glass opacities are the main radiological hallmark of ARDS, and can be linked to the histological observation of diffuse alveolar damage (DAD) with edema, hemorrhage, and intraalveolar fibrin deposition (Ackermann et al., 2020). These findings have also been reported for infections by Middle East respiratory syndrome coronavirus (MERS-CoV), SARS-CoV, and influenza viruses. Distinctive features of pulmonary involvement of Covid-19 include severe endothelial injury associated with the presence of intracellular virions and inflammation, disrupted cellular membranes, as well as widespread thrombosis with microangiopathy. As reported in Ackermann et al., 2020, alveolar capillary microthrombi were found to be nine times as prevalent in patients with Covid-19 as in patients with the also very aggressive H1N1 influenza A virus, also referred to as swine flu. Importantly, in Covid-19 lungs, a specific variant of new vessel growth - intussusceptive angiogenesis - was significantly more prevalent, that is, 2.7 times as high as in lungs of patients with H1N1 influenza A.\nHistomorphological assessment of formalin-fixed, paraffin-embedded (FFPE) tissue stained with haematoxylin and eosin still represents the gold standard in histological diagnostics of non-neoplastic lung diseases, including DAD and virus induced pneumonia. In order to unravel the corresponding pathophysiology of the lungs, digitalization, visualization and quantification of the morphological changes associated with Covid-19 represent a key challenge, and require both high resolution and the capability to screen larger volumes. For this reason, imaging the intricate three-dimensional (3D) tissue architecture of the lung and its pathological alterations on multiple length scales calls for 3D extensions of well-established histology techniques.\nIn this work, we want to demonstrate the potential of propagation-based phase contrast x-ray tomography as a tool for virtual 3D histology in general, and in particular for the histopathology of Covid-19. Our work is based on the assertion that integration of 3D morphological information with well-established histology techniques can provide a substantial asset for unraveling the pathophysiology of SARS-Cov-2 infections. To this end, we have collected x-ray tomography data from the same autopsies, which were previously studied by immunohistochemical analysis and measurements of gene expression (Ackermann et al., 2020). We ask in particular, whether DAD and the morphology of blood vessels can be visualized and quantified in 3D. This is a timely scientific question in Covid-19 research, especially in view of increased intussusceptive angiogenesis reported in Ackermann et al., 2020. Here we present first results obtained from the postmortem lung samples of six patients who succumbed from Covid-19. We exemplify the capability of this approach by 3D visualization of the DAD with hyaline membrane formation, by mapping the 3D distribution and density of angiocentric inflammation (perivascular T-cell infiltration), and by providing histograms of characteristic distances from the tissue interior to the closest air compartment.\nIn contrast to conventional histology based on thin sections, propagation-based x-ray phase-contrast tomography (PC-CT) offers a full 3D visualization with isotropic resolution and without destructive slicing of the specimen (Ding et al., 2019; Dejea et al., 2019; Saccomano et al., 2018; Töpperwien et al., 2018; Khimchenko et al., 2016). The interaction of x-rays with the object is described by the continuous complex-valued index of refraction n(𝐫)=1-δ(𝐫)+iβ(𝐫). Phase contrast capitalizes on the fact that for hard x-rays, the real-valued decrement δ is several orders of magnitude higher in soft biological tissues than β, which accounts for absorption (Nugent, 2010) and that small contrast levels can be reconstructed by propagation imaging even at low fluence (Jahn et al., 2017). Contrast is formed by transformation of the phase shifts into measurable intensity variations by self-interference of the exit wave during free-space propagation between sample and detector (Paganin and Nugent, 1998; Cloetens et al., 1999). However, problems with phase retrieval, phase wrapping, insufficient coherence, or on the contrary strong phase gradients can render its application challenging. For lung imaging it has already been demonstrated as an advantageous imaging technique up to macroscopic scales (Parsons et al., 2008; Stahr et al., 2016; Morgan et al., 2020), including live animal models of respiratory diseases. But in contrast to other full-field phase contrast techniques, e.g. based on grating interferometry or analyzer crystals, it can also reach a resolution below optical microscopy (Khimchenko et al., 2018). In this work we exploit both the high resolution capability of PC-CT and the fact that its phase sensitivity is high enough to probe the small electron density variations of unstained tissue, embedded in paraffin, ethanol, or even aqueous buffer (Töpperwien et al., 2019). However, this requires careful optimization of photon energy, illumination function, and phase retrieval algorithms, as detailed further below.\nThe 3D virtual pathohistology approach for Covid-19 presented here was realized by implementing a novel multi-scale phase contrast x-ray tomography concept, with dedicated x-ray optics and instrumentation. Overview and regions-of-interest (ROI) scans were recorded on the same paraffin-embedded sample, covering a maximum tissue cross section of 8 mm by stitching different tomograms, and with a minimum voxel size of 167 nm in certain ROIs. Scale-bridging and dynamic ROI selection in close spatial and temporal proximity was implemented with dedicated instrumentation the GINIX endstation of the beamline P10/PETRA III (DESY, Hamburg) (Salditt et al., 2015). Specifically, we combined two optical geometries, which has only been realized at different synchrotron beamlines before: (i) Parallel beam tomography, covering a large field of view (FOV), with a pixel size of 650 nm. In this setting, a volumetric throughput on the order of 107μm/3s was achieved, while maintaining the ability to segment isolated cells in unstained tissue. (ii) Cone beam geometry for recording of highly magnified holograms, based on advanced x-ray waveguide optics, providing mode filtering, that is, enhanced spatial coherence and smooth wavefronts. Based on the geometrical magnification, the effective pixel size can be adjusted in the range 10 nm–300 nm. The two imaging schemes are shown schematically in Figure 1c and d, respectively. Further, using this particular optics, together with appropriate choices of photon energy and geometric parameters, we can reach extremely small Fresnel numbers F of the deeply holographic regime, well below the typical range exploited at other nano-tomography instruments. This offers the advantage of highest phase sensitivity sufficient to even probe the small electron density variations of unstained tissue, at relatively low dose (Hagemann and Salditt, 2018). To exploit this sensitivity, we use advanced phase retrieval methods including non-linear generalizations of the CTF-method (Cloetens et al., 1999) based on Tikhonov regularization (Lohse et al., 2020).\nFigure 1. Multi-scale x-ray tomography setup.\n(a) HE stain of a tissue micro-array paraffin block with samples of all six patients who succumbed to Covid-19. (b) Schematic representation of the sample preparation and mounting. In a first step biopsy punches containing the entire individual lung tissues are transferred onto a holder for the parallel-beam local tomography acquisition, followed by a further reduction in size to a 1 mm biopsy punch, for cone-beam tomographic recordings. (c) Configuration for parallel-beam tomography. (d) Configuration for cone-beam holo-tomography."}
LitCovid-PD-UBERON
{"project":"LitCovid-PD-UBERON","denotations":[{"id":"T10","span":{"begin":188,"end":193},"obj":"Body_part"},{"id":"T11","span":{"begin":261,"end":265},"obj":"Body_part"},{"id":"T12","span":{"begin":334,"end":339},"obj":"Body_part"},{"id":"T13","span":{"begin":542,"end":546},"obj":"Body_part"},{"id":"T14","span":{"begin":1227,"end":1236},"obj":"Body_part"},{"id":"T15","span":{"begin":1475,"end":1481},"obj":"Body_part"},{"id":"T16","span":{"begin":1704,"end":1710},"obj":"Body_part"},{"id":"T17","span":{"begin":1828,"end":1832},"obj":"Body_part"},{"id":"T18","span":{"begin":2224,"end":2230},"obj":"Body_part"},{"id":"T19","span":{"begin":2251,"end":2255},"obj":"Body_part"},{"id":"T20","span":{"begin":2308,"end":2314},"obj":"Body_part"},{"id":"T21","span":{"begin":2328,"end":2338},"obj":"Body_part"},{"id":"T22","span":{"begin":3064,"end":3077},"obj":"Body_part"},{"id":"T23","span":{"begin":3064,"end":3069},"obj":"Body_part"},{"id":"T24","span":{"begin":3070,"end":3077},"obj":"Body_part"},{"id":"T25","span":{"begin":3333,"end":3337},"obj":"Body_part"},{"id":"T26","span":{"begin":3673,"end":3679},"obj":"Body_part"},{"id":"T27","span":{"begin":4922,"end":4926},"obj":"Body_part"},{"id":"T28","span":{"begin":5023,"end":5029},"obj":"Body_part"},{"id":"T29","span":{"begin":5538,"end":5544},"obj":"Body_part"},{"id":"T30","span":{"begin":5881,"end":5886},"obj":"Body_part"},{"id":"T31","span":{"begin":6093,"end":6099},"obj":"Body_part"},{"id":"T32","span":{"begin":6213,"end":6218},"obj":"Body_part"},{"id":"T33","span":{"begin":6800,"end":6806},"obj":"Body_part"},{"id":"T34","span":{"begin":7599,"end":7605},"obj":"Body_part"},{"id":"T35","span":{"begin":7880,"end":7885},"obj":"Body_part"},{"id":"T36","span":{"begin":7928,"end":7934},"obj":"Body_part"},{"id":"T37","span":{"begin":8155,"end":8159},"obj":"Body_part"}],"attributes":[{"id":"A10","pred":"uberon_id","subj":"T10","obj":"http://purl.obolibrary.org/obo/UBERON_0000062"},{"id":"A11","pred":"uberon_id","subj":"T11","obj":"http://purl.obolibrary.org/obo/UBERON_0002048"},{"id":"A12","pred":"uberon_id","subj":"T12","obj":"http://purl.obolibrary.org/obo/UBERON_0001443"},{"id":"A13","pred":"uberon_id","subj":"T13","obj":"http://purl.obolibrary.org/obo/UBERON_0002048"},{"id":"A14","pred":"uberon_id","subj":"T14","obj":"http://purl.obolibrary.org/obo/UBERON_0001982"},{"id":"A15","pred":"uberon_id","subj":"T15","obj":"http://purl.obolibrary.org/obo/UBERON_0000055"},{"id":"A16","pred":"uberon_id","subj":"T16","obj":"http://purl.obolibrary.org/obo/UBERON_0000479"},{"id":"A17","pred":"uberon_id","subj":"T17","obj":"http://purl.obolibrary.org/obo/UBERON_0002048"},{"id":"A18","pred":"uberon_id","subj":"T18","obj":"http://purl.obolibrary.org/obo/UBERON_0000479"},{"id":"A19","pred":"uberon_id","subj":"T19","obj":"http://purl.obolibrary.org/obo/UBERON_0002048"},{"id":"A20","pred":"uberon_id","subj":"T20","obj":"http://purl.obolibrary.org/obo/UBERON_0002542"},{"id":"A21","pred":"uberon_id","subj":"T21","obj":"http://purl.obolibrary.org/obo/UBERON_2000106"},{"id":"A22","pred":"uberon_id","subj":"T22","obj":"http://purl.obolibrary.org/obo/UBERON_0001981"},{"id":"A23","pred":"uberon_id","subj":"T23","obj":"http://purl.obolibrary.org/obo/UBERON_0000178"},{"id":"A24","pred":"uberon_id","subj":"T24","obj":"http://purl.obolibrary.org/obo/UBERON_0000055"},{"id":"A25","pred":"uberon_id","subj":"T25","obj":"http://purl.obolibrary.org/obo/UBERON_0002048"},{"id":"A26","pred":"uberon_id","subj":"T26","obj":"http://purl.obolibrary.org/obo/UBERON_0000479"},{"id":"A27","pred":"uberon_id","subj":"T27","obj":"http://purl.obolibrary.org/obo/UBERON_0002048"},{"id":"A28","pred":"uberon_id","subj":"T28","obj":"http://purl.obolibrary.org/obo/UBERON_0002542"},{"id":"A29","pred":"uberon_id","subj":"T29","obj":"http://purl.obolibrary.org/obo/UBERON_0000479"},{"id":"A30","pred":"uberon_id","subj":"T30","obj":"http://purl.obolibrary.org/obo/UBERON_0002542"},{"id":"A31","pred":"uberon_id","subj":"T31","obj":"http://purl.obolibrary.org/obo/UBERON_0000479"},{"id":"A32","pred":"uberon_id","subj":"T32","obj":"http://purl.obolibrary.org/obo/UBERON_0002542"},{"id":"A33","pred":"uberon_id","subj":"T33","obj":"http://purl.obolibrary.org/obo/UBERON_0000479"},{"id":"A34","pred":"uberon_id","subj":"T34","obj":"http://purl.obolibrary.org/obo/UBERON_0000479"},{"id":"A35","pred":"uberon_id","subj":"T35","obj":"http://purl.obolibrary.org/obo/UBERON_0002542"},{"id":"A36","pred":"uberon_id","subj":"T36","obj":"http://purl.obolibrary.org/obo/UBERON_0000479"},{"id":"A37","pred":"uberon_id","subj":"T37","obj":"http://purl.obolibrary.org/obo/UBERON_0002048"}],"text":"Introduction\nSevere progression of the 2019 coronavirus disease (Covid-19) is frequently accompanied by the clinical acute respiratory distress syndrome (ARDS) and respiratory failure, an organ manifestation responsible for the majority of Covid-19 fatalities. Lung injury associated with ARDS can be readily detected by radiographic chest imaging and clinical computed tomography (CT), which have assisted the diagnosis and management of Covid-19 patients (Lee et al., 2020; Shi et al., 2020; Chung et al., 2020). Here, so-called peripheral lung ground-glass opacities are the main radiological hallmark of ARDS, and can be linked to the histological observation of diffuse alveolar damage (DAD) with edema, hemorrhage, and intraalveolar fibrin deposition (Ackermann et al., 2020). These findings have also been reported for infections by Middle East respiratory syndrome coronavirus (MERS-CoV), SARS-CoV, and influenza viruses. Distinctive features of pulmonary involvement of Covid-19 include severe endothelial injury associated with the presence of intracellular virions and inflammation, disrupted cellular membranes, as well as widespread thrombosis with microangiopathy. As reported in Ackermann et al., 2020, alveolar capillary microthrombi were found to be nine times as prevalent in patients with Covid-19 as in patients with the also very aggressive H1N1 influenza A virus, also referred to as swine flu. Importantly, in Covid-19 lungs, a specific variant of new vessel growth - intussusceptive angiogenesis - was significantly more prevalent, that is, 2.7 times as high as in lungs of patients with H1N1 influenza A.\nHistomorphological assessment of formalin-fixed, paraffin-embedded (FFPE) tissue stained with haematoxylin and eosin still represents the gold standard in histological diagnostics of non-neoplastic lung diseases, including DAD and virus induced pneumonia. In order to unravel the corresponding pathophysiology of the lungs, digitalization, visualization and quantification of the morphological changes associated with Covid-19 represent a key challenge, and require both high resolution and the capability to screen larger volumes. For this reason, imaging the intricate three-dimensional (3D) tissue architecture of the lung and its pathological alterations on multiple length scales calls for 3D extensions of well-established histology techniques.\nIn this work, we want to demonstrate the potential of propagation-based phase contrast x-ray tomography as a tool for virtual 3D histology in general, and in particular for the histopathology of Covid-19. Our work is based on the assertion that integration of 3D morphological information with well-established histology techniques can provide a substantial asset for unraveling the pathophysiology of SARS-Cov-2 infections. To this end, we have collected x-ray tomography data from the same autopsies, which were previously studied by immunohistochemical analysis and measurements of gene expression (Ackermann et al., 2020). We ask in particular, whether DAD and the morphology of blood vessels can be visualized and quantified in 3D. This is a timely scientific question in Covid-19 research, especially in view of increased intussusceptive angiogenesis reported in Ackermann et al., 2020. Here we present first results obtained from the postmortem lung samples of six patients who succumbed from Covid-19. We exemplify the capability of this approach by 3D visualization of the DAD with hyaline membrane formation, by mapping the 3D distribution and density of angiocentric inflammation (perivascular T-cell infiltration), and by providing histograms of characteristic distances from the tissue interior to the closest air compartment.\nIn contrast to conventional histology based on thin sections, propagation-based x-ray phase-contrast tomography (PC-CT) offers a full 3D visualization with isotropic resolution and without destructive slicing of the specimen (Ding et al., 2019; Dejea et al., 2019; Saccomano et al., 2018; Töpperwien et al., 2018; Khimchenko et al., 2016). The interaction of x-rays with the object is described by the continuous complex-valued index of refraction n(𝐫)=1-δ(𝐫)+iβ(𝐫). Phase contrast capitalizes on the fact that for hard x-rays, the real-valued decrement δ is several orders of magnitude higher in soft biological tissues than β, which accounts for absorption (Nugent, 2010) and that small contrast levels can be reconstructed by propagation imaging even at low fluence (Jahn et al., 2017). Contrast is formed by transformation of the phase shifts into measurable intensity variations by self-interference of the exit wave during free-space propagation between sample and detector (Paganin and Nugent, 1998; Cloetens et al., 1999). However, problems with phase retrieval, phase wrapping, insufficient coherence, or on the contrary strong phase gradients can render its application challenging. For lung imaging it has already been demonstrated as an advantageous imaging technique up to macroscopic scales (Parsons et al., 2008; Stahr et al., 2016; Morgan et al., 2020), including live animal models of respiratory diseases. But in contrast to other full-field phase contrast techniques, e.g. based on grating interferometry or analyzer crystals, it can also reach a resolution below optical microscopy (Khimchenko et al., 2018). In this work we exploit both the high resolution capability of PC-CT and the fact that its phase sensitivity is high enough to probe the small electron density variations of unstained tissue, embedded in paraffin, ethanol, or even aqueous buffer (Töpperwien et al., 2019). However, this requires careful optimization of photon energy, illumination function, and phase retrieval algorithms, as detailed further below.\nThe 3D virtual pathohistology approach for Covid-19 presented here was realized by implementing a novel multi-scale phase contrast x-ray tomography concept, with dedicated x-ray optics and instrumentation. Overview and regions-of-interest (ROI) scans were recorded on the same paraffin-embedded sample, covering a maximum tissue cross section of 8 mm by stitching different tomograms, and with a minimum voxel size of 167 nm in certain ROIs. Scale-bridging and dynamic ROI selection in close spatial and temporal proximity was implemented with dedicated instrumentation the GINIX endstation of the beamline P10/PETRA III (DESY, Hamburg) (Salditt et al., 2015). Specifically, we combined two optical geometries, which has only been realized at different synchrotron beamlines before: (i) Parallel beam tomography, covering a large field of view (FOV), with a pixel size of 650 nm. In this setting, a volumetric throughput on the order of 107μm/3s was achieved, while maintaining the ability to segment isolated cells in unstained tissue. (ii) Cone beam geometry for recording of highly magnified holograms, based on advanced x-ray waveguide optics, providing mode filtering, that is, enhanced spatial coherence and smooth wavefronts. Based on the geometrical magnification, the effective pixel size can be adjusted in the range 10 nm–300 nm. The two imaging schemes are shown schematically in Figure 1c and d, respectively. Further, using this particular optics, together with appropriate choices of photon energy and geometric parameters, we can reach extremely small Fresnel numbers F of the deeply holographic regime, well below the typical range exploited at other nano-tomography instruments. This offers the advantage of highest phase sensitivity sufficient to even probe the small electron density variations of unstained tissue, at relatively low dose (Hagemann and Salditt, 2018). To exploit this sensitivity, we use advanced phase retrieval methods including non-linear generalizations of the CTF-method (Cloetens et al., 1999) based on Tikhonov regularization (Lohse et al., 2020).\nFigure 1. Multi-scale x-ray tomography setup.\n(a) HE stain of a tissue micro-array paraffin block with samples of all six patients who succumbed to Covid-19. (b) Schematic representation of the sample preparation and mounting. In a first step biopsy punches containing the entire individual lung tissues are transferred onto a holder for the parallel-beam local tomography acquisition, followed by a further reduction in size to a 1 mm biopsy punch, for cone-beam tomographic recordings. (c) Configuration for parallel-beam tomography. (d) Configuration for cone-beam holo-tomography."}
LitCovid-PD-MONDO
{"project":"LitCovid-PD-MONDO","denotations":[{"id":"T1","span":{"begin":117,"end":152},"obj":"Disease"},{"id":"T2","span":{"begin":123,"end":152},"obj":"Disease"},{"id":"T3","span":{"begin":154,"end":158},"obj":"Disease"},{"id":"T4","span":{"begin":164,"end":183},"obj":"Disease"},{"id":"T5","span":{"begin":266,"end":272},"obj":"Disease"},{"id":"T6","span":{"begin":289,"end":293},"obj":"Disease"},{"id":"T7","span":{"begin":608,"end":612},"obj":"Disease"},{"id":"T8","span":{"begin":826,"end":836},"obj":"Disease"},{"id":"T9","span":{"begin":897,"end":905},"obj":"Disease"},{"id":"T10","span":{"begin":911,"end":920},"obj":"Disease"},{"id":"T11","span":{"begin":1015,"end":1021},"obj":"Disease"},{"id":"T12","span":{"begin":1080,"end":1092},"obj":"Disease"},{"id":"T13","span":{"begin":1146,"end":1156},"obj":"Disease"},{"id":"T14","span":{"begin":1362,"end":1376},"obj":"Disease"},{"id":"T15","span":{"begin":1367,"end":1376},"obj":"Disease"},{"id":"T16","span":{"begin":1406,"end":1415},"obj":"Disease"},{"id":"T17","span":{"begin":1412,"end":1415},"obj":"Disease"},{"id":"T18","span":{"begin":1612,"end":1626},"obj":"Disease"},{"id":"T19","span":{"begin":1617,"end":1626},"obj":"Disease"},{"id":"T20","span":{"begin":1828,"end":1841},"obj":"Disease"},{"id":"T21","span":{"begin":1875,"end":1884},"obj":"Disease"},{"id":"T22","span":{"begin":2783,"end":2787},"obj":"Disease"},{"id":"T23","span":{"begin":2794,"end":2804},"obj":"Disease"},{"id":"T24","span":{"begin":3559,"end":3571},"obj":"Disease"},{"id":"T25","span":{"begin":5127,"end":5147},"obj":"Disease"}],"attributes":[{"id":"A1","pred":"mondo_id","subj":"T1","obj":"http://purl.obolibrary.org/obo/MONDO_0006502"},{"id":"A2","pred":"mondo_id","subj":"T2","obj":"http://purl.obolibrary.org/obo/MONDO_0009971"},{"id":"A3","pred":"mondo_id","subj":"T3","obj":"http://purl.obolibrary.org/obo/MONDO_0006502"},{"id":"A4","pred":"mondo_id","subj":"T4","obj":"http://purl.obolibrary.org/obo/MONDO_0021113"},{"id":"A5","pred":"mondo_id","subj":"T5","obj":"http://purl.obolibrary.org/obo/MONDO_0021178"},{"id":"A6","pred":"mondo_id","subj":"T6","obj":"http://purl.obolibrary.org/obo/MONDO_0006502"},{"id":"A7","pred":"mondo_id","subj":"T7","obj":"http://purl.obolibrary.org/obo/MONDO_0006502"},{"id":"A8","pred":"mondo_id","subj":"T8","obj":"http://purl.obolibrary.org/obo/MONDO_0005550"},{"id":"A9","pred":"mondo_id","subj":"T9","obj":"http://purl.obolibrary.org/obo/MONDO_0005091"},{"id":"A10","pred":"mondo_id","subj":"T10","obj":"http://purl.obolibrary.org/obo/MONDO_0005812"},{"id":"A11","pred":"mondo_id","subj":"T11","obj":"http://purl.obolibrary.org/obo/MONDO_0021178"},{"id":"A12","pred":"mondo_id","subj":"T12","obj":"http://purl.obolibrary.org/obo/MONDO_0021166"},{"id":"A13","pred":"mondo_id","subj":"T13","obj":"http://purl.obolibrary.org/obo/MONDO_0000831"},{"id":"A14","pred":"mondo_id","subj":"T14","obj":"http://purl.obolibrary.org/obo/MONDO_0005460"},{"id":"A15","pred":"mondo_id","subj":"T15","obj":"http://purl.obolibrary.org/obo/MONDO_0005812"},{"id":"A16","pred":"mondo_id","subj":"T16","obj":"http://purl.obolibrary.org/obo/MONDO_0005460"},{"id":"A17","pred":"mondo_id","subj":"T17","obj":"http://purl.obolibrary.org/obo/MONDO_0005812"},{"id":"A18","pred":"mondo_id","subj":"T18","obj":"http://purl.obolibrary.org/obo/MONDO_0005460"},{"id":"A19","pred":"mondo_id","subj":"T19","obj":"http://purl.obolibrary.org/obo/MONDO_0005812"},{"id":"A20","pred":"mondo_id","subj":"T20","obj":"http://purl.obolibrary.org/obo/MONDO_0005275"},{"id":"A21","pred":"mondo_id","subj":"T21","obj":"http://purl.obolibrary.org/obo/MONDO_0005249"},{"id":"A22","pred":"mondo_id","subj":"T22","obj":"http://purl.obolibrary.org/obo/MONDO_0005091"},{"id":"A23","pred":"mondo_id","subj":"T23","obj":"http://purl.obolibrary.org/obo/MONDO_0005550"},{"id":"A24","pred":"mondo_id","subj":"T24","obj":"http://purl.obolibrary.org/obo/MONDO_0021166"},{"id":"A25","pred":"mondo_id","subj":"T25","obj":"http://purl.obolibrary.org/obo/MONDO_0005087"}],"text":"Introduction\nSevere progression of the 2019 coronavirus disease (Covid-19) is frequently accompanied by the clinical acute respiratory distress syndrome (ARDS) and respiratory failure, an organ manifestation responsible for the majority of Covid-19 fatalities. Lung injury associated with ARDS can be readily detected by radiographic chest imaging and clinical computed tomography (CT), which have assisted the diagnosis and management of Covid-19 patients (Lee et al., 2020; Shi et al., 2020; Chung et al., 2020). Here, so-called peripheral lung ground-glass opacities are the main radiological hallmark of ARDS, and can be linked to the histological observation of diffuse alveolar damage (DAD) with edema, hemorrhage, and intraalveolar fibrin deposition (Ackermann et al., 2020). These findings have also been reported for infections by Middle East respiratory syndrome coronavirus (MERS-CoV), SARS-CoV, and influenza viruses. Distinctive features of pulmonary involvement of Covid-19 include severe endothelial injury associated with the presence of intracellular virions and inflammation, disrupted cellular membranes, as well as widespread thrombosis with microangiopathy. As reported in Ackermann et al., 2020, alveolar capillary microthrombi were found to be nine times as prevalent in patients with Covid-19 as in patients with the also very aggressive H1N1 influenza A virus, also referred to as swine flu. Importantly, in Covid-19 lungs, a specific variant of new vessel growth - intussusceptive angiogenesis - was significantly more prevalent, that is, 2.7 times as high as in lungs of patients with H1N1 influenza A.\nHistomorphological assessment of formalin-fixed, paraffin-embedded (FFPE) tissue stained with haematoxylin and eosin still represents the gold standard in histological diagnostics of non-neoplastic lung diseases, including DAD and virus induced pneumonia. In order to unravel the corresponding pathophysiology of the lungs, digitalization, visualization and quantification of the morphological changes associated with Covid-19 represent a key challenge, and require both high resolution and the capability to screen larger volumes. For this reason, imaging the intricate three-dimensional (3D) tissue architecture of the lung and its pathological alterations on multiple length scales calls for 3D extensions of well-established histology techniques.\nIn this work, we want to demonstrate the potential of propagation-based phase contrast x-ray tomography as a tool for virtual 3D histology in general, and in particular for the histopathology of Covid-19. Our work is based on the assertion that integration of 3D morphological information with well-established histology techniques can provide a substantial asset for unraveling the pathophysiology of SARS-Cov-2 infections. To this end, we have collected x-ray tomography data from the same autopsies, which were previously studied by immunohistochemical analysis and measurements of gene expression (Ackermann et al., 2020). We ask in particular, whether DAD and the morphology of blood vessels can be visualized and quantified in 3D. This is a timely scientific question in Covid-19 research, especially in view of increased intussusceptive angiogenesis reported in Ackermann et al., 2020. Here we present first results obtained from the postmortem lung samples of six patients who succumbed from Covid-19. We exemplify the capability of this approach by 3D visualization of the DAD with hyaline membrane formation, by mapping the 3D distribution and density of angiocentric inflammation (perivascular T-cell infiltration), and by providing histograms of characteristic distances from the tissue interior to the closest air compartment.\nIn contrast to conventional histology based on thin sections, propagation-based x-ray phase-contrast tomography (PC-CT) offers a full 3D visualization with isotropic resolution and without destructive slicing of the specimen (Ding et al., 2019; Dejea et al., 2019; Saccomano et al., 2018; Töpperwien et al., 2018; Khimchenko et al., 2016). The interaction of x-rays with the object is described by the continuous complex-valued index of refraction n(𝐫)=1-δ(𝐫)+iβ(𝐫). Phase contrast capitalizes on the fact that for hard x-rays, the real-valued decrement δ is several orders of magnitude higher in soft biological tissues than β, which accounts for absorption (Nugent, 2010) and that small contrast levels can be reconstructed by propagation imaging even at low fluence (Jahn et al., 2017). Contrast is formed by transformation of the phase shifts into measurable intensity variations by self-interference of the exit wave during free-space propagation between sample and detector (Paganin and Nugent, 1998; Cloetens et al., 1999). However, problems with phase retrieval, phase wrapping, insufficient coherence, or on the contrary strong phase gradients can render its application challenging. For lung imaging it has already been demonstrated as an advantageous imaging technique up to macroscopic scales (Parsons et al., 2008; Stahr et al., 2016; Morgan et al., 2020), including live animal models of respiratory diseases. But in contrast to other full-field phase contrast techniques, e.g. based on grating interferometry or analyzer crystals, it can also reach a resolution below optical microscopy (Khimchenko et al., 2018). In this work we exploit both the high resolution capability of PC-CT and the fact that its phase sensitivity is high enough to probe the small electron density variations of unstained tissue, embedded in paraffin, ethanol, or even aqueous buffer (Töpperwien et al., 2019). However, this requires careful optimization of photon energy, illumination function, and phase retrieval algorithms, as detailed further below.\nThe 3D virtual pathohistology approach for Covid-19 presented here was realized by implementing a novel multi-scale phase contrast x-ray tomography concept, with dedicated x-ray optics and instrumentation. Overview and regions-of-interest (ROI) scans were recorded on the same paraffin-embedded sample, covering a maximum tissue cross section of 8 mm by stitching different tomograms, and with a minimum voxel size of 167 nm in certain ROIs. Scale-bridging and dynamic ROI selection in close spatial and temporal proximity was implemented with dedicated instrumentation the GINIX endstation of the beamline P10/PETRA III (DESY, Hamburg) (Salditt et al., 2015). Specifically, we combined two optical geometries, which has only been realized at different synchrotron beamlines before: (i) Parallel beam tomography, covering a large field of view (FOV), with a pixel size of 650 nm. In this setting, a volumetric throughput on the order of 107μm/3s was achieved, while maintaining the ability to segment isolated cells in unstained tissue. (ii) Cone beam geometry for recording of highly magnified holograms, based on advanced x-ray waveguide optics, providing mode filtering, that is, enhanced spatial coherence and smooth wavefronts. Based on the geometrical magnification, the effective pixel size can be adjusted in the range 10 nm–300 nm. The two imaging schemes are shown schematically in Figure 1c and d, respectively. Further, using this particular optics, together with appropriate choices of photon energy and geometric parameters, we can reach extremely small Fresnel numbers F of the deeply holographic regime, well below the typical range exploited at other nano-tomography instruments. This offers the advantage of highest phase sensitivity sufficient to even probe the small electron density variations of unstained tissue, at relatively low dose (Hagemann and Salditt, 2018). To exploit this sensitivity, we use advanced phase retrieval methods including non-linear generalizations of the CTF-method (Cloetens et al., 1999) based on Tikhonov regularization (Lohse et al., 2020).\nFigure 1. Multi-scale x-ray tomography setup.\n(a) HE stain of a tissue micro-array paraffin block with samples of all six patients who succumbed to Covid-19. (b) Schematic representation of the sample preparation and mounting. In a first step biopsy punches containing the entire individual lung tissues are transferred onto a holder for the parallel-beam local tomography acquisition, followed by a further reduction in size to a 1 mm biopsy punch, for cone-beam tomographic recordings. (c) Configuration for parallel-beam tomography. (d) Configuration for cone-beam holo-tomography."}
LitCovid-PD-CLO
{"project":"LitCovid-PD-CLO","denotations":[{"id":"T13","span":{"begin":188,"end":193},"obj":"http://purl.obolibrary.org/obo/UBERON_0003103"},{"id":"T14","span":{"begin":261,"end":265},"obj":"http://purl.obolibrary.org/obo/UBERON_0002048"},{"id":"T15","span":{"begin":261,"end":265},"obj":"http://www.ebi.ac.uk/efo/EFO_0000934"},{"id":"T16","span":{"begin":334,"end":339},"obj":"http://www.ebi.ac.uk/efo/EFO_0000965"},{"id":"T17","span":{"begin":542,"end":546},"obj":"http://purl.obolibrary.org/obo/UBERON_0002048"},{"id":"T18","span":{"begin":542,"end":546},"obj":"http://www.ebi.ac.uk/efo/EFO_0000934"},{"id":"T19","span":{"begin":921,"end":928},"obj":"http://purl.obolibrary.org/obo/NCBITaxon_10239"},{"id":"T20","span":{"begin":1113,"end":1122},"obj":"http://purl.obolibrary.org/obo/UBERON_0000158"},{"id":"T21","span":{"begin":1377,"end":1378},"obj":"http://purl.obolibrary.org/obo/CLO_0001020"},{"id":"T22","span":{"begin":1379,"end":1384},"obj":"http://purl.obolibrary.org/obo/NCBITaxon_10239"},{"id":"T23","span":{"begin":1442,"end":1447},"obj":"http://www.ebi.ac.uk/efo/EFO_0000934"},{"id":"T24","span":{"begin":1449,"end":1450},"obj":"http://purl.obolibrary.org/obo/CLO_0001020"},{"id":"T25","span":{"begin":1475,"end":1481},"obj":"http://purl.obolibrary.org/obo/UBERON_0000055"},{"id":"T26","span":{"begin":1589,"end":1594},"obj":"http://www.ebi.ac.uk/efo/EFO_0000934"},{"id":"T27","span":{"begin":1627,"end":1628},"obj":"http://purl.obolibrary.org/obo/CLO_0001020"},{"id":"T28","span":{"begin":1828,"end":1832},"obj":"http://purl.obolibrary.org/obo/UBERON_0002048"},{"id":"T29","span":{"begin":1828,"end":1832},"obj":"http://www.ebi.ac.uk/efo/EFO_0000934"},{"id":"T30","span":{"begin":1861,"end":1866},"obj":"http://purl.obolibrary.org/obo/NCBITaxon_10239"},{"id":"T31","span":{"begin":1947,"end":1952},"obj":"http://www.ebi.ac.uk/efo/EFO_0000934"},{"id":"T32","span":{"begin":1954,"end":1968},"obj":"http://www.ebi.ac.uk/efo/EFO_0000881"},{"id":"T33","span":{"begin":2067,"end":2068},"obj":"http://purl.obolibrary.org/obo/CLO_0001020"},{"id":"T34","span":{"begin":2251,"end":2255},"obj":"http://purl.obolibrary.org/obo/UBERON_0002048"},{"id":"T35","span":{"begin":2251,"end":2255},"obj":"http://www.ebi.ac.uk/efo/EFO_0000934"},{"id":"T36","span":{"begin":2488,"end":2489},"obj":"http://purl.obolibrary.org/obo/CLO_0001020"},{"id":"T37","span":{"begin":2725,"end":2726},"obj":"http://purl.obolibrary.org/obo/CLO_0001020"},{"id":"T38","span":{"begin":2966,"end":2970},"obj":"http://purl.obolibrary.org/obo/OGG_0000000002"},{"id":"T39","span":{"begin":3011,"end":3014},"obj":"http://purl.obolibrary.org/obo/CLO_0001755"},{"id":"T40","span":{"begin":3064,"end":3077},"obj":"http://purl.obolibrary.org/obo/UBERON_0001981"},{"id":"T41","span":{"begin":3064,"end":3077},"obj":"http://www.ebi.ac.uk/efo/EFO_0000817"},{"id":"T42","span":{"begin":3126,"end":3127},"obj":"http://purl.obolibrary.org/obo/CLO_0001020"},{"id":"T43","span":{"begin":3333,"end":3337},"obj":"http://purl.obolibrary.org/obo/UBERON_0002048"},{"id":"T44","span":{"begin":3333,"end":3337},"obj":"http://www.ebi.ac.uk/efo/EFO_0000934"},{"id":"T45","span":{"begin":3480,"end":3488},"obj":"http://purl.obolibrary.org/obo/UBERON_0000158"},{"id":"T46","span":{"begin":3586,"end":3592},"obj":"http://purl.obolibrary.org/obo/CL_0000084"},{"id":"T47","span":{"begin":3848,"end":3849},"obj":"http://purl.obolibrary.org/obo/CLO_0001020"},{"id":"T48","span":{"begin":4004,"end":4008},"obj":"http://purl.obolibrary.org/obo/CLO_0001185"},{"id":"T49","span":{"begin":4029,"end":4033},"obj":"http://purl.obolibrary.org/obo/CLO_0001185"},{"id":"T50","span":{"begin":4096,"end":4102},"obj":"http://purl.obolibrary.org/obo/BFO_0000030"},{"id":"T51","span":{"begin":4922,"end":4926},"obj":"http://purl.obolibrary.org/obo/UBERON_0002048"},{"id":"T52","span":{"begin":4922,"end":4926},"obj":"http://www.ebi.ac.uk/efo/EFO_0000934"},{"id":"T53","span":{"begin":4938,"end":4941},"obj":"http://purl.obolibrary.org/obo/CLO_0051582"},{"id":"T54","span":{"begin":5110,"end":5116},"obj":"http://purl.obolibrary.org/obo/NCBITaxon_33208"},{"id":"T55","span":{"begin":5179,"end":5184},"obj":"http://purl.obolibrary.org/obo/UBERON_0007688"},{"id":"T56","span":{"begin":5289,"end":5290},"obj":"http://purl.obolibrary.org/obo/CLO_0001020"},{"id":"T57","span":{"begin":5347,"end":5351},"obj":"http://purl.obolibrary.org/obo/CLO_0001185"},{"id":"T58","span":{"begin":5867,"end":5868},"obj":"http://purl.obolibrary.org/obo/CLO_0001020"},{"id":"T59","span":{"begin":5960,"end":5975},"obj":"http://purl.obolibrary.org/obo/OBI_0000968"},{"id":"T60","span":{"begin":6083,"end":6084},"obj":"http://purl.obolibrary.org/obo/CLO_0001020"},{"id":"T61","span":{"begin":6165,"end":6166},"obj":"http://purl.obolibrary.org/obo/CLO_0001020"},{"id":"T62","span":{"begin":6325,"end":6340},"obj":"http://purl.obolibrary.org/obo/OBI_0000968"},{"id":"T63","span":{"begin":6488,"end":6491},"obj":"http://purl.obolibrary.org/obo/CLO_0051582"},{"id":"T64","span":{"begin":6593,"end":6594},"obj":"http://purl.obolibrary.org/obo/CLO_0001020"},{"id":"T65","span":{"begin":6601,"end":6606},"obj":"http://purl.obolibrary.org/obo/UBERON_0007688"},{"id":"T66","span":{"begin":6627,"end":6628},"obj":"http://purl.obolibrary.org/obo/CLO_0001020"},{"id":"T67","span":{"begin":6668,"end":6669},"obj":"http://purl.obolibrary.org/obo/CLO_0001020"},{"id":"T68","span":{"begin":6781,"end":6786},"obj":"http://purl.obolibrary.org/obo/GO_0005623"},{"id":"T69","span":{"begin":7323,"end":7332},"obj":"http://www.ebi.ac.uk/efo/EFO_0000876"},{"id":"T70","span":{"begin":7455,"end":7466},"obj":"http://purl.obolibrary.org/obo/OBI_0000968"},{"id":"T71","span":{"begin":7653,"end":7657},"obj":"http://purl.obolibrary.org/obo/CLO_0001185"},{"id":"T72","span":{"begin":7911,"end":7912},"obj":"http://purl.obolibrary.org/obo/CLO_0001020"},{"id":"T73","span":{"begin":7926,"end":7927},"obj":"http://purl.obolibrary.org/obo/CLO_0001020"},{"id":"T74","span":{"begin":8023,"end":8024},"obj":"http://purl.obolibrary.org/obo/CLO_0001021"},{"id":"T75","span":{"begin":8094,"end":8095},"obj":"http://purl.obolibrary.org/obo/CLO_0001020"},{"id":"T76","span":{"begin":8155,"end":8159},"obj":"http://purl.obolibrary.org/obo/UBERON_0002048"},{"id":"T77","span":{"begin":8155,"end":8159},"obj":"http://www.ebi.ac.uk/efo/EFO_0000934"},{"id":"T78","span":{"begin":8189,"end":8190},"obj":"http://purl.obolibrary.org/obo/CLO_0001020"},{"id":"T79","span":{"begin":8262,"end":8263},"obj":"http://purl.obolibrary.org/obo/CLO_0001020"},{"id":"T80","span":{"begin":8293,"end":8294},"obj":"http://purl.obolibrary.org/obo/CLO_0001020"}],"text":"Introduction\nSevere progression of the 2019 coronavirus disease (Covid-19) is frequently accompanied by the clinical acute respiratory distress syndrome (ARDS) and respiratory failure, an organ manifestation responsible for the majority of Covid-19 fatalities. Lung injury associated with ARDS can be readily detected by radiographic chest imaging and clinical computed tomography (CT), which have assisted the diagnosis and management of Covid-19 patients (Lee et al., 2020; Shi et al., 2020; Chung et al., 2020). Here, so-called peripheral lung ground-glass opacities are the main radiological hallmark of ARDS, and can be linked to the histological observation of diffuse alveolar damage (DAD) with edema, hemorrhage, and intraalveolar fibrin deposition (Ackermann et al., 2020). These findings have also been reported for infections by Middle East respiratory syndrome coronavirus (MERS-CoV), SARS-CoV, and influenza viruses. Distinctive features of pulmonary involvement of Covid-19 include severe endothelial injury associated with the presence of intracellular virions and inflammation, disrupted cellular membranes, as well as widespread thrombosis with microangiopathy. As reported in Ackermann et al., 2020, alveolar capillary microthrombi were found to be nine times as prevalent in patients with Covid-19 as in patients with the also very aggressive H1N1 influenza A virus, also referred to as swine flu. Importantly, in Covid-19 lungs, a specific variant of new vessel growth - intussusceptive angiogenesis - was significantly more prevalent, that is, 2.7 times as high as in lungs of patients with H1N1 influenza A.\nHistomorphological assessment of formalin-fixed, paraffin-embedded (FFPE) tissue stained with haematoxylin and eosin still represents the gold standard in histological diagnostics of non-neoplastic lung diseases, including DAD and virus induced pneumonia. In order to unravel the corresponding pathophysiology of the lungs, digitalization, visualization and quantification of the morphological changes associated with Covid-19 represent a key challenge, and require both high resolution and the capability to screen larger volumes. For this reason, imaging the intricate three-dimensional (3D) tissue architecture of the lung and its pathological alterations on multiple length scales calls for 3D extensions of well-established histology techniques.\nIn this work, we want to demonstrate the potential of propagation-based phase contrast x-ray tomography as a tool for virtual 3D histology in general, and in particular for the histopathology of Covid-19. Our work is based on the assertion that integration of 3D morphological information with well-established histology techniques can provide a substantial asset for unraveling the pathophysiology of SARS-Cov-2 infections. To this end, we have collected x-ray tomography data from the same autopsies, which were previously studied by immunohistochemical analysis and measurements of gene expression (Ackermann et al., 2020). We ask in particular, whether DAD and the morphology of blood vessels can be visualized and quantified in 3D. This is a timely scientific question in Covid-19 research, especially in view of increased intussusceptive angiogenesis reported in Ackermann et al., 2020. Here we present first results obtained from the postmortem lung samples of six patients who succumbed from Covid-19. We exemplify the capability of this approach by 3D visualization of the DAD with hyaline membrane formation, by mapping the 3D distribution and density of angiocentric inflammation (perivascular T-cell infiltration), and by providing histograms of characteristic distances from the tissue interior to the closest air compartment.\nIn contrast to conventional histology based on thin sections, propagation-based x-ray phase-contrast tomography (PC-CT) offers a full 3D visualization with isotropic resolution and without destructive slicing of the specimen (Ding et al., 2019; Dejea et al., 2019; Saccomano et al., 2018; Töpperwien et al., 2018; Khimchenko et al., 2016). The interaction of x-rays with the object is described by the continuous complex-valued index of refraction n(𝐫)=1-δ(𝐫)+iβ(𝐫). Phase contrast capitalizes on the fact that for hard x-rays, the real-valued decrement δ is several orders of magnitude higher in soft biological tissues than β, which accounts for absorption (Nugent, 2010) and that small contrast levels can be reconstructed by propagation imaging even at low fluence (Jahn et al., 2017). Contrast is formed by transformation of the phase shifts into measurable intensity variations by self-interference of the exit wave during free-space propagation between sample and detector (Paganin and Nugent, 1998; Cloetens et al., 1999). However, problems with phase retrieval, phase wrapping, insufficient coherence, or on the contrary strong phase gradients can render its application challenging. For lung imaging it has already been demonstrated as an advantageous imaging technique up to macroscopic scales (Parsons et al., 2008; Stahr et al., 2016; Morgan et al., 2020), including live animal models of respiratory diseases. But in contrast to other full-field phase contrast techniques, e.g. based on grating interferometry or analyzer crystals, it can also reach a resolution below optical microscopy (Khimchenko et al., 2018). In this work we exploit both the high resolution capability of PC-CT and the fact that its phase sensitivity is high enough to probe the small electron density variations of unstained tissue, embedded in paraffin, ethanol, or even aqueous buffer (Töpperwien et al., 2019). However, this requires careful optimization of photon energy, illumination function, and phase retrieval algorithms, as detailed further below.\nThe 3D virtual pathohistology approach for Covid-19 presented here was realized by implementing a novel multi-scale phase contrast x-ray tomography concept, with dedicated x-ray optics and instrumentation. Overview and regions-of-interest (ROI) scans were recorded on the same paraffin-embedded sample, covering a maximum tissue cross section of 8 mm by stitching different tomograms, and with a minimum voxel size of 167 nm in certain ROIs. Scale-bridging and dynamic ROI selection in close spatial and temporal proximity was implemented with dedicated instrumentation the GINIX endstation of the beamline P10/PETRA III (DESY, Hamburg) (Salditt et al., 2015). Specifically, we combined two optical geometries, which has only been realized at different synchrotron beamlines before: (i) Parallel beam tomography, covering a large field of view (FOV), with a pixel size of 650 nm. In this setting, a volumetric throughput on the order of 107μm/3s was achieved, while maintaining the ability to segment isolated cells in unstained tissue. (ii) Cone beam geometry for recording of highly magnified holograms, based on advanced x-ray waveguide optics, providing mode filtering, that is, enhanced spatial coherence and smooth wavefronts. Based on the geometrical magnification, the effective pixel size can be adjusted in the range 10 nm–300 nm. The two imaging schemes are shown schematically in Figure 1c and d, respectively. Further, using this particular optics, together with appropriate choices of photon energy and geometric parameters, we can reach extremely small Fresnel numbers F of the deeply holographic regime, well below the typical range exploited at other nano-tomography instruments. This offers the advantage of highest phase sensitivity sufficient to even probe the small electron density variations of unstained tissue, at relatively low dose (Hagemann and Salditt, 2018). To exploit this sensitivity, we use advanced phase retrieval methods including non-linear generalizations of the CTF-method (Cloetens et al., 1999) based on Tikhonov regularization (Lohse et al., 2020).\nFigure 1. Multi-scale x-ray tomography setup.\n(a) HE stain of a tissue micro-array paraffin block with samples of all six patients who succumbed to Covid-19. (b) Schematic representation of the sample preparation and mounting. In a first step biopsy punches containing the entire individual lung tissues are transferred onto a holder for the parallel-beam local tomography acquisition, followed by a further reduction in size to a 1 mm biopsy punch, for cone-beam tomographic recordings. (c) Configuration for parallel-beam tomography. (d) Configuration for cone-beam holo-tomography."}
LitCovid-PD-GO-BP
{"project":"LitCovid-PD-GO-BP","denotations":[{"id":"T4","span":{"begin":1080,"end":1092},"obj":"http://purl.obolibrary.org/obo/GO_0006954"},{"id":"T5","span":{"begin":1482,"end":1488},"obj":"http://purl.obolibrary.org/obo/GO_0040007"},{"id":"T6","span":{"begin":1491,"end":1519},"obj":"http://purl.obolibrary.org/obo/GO_0002041"},{"id":"T7","span":{"begin":1507,"end":1519},"obj":"http://purl.obolibrary.org/obo/GO_0001525"},{"id":"T8","span":{"begin":2966,"end":2981},"obj":"http://purl.obolibrary.org/obo/GO_0010467"},{"id":"T9","span":{"begin":3209,"end":3237},"obj":"http://purl.obolibrary.org/obo/GO_0002041"},{"id":"T10","span":{"begin":3225,"end":3237},"obj":"http://purl.obolibrary.org/obo/GO_0001525"},{"id":"T11","span":{"begin":3489,"end":3498},"obj":"http://purl.obolibrary.org/obo/GO_0009058"},{"id":"T12","span":{"begin":3559,"end":3571},"obj":"http://purl.obolibrary.org/obo/GO_0006954"}],"text":"Introduction\nSevere progression of the 2019 coronavirus disease (Covid-19) is frequently accompanied by the clinical acute respiratory distress syndrome (ARDS) and respiratory failure, an organ manifestation responsible for the majority of Covid-19 fatalities. Lung injury associated with ARDS can be readily detected by radiographic chest imaging and clinical computed tomography (CT), which have assisted the diagnosis and management of Covid-19 patients (Lee et al., 2020; Shi et al., 2020; Chung et al., 2020). Here, so-called peripheral lung ground-glass opacities are the main radiological hallmark of ARDS, and can be linked to the histological observation of diffuse alveolar damage (DAD) with edema, hemorrhage, and intraalveolar fibrin deposition (Ackermann et al., 2020). These findings have also been reported for infections by Middle East respiratory syndrome coronavirus (MERS-CoV), SARS-CoV, and influenza viruses. Distinctive features of pulmonary involvement of Covid-19 include severe endothelial injury associated with the presence of intracellular virions and inflammation, disrupted cellular membranes, as well as widespread thrombosis with microangiopathy. As reported in Ackermann et al., 2020, alveolar capillary microthrombi were found to be nine times as prevalent in patients with Covid-19 as in patients with the also very aggressive H1N1 influenza A virus, also referred to as swine flu. Importantly, in Covid-19 lungs, a specific variant of new vessel growth - intussusceptive angiogenesis - was significantly more prevalent, that is, 2.7 times as high as in lungs of patients with H1N1 influenza A.\nHistomorphological assessment of formalin-fixed, paraffin-embedded (FFPE) tissue stained with haematoxylin and eosin still represents the gold standard in histological diagnostics of non-neoplastic lung diseases, including DAD and virus induced pneumonia. In order to unravel the corresponding pathophysiology of the lungs, digitalization, visualization and quantification of the morphological changes associated with Covid-19 represent a key challenge, and require both high resolution and the capability to screen larger volumes. For this reason, imaging the intricate three-dimensional (3D) tissue architecture of the lung and its pathological alterations on multiple length scales calls for 3D extensions of well-established histology techniques.\nIn this work, we want to demonstrate the potential of propagation-based phase contrast x-ray tomography as a tool for virtual 3D histology in general, and in particular for the histopathology of Covid-19. Our work is based on the assertion that integration of 3D morphological information with well-established histology techniques can provide a substantial asset for unraveling the pathophysiology of SARS-Cov-2 infections. To this end, we have collected x-ray tomography data from the same autopsies, which were previously studied by immunohistochemical analysis and measurements of gene expression (Ackermann et al., 2020). We ask in particular, whether DAD and the morphology of blood vessels can be visualized and quantified in 3D. This is a timely scientific question in Covid-19 research, especially in view of increased intussusceptive angiogenesis reported in Ackermann et al., 2020. Here we present first results obtained from the postmortem lung samples of six patients who succumbed from Covid-19. We exemplify the capability of this approach by 3D visualization of the DAD with hyaline membrane formation, by mapping the 3D distribution and density of angiocentric inflammation (perivascular T-cell infiltration), and by providing histograms of characteristic distances from the tissue interior to the closest air compartment.\nIn contrast to conventional histology based on thin sections, propagation-based x-ray phase-contrast tomography (PC-CT) offers a full 3D visualization with isotropic resolution and without destructive slicing of the specimen (Ding et al., 2019; Dejea et al., 2019; Saccomano et al., 2018; Töpperwien et al., 2018; Khimchenko et al., 2016). The interaction of x-rays with the object is described by the continuous complex-valued index of refraction n(𝐫)=1-δ(𝐫)+iβ(𝐫). Phase contrast capitalizes on the fact that for hard x-rays, the real-valued decrement δ is several orders of magnitude higher in soft biological tissues than β, which accounts for absorption (Nugent, 2010) and that small contrast levels can be reconstructed by propagation imaging even at low fluence (Jahn et al., 2017). Contrast is formed by transformation of the phase shifts into measurable intensity variations by self-interference of the exit wave during free-space propagation between sample and detector (Paganin and Nugent, 1998; Cloetens et al., 1999). However, problems with phase retrieval, phase wrapping, insufficient coherence, or on the contrary strong phase gradients can render its application challenging. For lung imaging it has already been demonstrated as an advantageous imaging technique up to macroscopic scales (Parsons et al., 2008; Stahr et al., 2016; Morgan et al., 2020), including live animal models of respiratory diseases. But in contrast to other full-field phase contrast techniques, e.g. based on grating interferometry or analyzer crystals, it can also reach a resolution below optical microscopy (Khimchenko et al., 2018). In this work we exploit both the high resolution capability of PC-CT and the fact that its phase sensitivity is high enough to probe the small electron density variations of unstained tissue, embedded in paraffin, ethanol, or even aqueous buffer (Töpperwien et al., 2019). However, this requires careful optimization of photon energy, illumination function, and phase retrieval algorithms, as detailed further below.\nThe 3D virtual pathohistology approach for Covid-19 presented here was realized by implementing a novel multi-scale phase contrast x-ray tomography concept, with dedicated x-ray optics and instrumentation. Overview and regions-of-interest (ROI) scans were recorded on the same paraffin-embedded sample, covering a maximum tissue cross section of 8 mm by stitching different tomograms, and with a minimum voxel size of 167 nm in certain ROIs. Scale-bridging and dynamic ROI selection in close spatial and temporal proximity was implemented with dedicated instrumentation the GINIX endstation of the beamline P10/PETRA III (DESY, Hamburg) (Salditt et al., 2015). Specifically, we combined two optical geometries, which has only been realized at different synchrotron beamlines before: (i) Parallel beam tomography, covering a large field of view (FOV), with a pixel size of 650 nm. In this setting, a volumetric throughput on the order of 107μm/3s was achieved, while maintaining the ability to segment isolated cells in unstained tissue. (ii) Cone beam geometry for recording of highly magnified holograms, based on advanced x-ray waveguide optics, providing mode filtering, that is, enhanced spatial coherence and smooth wavefronts. Based on the geometrical magnification, the effective pixel size can be adjusted in the range 10 nm–300 nm. The two imaging schemes are shown schematically in Figure 1c and d, respectively. Further, using this particular optics, together with appropriate choices of photon energy and geometric parameters, we can reach extremely small Fresnel numbers F of the deeply holographic regime, well below the typical range exploited at other nano-tomography instruments. This offers the advantage of highest phase sensitivity sufficient to even probe the small electron density variations of unstained tissue, at relatively low dose (Hagemann and Salditt, 2018). To exploit this sensitivity, we use advanced phase retrieval methods including non-linear generalizations of the CTF-method (Cloetens et al., 1999) based on Tikhonov regularization (Lohse et al., 2020).\nFigure 1. Multi-scale x-ray tomography setup.\n(a) HE stain of a tissue micro-array paraffin block with samples of all six patients who succumbed to Covid-19. (b) Schematic representation of the sample preparation and mounting. In a first step biopsy punches containing the entire individual lung tissues are transferred onto a holder for the parallel-beam local tomography acquisition, followed by a further reduction in size to a 1 mm biopsy punch, for cone-beam tomographic recordings. (c) Configuration for parallel-beam tomography. (d) Configuration for cone-beam holo-tomography."}
LitCovid-sentences
{"project":"LitCovid-sentences","denotations":[{"id":"T8","span":{"begin":0,"end":12},"obj":"Sentence"},{"id":"T9","span":{"begin":13,"end":260},"obj":"Sentence"},{"id":"T10","span":{"begin":261,"end":514},"obj":"Sentence"},{"id":"T11","span":{"begin":515,"end":782},"obj":"Sentence"},{"id":"T12","span":{"begin":783,"end":929},"obj":"Sentence"},{"id":"T13","span":{"begin":930,"end":1178},"obj":"Sentence"},{"id":"T14","span":{"begin":1179,"end":1416},"obj":"Sentence"},{"id":"T15","span":{"begin":1417,"end":1629},"obj":"Sentence"},{"id":"T16","span":{"begin":1630,"end":1885},"obj":"Sentence"},{"id":"T17","span":{"begin":1886,"end":2161},"obj":"Sentence"},{"id":"T18","span":{"begin":2162,"end":2380},"obj":"Sentence"},{"id":"T19","span":{"begin":2381,"end":2585},"obj":"Sentence"},{"id":"T20","span":{"begin":2586,"end":2805},"obj":"Sentence"},{"id":"T21","span":{"begin":2806,"end":3007},"obj":"Sentence"},{"id":"T22","span":{"begin":3008,"end":3117},"obj":"Sentence"},{"id":"T23","span":{"begin":3118,"end":3273},"obj":"Sentence"},{"id":"T24","span":{"begin":3274,"end":3390},"obj":"Sentence"},{"id":"T25","span":{"begin":3391,"end":3720},"obj":"Sentence"},{"id":"T26","span":{"begin":3721,"end":4060},"obj":"Sentence"},{"id":"T27","span":{"begin":4061,"end":4191},"obj":"Sentence"},{"id":"T28","span":{"begin":4192,"end":4514},"obj":"Sentence"},{"id":"T29","span":{"begin":4515,"end":4755},"obj":"Sentence"},{"id":"T30","span":{"begin":4756,"end":4917},"obj":"Sentence"},{"id":"T31","span":{"begin":4918,"end":5148},"obj":"Sentence"},{"id":"T32","span":{"begin":5149,"end":5353},"obj":"Sentence"},{"id":"T33","span":{"begin":5354,"end":5626},"obj":"Sentence"},{"id":"T34","span":{"begin":5627,"end":5770},"obj":"Sentence"},{"id":"T35","span":{"begin":5771,"end":5976},"obj":"Sentence"},{"id":"T36","span":{"begin":5977,"end":6212},"obj":"Sentence"},{"id":"T37","span":{"begin":6213,"end":6431},"obj":"Sentence"},{"id":"T38","span":{"begin":6432,"end":6650},"obj":"Sentence"},{"id":"T39","span":{"begin":6651,"end":7003},"obj":"Sentence"},{"id":"T40","span":{"begin":7004,"end":7111},"obj":"Sentence"},{"id":"T41","span":{"begin":7112,"end":7193},"obj":"Sentence"},{"id":"T42","span":{"begin":7194,"end":7467},"obj":"Sentence"},{"id":"T43","span":{"begin":7468,"end":7659},"obj":"Sentence"},{"id":"T44","span":{"begin":7660,"end":7862},"obj":"Sentence"},{"id":"T45","span":{"begin":7863,"end":7872},"obj":"Sentence"},{"id":"T46","span":{"begin":7874,"end":7909},"obj":"Sentence"},{"id":"T47","span":{"begin":7910,"end":8090},"obj":"Sentence"},{"id":"T48","span":{"begin":8091,"end":8448},"obj":"Sentence"}],"namespaces":[{"prefix":"_base","uri":"http://pubannotation.org/ontology/tao.owl#"}],"text":"Introduction\nSevere progression of the 2019 coronavirus disease (Covid-19) is frequently accompanied by the clinical acute respiratory distress syndrome (ARDS) and respiratory failure, an organ manifestation responsible for the majority of Covid-19 fatalities. Lung injury associated with ARDS can be readily detected by radiographic chest imaging and clinical computed tomography (CT), which have assisted the diagnosis and management of Covid-19 patients (Lee et al., 2020; Shi et al., 2020; Chung et al., 2020). Here, so-called peripheral lung ground-glass opacities are the main radiological hallmark of ARDS, and can be linked to the histological observation of diffuse alveolar damage (DAD) with edema, hemorrhage, and intraalveolar fibrin deposition (Ackermann et al., 2020). These findings have also been reported for infections by Middle East respiratory syndrome coronavirus (MERS-CoV), SARS-CoV, and influenza viruses. Distinctive features of pulmonary involvement of Covid-19 include severe endothelial injury associated with the presence of intracellular virions and inflammation, disrupted cellular membranes, as well as widespread thrombosis with microangiopathy. As reported in Ackermann et al., 2020, alveolar capillary microthrombi were found to be nine times as prevalent in patients with Covid-19 as in patients with the also very aggressive H1N1 influenza A virus, also referred to as swine flu. Importantly, in Covid-19 lungs, a specific variant of new vessel growth - intussusceptive angiogenesis - was significantly more prevalent, that is, 2.7 times as high as in lungs of patients with H1N1 influenza A.\nHistomorphological assessment of formalin-fixed, paraffin-embedded (FFPE) tissue stained with haematoxylin and eosin still represents the gold standard in histological diagnostics of non-neoplastic lung diseases, including DAD and virus induced pneumonia. In order to unravel the corresponding pathophysiology of the lungs, digitalization, visualization and quantification of the morphological changes associated with Covid-19 represent a key challenge, and require both high resolution and the capability to screen larger volumes. For this reason, imaging the intricate three-dimensional (3D) tissue architecture of the lung and its pathological alterations on multiple length scales calls for 3D extensions of well-established histology techniques.\nIn this work, we want to demonstrate the potential of propagation-based phase contrast x-ray tomography as a tool for virtual 3D histology in general, and in particular for the histopathology of Covid-19. Our work is based on the assertion that integration of 3D morphological information with well-established histology techniques can provide a substantial asset for unraveling the pathophysiology of SARS-Cov-2 infections. To this end, we have collected x-ray tomography data from the same autopsies, which were previously studied by immunohistochemical analysis and measurements of gene expression (Ackermann et al., 2020). We ask in particular, whether DAD and the morphology of blood vessels can be visualized and quantified in 3D. This is a timely scientific question in Covid-19 research, especially in view of increased intussusceptive angiogenesis reported in Ackermann et al., 2020. Here we present first results obtained from the postmortem lung samples of six patients who succumbed from Covid-19. We exemplify the capability of this approach by 3D visualization of the DAD with hyaline membrane formation, by mapping the 3D distribution and density of angiocentric inflammation (perivascular T-cell infiltration), and by providing histograms of characteristic distances from the tissue interior to the closest air compartment.\nIn contrast to conventional histology based on thin sections, propagation-based x-ray phase-contrast tomography (PC-CT) offers a full 3D visualization with isotropic resolution and without destructive slicing of the specimen (Ding et al., 2019; Dejea et al., 2019; Saccomano et al., 2018; Töpperwien et al., 2018; Khimchenko et al., 2016). The interaction of x-rays with the object is described by the continuous complex-valued index of refraction n(𝐫)=1-δ(𝐫)+iβ(𝐫). Phase contrast capitalizes on the fact that for hard x-rays, the real-valued decrement δ is several orders of magnitude higher in soft biological tissues than β, which accounts for absorption (Nugent, 2010) and that small contrast levels can be reconstructed by propagation imaging even at low fluence (Jahn et al., 2017). Contrast is formed by transformation of the phase shifts into measurable intensity variations by self-interference of the exit wave during free-space propagation between sample and detector (Paganin and Nugent, 1998; Cloetens et al., 1999). However, problems with phase retrieval, phase wrapping, insufficient coherence, or on the contrary strong phase gradients can render its application challenging. For lung imaging it has already been demonstrated as an advantageous imaging technique up to macroscopic scales (Parsons et al., 2008; Stahr et al., 2016; Morgan et al., 2020), including live animal models of respiratory diseases. But in contrast to other full-field phase contrast techniques, e.g. based on grating interferometry or analyzer crystals, it can also reach a resolution below optical microscopy (Khimchenko et al., 2018). In this work we exploit both the high resolution capability of PC-CT and the fact that its phase sensitivity is high enough to probe the small electron density variations of unstained tissue, embedded in paraffin, ethanol, or even aqueous buffer (Töpperwien et al., 2019). However, this requires careful optimization of photon energy, illumination function, and phase retrieval algorithms, as detailed further below.\nThe 3D virtual pathohistology approach for Covid-19 presented here was realized by implementing a novel multi-scale phase contrast x-ray tomography concept, with dedicated x-ray optics and instrumentation. Overview and regions-of-interest (ROI) scans were recorded on the same paraffin-embedded sample, covering a maximum tissue cross section of 8 mm by stitching different tomograms, and with a minimum voxel size of 167 nm in certain ROIs. Scale-bridging and dynamic ROI selection in close spatial and temporal proximity was implemented with dedicated instrumentation the GINIX endstation of the beamline P10/PETRA III (DESY, Hamburg) (Salditt et al., 2015). Specifically, we combined two optical geometries, which has only been realized at different synchrotron beamlines before: (i) Parallel beam tomography, covering a large field of view (FOV), with a pixel size of 650 nm. In this setting, a volumetric throughput on the order of 107μm/3s was achieved, while maintaining the ability to segment isolated cells in unstained tissue. (ii) Cone beam geometry for recording of highly magnified holograms, based on advanced x-ray waveguide optics, providing mode filtering, that is, enhanced spatial coherence and smooth wavefronts. Based on the geometrical magnification, the effective pixel size can be adjusted in the range 10 nm–300 nm. The two imaging schemes are shown schematically in Figure 1c and d, respectively. Further, using this particular optics, together with appropriate choices of photon energy and geometric parameters, we can reach extremely small Fresnel numbers F of the deeply holographic regime, well below the typical range exploited at other nano-tomography instruments. This offers the advantage of highest phase sensitivity sufficient to even probe the small electron density variations of unstained tissue, at relatively low dose (Hagemann and Salditt, 2018). To exploit this sensitivity, we use advanced phase retrieval methods including non-linear generalizations of the CTF-method (Cloetens et al., 1999) based on Tikhonov regularization (Lohse et al., 2020).\nFigure 1. Multi-scale x-ray tomography setup.\n(a) HE stain of a tissue micro-array paraffin block with samples of all six patients who succumbed to Covid-19. (b) Schematic representation of the sample preparation and mounting. In a first step biopsy punches containing the entire individual lung tissues are transferred onto a holder for the parallel-beam local tomography acquisition, followed by a further reduction in size to a 1 mm biopsy punch, for cone-beam tomographic recordings. (c) Configuration for parallel-beam tomography. (d) Configuration for cone-beam holo-tomography."}
LitCovid-PD-HP
{"project":"LitCovid-PD-HP","denotations":[{"id":"T3","span":{"begin":123,"end":143},"obj":"Phenotype"},{"id":"T4","span":{"begin":164,"end":183},"obj":"Phenotype"},{"id":"T5","span":{"begin":667,"end":690},"obj":"Phenotype"},{"id":"T6","span":{"begin":692,"end":695},"obj":"Phenotype"},{"id":"T7","span":{"begin":702,"end":707},"obj":"Phenotype"},{"id":"T8","span":{"begin":1491,"end":1506},"obj":"Phenotype"},{"id":"T9","span":{"begin":1828,"end":1841},"obj":"Phenotype"},{"id":"T10","span":{"begin":1853,"end":1856},"obj":"Phenotype"},{"id":"T11","span":{"begin":1875,"end":1884},"obj":"Phenotype"},{"id":"T12","span":{"begin":3038,"end":3041},"obj":"Phenotype"},{"id":"T13","span":{"begin":3209,"end":3224},"obj":"Phenotype"},{"id":"T14","span":{"begin":3463,"end":3466},"obj":"Phenotype"}],"attributes":[{"id":"A3","pred":"hp_id","subj":"T3","obj":"http://purl.obolibrary.org/obo/HP_0002098"},{"id":"A4","pred":"hp_id","subj":"T4","obj":"http://purl.obolibrary.org/obo/HP_0002878"},{"id":"A5","pred":"hp_id","subj":"T5","obj":"http://purl.obolibrary.org/obo/HP_0033006"},{"id":"A6","pred":"hp_id","subj":"T6","obj":"http://purl.obolibrary.org/obo/HP_0033006"},{"id":"A7","pred":"hp_id","subj":"T7","obj":"http://purl.obolibrary.org/obo/HP_0000969"},{"id":"A8","pred":"hp_id","subj":"T8","obj":"http://purl.obolibrary.org/obo/HP_0002576"},{"id":"A9","pred":"hp_id","subj":"T9","obj":"http://purl.obolibrary.org/obo/HP_0002088"},{"id":"A10","pred":"hp_id","subj":"T10","obj":"http://purl.obolibrary.org/obo/HP_0033006"},{"id":"A11","pred":"hp_id","subj":"T11","obj":"http://purl.obolibrary.org/obo/HP_0002090"},{"id":"A12","pred":"hp_id","subj":"T12","obj":"http://purl.obolibrary.org/obo/HP_0033006"},{"id":"A13","pred":"hp_id","subj":"T13","obj":"http://purl.obolibrary.org/obo/HP_0002576"},{"id":"A14","pred":"hp_id","subj":"T14","obj":"http://purl.obolibrary.org/obo/HP_0033006"}],"text":"Introduction\nSevere progression of the 2019 coronavirus disease (Covid-19) is frequently accompanied by the clinical acute respiratory distress syndrome (ARDS) and respiratory failure, an organ manifestation responsible for the majority of Covid-19 fatalities. Lung injury associated with ARDS can be readily detected by radiographic chest imaging and clinical computed tomography (CT), which have assisted the diagnosis and management of Covid-19 patients (Lee et al., 2020; Shi et al., 2020; Chung et al., 2020). Here, so-called peripheral lung ground-glass opacities are the main radiological hallmark of ARDS, and can be linked to the histological observation of diffuse alveolar damage (DAD) with edema, hemorrhage, and intraalveolar fibrin deposition (Ackermann et al., 2020). These findings have also been reported for infections by Middle East respiratory syndrome coronavirus (MERS-CoV), SARS-CoV, and influenza viruses. Distinctive features of pulmonary involvement of Covid-19 include severe endothelial injury associated with the presence of intracellular virions and inflammation, disrupted cellular membranes, as well as widespread thrombosis with microangiopathy. As reported in Ackermann et al., 2020, alveolar capillary microthrombi were found to be nine times as prevalent in patients with Covid-19 as in patients with the also very aggressive H1N1 influenza A virus, also referred to as swine flu. Importantly, in Covid-19 lungs, a specific variant of new vessel growth - intussusceptive angiogenesis - was significantly more prevalent, that is, 2.7 times as high as in lungs of patients with H1N1 influenza A.\nHistomorphological assessment of formalin-fixed, paraffin-embedded (FFPE) tissue stained with haematoxylin and eosin still represents the gold standard in histological diagnostics of non-neoplastic lung diseases, including DAD and virus induced pneumonia. In order to unravel the corresponding pathophysiology of the lungs, digitalization, visualization and quantification of the morphological changes associated with Covid-19 represent a key challenge, and require both high resolution and the capability to screen larger volumes. For this reason, imaging the intricate three-dimensional (3D) tissue architecture of the lung and its pathological alterations on multiple length scales calls for 3D extensions of well-established histology techniques.\nIn this work, we want to demonstrate the potential of propagation-based phase contrast x-ray tomography as a tool for virtual 3D histology in general, and in particular for the histopathology of Covid-19. Our work is based on the assertion that integration of 3D morphological information with well-established histology techniques can provide a substantial asset for unraveling the pathophysiology of SARS-Cov-2 infections. To this end, we have collected x-ray tomography data from the same autopsies, which were previously studied by immunohistochemical analysis and measurements of gene expression (Ackermann et al., 2020). We ask in particular, whether DAD and the morphology of blood vessels can be visualized and quantified in 3D. This is a timely scientific question in Covid-19 research, especially in view of increased intussusceptive angiogenesis reported in Ackermann et al., 2020. Here we present first results obtained from the postmortem lung samples of six patients who succumbed from Covid-19. We exemplify the capability of this approach by 3D visualization of the DAD with hyaline membrane formation, by mapping the 3D distribution and density of angiocentric inflammation (perivascular T-cell infiltration), and by providing histograms of characteristic distances from the tissue interior to the closest air compartment.\nIn contrast to conventional histology based on thin sections, propagation-based x-ray phase-contrast tomography (PC-CT) offers a full 3D visualization with isotropic resolution and without destructive slicing of the specimen (Ding et al., 2019; Dejea et al., 2019; Saccomano et al., 2018; Töpperwien et al., 2018; Khimchenko et al., 2016). The interaction of x-rays with the object is described by the continuous complex-valued index of refraction n(𝐫)=1-δ(𝐫)+iβ(𝐫). Phase contrast capitalizes on the fact that for hard x-rays, the real-valued decrement δ is several orders of magnitude higher in soft biological tissues than β, which accounts for absorption (Nugent, 2010) and that small contrast levels can be reconstructed by propagation imaging even at low fluence (Jahn et al., 2017). Contrast is formed by transformation of the phase shifts into measurable intensity variations by self-interference of the exit wave during free-space propagation between sample and detector (Paganin and Nugent, 1998; Cloetens et al., 1999). However, problems with phase retrieval, phase wrapping, insufficient coherence, or on the contrary strong phase gradients can render its application challenging. For lung imaging it has already been demonstrated as an advantageous imaging technique up to macroscopic scales (Parsons et al., 2008; Stahr et al., 2016; Morgan et al., 2020), including live animal models of respiratory diseases. But in contrast to other full-field phase contrast techniques, e.g. based on grating interferometry or analyzer crystals, it can also reach a resolution below optical microscopy (Khimchenko et al., 2018). In this work we exploit both the high resolution capability of PC-CT and the fact that its phase sensitivity is high enough to probe the small electron density variations of unstained tissue, embedded in paraffin, ethanol, or even aqueous buffer (Töpperwien et al., 2019). However, this requires careful optimization of photon energy, illumination function, and phase retrieval algorithms, as detailed further below.\nThe 3D virtual pathohistology approach for Covid-19 presented here was realized by implementing a novel multi-scale phase contrast x-ray tomography concept, with dedicated x-ray optics and instrumentation. Overview and regions-of-interest (ROI) scans were recorded on the same paraffin-embedded sample, covering a maximum tissue cross section of 8 mm by stitching different tomograms, and with a minimum voxel size of 167 nm in certain ROIs. Scale-bridging and dynamic ROI selection in close spatial and temporal proximity was implemented with dedicated instrumentation the GINIX endstation of the beamline P10/PETRA III (DESY, Hamburg) (Salditt et al., 2015). Specifically, we combined two optical geometries, which has only been realized at different synchrotron beamlines before: (i) Parallel beam tomography, covering a large field of view (FOV), with a pixel size of 650 nm. In this setting, a volumetric throughput on the order of 107μm/3s was achieved, while maintaining the ability to segment isolated cells in unstained tissue. (ii) Cone beam geometry for recording of highly magnified holograms, based on advanced x-ray waveguide optics, providing mode filtering, that is, enhanced spatial coherence and smooth wavefronts. Based on the geometrical magnification, the effective pixel size can be adjusted in the range 10 nm–300 nm. The two imaging schemes are shown schematically in Figure 1c and d, respectively. Further, using this particular optics, together with appropriate choices of photon energy and geometric parameters, we can reach extremely small Fresnel numbers F of the deeply holographic regime, well below the typical range exploited at other nano-tomography instruments. This offers the advantage of highest phase sensitivity sufficient to even probe the small electron density variations of unstained tissue, at relatively low dose (Hagemann and Salditt, 2018). To exploit this sensitivity, we use advanced phase retrieval methods including non-linear generalizations of the CTF-method (Cloetens et al., 1999) based on Tikhonov regularization (Lohse et al., 2020).\nFigure 1. Multi-scale x-ray tomography setup.\n(a) HE stain of a tissue micro-array paraffin block with samples of all six patients who succumbed to Covid-19. (b) Schematic representation of the sample preparation and mounting. In a first step biopsy punches containing the entire individual lung tissues are transferred onto a holder for the parallel-beam local tomography acquisition, followed by a further reduction in size to a 1 mm biopsy punch, for cone-beam tomographic recordings. (c) Configuration for parallel-beam tomography. (d) Configuration for cone-beam holo-tomography."}
2_test
{"project":"2_test","denotations":[{"id":"32815517-32105641-26997839","span":{"begin":470,"end":474},"obj":"32105641"},{"id":"32815517-32105637-26997840","span":{"begin":488,"end":492},"obj":"32105637"},{"id":"32815517-32017661-26997841","span":{"begin":508,"end":512},"obj":"32017661"},{"id":"32815517-32437596-26997842","span":{"begin":776,"end":780},"obj":"32437596"},{"id":"32815517-32437596-26997843","span":{"begin":1212,"end":1216},"obj":"32437596"},{"id":"32815517-32437596-26997844","span":{"begin":3001,"end":3005},"obj":"32437596"},{"id":"32815517-32437596-26997845","span":{"begin":3268,"end":3272},"obj":"32437596"},{"id":"32815517-31063133-26997846","span":{"begin":3960,"end":3964},"obj":"31063133"},{"id":"32815517-31061429-26997847","span":{"begin":3980,"end":3984},"obj":"31061429"},{"id":"32815517-29979177-26997848","span":{"begin":4004,"end":4008},"obj":"29979177"},{"id":"32815517-29915047-26997849","span":{"begin":4029,"end":4033},"obj":"29915047"},{"id":"32815517-27321044-26997850","span":{"begin":4054,"end":4058},"obj":"27321044"},{"id":"32815517-28042800-26997851","span":{"begin":4508,"end":4512},"obj":"28042800"},{"id":"32815517-19172736-26997852","span":{"begin":5047,"end":5051},"obj":"19172736"},{"id":"32815517-27461961-26997853","span":{"begin":5067,"end":5071},"obj":"27461961"},{"id":"32815517-31868749-26997854","span":{"begin":5088,"end":5092},"obj":"31868749"},{"id":"32815517-29938163-26997855","span":{"begin":5347,"end":5351},"obj":"29938163"},{"id":"32815517-31129306-26997856","span":{"begin":5620,"end":5624},"obj":"31129306"},{"id":"32815517-26134789-26997857","span":{"begin":6425,"end":6429},"obj":"26134789"},{"id":"32815517-29328301-26997858","span":{"begin":7653,"end":7657},"obj":"29328301"},{"id":"32815517-32381790-26997859","span":{"begin":7856,"end":7860},"obj":"32381790"}],"text":"Introduction\nSevere progression of the 2019 coronavirus disease (Covid-19) is frequently accompanied by the clinical acute respiratory distress syndrome (ARDS) and respiratory failure, an organ manifestation responsible for the majority of Covid-19 fatalities. Lung injury associated with ARDS can be readily detected by radiographic chest imaging and clinical computed tomography (CT), which have assisted the diagnosis and management of Covid-19 patients (Lee et al., 2020; Shi et al., 2020; Chung et al., 2020). Here, so-called peripheral lung ground-glass opacities are the main radiological hallmark of ARDS, and can be linked to the histological observation of diffuse alveolar damage (DAD) with edema, hemorrhage, and intraalveolar fibrin deposition (Ackermann et al., 2020). These findings have also been reported for infections by Middle East respiratory syndrome coronavirus (MERS-CoV), SARS-CoV, and influenza viruses. Distinctive features of pulmonary involvement of Covid-19 include severe endothelial injury associated with the presence of intracellular virions and inflammation, disrupted cellular membranes, as well as widespread thrombosis with microangiopathy. As reported in Ackermann et al., 2020, alveolar capillary microthrombi were found to be nine times as prevalent in patients with Covid-19 as in patients with the also very aggressive H1N1 influenza A virus, also referred to as swine flu. Importantly, in Covid-19 lungs, a specific variant of new vessel growth - intussusceptive angiogenesis - was significantly more prevalent, that is, 2.7 times as high as in lungs of patients with H1N1 influenza A.\nHistomorphological assessment of formalin-fixed, paraffin-embedded (FFPE) tissue stained with haematoxylin and eosin still represents the gold standard in histological diagnostics of non-neoplastic lung diseases, including DAD and virus induced pneumonia. In order to unravel the corresponding pathophysiology of the lungs, digitalization, visualization and quantification of the morphological changes associated with Covid-19 represent a key challenge, and require both high resolution and the capability to screen larger volumes. For this reason, imaging the intricate three-dimensional (3D) tissue architecture of the lung and its pathological alterations on multiple length scales calls for 3D extensions of well-established histology techniques.\nIn this work, we want to demonstrate the potential of propagation-based phase contrast x-ray tomography as a tool for virtual 3D histology in general, and in particular for the histopathology of Covid-19. Our work is based on the assertion that integration of 3D morphological information with well-established histology techniques can provide a substantial asset for unraveling the pathophysiology of SARS-Cov-2 infections. To this end, we have collected x-ray tomography data from the same autopsies, which were previously studied by immunohistochemical analysis and measurements of gene expression (Ackermann et al., 2020). We ask in particular, whether DAD and the morphology of blood vessels can be visualized and quantified in 3D. This is a timely scientific question in Covid-19 research, especially in view of increased intussusceptive angiogenesis reported in Ackermann et al., 2020. Here we present first results obtained from the postmortem lung samples of six patients who succumbed from Covid-19. We exemplify the capability of this approach by 3D visualization of the DAD with hyaline membrane formation, by mapping the 3D distribution and density of angiocentric inflammation (perivascular T-cell infiltration), and by providing histograms of characteristic distances from the tissue interior to the closest air compartment.\nIn contrast to conventional histology based on thin sections, propagation-based x-ray phase-contrast tomography (PC-CT) offers a full 3D visualization with isotropic resolution and without destructive slicing of the specimen (Ding et al., 2019; Dejea et al., 2019; Saccomano et al., 2018; Töpperwien et al., 2018; Khimchenko et al., 2016). The interaction of x-rays with the object is described by the continuous complex-valued index of refraction n(𝐫)=1-δ(𝐫)+iβ(𝐫). Phase contrast capitalizes on the fact that for hard x-rays, the real-valued decrement δ is several orders of magnitude higher in soft biological tissues than β, which accounts for absorption (Nugent, 2010) and that small contrast levels can be reconstructed by propagation imaging even at low fluence (Jahn et al., 2017). Contrast is formed by transformation of the phase shifts into measurable intensity variations by self-interference of the exit wave during free-space propagation between sample and detector (Paganin and Nugent, 1998; Cloetens et al., 1999). However, problems with phase retrieval, phase wrapping, insufficient coherence, or on the contrary strong phase gradients can render its application challenging. For lung imaging it has already been demonstrated as an advantageous imaging technique up to macroscopic scales (Parsons et al., 2008; Stahr et al., 2016; Morgan et al., 2020), including live animal models of respiratory diseases. But in contrast to other full-field phase contrast techniques, e.g. based on grating interferometry or analyzer crystals, it can also reach a resolution below optical microscopy (Khimchenko et al., 2018). In this work we exploit both the high resolution capability of PC-CT and the fact that its phase sensitivity is high enough to probe the small electron density variations of unstained tissue, embedded in paraffin, ethanol, or even aqueous buffer (Töpperwien et al., 2019). However, this requires careful optimization of photon energy, illumination function, and phase retrieval algorithms, as detailed further below.\nThe 3D virtual pathohistology approach for Covid-19 presented here was realized by implementing a novel multi-scale phase contrast x-ray tomography concept, with dedicated x-ray optics and instrumentation. Overview and regions-of-interest (ROI) scans were recorded on the same paraffin-embedded sample, covering a maximum tissue cross section of 8 mm by stitching different tomograms, and with a minimum voxel size of 167 nm in certain ROIs. Scale-bridging and dynamic ROI selection in close spatial and temporal proximity was implemented with dedicated instrumentation the GINIX endstation of the beamline P10/PETRA III (DESY, Hamburg) (Salditt et al., 2015). Specifically, we combined two optical geometries, which has only been realized at different synchrotron beamlines before: (i) Parallel beam tomography, covering a large field of view (FOV), with a pixel size of 650 nm. In this setting, a volumetric throughput on the order of 107μm/3s was achieved, while maintaining the ability to segment isolated cells in unstained tissue. (ii) Cone beam geometry for recording of highly magnified holograms, based on advanced x-ray waveguide optics, providing mode filtering, that is, enhanced spatial coherence and smooth wavefronts. Based on the geometrical magnification, the effective pixel size can be adjusted in the range 10 nm–300 nm. The two imaging schemes are shown schematically in Figure 1c and d, respectively. Further, using this particular optics, together with appropriate choices of photon energy and geometric parameters, we can reach extremely small Fresnel numbers F of the deeply holographic regime, well below the typical range exploited at other nano-tomography instruments. This offers the advantage of highest phase sensitivity sufficient to even probe the small electron density variations of unstained tissue, at relatively low dose (Hagemann and Salditt, 2018). To exploit this sensitivity, we use advanced phase retrieval methods including non-linear generalizations of the CTF-method (Cloetens et al., 1999) based on Tikhonov regularization (Lohse et al., 2020).\nFigure 1. Multi-scale x-ray tomography setup.\n(a) HE stain of a tissue micro-array paraffin block with samples of all six patients who succumbed to Covid-19. (b) Schematic representation of the sample preparation and mounting. In a first step biopsy punches containing the entire individual lung tissues are transferred onto a holder for the parallel-beam local tomography acquisition, followed by a further reduction in size to a 1 mm biopsy punch, for cone-beam tomographic recordings. (c) Configuration for parallel-beam tomography. (d) Configuration for cone-beam holo-tomography."}
MyTest
{"project":"MyTest","denotations":[{"id":"32815517-32105641-26997839","span":{"begin":470,"end":474},"obj":"32105641"},{"id":"32815517-32105637-26997840","span":{"begin":488,"end":492},"obj":"32105637"},{"id":"32815517-32017661-26997841","span":{"begin":508,"end":512},"obj":"32017661"},{"id":"32815517-32437596-26997842","span":{"begin":776,"end":780},"obj":"32437596"},{"id":"32815517-32437596-26997843","span":{"begin":1212,"end":1216},"obj":"32437596"},{"id":"32815517-32437596-26997844","span":{"begin":3001,"end":3005},"obj":"32437596"},{"id":"32815517-32437596-26997845","span":{"begin":3268,"end":3272},"obj":"32437596"},{"id":"32815517-31063133-26997846","span":{"begin":3960,"end":3964},"obj":"31063133"},{"id":"32815517-31061429-26997847","span":{"begin":3980,"end":3984},"obj":"31061429"},{"id":"32815517-29979177-26997848","span":{"begin":4004,"end":4008},"obj":"29979177"},{"id":"32815517-29915047-26997849","span":{"begin":4029,"end":4033},"obj":"29915047"},{"id":"32815517-27321044-26997850","span":{"begin":4054,"end":4058},"obj":"27321044"},{"id":"32815517-28042800-26997851","span":{"begin":4508,"end":4512},"obj":"28042800"},{"id":"32815517-19172736-26997852","span":{"begin":5047,"end":5051},"obj":"19172736"},{"id":"32815517-27461961-26997853","span":{"begin":5067,"end":5071},"obj":"27461961"},{"id":"32815517-31868749-26997854","span":{"begin":5088,"end":5092},"obj":"31868749"},{"id":"32815517-29938163-26997855","span":{"begin":5347,"end":5351},"obj":"29938163"},{"id":"32815517-31129306-26997856","span":{"begin":5620,"end":5624},"obj":"31129306"},{"id":"32815517-26134789-26997857","span":{"begin":6425,"end":6429},"obj":"26134789"},{"id":"32815517-29328301-26997858","span":{"begin":7653,"end":7657},"obj":"29328301"},{"id":"32815517-32381790-26997859","span":{"begin":7856,"end":7860},"obj":"32381790"}],"namespaces":[{"prefix":"_base","uri":"https://www.uniprot.org/uniprot/testbase"},{"prefix":"UniProtKB","uri":"https://www.uniprot.org/uniprot/"},{"prefix":"uniprot","uri":"https://www.uniprot.org/uniprotkb/"}],"text":"Introduction\nSevere progression of the 2019 coronavirus disease (Covid-19) is frequently accompanied by the clinical acute respiratory distress syndrome (ARDS) and respiratory failure, an organ manifestation responsible for the majority of Covid-19 fatalities. Lung injury associated with ARDS can be readily detected by radiographic chest imaging and clinical computed tomography (CT), which have assisted the diagnosis and management of Covid-19 patients (Lee et al., 2020; Shi et al., 2020; Chung et al., 2020). Here, so-called peripheral lung ground-glass opacities are the main radiological hallmark of ARDS, and can be linked to the histological observation of diffuse alveolar damage (DAD) with edema, hemorrhage, and intraalveolar fibrin deposition (Ackermann et al., 2020). These findings have also been reported for infections by Middle East respiratory syndrome coronavirus (MERS-CoV), SARS-CoV, and influenza viruses. Distinctive features of pulmonary involvement of Covid-19 include severe endothelial injury associated with the presence of intracellular virions and inflammation, disrupted cellular membranes, as well as widespread thrombosis with microangiopathy. As reported in Ackermann et al., 2020, alveolar capillary microthrombi were found to be nine times as prevalent in patients with Covid-19 as in patients with the also very aggressive H1N1 influenza A virus, also referred to as swine flu. Importantly, in Covid-19 lungs, a specific variant of new vessel growth - intussusceptive angiogenesis - was significantly more prevalent, that is, 2.7 times as high as in lungs of patients with H1N1 influenza A.\nHistomorphological assessment of formalin-fixed, paraffin-embedded (FFPE) tissue stained with haematoxylin and eosin still represents the gold standard in histological diagnostics of non-neoplastic lung diseases, including DAD and virus induced pneumonia. In order to unravel the corresponding pathophysiology of the lungs, digitalization, visualization and quantification of the morphological changes associated with Covid-19 represent a key challenge, and require both high resolution and the capability to screen larger volumes. For this reason, imaging the intricate three-dimensional (3D) tissue architecture of the lung and its pathological alterations on multiple length scales calls for 3D extensions of well-established histology techniques.\nIn this work, we want to demonstrate the potential of propagation-based phase contrast x-ray tomography as a tool for virtual 3D histology in general, and in particular for the histopathology of Covid-19. Our work is based on the assertion that integration of 3D morphological information with well-established histology techniques can provide a substantial asset for unraveling the pathophysiology of SARS-Cov-2 infections. To this end, we have collected x-ray tomography data from the same autopsies, which were previously studied by immunohistochemical analysis and measurements of gene expression (Ackermann et al., 2020). We ask in particular, whether DAD and the morphology of blood vessels can be visualized and quantified in 3D. This is a timely scientific question in Covid-19 research, especially in view of increased intussusceptive angiogenesis reported in Ackermann et al., 2020. Here we present first results obtained from the postmortem lung samples of six patients who succumbed from Covid-19. We exemplify the capability of this approach by 3D visualization of the DAD with hyaline membrane formation, by mapping the 3D distribution and density of angiocentric inflammation (perivascular T-cell infiltration), and by providing histograms of characteristic distances from the tissue interior to the closest air compartment.\nIn contrast to conventional histology based on thin sections, propagation-based x-ray phase-contrast tomography (PC-CT) offers a full 3D visualization with isotropic resolution and without destructive slicing of the specimen (Ding et al., 2019; Dejea et al., 2019; Saccomano et al., 2018; Töpperwien et al., 2018; Khimchenko et al., 2016). The interaction of x-rays with the object is described by the continuous complex-valued index of refraction n(𝐫)=1-δ(𝐫)+iβ(𝐫). Phase contrast capitalizes on the fact that for hard x-rays, the real-valued decrement δ is several orders of magnitude higher in soft biological tissues than β, which accounts for absorption (Nugent, 2010) and that small contrast levels can be reconstructed by propagation imaging even at low fluence (Jahn et al., 2017). Contrast is formed by transformation of the phase shifts into measurable intensity variations by self-interference of the exit wave during free-space propagation between sample and detector (Paganin and Nugent, 1998; Cloetens et al., 1999). However, problems with phase retrieval, phase wrapping, insufficient coherence, or on the contrary strong phase gradients can render its application challenging. For lung imaging it has already been demonstrated as an advantageous imaging technique up to macroscopic scales (Parsons et al., 2008; Stahr et al., 2016; Morgan et al., 2020), including live animal models of respiratory diseases. But in contrast to other full-field phase contrast techniques, e.g. based on grating interferometry or analyzer crystals, it can also reach a resolution below optical microscopy (Khimchenko et al., 2018). In this work we exploit both the high resolution capability of PC-CT and the fact that its phase sensitivity is high enough to probe the small electron density variations of unstained tissue, embedded in paraffin, ethanol, or even aqueous buffer (Töpperwien et al., 2019). However, this requires careful optimization of photon energy, illumination function, and phase retrieval algorithms, as detailed further below.\nThe 3D virtual pathohistology approach for Covid-19 presented here was realized by implementing a novel multi-scale phase contrast x-ray tomography concept, with dedicated x-ray optics and instrumentation. Overview and regions-of-interest (ROI) scans were recorded on the same paraffin-embedded sample, covering a maximum tissue cross section of 8 mm by stitching different tomograms, and with a minimum voxel size of 167 nm in certain ROIs. Scale-bridging and dynamic ROI selection in close spatial and temporal proximity was implemented with dedicated instrumentation the GINIX endstation of the beamline P10/PETRA III (DESY, Hamburg) (Salditt et al., 2015). Specifically, we combined two optical geometries, which has only been realized at different synchrotron beamlines before: (i) Parallel beam tomography, covering a large field of view (FOV), with a pixel size of 650 nm. In this setting, a volumetric throughput on the order of 107μm/3s was achieved, while maintaining the ability to segment isolated cells in unstained tissue. (ii) Cone beam geometry for recording of highly magnified holograms, based on advanced x-ray waveguide optics, providing mode filtering, that is, enhanced spatial coherence and smooth wavefronts. Based on the geometrical magnification, the effective pixel size can be adjusted in the range 10 nm–300 nm. The two imaging schemes are shown schematically in Figure 1c and d, respectively. Further, using this particular optics, together with appropriate choices of photon energy and geometric parameters, we can reach extremely small Fresnel numbers F of the deeply holographic regime, well below the typical range exploited at other nano-tomography instruments. This offers the advantage of highest phase sensitivity sufficient to even probe the small electron density variations of unstained tissue, at relatively low dose (Hagemann and Salditt, 2018). To exploit this sensitivity, we use advanced phase retrieval methods including non-linear generalizations of the CTF-method (Cloetens et al., 1999) based on Tikhonov regularization (Lohse et al., 2020).\nFigure 1. Multi-scale x-ray tomography setup.\n(a) HE stain of a tissue micro-array paraffin block with samples of all six patients who succumbed to Covid-19. (b) Schematic representation of the sample preparation and mounting. In a first step biopsy punches containing the entire individual lung tissues are transferred onto a holder for the parallel-beam local tomography acquisition, followed by a further reduction in size to a 1 mm biopsy punch, for cone-beam tomographic recordings. (c) Configuration for parallel-beam tomography. (d) Configuration for cone-beam holo-tomography."}
LitCovid-PubTator
{"project":"LitCovid-PubTator","denotations":[{"id":"45","span":{"begin":448,"end":456},"obj":"Species"},{"id":"46","span":{"begin":840,"end":895},"obj":"Species"},{"id":"47","span":{"begin":897,"end":905},"obj":"Species"},{"id":"48","span":{"begin":911,"end":928},"obj":"Species"},{"id":"49","span":{"begin":1294,"end":1302},"obj":"Species"},{"id":"50","span":{"begin":1323,"end":1331},"obj":"Species"},{"id":"51","span":{"begin":1362,"end":1366},"obj":"Species"},{"id":"52","span":{"begin":1367,"end":1384},"obj":"Species"},{"id":"53","span":{"begin":1406,"end":1411},"obj":"Species"},{"id":"54","span":{"begin":1598,"end":1606},"obj":"Species"},{"id":"55","span":{"begin":1612,"end":1616},"obj":"Species"},{"id":"56","span":{"begin":1617,"end":1626},"obj":"Species"},{"id":"57","span":{"begin":39,"end":63},"obj":"Disease"},{"id":"58","span":{"begin":65,"end":73},"obj":"Disease"},{"id":"59","span":{"begin":123,"end":152},"obj":"Disease"},{"id":"60","span":{"begin":154,"end":158},"obj":"Disease"},{"id":"61","span":{"begin":164,"end":183},"obj":"Disease"},{"id":"62","span":{"begin":240,"end":248},"obj":"Disease"},{"id":"63","span":{"begin":261,"end":272},"obj":"Disease"},{"id":"64","span":{"begin":289,"end":293},"obj":"Disease"},{"id":"65","span":{"begin":439,"end":447},"obj":"Disease"},{"id":"66","span":{"begin":608,"end":612},"obj":"Disease"},{"id":"67","span":{"begin":675,"end":690},"obj":"Disease"},{"id":"68","span":{"begin":702,"end":707},"obj":"Disease"},{"id":"69","span":{"begin":709,"end":719},"obj":"Disease"},{"id":"70","span":{"begin":826,"end":836},"obj":"Disease"},{"id":"71","span":{"begin":979,"end":987},"obj":"Disease"},{"id":"72","span":{"begin":1003,"end":1021},"obj":"Disease"},{"id":"73","span":{"begin":1080,"end":1092},"obj":"Disease"},{"id":"74","span":{"begin":1146,"end":1156},"obj":"Disease"},{"id":"75","span":{"begin":1162,"end":1177},"obj":"Disease"},{"id":"76","span":{"begin":1308,"end":1316},"obj":"Disease"},{"id":"77","span":{"begin":1433,"end":1441},"obj":"Disease"},{"id":"85","span":{"begin":1663,"end":1671},"obj":"Chemical"},{"id":"86","span":{"begin":1679,"end":1687},"obj":"Chemical"},{"id":"87","span":{"begin":1724,"end":1736},"obj":"Chemical"},{"id":"88","span":{"begin":1741,"end":1746},"obj":"Chemical"},{"id":"89","span":{"begin":1828,"end":1841},"obj":"Disease"},{"id":"90","span":{"begin":1875,"end":1884},"obj":"Disease"},{"id":"91","span":{"begin":2048,"end":2056},"obj":"Disease"},{"id":"98","span":{"begin":3353,"end":3361},"obj":"Species"},{"id":"99","span":{"begin":2576,"end":2584},"obj":"Disease"},{"id":"100","span":{"begin":2783,"end":2804},"obj":"Disease"},{"id":"101","span":{"begin":3158,"end":3166},"obj":"Disease"},{"id":"102","span":{"begin":3381,"end":3389},"obj":"Disease"},{"id":"103","span":{"begin":3546,"end":3571},"obj":"Disease"},{"id":"109","span":{"begin":5558,"end":5566},"obj":"Chemical"},{"id":"110","span":{"begin":5568,"end":5575},"obj":"Chemical"},{"id":"111","span":{"begin":5127,"end":5147},"obj":"Disease"},{"id":"112","span":{"begin":3834,"end":3839},"obj":"CellLine"},{"id":"113","span":{"begin":5417,"end":5422},"obj":"CellLine"},{"id":"116","span":{"begin":6048,"end":6056},"obj":"Chemical"},{"id":"117","span":{"begin":5814,"end":5822},"obj":"Disease"},{"id":"122","span":{"begin":7986,"end":7994},"obj":"Species"},{"id":"123","span":{"begin":7914,"end":7916},"obj":"Chemical"},{"id":"124","span":{"begin":7947,"end":7955},"obj":"Chemical"},{"id":"125","span":{"begin":8012,"end":8020},"obj":"Disease"}],"attributes":[{"id":"A45","pred":"tao:has_database_id","subj":"45","obj":"Tax:9606"},{"id":"A46","pred":"tao:has_database_id","subj":"46","obj":"Tax:1335626"},{"id":"A47","pred":"tao:has_database_id","subj":"47","obj":"Tax:694009"},{"id":"A48","pred":"tao:has_database_id","subj":"48","obj":"Tax:11308"},{"id":"A49","pred":"tao:has_database_id","subj":"49","obj":"Tax:9606"},{"id":"A50","pred":"tao:has_database_id","subj":"50","obj":"Tax:9606"},{"id":"A51","pred":"tao:has_database_id","subj":"51","obj":"Tax:114727"},{"id":"A52","pred":"tao:has_database_id","subj":"52","obj":"Tax:11320"},{"id":"A53","pred":"tao:has_database_id","subj":"53","obj":"Tax:9823"},{"id":"A54","pred":"tao:has_database_id","subj":"54","obj":"Tax:9606"},{"id":"A55","pred":"tao:has_database_id","subj":"55","obj":"Tax:114727"},{"id":"A56","pred":"tao:has_database_id","subj":"56","obj":"Tax:11320"},{"id":"A57","pred":"tao:has_database_id","subj":"57","obj":"MESH:C000657245"},{"id":"A58","pred":"tao:has_database_id","subj":"58","obj":"MESH:C000657245"},{"id":"A59","pred":"tao:has_database_id","subj":"59","obj":"MESH:D012128"},{"id":"A60","pred":"tao:has_database_id","subj":"60","obj":"MESH:D012128"},{"id":"A61","pred":"tao:has_database_id","subj":"61","obj":"MESH:D012131"},{"id":"A62","pred":"tao:has_database_id","subj":"62","obj":"MESH:C000657245"},{"id":"A63","pred":"tao:has_database_id","subj":"63","obj":"MESH:D055370"},{"id":"A64","pred":"tao:has_database_id","subj":"64","obj":"MESH:D012128"},{"id":"A65","pred":"tao:has_database_id","subj":"65","obj":"MESH:C000657245"},{"id":"A66","pred":"tao:has_database_id","subj":"66","obj":"MESH:D012128"},{"id":"A67","pred":"tao:has_database_id","subj":"67","obj":"MESH:D055370"},{"id":"A68","pred":"tao:has_database_id","subj":"68","obj":"MESH:D004487"},{"id":"A69","pred":"tao:has_database_id","subj":"69","obj":"MESH:D006470"},{"id":"A70","pred":"tao:has_database_id","subj":"70","obj":"MESH:D007239"},{"id":"A71","pred":"tao:has_database_id","subj":"71","obj":"MESH:C000657245"},{"id":"A72","pred":"tao:has_database_id","subj":"72","obj":"MESH:D014947"},{"id":"A73","pred":"tao:has_database_id","subj":"73","obj":"MESH:D007249"},{"id":"A74","pred":"tao:has_database_id","subj":"74","obj":"MESH:D013927"},{"id":"A75","pred":"tao:has_database_id","subj":"75","obj":"MESH:D000783"},{"id":"A76","pred":"tao:has_database_id","subj":"76","obj":"MESH:C000657245"},{"id":"A77","pred":"tao:has_database_id","subj":"77","obj":"MESH:C000657245"},{"id":"A85","pred":"tao:has_database_id","subj":"85","obj":"MESH:D005557"},{"id":"A86","pred":"tao:has_database_id","subj":"86","obj":"MESH:D010232"},{"id":"A87","pred":"tao:has_database_id","subj":"87","obj":"MESH:D006416"},{"id":"A88","pred":"tao:has_database_id","subj":"88","obj":"MESH:D004801"},{"id":"A89","pred":"tao:has_database_id","subj":"89","obj":"MESH:D008171"},{"id":"A90","pred":"tao:has_database_id","subj":"90","obj":"MESH:D011014"},{"id":"A91","pred":"tao:has_database_id","subj":"91","obj":"MESH:C000657245"},{"id":"A98","pred":"tao:has_database_id","subj":"98","obj":"Tax:9606"},{"id":"A99","pred":"tao:has_database_id","subj":"99","obj":"MESH:C000657245"},{"id":"A100","pred":"tao:has_database_id","subj":"100","obj":"MESH:C000657245"},{"id":"A101","pred":"tao:has_database_id","subj":"101","obj":"MESH:C000657245"},{"id":"A102","pred":"tao:has_database_id","subj":"102","obj":"MESH:C000657245"},{"id":"A103","pred":"tao:has_database_id","subj":"103","obj":"MESH:D007249"},{"id":"A109","pred":"tao:has_database_id","subj":"109","obj":"MESH:D010232"},{"id":"A110","pred":"tao:has_database_id","subj":"110","obj":"MESH:D000431"},{"id":"A111","pred":"tao:has_database_id","subj":"111","obj":"MESH:D012140"},{"id":"A112","pred":"tao:has_database_id","subj":"112","obj":"CVCL:B848"},{"id":"A113","pred":"tao:has_database_id","subj":"113","obj":"CVCL:B848"},{"id":"A116","pred":"tao:has_database_id","subj":"116","obj":"MESH:D010232"},{"id":"A117","pred":"tao:has_database_id","subj":"117","obj":"MESH:C000657245"},{"id":"A122","pred":"tao:has_database_id","subj":"122","obj":"Tax:9606"},{"id":"A124","pred":"tao:has_database_id","subj":"124","obj":"MESH:D010232"},{"id":"A125","pred":"tao:has_database_id","subj":"125","obj":"MESH:C000657245"}],"namespaces":[{"prefix":"Tax","uri":"https://www.ncbi.nlm.nih.gov/taxonomy/"},{"prefix":"MESH","uri":"https://id.nlm.nih.gov/mesh/"},{"prefix":"Gene","uri":"https://www.ncbi.nlm.nih.gov/gene/"},{"prefix":"CVCL","uri":"https://web.expasy.org/cellosaurus/CVCL_"}],"text":"Introduction\nSevere progression of the 2019 coronavirus disease (Covid-19) is frequently accompanied by the clinical acute respiratory distress syndrome (ARDS) and respiratory failure, an organ manifestation responsible for the majority of Covid-19 fatalities. Lung injury associated with ARDS can be readily detected by radiographic chest imaging and clinical computed tomography (CT), which have assisted the diagnosis and management of Covid-19 patients (Lee et al., 2020; Shi et al., 2020; Chung et al., 2020). Here, so-called peripheral lung ground-glass opacities are the main radiological hallmark of ARDS, and can be linked to the histological observation of diffuse alveolar damage (DAD) with edema, hemorrhage, and intraalveolar fibrin deposition (Ackermann et al., 2020). These findings have also been reported for infections by Middle East respiratory syndrome coronavirus (MERS-CoV), SARS-CoV, and influenza viruses. Distinctive features of pulmonary involvement of Covid-19 include severe endothelial injury associated with the presence of intracellular virions and inflammation, disrupted cellular membranes, as well as widespread thrombosis with microangiopathy. As reported in Ackermann et al., 2020, alveolar capillary microthrombi were found to be nine times as prevalent in patients with Covid-19 as in patients with the also very aggressive H1N1 influenza A virus, also referred to as swine flu. Importantly, in Covid-19 lungs, a specific variant of new vessel growth - intussusceptive angiogenesis - was significantly more prevalent, that is, 2.7 times as high as in lungs of patients with H1N1 influenza A.\nHistomorphological assessment of formalin-fixed, paraffin-embedded (FFPE) tissue stained with haematoxylin and eosin still represents the gold standard in histological diagnostics of non-neoplastic lung diseases, including DAD and virus induced pneumonia. In order to unravel the corresponding pathophysiology of the lungs, digitalization, visualization and quantification of the morphological changes associated with Covid-19 represent a key challenge, and require both high resolution and the capability to screen larger volumes. For this reason, imaging the intricate three-dimensional (3D) tissue architecture of the lung and its pathological alterations on multiple length scales calls for 3D extensions of well-established histology techniques.\nIn this work, we want to demonstrate the potential of propagation-based phase contrast x-ray tomography as a tool for virtual 3D histology in general, and in particular for the histopathology of Covid-19. Our work is based on the assertion that integration of 3D morphological information with well-established histology techniques can provide a substantial asset for unraveling the pathophysiology of SARS-Cov-2 infections. To this end, we have collected x-ray tomography data from the same autopsies, which were previously studied by immunohistochemical analysis and measurements of gene expression (Ackermann et al., 2020). We ask in particular, whether DAD and the morphology of blood vessels can be visualized and quantified in 3D. This is a timely scientific question in Covid-19 research, especially in view of increased intussusceptive angiogenesis reported in Ackermann et al., 2020. Here we present first results obtained from the postmortem lung samples of six patients who succumbed from Covid-19. We exemplify the capability of this approach by 3D visualization of the DAD with hyaline membrane formation, by mapping the 3D distribution and density of angiocentric inflammation (perivascular T-cell infiltration), and by providing histograms of characteristic distances from the tissue interior to the closest air compartment.\nIn contrast to conventional histology based on thin sections, propagation-based x-ray phase-contrast tomography (PC-CT) offers a full 3D visualization with isotropic resolution and without destructive slicing of the specimen (Ding et al., 2019; Dejea et al., 2019; Saccomano et al., 2018; Töpperwien et al., 2018; Khimchenko et al., 2016). The interaction of x-rays with the object is described by the continuous complex-valued index of refraction n(𝐫)=1-δ(𝐫)+iβ(𝐫). Phase contrast capitalizes on the fact that for hard x-rays, the real-valued decrement δ is several orders of magnitude higher in soft biological tissues than β, which accounts for absorption (Nugent, 2010) and that small contrast levels can be reconstructed by propagation imaging even at low fluence (Jahn et al., 2017). Contrast is formed by transformation of the phase shifts into measurable intensity variations by self-interference of the exit wave during free-space propagation between sample and detector (Paganin and Nugent, 1998; Cloetens et al., 1999). However, problems with phase retrieval, phase wrapping, insufficient coherence, or on the contrary strong phase gradients can render its application challenging. For lung imaging it has already been demonstrated as an advantageous imaging technique up to macroscopic scales (Parsons et al., 2008; Stahr et al., 2016; Morgan et al., 2020), including live animal models of respiratory diseases. But in contrast to other full-field phase contrast techniques, e.g. based on grating interferometry or analyzer crystals, it can also reach a resolution below optical microscopy (Khimchenko et al., 2018). In this work we exploit both the high resolution capability of PC-CT and the fact that its phase sensitivity is high enough to probe the small electron density variations of unstained tissue, embedded in paraffin, ethanol, or even aqueous buffer (Töpperwien et al., 2019). However, this requires careful optimization of photon energy, illumination function, and phase retrieval algorithms, as detailed further below.\nThe 3D virtual pathohistology approach for Covid-19 presented here was realized by implementing a novel multi-scale phase contrast x-ray tomography concept, with dedicated x-ray optics and instrumentation. Overview and regions-of-interest (ROI) scans were recorded on the same paraffin-embedded sample, covering a maximum tissue cross section of 8 mm by stitching different tomograms, and with a minimum voxel size of 167 nm in certain ROIs. Scale-bridging and dynamic ROI selection in close spatial and temporal proximity was implemented with dedicated instrumentation the GINIX endstation of the beamline P10/PETRA III (DESY, Hamburg) (Salditt et al., 2015). Specifically, we combined two optical geometries, which has only been realized at different synchrotron beamlines before: (i) Parallel beam tomography, covering a large field of view (FOV), with a pixel size of 650 nm. In this setting, a volumetric throughput on the order of 107μm/3s was achieved, while maintaining the ability to segment isolated cells in unstained tissue. (ii) Cone beam geometry for recording of highly magnified holograms, based on advanced x-ray waveguide optics, providing mode filtering, that is, enhanced spatial coherence and smooth wavefronts. Based on the geometrical magnification, the effective pixel size can be adjusted in the range 10 nm–300 nm. The two imaging schemes are shown schematically in Figure 1c and d, respectively. Further, using this particular optics, together with appropriate choices of photon energy and geometric parameters, we can reach extremely small Fresnel numbers F of the deeply holographic regime, well below the typical range exploited at other nano-tomography instruments. This offers the advantage of highest phase sensitivity sufficient to even probe the small electron density variations of unstained tissue, at relatively low dose (Hagemann and Salditt, 2018). To exploit this sensitivity, we use advanced phase retrieval methods including non-linear generalizations of the CTF-method (Cloetens et al., 1999) based on Tikhonov regularization (Lohse et al., 2020).\nFigure 1. Multi-scale x-ray tomography setup.\n(a) HE stain of a tissue micro-array paraffin block with samples of all six patients who succumbed to Covid-19. (b) Schematic representation of the sample preparation and mounting. In a first step biopsy punches containing the entire individual lung tissues are transferred onto a holder for the parallel-beam local tomography acquisition, followed by a further reduction in size to a 1 mm biopsy punch, for cone-beam tomographic recordings. (c) Configuration for parallel-beam tomography. (d) Configuration for cone-beam holo-tomography."}