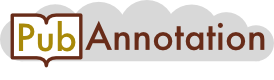
PMC:7443692 / 19131-23396
Annnotations
LitCovid-sample-CHEBI
{"project":"LitCovid-sample-CHEBI","denotations":[{"id":"T133","span":{"begin":108,"end":120},"obj":"Chemical"},{"id":"T134","span":{"begin":878,"end":885},"obj":"Chemical"},{"id":"T135","span":{"begin":997,"end":1005},"obj":"Chemical"},{"id":"T136","span":{"begin":1063,"end":1071},"obj":"Chemical"},{"id":"T137","span":{"begin":1151,"end":1159},"obj":"Chemical"},{"id":"T138","span":{"begin":1277,"end":1284},"obj":"Chemical"},{"id":"T139","span":{"begin":1355,"end":1362},"obj":"Chemical"},{"id":"T140","span":{"begin":1526,"end":1533},"obj":"Chemical"},{"id":"T141","span":{"begin":1972,"end":1979},"obj":"Chemical"},{"id":"T142","span":{"begin":2160,"end":2167},"obj":"Chemical"},{"id":"T143","span":{"begin":2316,"end":2323},"obj":"Chemical"},{"id":"T144","span":{"begin":2324,"end":2331},"obj":"Chemical"},{"id":"T145","span":{"begin":2623,"end":2630},"obj":"Chemical"},{"id":"T146","span":{"begin":3057,"end":3064},"obj":"Chemical"},{"id":"T147","span":{"begin":3166,"end":3169},"obj":"Chemical"},{"id":"T148","span":{"begin":3200,"end":3211},"obj":"Chemical"},{"id":"T149","span":{"begin":3335,"end":3342},"obj":"Chemical"},{"id":"T150","span":{"begin":3648,"end":3655},"obj":"Chemical"},{"id":"T151","span":{"begin":3833,"end":3841},"obj":"Chemical"},{"id":"T152","span":{"begin":3886,"end":3895},"obj":"Chemical"},{"id":"T153","span":{"begin":3896,"end":3904},"obj":"Chemical"},{"id":"T154","span":{"begin":4019,"end":4028},"obj":"Chemical"},{"id":"T155","span":{"begin":4035,"end":4042},"obj":"Chemical"},{"id":"T156","span":{"begin":4092,"end":4099},"obj":"Chemical"},{"id":"T157","span":{"begin":4254,"end":4264},"obj":"Chemical"}],"attributes":[{"id":"A153","pred":"chebi_id","subj":"T153","obj":"http://purl.obolibrary.org/obo/CHEBI_59520"},{"id":"A135","pred":"chebi_id","subj":"T135","obj":"http://purl.obolibrary.org/obo/CHEBI_59520"},{"id":"A144","pred":"chebi_id","subj":"T144","obj":"http://purl.obolibrary.org/obo/CHEBI_18154"},{"id":"A150","pred":"chebi_id","subj":"T150","obj":"http://purl.obolibrary.org/obo/CHEBI_18154"},{"id":"A154","pred":"chebi_id","subj":"T154","obj":"http://purl.obolibrary.org/obo/CHEBI_59520"},{"id":"A136","pred":"chebi_id","subj":"T136","obj":"http://purl.obolibrary.org/obo/CHEBI_59520"},{"id":"A149","pred":"chebi_id","subj":"T149","obj":"http://purl.obolibrary.org/obo/CHEBI_37684"},{"id":"A139","pred":"chebi_id","subj":"T139","obj":"http://purl.obolibrary.org/obo/CHEBI_37684"},{"id":"A152","pred":"chebi_id","subj":"T152","obj":"http://purl.obolibrary.org/obo/CHEBI_59520"},{"id":"A143","pred":"chebi_id","subj":"T143","obj":"http://purl.obolibrary.org/obo/CHEBI_37684"},{"id":"A141","pred":"chebi_id","subj":"T141","obj":"http://purl.obolibrary.org/obo/CHEBI_18154"},{"id":"A157","pred":"chebi_id","subj":"T157","obj":"http://purl.obolibrary.org/obo/CHEBI_33709"},{"id":"A148","pred":"chebi_id","subj":"T148","obj":"http://purl.obolibrary.org/obo/CHEBI_82646"},{"id":"A145","pred":"chebi_id","subj":"T145","obj":"http://purl.obolibrary.org/obo/CHEBI_37684"},{"id":"A156","pred":"chebi_id","subj":"T156","obj":"http://purl.obolibrary.org/obo/CHEBI_16670"},{"id":"A151","pred":"chebi_id","subj":"T151","obj":"http://purl.obolibrary.org/obo/CHEBI_59520"},{"id":"A134","pred":"chebi_id","subj":"T134","obj":"http://purl.obolibrary.org/obo/CHEBI_18154"},{"id":"A147","pred":"chebi_id","subj":"T147","obj":"http://purl.obolibrary.org/obo/CHEBI_37684"},{"id":"A138","pred":"chebi_id","subj":"T138","obj":"http://purl.obolibrary.org/obo/CHEBI_37684"},{"id":"A140","pred":"chebi_id","subj":"T140","obj":"http://purl.obolibrary.org/obo/CHEBI_37684"},{"id":"A142","pred":"chebi_id","subj":"T142","obj":"http://purl.obolibrary.org/obo/CHEBI_37684"},{"id":"A137","pred":"chebi_id","subj":"T137","obj":"http://purl.obolibrary.org/obo/CHEBI_59520"},{"id":"A133","pred":"chebi_id","subj":"T133","obj":"http://purl.obolibrary.org/obo/CHEBI_24396"},{"id":"A155","pred":"chebi_id","subj":"T155","obj":"http://purl.obolibrary.org/obo/CHEBI_37684"},{"id":"A146","pred":"chebi_id","subj":"T146","obj":"http://purl.obolibrary.org/obo/CHEBI_37684"}],"text":"Next, we applied three different proteolytic digestion strategies to the SARS-CoV-2 S immunogen to maximize glycopeptide coverage by subsequent LC-MS/MS analyses. Extended gradient nanoflow reverse-phase LC-MS/MS was carried out on a ThermoFisher Lumos Tribrid instrument using Step-HCD fragmentation on each of the samples (see STAR Methods for details, as well as Duan et al., 2018; Escolano et al., 2019; Wang et al., 2020; Yu et al., 2018; Zhou et al., 2017). After data analyses using pGlyco 2.2.2 (Liu et al., 2017), Byonic (Bern et al., 2012), and manual validation of glycan compositions against our released glycomics findings (Figure 2A; Tables S3 and S13), we were able to determine the microheterogeneity at each of the 22 canonical sites (Figures 2B–2E; Table S6). Notably, none of the non-canonical consensus sequences, including N0501, displayed any quantifiable glycans. The N-glycosites N0074 (Figure 2B) and N0149 (Figure 2C) are highly processed and display a typical mammalian N-glycan profile. N0149 is, however, modified with several hybrid N-glycan structures, whereas N0074 is not. N0234 (Figure 2D) and N0801 (Figure 2E) have N-glycan profiles more similar to those found on other viruses such as HIV (Watanabe et al., 2019) that are dominated by high-mannose structures. N0234 (Figure 2D) displays an abundance of Man7-Man9 high-mannose structures, suggesting stalled processing by early-acting ER and cis-Golgi mannosidases. In contrast, N0801 (Figure 2E) is processed more efficiently to Man5 high-mannose and hybrid structures, suggesting that access to the glycan at this site by MGAT1 and α-Mannosidase II is hindered. In general, for all 22 sites (Figures 2B–2E; Table S6), we observed underprocessing of complex glycan antennae (i.e., under-galactosylation and under-sialylation) and a high degree of core fucosylation in agreement with released glycan analyses (Figure 2A; Table S3). We also observed a small percent of sulfated N-linked glycans at several sites (Tables S6 and S8). Based on the assignments and the spectral counts for each topology, we were able to determine the percent of total N-linked glycan types (high-mannose, hybrid, or complex) present at each site (Figure 3 ; Table S7). Notably, three of the sites (N0234, N0709, and N0717) displayed more than 50% high-mannose glycans, whereas 11 other sites (N0017, N0074, N0149, N0165, N0282, N0331, N0657, N1134, N1158, N1173, and N1194) were more than 90% complex when occupied. The other eight sites were distributed between these two extremes. Notably, only one site (N0717 at 45%), which also had greater than 50% high mannose (55%), had greater than 33% hybrid structures. To further evaluate the heterogeneity, we grouped all the topologies into the 20 classes recently described by the Crispin laboratory, adding two categories (sulfated and unoccupied) that we refer to here as the Oxford classification (Table S8) (Watanabe et al., 2020a). Among other features observed, this classification allowed us to observe that although most sites with high-mannose structures were dominated by the Man5GlcNAc2 structure, N0234 and N0717 were dominated by the higher Man structures of Man8GlcNAc2 and Man7GlcNAc2, respectively (Figure S7; Table S8). Limited processing at N0234 is in agreement with a recent report suggesting that high-mannose structures at this site help to stabilize the receptor-binding domain of S (www.biorxiv.org/content/10.1101/2020.06.11.146522v1). Furthermore, applying the Oxford classifications to our dataset clearly demonstrates that the three most C-terminal sites (N1158, N1173, and N1194), dominated by complex-type glycans, were more often further processed (i.e., multiple antennae) and elaborated (i.e., galactosylation and sialylation) than other sites (Table S8).\nFigure 3 SARS-CoV-2 S Immunogen N-glycan Sites Are Predominantly Modified by Complex N-glycans\nN-glycan topologies were assigned to all 22 sites of the S protomer and the spectral counts for each of the three types of N-glycans (high-mannose, hybrid, and complex), as well as the unoccupied peptide spectral match counts at each site, were summed and visualized as pie charts. Note that only N1173 and N1194 show an appreciable amount of the unoccupied amino acid."}
LitCovid-sample-PD-NCBITaxon
{"project":"LitCovid-sample-PD-NCBITaxon","denotations":[{"id":"T60","span":{"begin":73,"end":83},"obj":"Species"},{"id":"T61","span":{"begin":1206,"end":1213},"obj":"Species"},{"id":"T62","span":{"begin":1222,"end":1225},"obj":"Species"},{"id":"T67","span":{"begin":2793,"end":2800},"obj":"Species"},{"id":"T68","span":{"begin":3810,"end":3820},"obj":"Species"}],"attributes":[{"id":"A62","pred":"ncbi_taxonomy_id","subj":"T62","obj":"NCBItxid:57667"},{"id":"A63","pred":"ncbi_taxonomy_id","subj":"T62","obj":"NCBItxid:35274"},{"id":"A64","pred":"ncbi_taxonomy_id","subj":"T62","obj":"NCBItxid:12721"},{"id":"A65","pred":"ncbi_taxonomy_id","subj":"T62","obj":"NCBItxid:11709"},{"id":"A66","pred":"ncbi_taxonomy_id","subj":"T62","obj":"NCBItxid:11676"},{"id":"A67","pred":"ncbi_taxonomy_id","subj":"T67","obj":"NCBItxid:300214"},{"id":"A61","pred":"ncbi_taxonomy_id","subj":"T61","obj":"NCBItxid:10239"},{"id":"A68","pred":"ncbi_taxonomy_id","subj":"T68","obj":"NCBItxid:2697049"},{"id":"A60","pred":"ncbi_taxonomy_id","subj":"T60","obj":"NCBItxid:2697049"}],"namespaces":[{"prefix":"NCBItxid","uri":"http://purl.bioontology.org/ontology/NCBITAXON/"}],"text":"Next, we applied three different proteolytic digestion strategies to the SARS-CoV-2 S immunogen to maximize glycopeptide coverage by subsequent LC-MS/MS analyses. Extended gradient nanoflow reverse-phase LC-MS/MS was carried out on a ThermoFisher Lumos Tribrid instrument using Step-HCD fragmentation on each of the samples (see STAR Methods for details, as well as Duan et al., 2018; Escolano et al., 2019; Wang et al., 2020; Yu et al., 2018; Zhou et al., 2017). After data analyses using pGlyco 2.2.2 (Liu et al., 2017), Byonic (Bern et al., 2012), and manual validation of glycan compositions against our released glycomics findings (Figure 2A; Tables S3 and S13), we were able to determine the microheterogeneity at each of the 22 canonical sites (Figures 2B–2E; Table S6). Notably, none of the non-canonical consensus sequences, including N0501, displayed any quantifiable glycans. The N-glycosites N0074 (Figure 2B) and N0149 (Figure 2C) are highly processed and display a typical mammalian N-glycan profile. N0149 is, however, modified with several hybrid N-glycan structures, whereas N0074 is not. N0234 (Figure 2D) and N0801 (Figure 2E) have N-glycan profiles more similar to those found on other viruses such as HIV (Watanabe et al., 2019) that are dominated by high-mannose structures. N0234 (Figure 2D) displays an abundance of Man7-Man9 high-mannose structures, suggesting stalled processing by early-acting ER and cis-Golgi mannosidases. In contrast, N0801 (Figure 2E) is processed more efficiently to Man5 high-mannose and hybrid structures, suggesting that access to the glycan at this site by MGAT1 and α-Mannosidase II is hindered. In general, for all 22 sites (Figures 2B–2E; Table S6), we observed underprocessing of complex glycan antennae (i.e., under-galactosylation and under-sialylation) and a high degree of core fucosylation in agreement with released glycan analyses (Figure 2A; Table S3). We also observed a small percent of sulfated N-linked glycans at several sites (Tables S6 and S8). Based on the assignments and the spectral counts for each topology, we were able to determine the percent of total N-linked glycan types (high-mannose, hybrid, or complex) present at each site (Figure 3 ; Table S7). Notably, three of the sites (N0234, N0709, and N0717) displayed more than 50% high-mannose glycans, whereas 11 other sites (N0017, N0074, N0149, N0165, N0282, N0331, N0657, N1134, N1158, N1173, and N1194) were more than 90% complex when occupied. The other eight sites were distributed between these two extremes. Notably, only one site (N0717 at 45%), which also had greater than 50% high mannose (55%), had greater than 33% hybrid structures. To further evaluate the heterogeneity, we grouped all the topologies into the 20 classes recently described by the Crispin laboratory, adding two categories (sulfated and unoccupied) that we refer to here as the Oxford classification (Table S8) (Watanabe et al., 2020a). Among other features observed, this classification allowed us to observe that although most sites with high-mannose structures were dominated by the Man5GlcNAc2 structure, N0234 and N0717 were dominated by the higher Man structures of Man8GlcNAc2 and Man7GlcNAc2, respectively (Figure S7; Table S8). Limited processing at N0234 is in agreement with a recent report suggesting that high-mannose structures at this site help to stabilize the receptor-binding domain of S (www.biorxiv.org/content/10.1101/2020.06.11.146522v1). Furthermore, applying the Oxford classifications to our dataset clearly demonstrates that the three most C-terminal sites (N1158, N1173, and N1194), dominated by complex-type glycans, were more often further processed (i.e., multiple antennae) and elaborated (i.e., galactosylation and sialylation) than other sites (Table S8).\nFigure 3 SARS-CoV-2 S Immunogen N-glycan Sites Are Predominantly Modified by Complex N-glycans\nN-glycan topologies were assigned to all 22 sites of the S protomer and the spectral counts for each of the three types of N-glycans (high-mannose, hybrid, and complex), as well as the unoccupied peptide spectral match counts at each site, were summed and visualized as pie charts. Note that only N1173 and N1194 show an appreciable amount of the unoccupied amino acid."}
LitCovid-sample-sentences
{"project":"LitCovid-sample-sentences","denotations":[{"id":"T109","span":{"begin":0,"end":162},"obj":"Sentence"},{"id":"T110","span":{"begin":163,"end":463},"obj":"Sentence"},{"id":"T111","span":{"begin":464,"end":777},"obj":"Sentence"},{"id":"T112","span":{"begin":778,"end":886},"obj":"Sentence"},{"id":"T113","span":{"begin":887,"end":1014},"obj":"Sentence"},{"id":"T114","span":{"begin":1015,"end":1105},"obj":"Sentence"},{"id":"T115","span":{"begin":1106,"end":1296},"obj":"Sentence"},{"id":"T116","span":{"begin":1297,"end":1451},"obj":"Sentence"},{"id":"T117","span":{"begin":1452,"end":1649},"obj":"Sentence"},{"id":"T118","span":{"begin":1650,"end":1917},"obj":"Sentence"},{"id":"T119","span":{"begin":1918,"end":2016},"obj":"Sentence"},{"id":"T120","span":{"begin":2017,"end":2232},"obj":"Sentence"},{"id":"T121","span":{"begin":2233,"end":2479},"obj":"Sentence"},{"id":"T122","span":{"begin":2480,"end":2546},"obj":"Sentence"},{"id":"T123","span":{"begin":2547,"end":2677},"obj":"Sentence"},{"id":"T124","span":{"begin":2678,"end":2948},"obj":"Sentence"},{"id":"T125","span":{"begin":2949,"end":3248},"obj":"Sentence"},{"id":"T126","span":{"begin":3249,"end":3472},"obj":"Sentence"},{"id":"T127","span":{"begin":3473,"end":3800},"obj":"Sentence"},{"id":"T128","span":{"begin":3801,"end":3895},"obj":"Sentence"},{"id":"T129","span":{"begin":3896,"end":4177},"obj":"Sentence"},{"id":"T130","span":{"begin":4178,"end":4265},"obj":"Sentence"}],"namespaces":[{"prefix":"_base","uri":"http://pubannotation.org/ontology/tao.owl#"}],"text":"Next, we applied three different proteolytic digestion strategies to the SARS-CoV-2 S immunogen to maximize glycopeptide coverage by subsequent LC-MS/MS analyses. Extended gradient nanoflow reverse-phase LC-MS/MS was carried out on a ThermoFisher Lumos Tribrid instrument using Step-HCD fragmentation on each of the samples (see STAR Methods for details, as well as Duan et al., 2018; Escolano et al., 2019; Wang et al., 2020; Yu et al., 2018; Zhou et al., 2017). After data analyses using pGlyco 2.2.2 (Liu et al., 2017), Byonic (Bern et al., 2012), and manual validation of glycan compositions against our released glycomics findings (Figure 2A; Tables S3 and S13), we were able to determine the microheterogeneity at each of the 22 canonical sites (Figures 2B–2E; Table S6). Notably, none of the non-canonical consensus sequences, including N0501, displayed any quantifiable glycans. The N-glycosites N0074 (Figure 2B) and N0149 (Figure 2C) are highly processed and display a typical mammalian N-glycan profile. N0149 is, however, modified with several hybrid N-glycan structures, whereas N0074 is not. N0234 (Figure 2D) and N0801 (Figure 2E) have N-glycan profiles more similar to those found on other viruses such as HIV (Watanabe et al., 2019) that are dominated by high-mannose structures. N0234 (Figure 2D) displays an abundance of Man7-Man9 high-mannose structures, suggesting stalled processing by early-acting ER and cis-Golgi mannosidases. In contrast, N0801 (Figure 2E) is processed more efficiently to Man5 high-mannose and hybrid structures, suggesting that access to the glycan at this site by MGAT1 and α-Mannosidase II is hindered. In general, for all 22 sites (Figures 2B–2E; Table S6), we observed underprocessing of complex glycan antennae (i.e., under-galactosylation and under-sialylation) and a high degree of core fucosylation in agreement with released glycan analyses (Figure 2A; Table S3). We also observed a small percent of sulfated N-linked glycans at several sites (Tables S6 and S8). Based on the assignments and the spectral counts for each topology, we were able to determine the percent of total N-linked glycan types (high-mannose, hybrid, or complex) present at each site (Figure 3 ; Table S7). Notably, three of the sites (N0234, N0709, and N0717) displayed more than 50% high-mannose glycans, whereas 11 other sites (N0017, N0074, N0149, N0165, N0282, N0331, N0657, N1134, N1158, N1173, and N1194) were more than 90% complex when occupied. The other eight sites were distributed between these two extremes. Notably, only one site (N0717 at 45%), which also had greater than 50% high mannose (55%), had greater than 33% hybrid structures. To further evaluate the heterogeneity, we grouped all the topologies into the 20 classes recently described by the Crispin laboratory, adding two categories (sulfated and unoccupied) that we refer to here as the Oxford classification (Table S8) (Watanabe et al., 2020a). Among other features observed, this classification allowed us to observe that although most sites with high-mannose structures were dominated by the Man5GlcNAc2 structure, N0234 and N0717 were dominated by the higher Man structures of Man8GlcNAc2 and Man7GlcNAc2, respectively (Figure S7; Table S8). Limited processing at N0234 is in agreement with a recent report suggesting that high-mannose structures at this site help to stabilize the receptor-binding domain of S (www.biorxiv.org/content/10.1101/2020.06.11.146522v1). Furthermore, applying the Oxford classifications to our dataset clearly demonstrates that the three most C-terminal sites (N1158, N1173, and N1194), dominated by complex-type glycans, were more often further processed (i.e., multiple antennae) and elaborated (i.e., galactosylation and sialylation) than other sites (Table S8).\nFigure 3 SARS-CoV-2 S Immunogen N-glycan Sites Are Predominantly Modified by Complex N-glycans\nN-glycan topologies were assigned to all 22 sites of the S protomer and the spectral counts for each of the three types of N-glycans (high-mannose, hybrid, and complex), as well as the unoccupied peptide spectral match counts at each site, were summed and visualized as pie charts. Note that only N1173 and N1194 show an appreciable amount of the unoccupied amino acid."}
LitCovid-sample-PD-MONDO
{"project":"LitCovid-sample-PD-MONDO","denotations":[{"id":"T44","span":{"begin":73,"end":83},"obj":"Disease"},{"id":"T45","span":{"begin":329,"end":333},"obj":"Disease"},{"id":"T46","span":{"begin":3810,"end":3820},"obj":"Disease"}],"attributes":[{"id":"A46","pred":"mondo_id","subj":"T46","obj":"http://purl.obolibrary.org/obo/MONDO_0100096"},{"id":"A45","pred":"mondo_id","subj":"T45","obj":"http://purl.obolibrary.org/obo/MONDO_0010408"},{"id":"A44","pred":"mondo_id","subj":"T44","obj":"http://purl.obolibrary.org/obo/MONDO_0100096"}],"text":"Next, we applied three different proteolytic digestion strategies to the SARS-CoV-2 S immunogen to maximize glycopeptide coverage by subsequent LC-MS/MS analyses. Extended gradient nanoflow reverse-phase LC-MS/MS was carried out on a ThermoFisher Lumos Tribrid instrument using Step-HCD fragmentation on each of the samples (see STAR Methods for details, as well as Duan et al., 2018; Escolano et al., 2019; Wang et al., 2020; Yu et al., 2018; Zhou et al., 2017). After data analyses using pGlyco 2.2.2 (Liu et al., 2017), Byonic (Bern et al., 2012), and manual validation of glycan compositions against our released glycomics findings (Figure 2A; Tables S3 and S13), we were able to determine the microheterogeneity at each of the 22 canonical sites (Figures 2B–2E; Table S6). Notably, none of the non-canonical consensus sequences, including N0501, displayed any quantifiable glycans. The N-glycosites N0074 (Figure 2B) and N0149 (Figure 2C) are highly processed and display a typical mammalian N-glycan profile. N0149 is, however, modified with several hybrid N-glycan structures, whereas N0074 is not. N0234 (Figure 2D) and N0801 (Figure 2E) have N-glycan profiles more similar to those found on other viruses such as HIV (Watanabe et al., 2019) that are dominated by high-mannose structures. N0234 (Figure 2D) displays an abundance of Man7-Man9 high-mannose structures, suggesting stalled processing by early-acting ER and cis-Golgi mannosidases. In contrast, N0801 (Figure 2E) is processed more efficiently to Man5 high-mannose and hybrid structures, suggesting that access to the glycan at this site by MGAT1 and α-Mannosidase II is hindered. In general, for all 22 sites (Figures 2B–2E; Table S6), we observed underprocessing of complex glycan antennae (i.e., under-galactosylation and under-sialylation) and a high degree of core fucosylation in agreement with released glycan analyses (Figure 2A; Table S3). We also observed a small percent of sulfated N-linked glycans at several sites (Tables S6 and S8). Based on the assignments and the spectral counts for each topology, we were able to determine the percent of total N-linked glycan types (high-mannose, hybrid, or complex) present at each site (Figure 3 ; Table S7). Notably, three of the sites (N0234, N0709, and N0717) displayed more than 50% high-mannose glycans, whereas 11 other sites (N0017, N0074, N0149, N0165, N0282, N0331, N0657, N1134, N1158, N1173, and N1194) were more than 90% complex when occupied. The other eight sites were distributed between these two extremes. Notably, only one site (N0717 at 45%), which also had greater than 50% high mannose (55%), had greater than 33% hybrid structures. To further evaluate the heterogeneity, we grouped all the topologies into the 20 classes recently described by the Crispin laboratory, adding two categories (sulfated and unoccupied) that we refer to here as the Oxford classification (Table S8) (Watanabe et al., 2020a). Among other features observed, this classification allowed us to observe that although most sites with high-mannose structures were dominated by the Man5GlcNAc2 structure, N0234 and N0717 were dominated by the higher Man structures of Man8GlcNAc2 and Man7GlcNAc2, respectively (Figure S7; Table S8). Limited processing at N0234 is in agreement with a recent report suggesting that high-mannose structures at this site help to stabilize the receptor-binding domain of S (www.biorxiv.org/content/10.1101/2020.06.11.146522v1). Furthermore, applying the Oxford classifications to our dataset clearly demonstrates that the three most C-terminal sites (N1158, N1173, and N1194), dominated by complex-type glycans, were more often further processed (i.e., multiple antennae) and elaborated (i.e., galactosylation and sialylation) than other sites (Table S8).\nFigure 3 SARS-CoV-2 S Immunogen N-glycan Sites Are Predominantly Modified by Complex N-glycans\nN-glycan topologies were assigned to all 22 sites of the S protomer and the spectral counts for each of the three types of N-glycans (high-mannose, hybrid, and complex), as well as the unoccupied peptide spectral match counts at each site, were summed and visualized as pie charts. Note that only N1173 and N1194 show an appreciable amount of the unoccupied amino acid."}
LitCovid-sample-UniProt
{"project":"LitCovid-sample-UniProt","denotations":[{"id":"T1760","span":{"begin":329,"end":333},"obj":"Protein"},{"id":"T1784","span":{"begin":1610,"end":1615},"obj":"Protein"}],"attributes":[{"id":"A1760","pred":"uniprot_id","subj":"T1760","obj":"https://www.uniprot.org/uniprot/Q9GMD0"},{"id":"A1761","pred":"uniprot_id","subj":"T1760","obj":"https://www.uniprot.org/uniprot/Q9DG10"},{"id":"A1762","pred":"uniprot_id","subj":"T1760","obj":"https://www.uniprot.org/uniprot/Q9DG09"},{"id":"A1763","pred":"uniprot_id","subj":"T1760","obj":"https://www.uniprot.org/uniprot/Q9DG08"},{"id":"A1764","pred":"uniprot_id","subj":"T1760","obj":"https://www.uniprot.org/uniprot/Q9DEB4"},{"id":"A1765","pred":"uniprot_id","subj":"T1760","obj":"https://www.uniprot.org/uniprot/Q9DE06"},{"id":"A1766","pred":"uniprot_id","subj":"T1760","obj":"https://www.uniprot.org/uniprot/Q9D2G1"},{"id":"A1767","pred":"uniprot_id","subj":"T1760","obj":"https://www.uniprot.org/uniprot/Q95259"},{"id":"A1768","pred":"uniprot_id","subj":"T1760","obj":"https://www.uniprot.org/uniprot/Q924Y5"},{"id":"A1769","pred":"uniprot_id","subj":"T1760","obj":"https://www.uniprot.org/uniprot/Q543A5"},{"id":"A1770","pred":"uniprot_id","subj":"T1760","obj":"https://www.uniprot.org/uniprot/Q53Z33"},{"id":"A1771","pred":"uniprot_id","subj":"T1760","obj":"https://www.uniprot.org/uniprot/Q501Q7"},{"id":"A1772","pred":"uniprot_id","subj":"T1760","obj":"https://www.uniprot.org/uniprot/Q2YDI1"},{"id":"A1773","pred":"uniprot_id","subj":"T1760","obj":"https://www.uniprot.org/uniprot/Q28996"},{"id":"A1774","pred":"uniprot_id","subj":"T1760","obj":"https://www.uniprot.org/uniprot/Q28918"},{"id":"A1775","pred":"uniprot_id","subj":"T1760","obj":"https://www.uniprot.org/uniprot/Q16396"},{"id":"A1776","pred":"uniprot_id","subj":"T1760","obj":"https://www.uniprot.org/uniprot/P97826"},{"id":"A1777","pred":"uniprot_id","subj":"T1760","obj":"https://www.uniprot.org/uniprot/P97628"},{"id":"A1778","pred":"uniprot_id","subj":"T1760","obj":"https://www.uniprot.org/uniprot/P79245"},{"id":"A1779","pred":"uniprot_id","subj":"T1760","obj":"https://www.uniprot.org/uniprot/P70114"},{"id":"A1780","pred":"uniprot_id","subj":"T1760","obj":"https://www.uniprot.org/uniprot/P58864"},{"id":"A1781","pred":"uniprot_id","subj":"T1760","obj":"https://www.uniprot.org/uniprot/P51557"},{"id":"A1782","pred":"uniprot_id","subj":"T1760","obj":"https://www.uniprot.org/uniprot/P49675"},{"id":"A1783","pred":"uniprot_id","subj":"T1760","obj":"https://www.uniprot.org/uniprot/O46689"},{"id":"A1784","pred":"uniprot_id","subj":"T1784","obj":"https://www.uniprot.org/uniprot/Q9DCL0"},{"id":"A1785","pred":"uniprot_id","subj":"T1784","obj":"https://www.uniprot.org/uniprot/Q96PD6"},{"id":"A1786","pred":"uniprot_id","subj":"T1784","obj":"https://www.uniprot.org/uniprot/Q91ZV4"},{"id":"A1787","pred":"uniprot_id","subj":"T1784","obj":"https://www.uniprot.org/uniprot/Q7ZXC3"},{"id":"A1788","pred":"uniprot_id","subj":"T1784","obj":"https://www.uniprot.org/uniprot/Q70VZ7"},{"id":"A1789","pred":"uniprot_id","subj":"T1784","obj":"https://www.uniprot.org/uniprot/Q6IEE5"},{"id":"A1790","pred":"uniprot_id","subj":"T1784","obj":"https://www.uniprot.org/uniprot/Q3KPP4"},{"id":"A1791","pred":"uniprot_id","subj":"T1784","obj":"https://www.uniprot.org/uniprot/Q28C88"},{"id":"A1792","pred":"uniprot_id","subj":"T1784","obj":"https://www.uniprot.org/uniprot/E9QLA2"}],"text":"Next, we applied three different proteolytic digestion strategies to the SARS-CoV-2 S immunogen to maximize glycopeptide coverage by subsequent LC-MS/MS analyses. Extended gradient nanoflow reverse-phase LC-MS/MS was carried out on a ThermoFisher Lumos Tribrid instrument using Step-HCD fragmentation on each of the samples (see STAR Methods for details, as well as Duan et al., 2018; Escolano et al., 2019; Wang et al., 2020; Yu et al., 2018; Zhou et al., 2017). After data analyses using pGlyco 2.2.2 (Liu et al., 2017), Byonic (Bern et al., 2012), and manual validation of glycan compositions against our released glycomics findings (Figure 2A; Tables S3 and S13), we were able to determine the microheterogeneity at each of the 22 canonical sites (Figures 2B–2E; Table S6). Notably, none of the non-canonical consensus sequences, including N0501, displayed any quantifiable glycans. The N-glycosites N0074 (Figure 2B) and N0149 (Figure 2C) are highly processed and display a typical mammalian N-glycan profile. N0149 is, however, modified with several hybrid N-glycan structures, whereas N0074 is not. N0234 (Figure 2D) and N0801 (Figure 2E) have N-glycan profiles more similar to those found on other viruses such as HIV (Watanabe et al., 2019) that are dominated by high-mannose structures. N0234 (Figure 2D) displays an abundance of Man7-Man9 high-mannose structures, suggesting stalled processing by early-acting ER and cis-Golgi mannosidases. In contrast, N0801 (Figure 2E) is processed more efficiently to Man5 high-mannose and hybrid structures, suggesting that access to the glycan at this site by MGAT1 and α-Mannosidase II is hindered. In general, for all 22 sites (Figures 2B–2E; Table S6), we observed underprocessing of complex glycan antennae (i.e., under-galactosylation and under-sialylation) and a high degree of core fucosylation in agreement with released glycan analyses (Figure 2A; Table S3). We also observed a small percent of sulfated N-linked glycans at several sites (Tables S6 and S8). Based on the assignments and the spectral counts for each topology, we were able to determine the percent of total N-linked glycan types (high-mannose, hybrid, or complex) present at each site (Figure 3 ; Table S7). Notably, three of the sites (N0234, N0709, and N0717) displayed more than 50% high-mannose glycans, whereas 11 other sites (N0017, N0074, N0149, N0165, N0282, N0331, N0657, N1134, N1158, N1173, and N1194) were more than 90% complex when occupied. The other eight sites were distributed between these two extremes. Notably, only one site (N0717 at 45%), which also had greater than 50% high mannose (55%), had greater than 33% hybrid structures. To further evaluate the heterogeneity, we grouped all the topologies into the 20 classes recently described by the Crispin laboratory, adding two categories (sulfated and unoccupied) that we refer to here as the Oxford classification (Table S8) (Watanabe et al., 2020a). Among other features observed, this classification allowed us to observe that although most sites with high-mannose structures were dominated by the Man5GlcNAc2 structure, N0234 and N0717 were dominated by the higher Man structures of Man8GlcNAc2 and Man7GlcNAc2, respectively (Figure S7; Table S8). Limited processing at N0234 is in agreement with a recent report suggesting that high-mannose structures at this site help to stabilize the receptor-binding domain of S (www.biorxiv.org/content/10.1101/2020.06.11.146522v1). Furthermore, applying the Oxford classifications to our dataset clearly demonstrates that the three most C-terminal sites (N1158, N1173, and N1194), dominated by complex-type glycans, were more often further processed (i.e., multiple antennae) and elaborated (i.e., galactosylation and sialylation) than other sites (Table S8).\nFigure 3 SARS-CoV-2 S Immunogen N-glycan Sites Are Predominantly Modified by Complex N-glycans\nN-glycan topologies were assigned to all 22 sites of the S protomer and the spectral counts for each of the three types of N-glycans (high-mannose, hybrid, and complex), as well as the unoccupied peptide spectral match counts at each site, were summed and visualized as pie charts. Note that only N1173 and N1194 show an appreciable amount of the unoccupied amino acid."}
LitCovid-sample-PD-IDO
{"project":"LitCovid-sample-PD-IDO","denotations":[{"id":"T60","span":{"begin":745,"end":750},"obj":"http://purl.obolibrary.org/obo/BFO_0000029"},{"id":"T61","span":{"begin":1206,"end":1213},"obj":"http://purl.obolibrary.org/obo/NCBITaxon_10239"},{"id":"T62","span":{"begin":1602,"end":1606},"obj":"http://purl.obolibrary.org/obo/BFO_0000029"},{"id":"T63","span":{"begin":1673,"end":1678},"obj":"http://purl.obolibrary.org/obo/BFO_0000029"},{"id":"T64","span":{"begin":1991,"end":1996},"obj":"http://purl.obolibrary.org/obo/BFO_0000029"},{"id":"T65","span":{"begin":2205,"end":2209},"obj":"http://purl.obolibrary.org/obo/BFO_0000029"},{"id":"T66","span":{"begin":2255,"end":2260},"obj":"http://purl.obolibrary.org/obo/BFO_0000029"},{"id":"T67","span":{"begin":2350,"end":2355},"obj":"http://purl.obolibrary.org/obo/BFO_0000029"},{"id":"T68","span":{"begin":2496,"end":2501},"obj":"http://purl.obolibrary.org/obo/BFO_0000029"},{"id":"T69","span":{"begin":2565,"end":2569},"obj":"http://purl.obolibrary.org/obo/BFO_0000029"},{"id":"T70","span":{"begin":3041,"end":3046},"obj":"http://purl.obolibrary.org/obo/BFO_0000029"},{"id":"T71","span":{"begin":3362,"end":3366},"obj":"http://purl.obolibrary.org/obo/BFO_0000029"},{"id":"T72","span":{"begin":3589,"end":3594},"obj":"http://purl.obolibrary.org/obo/BFO_0000029"},{"id":"T73","span":{"begin":3783,"end":3788},"obj":"http://purl.obolibrary.org/obo/BFO_0000029"},{"id":"T74","span":{"begin":3842,"end":3847},"obj":"http://purl.obolibrary.org/obo/BFO_0000029"},{"id":"T75","span":{"begin":3940,"end":3945},"obj":"http://purl.obolibrary.org/obo/BFO_0000029"},{"id":"T76","span":{"begin":4130,"end":4134},"obj":"http://purl.obolibrary.org/obo/BFO_0000029"}],"text":"Next, we applied three different proteolytic digestion strategies to the SARS-CoV-2 S immunogen to maximize glycopeptide coverage by subsequent LC-MS/MS analyses. Extended gradient nanoflow reverse-phase LC-MS/MS was carried out on a ThermoFisher Lumos Tribrid instrument using Step-HCD fragmentation on each of the samples (see STAR Methods for details, as well as Duan et al., 2018; Escolano et al., 2019; Wang et al., 2020; Yu et al., 2018; Zhou et al., 2017). After data analyses using pGlyco 2.2.2 (Liu et al., 2017), Byonic (Bern et al., 2012), and manual validation of glycan compositions against our released glycomics findings (Figure 2A; Tables S3 and S13), we were able to determine the microheterogeneity at each of the 22 canonical sites (Figures 2B–2E; Table S6). Notably, none of the non-canonical consensus sequences, including N0501, displayed any quantifiable glycans. The N-glycosites N0074 (Figure 2B) and N0149 (Figure 2C) are highly processed and display a typical mammalian N-glycan profile. N0149 is, however, modified with several hybrid N-glycan structures, whereas N0074 is not. N0234 (Figure 2D) and N0801 (Figure 2E) have N-glycan profiles more similar to those found on other viruses such as HIV (Watanabe et al., 2019) that are dominated by high-mannose structures. N0234 (Figure 2D) displays an abundance of Man7-Man9 high-mannose structures, suggesting stalled processing by early-acting ER and cis-Golgi mannosidases. In contrast, N0801 (Figure 2E) is processed more efficiently to Man5 high-mannose and hybrid structures, suggesting that access to the glycan at this site by MGAT1 and α-Mannosidase II is hindered. In general, for all 22 sites (Figures 2B–2E; Table S6), we observed underprocessing of complex glycan antennae (i.e., under-galactosylation and under-sialylation) and a high degree of core fucosylation in agreement with released glycan analyses (Figure 2A; Table S3). We also observed a small percent of sulfated N-linked glycans at several sites (Tables S6 and S8). Based on the assignments and the spectral counts for each topology, we were able to determine the percent of total N-linked glycan types (high-mannose, hybrid, or complex) present at each site (Figure 3 ; Table S7). Notably, three of the sites (N0234, N0709, and N0717) displayed more than 50% high-mannose glycans, whereas 11 other sites (N0017, N0074, N0149, N0165, N0282, N0331, N0657, N1134, N1158, N1173, and N1194) were more than 90% complex when occupied. The other eight sites were distributed between these two extremes. Notably, only one site (N0717 at 45%), which also had greater than 50% high mannose (55%), had greater than 33% hybrid structures. To further evaluate the heterogeneity, we grouped all the topologies into the 20 classes recently described by the Crispin laboratory, adding two categories (sulfated and unoccupied) that we refer to here as the Oxford classification (Table S8) (Watanabe et al., 2020a). Among other features observed, this classification allowed us to observe that although most sites with high-mannose structures were dominated by the Man5GlcNAc2 structure, N0234 and N0717 were dominated by the higher Man structures of Man8GlcNAc2 and Man7GlcNAc2, respectively (Figure S7; Table S8). Limited processing at N0234 is in agreement with a recent report suggesting that high-mannose structures at this site help to stabilize the receptor-binding domain of S (www.biorxiv.org/content/10.1101/2020.06.11.146522v1). Furthermore, applying the Oxford classifications to our dataset clearly demonstrates that the three most C-terminal sites (N1158, N1173, and N1194), dominated by complex-type glycans, were more often further processed (i.e., multiple antennae) and elaborated (i.e., galactosylation and sialylation) than other sites (Table S8).\nFigure 3 SARS-CoV-2 S Immunogen N-glycan Sites Are Predominantly Modified by Complex N-glycans\nN-glycan topologies were assigned to all 22 sites of the S protomer and the spectral counts for each of the three types of N-glycans (high-mannose, hybrid, and complex), as well as the unoccupied peptide spectral match counts at each site, were summed and visualized as pie charts. Note that only N1173 and N1194 show an appreciable amount of the unoccupied amino acid."}
LitCovid-sample-PD-FMA
{"project":"LitCovid-sample-PD-FMA","denotations":[{"id":"T76","span":{"begin":108,"end":120},"obj":"Body_part"},{"id":"T77","span":{"begin":1222,"end":1225},"obj":"Body_part"},{"id":"T78","span":{"begin":1277,"end":1284},"obj":"Body_part"},{"id":"T79","span":{"begin":1355,"end":1362},"obj":"Body_part"},{"id":"T80","span":{"begin":1526,"end":1533},"obj":"Body_part"},{"id":"T81","span":{"begin":2160,"end":2167},"obj":"Body_part"},{"id":"T82","span":{"begin":2316,"end":2323},"obj":"Body_part"},{"id":"T83","span":{"begin":2623,"end":2630},"obj":"Body_part"},{"id":"T84","span":{"begin":3057,"end":3064},"obj":"Body_part"},{"id":"T85","span":{"begin":3335,"end":3342},"obj":"Body_part"},{"id":"T86","span":{"begin":4035,"end":4042},"obj":"Body_part"},{"id":"T87","span":{"begin":4254,"end":4264},"obj":"Body_part"}],"attributes":[{"id":"A86","pred":"fma_id","subj":"T86","obj":"http://purl.org/sig/ont/fma/fma82801"},{"id":"A80","pred":"fma_id","subj":"T80","obj":"http://purl.org/sig/ont/fma/fma82801"},{"id":"A79","pred":"fma_id","subj":"T79","obj":"http://purl.org/sig/ont/fma/fma82801"},{"id":"A76","pred":"fma_id","subj":"T76","obj":"http://purl.org/sig/ont/fma/fma82784"},{"id":"A78","pred":"fma_id","subj":"T78","obj":"http://purl.org/sig/ont/fma/fma82801"},{"id":"A81","pred":"fma_id","subj":"T81","obj":"http://purl.org/sig/ont/fma/fma82801"},{"id":"A82","pred":"fma_id","subj":"T82","obj":"http://purl.org/sig/ont/fma/fma82801"},{"id":"A77","pred":"fma_id","subj":"T77","obj":"http://purl.org/sig/ont/fma/fma278683"},{"id":"A83","pred":"fma_id","subj":"T83","obj":"http://purl.org/sig/ont/fma/fma82801"},{"id":"A84","pred":"fma_id","subj":"T84","obj":"http://purl.org/sig/ont/fma/fma82801"},{"id":"A87","pred":"fma_id","subj":"T87","obj":"http://purl.org/sig/ont/fma/fma82739"},{"id":"A85","pred":"fma_id","subj":"T85","obj":"http://purl.org/sig/ont/fma/fma82801"}],"text":"Next, we applied three different proteolytic digestion strategies to the SARS-CoV-2 S immunogen to maximize glycopeptide coverage by subsequent LC-MS/MS analyses. Extended gradient nanoflow reverse-phase LC-MS/MS was carried out on a ThermoFisher Lumos Tribrid instrument using Step-HCD fragmentation on each of the samples (see STAR Methods for details, as well as Duan et al., 2018; Escolano et al., 2019; Wang et al., 2020; Yu et al., 2018; Zhou et al., 2017). After data analyses using pGlyco 2.2.2 (Liu et al., 2017), Byonic (Bern et al., 2012), and manual validation of glycan compositions against our released glycomics findings (Figure 2A; Tables S3 and S13), we were able to determine the microheterogeneity at each of the 22 canonical sites (Figures 2B–2E; Table S6). Notably, none of the non-canonical consensus sequences, including N0501, displayed any quantifiable glycans. The N-glycosites N0074 (Figure 2B) and N0149 (Figure 2C) are highly processed and display a typical mammalian N-glycan profile. N0149 is, however, modified with several hybrid N-glycan structures, whereas N0074 is not. N0234 (Figure 2D) and N0801 (Figure 2E) have N-glycan profiles more similar to those found on other viruses such as HIV (Watanabe et al., 2019) that are dominated by high-mannose structures. N0234 (Figure 2D) displays an abundance of Man7-Man9 high-mannose structures, suggesting stalled processing by early-acting ER and cis-Golgi mannosidases. In contrast, N0801 (Figure 2E) is processed more efficiently to Man5 high-mannose and hybrid structures, suggesting that access to the glycan at this site by MGAT1 and α-Mannosidase II is hindered. In general, for all 22 sites (Figures 2B–2E; Table S6), we observed underprocessing of complex glycan antennae (i.e., under-galactosylation and under-sialylation) and a high degree of core fucosylation in agreement with released glycan analyses (Figure 2A; Table S3). We also observed a small percent of sulfated N-linked glycans at several sites (Tables S6 and S8). Based on the assignments and the spectral counts for each topology, we were able to determine the percent of total N-linked glycan types (high-mannose, hybrid, or complex) present at each site (Figure 3 ; Table S7). Notably, three of the sites (N0234, N0709, and N0717) displayed more than 50% high-mannose glycans, whereas 11 other sites (N0017, N0074, N0149, N0165, N0282, N0331, N0657, N1134, N1158, N1173, and N1194) were more than 90% complex when occupied. The other eight sites were distributed between these two extremes. Notably, only one site (N0717 at 45%), which also had greater than 50% high mannose (55%), had greater than 33% hybrid structures. To further evaluate the heterogeneity, we grouped all the topologies into the 20 classes recently described by the Crispin laboratory, adding two categories (sulfated and unoccupied) that we refer to here as the Oxford classification (Table S8) (Watanabe et al., 2020a). Among other features observed, this classification allowed us to observe that although most sites with high-mannose structures were dominated by the Man5GlcNAc2 structure, N0234 and N0717 were dominated by the higher Man structures of Man8GlcNAc2 and Man7GlcNAc2, respectively (Figure S7; Table S8). Limited processing at N0234 is in agreement with a recent report suggesting that high-mannose structures at this site help to stabilize the receptor-binding domain of S (www.biorxiv.org/content/10.1101/2020.06.11.146522v1). Furthermore, applying the Oxford classifications to our dataset clearly demonstrates that the three most C-terminal sites (N1158, N1173, and N1194), dominated by complex-type glycans, were more often further processed (i.e., multiple antennae) and elaborated (i.e., galactosylation and sialylation) than other sites (Table S8).\nFigure 3 SARS-CoV-2 S Immunogen N-glycan Sites Are Predominantly Modified by Complex N-glycans\nN-glycan topologies were assigned to all 22 sites of the S protomer and the spectral counts for each of the three types of N-glycans (high-mannose, hybrid, and complex), as well as the unoccupied peptide spectral match counts at each site, were summed and visualized as pie charts. Note that only N1173 and N1194 show an appreciable amount of the unoccupied amino acid."}
LitCovid-sample-PD-MAT
{"project":"LitCovid-sample-PD-MAT","denotations":[{"id":"T3","span":{"begin":1752,"end":1760},"obj":"http://purl.obolibrary.org/obo/MAT_0000086"},{"id":"T4","span":{"begin":3707,"end":3715},"obj":"http://purl.obolibrary.org/obo/MAT_0000086"}],"text":"Next, we applied three different proteolytic digestion strategies to the SARS-CoV-2 S immunogen to maximize glycopeptide coverage by subsequent LC-MS/MS analyses. Extended gradient nanoflow reverse-phase LC-MS/MS was carried out on a ThermoFisher Lumos Tribrid instrument using Step-HCD fragmentation on each of the samples (see STAR Methods for details, as well as Duan et al., 2018; Escolano et al., 2019; Wang et al., 2020; Yu et al., 2018; Zhou et al., 2017). After data analyses using pGlyco 2.2.2 (Liu et al., 2017), Byonic (Bern et al., 2012), and manual validation of glycan compositions against our released glycomics findings (Figure 2A; Tables S3 and S13), we were able to determine the microheterogeneity at each of the 22 canonical sites (Figures 2B–2E; Table S6). Notably, none of the non-canonical consensus sequences, including N0501, displayed any quantifiable glycans. The N-glycosites N0074 (Figure 2B) and N0149 (Figure 2C) are highly processed and display a typical mammalian N-glycan profile. N0149 is, however, modified with several hybrid N-glycan structures, whereas N0074 is not. N0234 (Figure 2D) and N0801 (Figure 2E) have N-glycan profiles more similar to those found on other viruses such as HIV (Watanabe et al., 2019) that are dominated by high-mannose structures. N0234 (Figure 2D) displays an abundance of Man7-Man9 high-mannose structures, suggesting stalled processing by early-acting ER and cis-Golgi mannosidases. In contrast, N0801 (Figure 2E) is processed more efficiently to Man5 high-mannose and hybrid structures, suggesting that access to the glycan at this site by MGAT1 and α-Mannosidase II is hindered. In general, for all 22 sites (Figures 2B–2E; Table S6), we observed underprocessing of complex glycan antennae (i.e., under-galactosylation and under-sialylation) and a high degree of core fucosylation in agreement with released glycan analyses (Figure 2A; Table S3). We also observed a small percent of sulfated N-linked glycans at several sites (Tables S6 and S8). Based on the assignments and the spectral counts for each topology, we were able to determine the percent of total N-linked glycan types (high-mannose, hybrid, or complex) present at each site (Figure 3 ; Table S7). Notably, three of the sites (N0234, N0709, and N0717) displayed more than 50% high-mannose glycans, whereas 11 other sites (N0017, N0074, N0149, N0165, N0282, N0331, N0657, N1134, N1158, N1173, and N1194) were more than 90% complex when occupied. The other eight sites were distributed between these two extremes. Notably, only one site (N0717 at 45%), which also had greater than 50% high mannose (55%), had greater than 33% hybrid structures. To further evaluate the heterogeneity, we grouped all the topologies into the 20 classes recently described by the Crispin laboratory, adding two categories (sulfated and unoccupied) that we refer to here as the Oxford classification (Table S8) (Watanabe et al., 2020a). Among other features observed, this classification allowed us to observe that although most sites with high-mannose structures were dominated by the Man5GlcNAc2 structure, N0234 and N0717 were dominated by the higher Man structures of Man8GlcNAc2 and Man7GlcNAc2, respectively (Figure S7; Table S8). Limited processing at N0234 is in agreement with a recent report suggesting that high-mannose structures at this site help to stabilize the receptor-binding domain of S (www.biorxiv.org/content/10.1101/2020.06.11.146522v1). Furthermore, applying the Oxford classifications to our dataset clearly demonstrates that the three most C-terminal sites (N1158, N1173, and N1194), dominated by complex-type glycans, were more often further processed (i.e., multiple antennae) and elaborated (i.e., galactosylation and sialylation) than other sites (Table S8).\nFigure 3 SARS-CoV-2 S Immunogen N-glycan Sites Are Predominantly Modified by Complex N-glycans\nN-glycan topologies were assigned to all 22 sites of the S protomer and the spectral counts for each of the three types of N-glycans (high-mannose, hybrid, and complex), as well as the unoccupied peptide spectral match counts at each site, were summed and visualized as pie charts. Note that only N1173 and N1194 show an appreciable amount of the unoccupied amino acid."}
LitCovid-sample-PD-GO-BP-0
{"project":"LitCovid-sample-PD-GO-BP-0","denotations":[{"id":"T57","span":{"begin":45,"end":54},"obj":"http://purl.obolibrary.org/obo/GO_0007586"},{"id":"T58","span":{"begin":1800,"end":1811},"obj":"http://purl.obolibrary.org/obo/GO_0097503"},{"id":"T59","span":{"begin":1839,"end":1851},"obj":"http://purl.obolibrary.org/obo/GO_0036065"},{"id":"T60","span":{"begin":3759,"end":3770},"obj":"http://purl.obolibrary.org/obo/GO_0097503"}],"text":"Next, we applied three different proteolytic digestion strategies to the SARS-CoV-2 S immunogen to maximize glycopeptide coverage by subsequent LC-MS/MS analyses. Extended gradient nanoflow reverse-phase LC-MS/MS was carried out on a ThermoFisher Lumos Tribrid instrument using Step-HCD fragmentation on each of the samples (see STAR Methods for details, as well as Duan et al., 2018; Escolano et al., 2019; Wang et al., 2020; Yu et al., 2018; Zhou et al., 2017). After data analyses using pGlyco 2.2.2 (Liu et al., 2017), Byonic (Bern et al., 2012), and manual validation of glycan compositions against our released glycomics findings (Figure 2A; Tables S3 and S13), we were able to determine the microheterogeneity at each of the 22 canonical sites (Figures 2B–2E; Table S6). Notably, none of the non-canonical consensus sequences, including N0501, displayed any quantifiable glycans. The N-glycosites N0074 (Figure 2B) and N0149 (Figure 2C) are highly processed and display a typical mammalian N-glycan profile. N0149 is, however, modified with several hybrid N-glycan structures, whereas N0074 is not. N0234 (Figure 2D) and N0801 (Figure 2E) have N-glycan profiles more similar to those found on other viruses such as HIV (Watanabe et al., 2019) that are dominated by high-mannose structures. N0234 (Figure 2D) displays an abundance of Man7-Man9 high-mannose structures, suggesting stalled processing by early-acting ER and cis-Golgi mannosidases. In contrast, N0801 (Figure 2E) is processed more efficiently to Man5 high-mannose and hybrid structures, suggesting that access to the glycan at this site by MGAT1 and α-Mannosidase II is hindered. In general, for all 22 sites (Figures 2B–2E; Table S6), we observed underprocessing of complex glycan antennae (i.e., under-galactosylation and under-sialylation) and a high degree of core fucosylation in agreement with released glycan analyses (Figure 2A; Table S3). We also observed a small percent of sulfated N-linked glycans at several sites (Tables S6 and S8). Based on the assignments and the spectral counts for each topology, we were able to determine the percent of total N-linked glycan types (high-mannose, hybrid, or complex) present at each site (Figure 3 ; Table S7). Notably, three of the sites (N0234, N0709, and N0717) displayed more than 50% high-mannose glycans, whereas 11 other sites (N0017, N0074, N0149, N0165, N0282, N0331, N0657, N1134, N1158, N1173, and N1194) were more than 90% complex when occupied. The other eight sites were distributed between these two extremes. Notably, only one site (N0717 at 45%), which also had greater than 50% high mannose (55%), had greater than 33% hybrid structures. To further evaluate the heterogeneity, we grouped all the topologies into the 20 classes recently described by the Crispin laboratory, adding two categories (sulfated and unoccupied) that we refer to here as the Oxford classification (Table S8) (Watanabe et al., 2020a). Among other features observed, this classification allowed us to observe that although most sites with high-mannose structures were dominated by the Man5GlcNAc2 structure, N0234 and N0717 were dominated by the higher Man structures of Man8GlcNAc2 and Man7GlcNAc2, respectively (Figure S7; Table S8). Limited processing at N0234 is in agreement with a recent report suggesting that high-mannose structures at this site help to stabilize the receptor-binding domain of S (www.biorxiv.org/content/10.1101/2020.06.11.146522v1). Furthermore, applying the Oxford classifications to our dataset clearly demonstrates that the three most C-terminal sites (N1158, N1173, and N1194), dominated by complex-type glycans, were more often further processed (i.e., multiple antennae) and elaborated (i.e., galactosylation and sialylation) than other sites (Table S8).\nFigure 3 SARS-CoV-2 S Immunogen N-glycan Sites Are Predominantly Modified by Complex N-glycans\nN-glycan topologies were assigned to all 22 sites of the S protomer and the spectral counts for each of the three types of N-glycans (high-mannose, hybrid, and complex), as well as the unoccupied peptide spectral match counts at each site, were summed and visualized as pie charts. Note that only N1173 and N1194 show an appreciable amount of the unoccupied amino acid."}
LitCovid-sample-GO-BP
{"project":"LitCovid-sample-GO-BP","denotations":[{"id":"T53","span":{"begin":45,"end":54},"obj":"http://purl.obolibrary.org/obo/GO_0007586"},{"id":"T54","span":{"begin":1800,"end":1811},"obj":"http://purl.obolibrary.org/obo/GO_0097503"},{"id":"T55","span":{"begin":1839,"end":1851},"obj":"http://purl.obolibrary.org/obo/GO_0036065"},{"id":"T56","span":{"begin":3759,"end":3770},"obj":"http://purl.obolibrary.org/obo/GO_0097503"}],"text":"Next, we applied three different proteolytic digestion strategies to the SARS-CoV-2 S immunogen to maximize glycopeptide coverage by subsequent LC-MS/MS analyses. Extended gradient nanoflow reverse-phase LC-MS/MS was carried out on a ThermoFisher Lumos Tribrid instrument using Step-HCD fragmentation on each of the samples (see STAR Methods for details, as well as Duan et al., 2018; Escolano et al., 2019; Wang et al., 2020; Yu et al., 2018; Zhou et al., 2017). After data analyses using pGlyco 2.2.2 (Liu et al., 2017), Byonic (Bern et al., 2012), and manual validation of glycan compositions against our released glycomics findings (Figure 2A; Tables S3 and S13), we were able to determine the microheterogeneity at each of the 22 canonical sites (Figures 2B–2E; Table S6). Notably, none of the non-canonical consensus sequences, including N0501, displayed any quantifiable glycans. The N-glycosites N0074 (Figure 2B) and N0149 (Figure 2C) are highly processed and display a typical mammalian N-glycan profile. N0149 is, however, modified with several hybrid N-glycan structures, whereas N0074 is not. N0234 (Figure 2D) and N0801 (Figure 2E) have N-glycan profiles more similar to those found on other viruses such as HIV (Watanabe et al., 2019) that are dominated by high-mannose structures. N0234 (Figure 2D) displays an abundance of Man7-Man9 high-mannose structures, suggesting stalled processing by early-acting ER and cis-Golgi mannosidases. In contrast, N0801 (Figure 2E) is processed more efficiently to Man5 high-mannose and hybrid structures, suggesting that access to the glycan at this site by MGAT1 and α-Mannosidase II is hindered. In general, for all 22 sites (Figures 2B–2E; Table S6), we observed underprocessing of complex glycan antennae (i.e., under-galactosylation and under-sialylation) and a high degree of core fucosylation in agreement with released glycan analyses (Figure 2A; Table S3). We also observed a small percent of sulfated N-linked glycans at several sites (Tables S6 and S8). Based on the assignments and the spectral counts for each topology, we were able to determine the percent of total N-linked glycan types (high-mannose, hybrid, or complex) present at each site (Figure 3 ; Table S7). Notably, three of the sites (N0234, N0709, and N0717) displayed more than 50% high-mannose glycans, whereas 11 other sites (N0017, N0074, N0149, N0165, N0282, N0331, N0657, N1134, N1158, N1173, and N1194) were more than 90% complex when occupied. The other eight sites were distributed between these two extremes. Notably, only one site (N0717 at 45%), which also had greater than 50% high mannose (55%), had greater than 33% hybrid structures. To further evaluate the heterogeneity, we grouped all the topologies into the 20 classes recently described by the Crispin laboratory, adding two categories (sulfated and unoccupied) that we refer to here as the Oxford classification (Table S8) (Watanabe et al., 2020a). Among other features observed, this classification allowed us to observe that although most sites with high-mannose structures were dominated by the Man5GlcNAc2 structure, N0234 and N0717 were dominated by the higher Man structures of Man8GlcNAc2 and Man7GlcNAc2, respectively (Figure S7; Table S8). Limited processing at N0234 is in agreement with a recent report suggesting that high-mannose structures at this site help to stabilize the receptor-binding domain of S (www.biorxiv.org/content/10.1101/2020.06.11.146522v1). Furthermore, applying the Oxford classifications to our dataset clearly demonstrates that the three most C-terminal sites (N1158, N1173, and N1194), dominated by complex-type glycans, were more often further processed (i.e., multiple antennae) and elaborated (i.e., galactosylation and sialylation) than other sites (Table S8).\nFigure 3 SARS-CoV-2 S Immunogen N-glycan Sites Are Predominantly Modified by Complex N-glycans\nN-glycan topologies were assigned to all 22 sites of the S protomer and the spectral counts for each of the three types of N-glycans (high-mannose, hybrid, and complex), as well as the unoccupied peptide spectral match counts at each site, were summed and visualized as pie charts. Note that only N1173 and N1194 show an appreciable amount of the unoccupied amino acid."}
LitCovid-sample-Glycan
{"project":"LitCovid-sample-Glycan","denotations":[{"id":"T49","span":{"begin":1277,"end":1284},"obj":"http://rdf.glyconavi.org/CarTNa/CarTNa218/trivialname"},{"id":"T50","span":{"begin":1355,"end":1362},"obj":"http://rdf.glyconavi.org/CarTNa/CarTNa218/trivialname"},{"id":"T51","span":{"begin":1526,"end":1533},"obj":"http://rdf.glyconavi.org/CarTNa/CarTNa218/trivialname"},{"id":"T52","span":{"begin":2160,"end":2167},"obj":"http://rdf.glyconavi.org/CarTNa/CarTNa218/trivialname"},{"id":"T53","span":{"begin":2316,"end":2323},"obj":"http://rdf.glyconavi.org/CarTNa/CarTNa218/trivialname"},{"id":"T54","span":{"begin":2623,"end":2630},"obj":"http://rdf.glyconavi.org/CarTNa/CarTNa218/trivialname"},{"id":"T55","span":{"begin":3057,"end":3064},"obj":"http://rdf.glyconavi.org/CarTNa/CarTNa218/trivialname"},{"id":"T56","span":{"begin":3166,"end":3169},"obj":"https://glytoucan.org/Structures/Glycans/G70765DS"},{"id":"T57","span":{"begin":3166,"end":3169},"obj":"https://glytoucan.org/Structures/Glycans/G18477VG"},{"id":"T58","span":{"begin":3166,"end":3169},"obj":"https://glytoucan.org/Structures/Glycans/G72867LN"},{"id":"T59","span":{"begin":3335,"end":3342},"obj":"http://rdf.glyconavi.org/CarTNa/CarTNa218/trivialname"},{"id":"T60","span":{"begin":4035,"end":4042},"obj":"http://rdf.glyconavi.org/CarTNa/CarTNa218/trivialname"}],"text":"Next, we applied three different proteolytic digestion strategies to the SARS-CoV-2 S immunogen to maximize glycopeptide coverage by subsequent LC-MS/MS analyses. Extended gradient nanoflow reverse-phase LC-MS/MS was carried out on a ThermoFisher Lumos Tribrid instrument using Step-HCD fragmentation on each of the samples (see STAR Methods for details, as well as Duan et al., 2018; Escolano et al., 2019; Wang et al., 2020; Yu et al., 2018; Zhou et al., 2017). After data analyses using pGlyco 2.2.2 (Liu et al., 2017), Byonic (Bern et al., 2012), and manual validation of glycan compositions against our released glycomics findings (Figure 2A; Tables S3 and S13), we were able to determine the microheterogeneity at each of the 22 canonical sites (Figures 2B–2E; Table S6). Notably, none of the non-canonical consensus sequences, including N0501, displayed any quantifiable glycans. The N-glycosites N0074 (Figure 2B) and N0149 (Figure 2C) are highly processed and display a typical mammalian N-glycan profile. N0149 is, however, modified with several hybrid N-glycan structures, whereas N0074 is not. N0234 (Figure 2D) and N0801 (Figure 2E) have N-glycan profiles more similar to those found on other viruses such as HIV (Watanabe et al., 2019) that are dominated by high-mannose structures. N0234 (Figure 2D) displays an abundance of Man7-Man9 high-mannose structures, suggesting stalled processing by early-acting ER and cis-Golgi mannosidases. In contrast, N0801 (Figure 2E) is processed more efficiently to Man5 high-mannose and hybrid structures, suggesting that access to the glycan at this site by MGAT1 and α-Mannosidase II is hindered. In general, for all 22 sites (Figures 2B–2E; Table S6), we observed underprocessing of complex glycan antennae (i.e., under-galactosylation and under-sialylation) and a high degree of core fucosylation in agreement with released glycan analyses (Figure 2A; Table S3). We also observed a small percent of sulfated N-linked glycans at several sites (Tables S6 and S8). Based on the assignments and the spectral counts for each topology, we were able to determine the percent of total N-linked glycan types (high-mannose, hybrid, or complex) present at each site (Figure 3 ; Table S7). Notably, three of the sites (N0234, N0709, and N0717) displayed more than 50% high-mannose glycans, whereas 11 other sites (N0017, N0074, N0149, N0165, N0282, N0331, N0657, N1134, N1158, N1173, and N1194) were more than 90% complex when occupied. The other eight sites were distributed between these two extremes. Notably, only one site (N0717 at 45%), which also had greater than 50% high mannose (55%), had greater than 33% hybrid structures. To further evaluate the heterogeneity, we grouped all the topologies into the 20 classes recently described by the Crispin laboratory, adding two categories (sulfated and unoccupied) that we refer to here as the Oxford classification (Table S8) (Watanabe et al., 2020a). Among other features observed, this classification allowed us to observe that although most sites with high-mannose structures were dominated by the Man5GlcNAc2 structure, N0234 and N0717 were dominated by the higher Man structures of Man8GlcNAc2 and Man7GlcNAc2, respectively (Figure S7; Table S8). Limited processing at N0234 is in agreement with a recent report suggesting that high-mannose structures at this site help to stabilize the receptor-binding domain of S (www.biorxiv.org/content/10.1101/2020.06.11.146522v1). Furthermore, applying the Oxford classifications to our dataset clearly demonstrates that the three most C-terminal sites (N1158, N1173, and N1194), dominated by complex-type glycans, were more often further processed (i.e., multiple antennae) and elaborated (i.e., galactosylation and sialylation) than other sites (Table S8).\nFigure 3 SARS-CoV-2 S Immunogen N-glycan Sites Are Predominantly Modified by Complex N-glycans\nN-glycan topologies were assigned to all 22 sites of the S protomer and the spectral counts for each of the three types of N-glycans (high-mannose, hybrid, and complex), as well as the unoccupied peptide spectral match counts at each site, were summed and visualized as pie charts. Note that only N1173 and N1194 show an appreciable amount of the unoccupied amino acid."}
2_test
{"project":"2_test","denotations":[{"id":"32841605-30076101-19659510","span":{"begin":379,"end":383},"obj":"30076101"},{"id":"32841605-31142836-19659511","span":{"begin":402,"end":406},"obj":"31142836"},{"id":"32841605-29677181-19659512","span":{"begin":438,"end":442},"obj":"29677181"},{"id":"32841605-28445724-19659513","span":{"begin":457,"end":461},"obj":"28445724"},{"id":"32841605-28874712-19659514","span":{"begin":516,"end":520},"obj":"28874712"},{"id":"32841605-31121217-19659515","span":{"begin":1244,"end":1248},"obj":"31121217"}],"text":"Next, we applied three different proteolytic digestion strategies to the SARS-CoV-2 S immunogen to maximize glycopeptide coverage by subsequent LC-MS/MS analyses. Extended gradient nanoflow reverse-phase LC-MS/MS was carried out on a ThermoFisher Lumos Tribrid instrument using Step-HCD fragmentation on each of the samples (see STAR Methods for details, as well as Duan et al., 2018; Escolano et al., 2019; Wang et al., 2020; Yu et al., 2018; Zhou et al., 2017). After data analyses using pGlyco 2.2.2 (Liu et al., 2017), Byonic (Bern et al., 2012), and manual validation of glycan compositions against our released glycomics findings (Figure 2A; Tables S3 and S13), we were able to determine the microheterogeneity at each of the 22 canonical sites (Figures 2B–2E; Table S6). Notably, none of the non-canonical consensus sequences, including N0501, displayed any quantifiable glycans. The N-glycosites N0074 (Figure 2B) and N0149 (Figure 2C) are highly processed and display a typical mammalian N-glycan profile. N0149 is, however, modified with several hybrid N-glycan structures, whereas N0074 is not. N0234 (Figure 2D) and N0801 (Figure 2E) have N-glycan profiles more similar to those found on other viruses such as HIV (Watanabe et al., 2019) that are dominated by high-mannose structures. N0234 (Figure 2D) displays an abundance of Man7-Man9 high-mannose structures, suggesting stalled processing by early-acting ER and cis-Golgi mannosidases. In contrast, N0801 (Figure 2E) is processed more efficiently to Man5 high-mannose and hybrid structures, suggesting that access to the glycan at this site by MGAT1 and α-Mannosidase II is hindered. In general, for all 22 sites (Figures 2B–2E; Table S6), we observed underprocessing of complex glycan antennae (i.e., under-galactosylation and under-sialylation) and a high degree of core fucosylation in agreement with released glycan analyses (Figure 2A; Table S3). We also observed a small percent of sulfated N-linked glycans at several sites (Tables S6 and S8). Based on the assignments and the spectral counts for each topology, we were able to determine the percent of total N-linked glycan types (high-mannose, hybrid, or complex) present at each site (Figure 3 ; Table S7). Notably, three of the sites (N0234, N0709, and N0717) displayed more than 50% high-mannose glycans, whereas 11 other sites (N0017, N0074, N0149, N0165, N0282, N0331, N0657, N1134, N1158, N1173, and N1194) were more than 90% complex when occupied. The other eight sites were distributed between these two extremes. Notably, only one site (N0717 at 45%), which also had greater than 50% high mannose (55%), had greater than 33% hybrid structures. To further evaluate the heterogeneity, we grouped all the topologies into the 20 classes recently described by the Crispin laboratory, adding two categories (sulfated and unoccupied) that we refer to here as the Oxford classification (Table S8) (Watanabe et al., 2020a). Among other features observed, this classification allowed us to observe that although most sites with high-mannose structures were dominated by the Man5GlcNAc2 structure, N0234 and N0717 were dominated by the higher Man structures of Man8GlcNAc2 and Man7GlcNAc2, respectively (Figure S7; Table S8). Limited processing at N0234 is in agreement with a recent report suggesting that high-mannose structures at this site help to stabilize the receptor-binding domain of S (www.biorxiv.org/content/10.1101/2020.06.11.146522v1). Furthermore, applying the Oxford classifications to our dataset clearly demonstrates that the three most C-terminal sites (N1158, N1173, and N1194), dominated by complex-type glycans, were more often further processed (i.e., multiple antennae) and elaborated (i.e., galactosylation and sialylation) than other sites (Table S8).\nFigure 3 SARS-CoV-2 S Immunogen N-glycan Sites Are Predominantly Modified by Complex N-glycans\nN-glycan topologies were assigned to all 22 sites of the S protomer and the spectral counts for each of the three types of N-glycans (high-mannose, hybrid, and complex), as well as the unoccupied peptide spectral match counts at each site, were summed and visualized as pie charts. Note that only N1173 and N1194 show an appreciable amount of the unoccupied amino acid."}