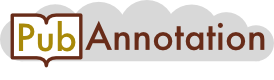
PMC:7432599 / 4898-25427
Annnotations
2_test
{"project":"2_test","denotations":[{"id":"32752280-21460597-52425998","span":{"begin":1767,"end":1769},"obj":"21460597"},{"id":"32752280-22021650-52425999","span":{"begin":2586,"end":2588},"obj":"22021650"},{"id":"32752280-28042652-52426000","span":{"begin":3037,"end":3039},"obj":"28042652"},{"id":"32752280-17416680-52426001","span":{"begin":4860,"end":4862},"obj":"17416680"},{"id":"32752280-28794154-52426002","span":{"begin":8263,"end":8265},"obj":"28794154"},{"id":"32752280-26627217-52426003","span":{"begin":8884,"end":8886},"obj":"26627217"},{"id":"32752280-28641779-52426004","span":{"begin":11794,"end":11796},"obj":"28641779"},{"id":"32752280-29514884-52426005","span":{"begin":13459,"end":13461},"obj":"29514884"},{"id":"32752280-17465682-52426006","span":{"begin":14034,"end":14036},"obj":"17465682"},{"id":"32752280-28641779-52426007","span":{"begin":14517,"end":14519},"obj":"28641779"},{"id":"32752280-18306430-52426008","span":{"begin":15804,"end":15806},"obj":"18306430"},{"id":"32752280-30271747-52426009","span":{"begin":17382,"end":17384},"obj":"30271747"},{"id":"32752280-27863448-52426010","span":{"begin":18456,"end":18458},"obj":"27863448"},{"id":"32752280-22739758-52426011","span":{"begin":18811,"end":18813},"obj":"22739758"},{"id":"32752280-25132496-52426012","span":{"begin":19493,"end":19495},"obj":"25132496"},{"id":"32752280-25173718-52426013","span":{"begin":20194,"end":20196},"obj":"25173718"},{"id":"T51286","span":{"begin":1767,"end":1769},"obj":"21460597"},{"id":"T655","span":{"begin":2586,"end":2588},"obj":"22021650"},{"id":"T24470","span":{"begin":3037,"end":3039},"obj":"28042652"},{"id":"T87938","span":{"begin":4860,"end":4862},"obj":"17416680"},{"id":"T75943","span":{"begin":8263,"end":8265},"obj":"28794154"},{"id":"T54166","span":{"begin":8884,"end":8886},"obj":"26627217"},{"id":"T3538","span":{"begin":11794,"end":11796},"obj":"28641779"},{"id":"T302","span":{"begin":13459,"end":13461},"obj":"29514884"},{"id":"T7443","span":{"begin":14034,"end":14036},"obj":"17465682"},{"id":"T59550","span":{"begin":14517,"end":14519},"obj":"28641779"},{"id":"T98644","span":{"begin":15804,"end":15806},"obj":"18306430"},{"id":"T5291","span":{"begin":17382,"end":17384},"obj":"30271747"},{"id":"T23424","span":{"begin":18456,"end":18458},"obj":"27863448"},{"id":"T78773","span":{"begin":18811,"end":18813},"obj":"22739758"},{"id":"T28876","span":{"begin":19493,"end":19495},"obj":"25132496"},{"id":"T4392","span":{"begin":20194,"end":20196},"obj":"25173718"}],"text":"2. Results\n\n2.1. Goat’s Milk Intake Attenuates Body Weight Gain, Increases Food Intake, and Modifies Body Composition and Serum Parameters in Mice Fed A High-Fat Diet\nTo evaluate the effect of goat milk intake in the development of obesity and its metabolic consequences, we fed mice with a control diet (control), a high fat diet (HF) or a HF diet supplemented with lyophilized milk from goats fed a conventional diet (HFCD), grazing (HFG) or a conventional diet supplemented with Acacia farnesiana pods (HFAF) for 14 weeks. As expected, at the end of the study, mice fed the HF diet showed a significant increase (p \u003c 0.05) in body weight with respect to those fed the control diet. Interestingly, the three groups of mice fed HF diet supplemented with goat milk (HFCD, HFG, and HFAF) presented similar body weight to those fed the control diet throughout the study period (Figure 1A,B). The lower final body weight of mice fed goat milk with respect to those fed the HF was not caused by a reduction in food or energy intake. As observed in Figure 1C, mice fed HFCD, HFG or HFAF had higher food intake than those fed the HF. Since HF diets are more energy-dense than the control diet, energy intake of mice fed either diet containing goat milk was higher than those fed control or HF diets (p \u003c 0.05) (Figure 1D). The increased body weight of HF mice was due to a significant increment in body fat mass (p \u003c 0.05) and reduced lean mass with respect to control (Figure 1E,F). Notably, mice fed goat’s milk presented no significant differences in fat and lean mass with respect to control except the HFCD group that had lower fat mass and higher lean mass than all other groups. Leptin is an adipose-derived hormone that is secreted in proportion to total body fat [13]. As expected, circulating leptin concentration was directly associated with fat mass in all groups (Figure 1G). To evaluate the effect of dietary goat milk in circulating lipids we determined serum triglycerides and cholesterol. As expected, HF mice presented higher serum triglycerides than control mice (p \u003c 0.05). Nevertheless, HFCD, HFG, and HFAF mice presented significantly lower triglycerides levels than HF mice (p \u003c 0.05), similar to those of control mice (Figure 1H).\nHigh-fat diet feeding did not increase serum cholesterol with respect to control mice. Only the HFG and HFAF diets presented a modest but significant increase in serum cholesterol with respect to all other groups (Figure 1I). However, neither group presented cholesterol concentration above reference values for C57BL/6 mice (130 ± 10) [14]. These results indicate that consumption of goat milk prevents fat accretion and body weight gain and decreases serum leptin and triglycerides levels of mice fed a HF diet despite increased energy intake.\n\n2.2. Goat’s Milk Consumption Prevents Insulin Resistance and Pancreatic Islets Hypertrophy in Mice Fed A High-Fat Diet\nChronic consumption of a high-fat diet induces metabolic derangements such as glucose intolerance and insulin resistance [15]. At the end of the study, fasting serum glucose was higher in the HF mice when compared to controls and mice fed HFCD (p \u003c 0.05) (Figure 2A). Mice fed HFG and HFAF had no significant difference in fasting glucose concentration with respect to HF or control groups. However, fasting serum insulin at the end of the study was significantly lower in all groups fed goat milk than in those fed the HF or control diets (Figure 2B). To determine whether goat milk modifies glucose homeostasis in mice fed a HF diet, we performed an intraperitoneal glucose tolerance test (IpGTT). As expected, mice fed HF presented a significant (p \u003c 0.05) decrease in glucose tolerance as observed by the higher area under the curve (AUC) when compared to control mice (Figure 2C,D).\nInterestingly, mice fed with either goat’s milk had the same glucose tolerance than control. Then, to examine whether the improved glucose tolerance observed in animals fed goat milk was associated with higher insulin sensitivity, we performed an intraperitoneal insulin tolerance test (ipITT). As expected, mice fed HF had a blunted response to exogenous insulin reflected by a higher AUC (Figure 2E,F).\nInterestingly, the glucose curve of mice fed goat’s milk during the ipITT challenge was lower than those fed HF and similar to that of control mice. The AUC of all groups fed goat milk had a trend to be lower than that of the HF, but only in HFAF was significantly lower than the other groups. The development of insulin resistance is accompanied with a parallel increase in insulin secretion in order to maintain euglycemia. Moreover, glucose and insulin are trophic factors that induces islet compensatory growth response to insulin resistance. Thus, persistently elevated glucose and insulin concentrations induces hypertrophy of pancreatic islets [16].\nConsistently with the aforementioned results, pancreatic islets size was higher in mice fed the HF diet than control, HFCD and HFAF (p \u003c 0.05) (Figure 2G,H). These results indicate that goat’s milk decreases HF-associated glucose intolerance, preventing hyperinsulinemia. Notably, the lower insulin concentration of mice fed goat milk was associated with a decrease in HF-induced pancreatic islet hypertrophy, since HFCD, HFG and HFAF mice presented pancreatic islets size similar to those from control mice. Altogether, these results indicate that goat’s milk intake attenuates glucose intolerance and hyperinsulinemia associated to a decrease in pancreatic islet size in mice fed a HF diet.\n\n2.3. Goat’s Milk Consumption Increases Whole-Body Energy Expenditure and Attenuates Metabolic Inflexibility by an Increase in UCP-1 in BAT and Oxidative Fibers in Muscle of Mice Fed A High-Fat Diet\nTo evaluate whether the lower body weight and fat mass, and the improvement on glucose tolerance observed in HF mice fed goat’s milk was associated with increased energy expenditure, we evaluated oxygen consumption by indirect calorimetry using a CLAMS system. As expected, HF mice had lower average oxygen consumption than control mice in both fasting and feeding conditions (Figure 3A,B). Interestingly, HFCD, HFG and HFAF mice presented significantly higher oxygen consumption than HF and control mice in fed conditions (Figure 3A,B), indicating an increase in energy expenditure. When we calculated oxygen consumption per Kg of lean mass, only de HFAF group had higher oxygen consumption than the rest of the groups (Figure 3C). In addition, a linear regression analysis showed that the slopes of change of VO2 (mL/h) per unit change of body weight (Figure 3D) or lean mass (Figure 3E) were similar between control, HF and HFCD groups but not for the HFG and HFAF groups. Moreover, when we evaluated the effect of goat’s milk on substrate utilization by calculating the respiratory exchange ratio (RER), control mice had a RER of 0.8 during fasting conditions (Figure 3F,G), which increased to 1.0 during the fed state indicating an adequate metabolic flexibility, that is the ability to change from fatty acids to glucose utilization when substrates become available during the feeding period. Conversely, the RER of HF mice remained close to 0.8 during the feeding period (Figure 3F,G), indicating the presence of metabolic inflexibility or the inability to change substrate utilization. HFCD, HFG, and HFAF mice presented a significantly higher RER than HF mice, indicating an improvement on substrate utilization. The lower RER of mice fed goat’s milk with respect to those fed the control diet indicates that these mice uses fatty acids as an energy substrate even during the feeding period. However, since these mice also presented increased energy expenditure, these results indicate that mice fed goat’s milk had augmented fat oxidation capacity with respect to those fed HF, preventing excessive fat deposition in adipose tissue or other organs.\nMaintenance of a stable body weight despite a positive energy balance in response to consumption of an energy-dense diet is achieved by increased energy expenditure. Non-shivering thermogenesis in brown adipose tissue (BAT) and mitochondrial oxidation in skeletal muscle are two high energy-demanding activities that increases energy expenditure [17]. To evaluate whether the increase on energy expenditure was associated with BAT remodeling and skeletal muscle mitochondrial content, we evaluated BAT morphology and lipid content and mitochondrial activity in skeletal muscle. BAT of mice fed the HF diet contained a majority of adipocytes with one single large lipid vacuole, in contrast with the BAT of control mice, composed of multilocular adipocytes with small lipid vacuoles (Figure 4A). The increase in lipid vacuoles size of brown adipocytes is named “whitening” and reflects an impaired capacity to oxidize substrates and thus, reduced thermogenic activity [18]. The mean vacuole size in brown adipocytes from mice fed the HF diet was significantly higher with respect to controls (Figure 4B). Interestingly, the mean lipid vacuoles size of BAT from mice fed goat’s milk were similar than that of the control mice. To evaluate if the reduced lipid content in BAT was associated with increased thermogenesis, we evaluated uncoupling protein 1 (UCP-1) abundance in BAT by immunohistochemistry. Notably, mice fed with either goat’s milk had higher expression (p \u003c 0.05) of UCP-1 with respect to mice fed the HF diet (Figure 4A,C). UCP-1 expression was also evaluated by western blot. UCP-1 protein abundance was higher (p \u003c 0.05) in BAT of mice fed the HFG and HFAF than in mice fed the HF diet (Figure 4D,E). With regard to skeletal muscle, BODIPY staining showed increased lipid content in muscle fibers of mice fed HF with respect to control (Figure 4F). Interestingly, lipid accumulation in muscle fibers of mice fed with HFCD, HFG and HFAF diets was significant (p \u003c 0.05) lower than those fed HF as determined by densitometric quantification of BODIPY staining (Figure 4G). Succinate dehydrogenase (SDH) staining is a measure of mitochondrial oxidative capacity. As expected, mice fed HF had significantly lower oxidative capacity than those fed the control diet (Figure 4F). Interestingly, mice fed with HFCD, HFG and HFAF diets presented significantly higher SDH enzyme activity in skeletal muscle than those fed HF and even higher than control (p \u003c 0.05) (Figure 4H). Mitochondrial biogenesis and activity in skeletal muscle is regulated by the combined action of the cellular energy sensor AMP-activated protein kinase (AMPK) and the nuclear receptor peroxisome proliferator-activated receptor delta (PPARδ) [19]. Interestingly, AMPK phosphorylation significantly increased in skeletal muscle of HFCD, HFG and HFAF when compared to HF and control mice (Figure 4I,J) despite no significant difference in the mRNA abundance of AMPK between groups (Figure 4K). Messenger RNA abundance of PPARδ in skeletal muscle was higher (p \u003c 0.05) in HFG and HFAF compared with the rest of dietary treatments (Figure 4L). These results indicate that goat’s milk intake increases oxidative metabolism in skeletal muscle and substrate oxidation in BAT, preventing body fat accumulation and body weight gain despite higher energy intake with respect to control or the HF group.\n\n2.4. Goat’s Milk Intake Prevent White Adipose Tissue Hypertrophy and Modulates Adipokine mRNA in Adipocytes of Mice Fed A High-Fat Diet\nChronic intake of high-energy diets induces adipose tissue expansion in order to efficiently store energy excess as triglycerides, preventing excessive lipid accumulation in other organs. However, macrophage activation in adipose tissue induces a local inflammatory response leading to a reduction in adipocyte proliferation and hypertrophy of existing adipocytes [20]. Histological analysis of subcutaneous and visceral adipose tissue sections revealed that the HF diet significantly increased mean adipocyte size in both adipose tissue depots with respect to control (Figure 5A,B,H). Despite the high-fat diets added with goat’s milk, were isoenergetic with respect to the HF diet; subcutaneous and visceral adipose tissues of mice fed goat’s milk presented adipocytes significantly smaller than those fed HF. Frequency distribution analysis revealed that 50% of adipocytes from control mice were smaller than 1000 µm whereas less than 25% of adipocytes from HF mice were in that size range (Figure 5C). Interestingly, as in control, more than 40% of adipocytes of mice fed HFCD, HFG or HFAF were smaller than 1000 µm. In visceral adipose tissue of HF mice, less than 35% of adipocytes were under 1500 µm, whereas in control mice and those fed goat’s milk more than 50% of adipocytes were under that range (Figure 5I). To determine if the smaller size of adipocytes from mice fed goat’s milk was associated with increased fatty acid lipolysis and thermogenesis we evaluated UCP-1 distribution and the activity of hormone sensitive lipase (HSL) in subcutaneous adipose tissue. UCP-1 expression in subcutaneous adipose tissue of all groups fed goat’s milk was higher with respect to the group fed HF (Figure 5A,D). The activity of HSL in all groups fed goat’s milk was higher (p \u003c 0.05) respect to control and HF diets (Figure 5E,F). These results indicate that goat’s milk intake increases the hydrolysis of triglycerides in white adipose tissue to sustain in situ UCP-1 mediated thermogenesis, preventing adipocyte hypertrophy [21]. As expected, a response to reduced adipocyte size is the reduction in leptin mRNA expression. Leptin mRNA content in HFCD, HFG and HFAF was higher than in control but significantly lower than in HF (Figure 5J). These results indicate that goat’s milk favors adipose tissue expansion during chronic energy intake, preventing adipocyte hypertrophy. Adipose tissue expandability in response to positive energy balance is mediated by the activity of the adipose-tissue specific transcription factor PPARγ2 which in turn stimulates adiponectin gene expression and secretion [22]. The mRNA abundance of PPARγ2 was significantly higher (p \u003c 0.05) in subcutaneous adipose tissue of mice fed HFCD, HFG or HFAF with respect to those fed control or HF (Figure 5K). Accordingly, adiponectin mRNA content in adipose tissue was also higher in subcutaneous adipose tissue of mice fed goat’s milk than in those fed control or HF diet (Figure 5L). On the contrary, hypertrophic adipose tissue is characterized by macrophage recruitment and increased cytokine secretion [20]. Immunohistochemistry of the macrophage marker F4780 revealed numerous macrophage infiltrates in adipose tissue of mice fed HF (Figure 5A). No positive staining was detected in adipose tissue sections of mice fed control or goat’s milk. However, tumor necrosis factor α content in adipose tissue homogenates was not significantly between diets (Figure 5M). These results indicate that goat’s milk intake can activate the nuclear receptor PPARγ2, increasing adiponectin gene expression preventing infiltration of macrophages in mice fed a HF diet.\n\n2.5. The Anti-Inflammatory Fatty Acid Profile of Goat Milk’s Prevents Hepatic Lipid Deposition and Inflammatory Markers and Increases AMPK Phosphorylation\nExcessive lipid deposition in liver induces cytotoxic cell death and inflammation, leading to the progression of non-alcoholic fatty liver disease to steatohepatitis. However, dietary fatty acid composition can modify hepatic lipid metabolism and the inflammatory response. Long-chain saturated fatty acids such as stearic acid favors lipid accumulation and inflammation whereas long-chain n-3 polyunsaturated fatty acids such as eicosapentaenoic (EPA), docosahexaenoic (DHA) and rumen bacteria-derived conjugated linoleic acid (CLA) exerts anti-inflammatory activities in liver [23].\nTo evaluate if the fatty acid profile in liver reflects that of experimental diets, we determined the fatty acid profile of experimental diets and liver. The most abundant saturated fatty acids in goat milk are the short-chain myristic (C14:0), capric (C8:0) and the long-chain stearic (C18:0). Regardless of the origin of the goat milk, all diets containing goat’s milk showed a greater amount of myristic and capric acids with respect to control or HF (Figure 6A,B). Moreover, the amount of stearic acid in diets containing goat’s milk was lower compared to the HF diet (Figure 6C). This pattern was reflected in the fatty acids present in the liver (Figure 6A–C). The level of the pro-inflammatory fatty acid arachidonic acid was lower in the diets containing goat’s milk than in HF or control (Figure 6D). Hepatic arachidonic acid levels presented the same pattern. Moreover, the levels of EPA and CLA were higher in the diets that contains goat milk with respect to HF or control (Figure 6E,F), which was reflected in the hepatic content of these fatty acids. These results indicate that hepatic fatty acid composition reflects the composition of dietary fatty acids and suggest that goat’s milk fatty acid profile could exert beneficial effects in liver such as reduced lipid accumulation and lower inflammation.\nThe primary source of hepatic fat due to calorie excess is de novo lipogenesis mediated by the activity of the sterol regulatory element binding protein-1c (SREBP-1c). This transcription factor mediates gene expression of lipogenic enzymes in the liver [24]. To evaluate the effect of goat milk intake on the development of fatty liver and SREBP-1c expression, H\u0026E and ORO staining and real-time PCR quantification of SREBP-1c mRNA were performed on liver samples from the different experimental groups. As expected, mice fed HF presented a high number of hepatocytes containing enlarged lipid vacuoles (Figure 6G). Accordingly, densitometric quantification of ORO staining revealed a significant increase in lipid content of liver of HF mice with respect to control (Figure 6H). Notably, mice fed with the HFCD, HFG and HFAF diets showed less fat accumulation in liver and cytostructural features similar to those of control mice. Messenger RNA abundance of SREBP-1c was higher in the liver of mice fed HF with respect to control (Figure 6I). Strikingly, SREBP-1c gene expression was lower in the liver of animals fed with HFCD, HFG and HFAF diets with respect to HF. The expression of SREBP-1c in liver increases in response to hyperinsulinemia/insulin resistance but is reduced by particular fatty acids such as EPA and DHA [25]. Accordingly, SREBP-1c mRNA abundance in liver was inversely correlated with hepatic DHA content (Figure 6J). Another beneficial effect of the fatty acids presents in goat’s milk, such as EPA is the activation of AMPK, the cellular energy sensor [26]. Upon activation, AMPK increases glucose and fatty acid oxidation, reducing lipid content in liver [27]. As observed in Figure 6K,L, HFG and HFAF increased AMPK phosphorylation in liver with respect to the rest of the experimental diets (Figure 6K). These results indicate that consumption of goat’s milk in mice fed a HF diet prevents hepatic steatosis in part by reducing SREBP-1c-mediated lipogenesis and, simultaneously, by increasing AMPK-mediated lipid oxidation.\nBesides the increase in lipogenesis, an increase in hepatic oxidative and endoplasmic reticulum stress are determining factors in the development of non-alcoholic fatty liver disease by activating JNK phosphorylation, a common downstream element in oxidative and endoplasmic reticulum stress signaling pathways [28]. As observed in Figure 6K,M, the abundance of phosphorylated JNK was higher in liver of mice fed HF with respect to control. Notably, HFCD, HFG and HFAF fed mice presented lower levels of p-JNK than those observed in HF. To evaluate if the reduced JNK phosphorylation was associated with lesser intrahepatic TNF-α content, we determined this cytokine in liver homogenates. Accordingly, TNF-α abundance was higher in liver homogenates of HF mice with respect to control, HFCD, HFG and HFAF (Figure 6N).\nFinally, we determined the hepatic EPA+DHA/AA ratio. This ratio is used as an anti-inflammatory index, where a higher ratio indicates a protective role in the development of inflammatory states [29]. Notably, mice fed goat’s milk presented a significant higher hepatic EPA+DHA/AA ratio with respect to those fed HF or control (Figure 6O). These results indicate that goat’s milk intake prevents hepatic inflammation in mice fed a HF associated to a protective proportion of n-3/n-6 long chain polyunsaturated fatty acids in liver."}