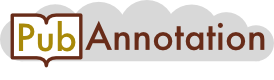
PMC:7152911 / 92026-99350
Annnotations
LitCovid-PubTator
{"project":"LitCovid-PubTator","denotations":[{"id":"1571","span":{"begin":1261,"end":1268},"obj":"Species"},{"id":"1572","span":{"begin":1387,"end":1394},"obj":"Species"},{"id":"1573","span":{"begin":1097,"end":1102},"obj":"Chemical"},{"id":"1574","span":{"begin":1106,"end":1113},"obj":"Chemical"},{"id":"1575","span":{"begin":1272,"end":1275},"obj":"Chemical"},{"id":"1576","span":{"begin":1292,"end":1294},"obj":"Chemical"},{"id":"1577","span":{"begin":1302,"end":1306},"obj":"Chemical"},{"id":"1578","span":{"begin":1358,"end":1366},"obj":"Chemical"},{"id":"1579","span":{"begin":1426,"end":1451},"obj":"Chemical"},{"id":"1586","span":{"begin":1708,"end":1717},"obj":"Gene"},{"id":"1587","span":{"begin":1767,"end":1769},"obj":"Gene"},{"id":"1588","span":{"begin":1593,"end":1616},"obj":"Species"},{"id":"1589","span":{"begin":1865,"end":1872},"obj":"Species"},{"id":"1590","span":{"begin":1877,"end":1886},"obj":"Species"},{"id":"1591","span":{"begin":1649,"end":1663},"obj":"Chemical"},{"id":"1599","span":{"begin":3280,"end":3288},"obj":"Gene"},{"id":"1600","span":{"begin":3157,"end":3165},"obj":"Gene"},{"id":"1601","span":{"begin":3200,"end":3207},"obj":"Species"},{"id":"1602","span":{"begin":3323,"end":3332},"obj":"Species"},{"id":"1603","span":{"begin":3575,"end":3589},"obj":"Species"},{"id":"1604","span":{"begin":3669,"end":3680},"obj":"Species"},{"id":"1605","span":{"begin":3415,"end":3421},"obj":"Chemical"},{"id":"1610","span":{"begin":2747,"end":2756},"obj":"Gene"},{"id":"1611","span":{"begin":2660,"end":2669},"obj":"Gene"},{"id":"1612","span":{"begin":2849,"end":2856},"obj":"Species"},{"id":"1613","span":{"begin":2870,"end":2877},"obj":"Chemical"},{"id":"1624","span":{"begin":5142,"end":5144},"obj":"Gene"},{"id":"1625","span":{"begin":4997,"end":4999},"obj":"Gene"},{"id":"1626","span":{"begin":4845,"end":4854},"obj":"Species"},{"id":"1627","span":{"begin":5252,"end":5261},"obj":"Species"},{"id":"1628","span":{"begin":4810,"end":4816},"obj":"Chemical"},{"id":"1629","span":{"begin":4939,"end":4941},"obj":"Chemical"},{"id":"1630","span":{"begin":5042,"end":5045},"obj":"Chemical"},{"id":"1631","span":{"begin":5067,"end":5074},"obj":"Chemical"},{"id":"1632","span":{"begin":5208,"end":5211},"obj":"Chemical"},{"id":"1633","span":{"begin":5219,"end":5225},"obj":"Chemical"},{"id":"1635","span":{"begin":5778,"end":5783},"obj":"Chemical"},{"id":"1649","span":{"begin":6903,"end":6905},"obj":"Gene"},{"id":"1650","span":{"begin":6885,"end":6887},"obj":"Gene"},{"id":"1651","span":{"begin":6871,"end":6873},"obj":"Gene"},{"id":"1652","span":{"begin":6293,"end":6295},"obj":"Gene"},{"id":"1653","span":{"begin":6266,"end":6273},"obj":"Species"},{"id":"1654","span":{"begin":6370,"end":6377},"obj":"Species"},{"id":"1655","span":{"begin":6819,"end":6833},"obj":"Species"},{"id":"1656","span":{"begin":6839,"end":6846},"obj":"Species"},{"id":"1657","span":{"begin":6804,"end":6817},"obj":"Species"},{"id":"1658","span":{"begin":6431,"end":6437},"obj":"Species"},{"id":"1659","span":{"begin":6218,"end":6225},"obj":"Chemical"},{"id":"1660","span":{"begin":6634,"end":6639},"obj":"Chemical"},{"id":"1661","span":{"begin":6701,"end":6704},"obj":"Chemical"}],"attributes":[{"id":"A1571","pred":"tao:has_database_id","subj":"1571","obj":"Tax:562"},{"id":"A1572","pred":"tao:has_database_id","subj":"1572","obj":"Tax:562"},{"id":"A1573","pred":"tao:has_database_id","subj":"1573","obj":"MESH:D008670"},{"id":"A1574","pred":"tao:has_database_id","subj":"1574","obj":"MESH:D011108"},{"id":"A1575","pred":"tao:has_database_id","subj":"1575","obj":"MESH:D011189"},{"id":"A1576","pred":"tao:has_database_id","subj":"1576","obj":"MESH:D010984"},{"id":"A1578","pred":"tao:has_database_id","subj":"1578","obj":"MESH:D006108"},{"id":"A1586","pred":"tao:has_database_id","subj":"1586","obj":"Gene:2960"},{"id":"A1587","pred":"tao:has_database_id","subj":"1587","obj":"Gene:21832"},{"id":"A1588","pred":"tao:has_database_id","subj":"1588","obj":"Tax:11320"},{"id":"A1589","pred":"tao:has_database_id","subj":"1589","obj":"Tax:562"},{"id":"A1590","pred":"tao:has_database_id","subj":"1590","obj":"Tax:1280"},{"id":"A1591","pred":"tao:has_database_id","subj":"1591","obj":"MESH:C000628730"},{"id":"A1599","pred":"tao:has_database_id","subj":"1599","obj":"Gene:2960"},{"id":"A1600","pred":"tao:has_database_id","subj":"1600","obj":"Gene:2960"},{"id":"A1601","pred":"tao:has_database_id","subj":"1601","obj":"Tax:562"},{"id":"A1602","pred":"tao:has_database_id","subj":"1602","obj":"Tax:1280"},{"id":"A1603","pred":"tao:has_database_id","subj":"1603","obj":"Tax:90371"},{"id":"A1604","pred":"tao:has_database_id","subj":"1604","obj":"Tax:1423"},{"id":"A1605","pred":"tao:has_database_id","subj":"1605","obj":"MESH:C054684"},{"id":"A1610","pred":"tao:has_database_id","subj":"1610","obj":"Gene:2960"},{"id":"A1611","pred":"tao:has_database_id","subj":"1611","obj":"Gene:2960"},{"id":"A1612","pred":"tao:has_database_id","subj":"1612","obj":"Tax:562"},{"id":"A1613","pred":"tao:has_database_id","subj":"1613","obj":"MESH:D011108"},{"id":"A1624","pred":"tao:has_database_id","subj":"1624","obj":"Gene:21832"},{"id":"A1625","pred":"tao:has_database_id","subj":"1625","obj":"Gene:21832"},{"id":"A1626","pred":"tao:has_database_id","subj":"1626","obj":"Tax:5807"},{"id":"A1627","pred":"tao:has_database_id","subj":"1627","obj":"Tax:1280"},{"id":"A1628","pred":"tao:has_database_id","subj":"1628","obj":"MESH:D002244"},{"id":"A1629","pred":"tao:has_database_id","subj":"1629","obj":"MESH:D006046"},{"id":"A1631","pred":"tao:has_database_id","subj":"1631","obj":"MESH:D000537"},{"id":"A1633","pred":"tao:has_database_id","subj":"1633","obj":"MESH:D002244"},{"id":"A1635","pred":"tao:has_database_id","subj":"1635","obj":"MESH:D008670"},{"id":"A1649","pred":"tao:has_database_id","subj":"1649","obj":"Gene:21832"},{"id":"A1650","pred":"tao:has_database_id","subj":"1650","obj":"Gene:21832"},{"id":"A1651","pred":"tao:has_database_id","subj":"1651","obj":"Gene:21832"},{"id":"A1652","pred":"tao:has_database_id","subj":"1652","obj":"Gene:21832"},{"id":"A1653","pred":"tao:has_database_id","subj":"1653","obj":"Tax:562"},{"id":"A1654","pred":"tao:has_database_id","subj":"1654","obj":"Tax:562"},{"id":"A1655","pred":"tao:has_database_id","subj":"1655","obj":"Tax:90371"},{"id":"A1656","pred":"tao:has_database_id","subj":"1656","obj":"Tax:562"},{"id":"A1657","pred":"tao:has_database_id","subj":"1657","obj":"Tax:197"},{"id":"A1658","pred":"tao:has_database_id","subj":"1658","obj":"Tax:562"},{"id":"A1659","pred":"tao:has_database_id","subj":"1659","obj":"MESH:D011108"},{"id":"A1660","pred":"tao:has_database_id","subj":"1660","obj":"MESH:D012834"}],"namespaces":[{"prefix":"Tax","uri":"https://www.ncbi.nlm.nih.gov/taxonomy/"},{"prefix":"MESH","uri":"https://id.nlm.nih.gov/mesh/"},{"prefix":"Gene","uri":"https://www.ncbi.nlm.nih.gov/gene/"},{"prefix":"CVCL","uri":"https://web.expasy.org/cellosaurus/CVCL_"}],"text":"3.3.2 Voltammetry\nVoltammetric methods, also referred to as controlled-potential methods, are those in which a current is measured in response to an applied electrical potential that drives redox reactions (Bard and Faulkner, 2000). The measured current is indicative of electron transfer within the sample and at the electrode surface, and thus, the concentration of the analyte. In chronoamperometry, the electrical potential at the working electrode is applied in steps, and the resulting current is measured as a function of time. The applied electrical potential can also be held constant or varied with time as the current is measured.\nAlthough various types of biosensors are compatible with voltammetry-based measurements, field-effect transistor (FET)-based biosensors often utilize amperometric-based methods for pathogen detection (Huang et al. 2011; Liu et al. 2013). FET biosensors detect pathogens via measured changes in source-drain channel conductivity that arise from the electric field of the sample environment. This is achieved by immobilizing biorecognition elements on the metal or polymer gate electrode of the device. He et al. showed that FETs based on PEDOT:PSS organic electrochemical transistor electrodes enabled the detection of E. coli in KCl solutions using Pt and Ag/AgCl gate electrodes (He et al. 2012). Wu et al. used a graphene-based FET to detect E. coli in nutrient broth diluted with phosphate buffered saline solution with amperometry using a Ag/AgCl gate electrode (Wu et al. 2016).\nFurther examples of amperometric sensing include the detection of human influenza A virus by Singh et al. using a reduced graphene oxide-based electrode and chronoamperometry using Fe(CN)6 3 - /4- at a LOD of 0.5 plaque-forming units (PFU)/mL (Singh et al. 2017b). Lee and Jun utilized wire-based electrodes for amperometric detection of E. coli and S. aureus (Lee and Jun 2016). A detailed list of studies that utilize amperometric methods for pathogen detection is provided in Table 1, Table 2\n\n3.3.2.1 Linear sweep and cyclic voltammetry\nLinear sweep voltammetry (LSV) methods are those in which a current is measured in response to an applied electrical potential that is swept at a constant rate across a range of electrical potentials (Bard and Faulkner, 2000). Cyclic voltammetry (CV) is a commonly used linear-sweep method in which the electrical potential is swept in both the forward and reverse directions in partial cycles, full cycles, or a series of cycles. CV is one of the most widely used voltammetric methods for pathogen detection.\nHong et al. used sweep voltammetry to detect norovirus in a sample solution with Fe(CN)6 3 - /4- extracted from lettuce (Hong et al. 2015). A typical CV response using Fe(CN)6 3 - /4- associated with pathogen detection is shown in Fig. 5 a for various concentrations of E. coli binding to a polymer composite electrode (Güner et al. 2017). A detailed overview of pathogen detection studies based on CV is provided in Table 1, Table 2.\nFig. 5 Typical responses associated with the common electrochemical methods used for pathogen detection. a) Cyclic voltammetry (CV) data using Fe(CN)63-/4- for varying concentrations of E. coli (Güner et al. 2017). b) Differential pulse voltammetry (DPV) data using Fe(CN)63-/4- for varying concentrations of S. aureus (Bhardwaj et al. 2017). c) Electrochemical impedance spectroscopy (EIS) in 100 mM LiClO4 solution in the form of a Nyquist plot and corresponding equivalent circuit model associated with biorecognition element immobilization and detection of S. typhimurium (Sheikhzadeh et al. 2016). d) Conductometry data for varying concentrations of B. subtilis (Yoo et al. 2017).\n\n3.3.2.2 Pulse voltammetry\nPulse voltammetry is a type of voltammetry in which the electrical potential is applied in pulses. The technique has the advantage of improved speed and sensitivity relative to traditional voltammetric techniques (Bard and Faulkner, 2000; Molina and González, 2016). In staircase voltammetry, the electrical potential is pulsed in a series of stair steps and the current is measured following each step change, which reduces the effect of capacitive charging on the current signal. Square wave voltammetry (SWV) is a type of staircase voltammetry that applies a symmetric square-wave pulse superimposed on a staircase potential waveform. The forward pulse of the waveform coincides with the staircase step. In differential pulse voltammetry (DPV), the electrical potential is scanned with a series of fixed amplitude pulses and superimposed on a changing base potential. The current is measured before the pulse application and again at the end of the pulse, which allows for the decay of the nonfaradaic current (Scott, 2016).\nFor example, Iqbal et al. used SWV with AuNP-modified carbon electrodes for detection of C. parvum in samples taken from fruit (Iqbal et al. 2015). Kitajima et al. also used SWV with Au microelectrodes to detect norovirus at a LOD of 10 PFU/mL (Kitajima et al. 2016). Cheng et al. used DPV and a nanostructured alumina electrode for detection of dengue type 2 virus with a LOD of 1 PFU/mL (Cheng et al. 2012). As shown in Fig. 5b, Bhardwaj et al. used DPV with a carbon-based electrode to detect S. aureus (Bhardwaj et al. 2017). Additional studies that utilize pulse voltammetry methods forpathogen detection are listed in Table 1, Table 2.\n\n3.3.2.3 Stripping voltammetry\nMany of the previously described voltammetric methods can be modified to include a step that pre-concentrates the target on the electrode surface. Subsequently, the pre-concentrated target is stripped from the surface by application of an electrical potential. In anodic stripping voltammetry (ASV), a negative potential is used to pre-concentrate metal ions onto the electrode surface. These ions are then stripped from the surface by applied positive potentials. Although most commonly used to detect trace amounts of metals, this method has been adapted for pathogen detection by electrocatalytically coating metallic labels on bound targets for oxidative stripping and subsequently measuring the current response (Abbaspour et al. 2015).\nChen et al. used stripping voltammetry with a polymer-CNT composite-based electrode to detect E. coli at a LOD of 13 CFU/mL (Chen et al. 2014). In that study, the biosensor was first incubated with E. coli. Silica-coated Ag nanoparticles conjugated with anti-E.coli were subsequently introduced to the system, inducing a binding reaction between the bacteria and the nanoparticles. After rinsing non-specifically bound particles, acid was introduced to dissolve Ag(s), and the resulting Ag+-rich solution was characterized using DPV. Viswanathan et al. used ASV with screen-printed composite electrodes for multiplexed detection of Campylobacter, S. typhimurium, and E. coli with a LOD of 400 cells/mL, 400 cells/mL, and 800 cells/mL, respectively (Viswanathan et al. 2012). In that study, antibody-functionalized nanocrystalline bioconjugates were first introduced to biosensor-bound bacteria, the specifically bound particles were dissolved with acid, and the ions were then stripped using a square-wave voltammetric waveform. Additional studies using stripping voltammetry for electrochemical detection of pathogens can be found in Table 1, Table 2."}
LitCovid-PD-FMA-UBERON
{"project":"LitCovid-PD-FMA-UBERON","denotations":[{"id":"T185","span":{"begin":1299,"end":1301},"obj":"Body_part"},{"id":"T186","span":{"begin":1486,"end":1488},"obj":"Body_part"},{"id":"T187","span":{"begin":6393,"end":6395},"obj":"Body_part"},{"id":"T188","span":{"begin":6634,"end":6636},"obj":"Body_part"},{"id":"T189","span":{"begin":6659,"end":6661},"obj":"Body_part"},{"id":"T190","span":{"begin":6865,"end":6870},"obj":"Body_part"},{"id":"T191","span":{"begin":6879,"end":6884},"obj":"Body_part"},{"id":"T192","span":{"begin":6897,"end":6902},"obj":"Body_part"},{"id":"T193","span":{"begin":6962,"end":6970},"obj":"Body_part"}],"attributes":[{"id":"A185","pred":"fma_id","subj":"T185","obj":"http://purl.org/sig/ont/fma/fma61898"},{"id":"A186","pred":"fma_id","subj":"T186","obj":"http://purl.org/sig/ont/fma/fma61898"},{"id":"A187","pred":"fma_id","subj":"T187","obj":"http://purl.org/sig/ont/fma/fma61898"},{"id":"A188","pred":"fma_id","subj":"T188","obj":"http://purl.org/sig/ont/fma/fma61898"},{"id":"A189","pred":"fma_id","subj":"T189","obj":"http://purl.org/sig/ont/fma/fma61898"},{"id":"A190","pred":"fma_id","subj":"T190","obj":"http://purl.org/sig/ont/fma/fma68646"},{"id":"A191","pred":"fma_id","subj":"T191","obj":"http://purl.org/sig/ont/fma/fma68646"},{"id":"A192","pred":"fma_id","subj":"T192","obj":"http://purl.org/sig/ont/fma/fma68646"},{"id":"A193","pred":"fma_id","subj":"T193","obj":"http://purl.org/sig/ont/fma/fma62871"}],"text":"3.3.2 Voltammetry\nVoltammetric methods, also referred to as controlled-potential methods, are those in which a current is measured in response to an applied electrical potential that drives redox reactions (Bard and Faulkner, 2000). The measured current is indicative of electron transfer within the sample and at the electrode surface, and thus, the concentration of the analyte. In chronoamperometry, the electrical potential at the working electrode is applied in steps, and the resulting current is measured as a function of time. The applied electrical potential can also be held constant or varied with time as the current is measured.\nAlthough various types of biosensors are compatible with voltammetry-based measurements, field-effect transistor (FET)-based biosensors often utilize amperometric-based methods for pathogen detection (Huang et al. 2011; Liu et al. 2013). FET biosensors detect pathogens via measured changes in source-drain channel conductivity that arise from the electric field of the sample environment. This is achieved by immobilizing biorecognition elements on the metal or polymer gate electrode of the device. He et al. showed that FETs based on PEDOT:PSS organic electrochemical transistor electrodes enabled the detection of E. coli in KCl solutions using Pt and Ag/AgCl gate electrodes (He et al. 2012). Wu et al. used a graphene-based FET to detect E. coli in nutrient broth diluted with phosphate buffered saline solution with amperometry using a Ag/AgCl gate electrode (Wu et al. 2016).\nFurther examples of amperometric sensing include the detection of human influenza A virus by Singh et al. using a reduced graphene oxide-based electrode and chronoamperometry using Fe(CN)6 3 - /4- at a LOD of 0.5 plaque-forming units (PFU)/mL (Singh et al. 2017b). Lee and Jun utilized wire-based electrodes for amperometric detection of E. coli and S. aureus (Lee and Jun 2016). A detailed list of studies that utilize amperometric methods for pathogen detection is provided in Table 1, Table 2\n\n3.3.2.1 Linear sweep and cyclic voltammetry\nLinear sweep voltammetry (LSV) methods are those in which a current is measured in response to an applied electrical potential that is swept at a constant rate across a range of electrical potentials (Bard and Faulkner, 2000). Cyclic voltammetry (CV) is a commonly used linear-sweep method in which the electrical potential is swept in both the forward and reverse directions in partial cycles, full cycles, or a series of cycles. CV is one of the most widely used voltammetric methods for pathogen detection.\nHong et al. used sweep voltammetry to detect norovirus in a sample solution with Fe(CN)6 3 - /4- extracted from lettuce (Hong et al. 2015). A typical CV response using Fe(CN)6 3 - /4- associated with pathogen detection is shown in Fig. 5 a for various concentrations of E. coli binding to a polymer composite electrode (Güner et al. 2017). A detailed overview of pathogen detection studies based on CV is provided in Table 1, Table 2.\nFig. 5 Typical responses associated with the common electrochemical methods used for pathogen detection. a) Cyclic voltammetry (CV) data using Fe(CN)63-/4- for varying concentrations of E. coli (Güner et al. 2017). b) Differential pulse voltammetry (DPV) data using Fe(CN)63-/4- for varying concentrations of S. aureus (Bhardwaj et al. 2017). c) Electrochemical impedance spectroscopy (EIS) in 100 mM LiClO4 solution in the form of a Nyquist plot and corresponding equivalent circuit model associated with biorecognition element immobilization and detection of S. typhimurium (Sheikhzadeh et al. 2016). d) Conductometry data for varying concentrations of B. subtilis (Yoo et al. 2017).\n\n3.3.2.2 Pulse voltammetry\nPulse voltammetry is a type of voltammetry in which the electrical potential is applied in pulses. The technique has the advantage of improved speed and sensitivity relative to traditional voltammetric techniques (Bard and Faulkner, 2000; Molina and González, 2016). In staircase voltammetry, the electrical potential is pulsed in a series of stair steps and the current is measured following each step change, which reduces the effect of capacitive charging on the current signal. Square wave voltammetry (SWV) is a type of staircase voltammetry that applies a symmetric square-wave pulse superimposed on a staircase potential waveform. The forward pulse of the waveform coincides with the staircase step. In differential pulse voltammetry (DPV), the electrical potential is scanned with a series of fixed amplitude pulses and superimposed on a changing base potential. The current is measured before the pulse application and again at the end of the pulse, which allows for the decay of the nonfaradaic current (Scott, 2016).\nFor example, Iqbal et al. used SWV with AuNP-modified carbon electrodes for detection of C. parvum in samples taken from fruit (Iqbal et al. 2015). Kitajima et al. also used SWV with Au microelectrodes to detect norovirus at a LOD of 10 PFU/mL (Kitajima et al. 2016). Cheng et al. used DPV and a nanostructured alumina electrode for detection of dengue type 2 virus with a LOD of 1 PFU/mL (Cheng et al. 2012). As shown in Fig. 5b, Bhardwaj et al. used DPV with a carbon-based electrode to detect S. aureus (Bhardwaj et al. 2017). Additional studies that utilize pulse voltammetry methods forpathogen detection are listed in Table 1, Table 2.\n\n3.3.2.3 Stripping voltammetry\nMany of the previously described voltammetric methods can be modified to include a step that pre-concentrates the target on the electrode surface. Subsequently, the pre-concentrated target is stripped from the surface by application of an electrical potential. In anodic stripping voltammetry (ASV), a negative potential is used to pre-concentrate metal ions onto the electrode surface. These ions are then stripped from the surface by applied positive potentials. Although most commonly used to detect trace amounts of metals, this method has been adapted for pathogen detection by electrocatalytically coating metallic labels on bound targets for oxidative stripping and subsequently measuring the current response (Abbaspour et al. 2015).\nChen et al. used stripping voltammetry with a polymer-CNT composite-based electrode to detect E. coli at a LOD of 13 CFU/mL (Chen et al. 2014). In that study, the biosensor was first incubated with E. coli. Silica-coated Ag nanoparticles conjugated with anti-E.coli were subsequently introduced to the system, inducing a binding reaction between the bacteria and the nanoparticles. After rinsing non-specifically bound particles, acid was introduced to dissolve Ag(s), and the resulting Ag+-rich solution was characterized using DPV. Viswanathan et al. used ASV with screen-printed composite electrodes for multiplexed detection of Campylobacter, S. typhimurium, and E. coli with a LOD of 400 cells/mL, 400 cells/mL, and 800 cells/mL, respectively (Viswanathan et al. 2012). In that study, antibody-functionalized nanocrystalline bioconjugates were first introduced to biosensor-bound bacteria, the specifically bound particles were dissolved with acid, and the ions were then stripped using a square-wave voltammetric waveform. Additional studies using stripping voltammetry for electrochemical detection of pathogens can be found in Table 1, Table 2."}
LitCovid-PD-MONDO
{"project":"LitCovid-PD-MONDO","denotations":[{"id":"T77","span":{"begin":1599,"end":1608},"obj":"Disease"},{"id":"T78","span":{"begin":5102,"end":5108},"obj":"Disease"}],"attributes":[{"id":"A77","pred":"mondo_id","subj":"T77","obj":"http://purl.obolibrary.org/obo/MONDO_0005812"},{"id":"A78","pred":"mondo_id","subj":"T78","obj":"http://purl.obolibrary.org/obo/MONDO_0005502"}],"text":"3.3.2 Voltammetry\nVoltammetric methods, also referred to as controlled-potential methods, are those in which a current is measured in response to an applied electrical potential that drives redox reactions (Bard and Faulkner, 2000). The measured current is indicative of electron transfer within the sample and at the electrode surface, and thus, the concentration of the analyte. In chronoamperometry, the electrical potential at the working electrode is applied in steps, and the resulting current is measured as a function of time. The applied electrical potential can also be held constant or varied with time as the current is measured.\nAlthough various types of biosensors are compatible with voltammetry-based measurements, field-effect transistor (FET)-based biosensors often utilize amperometric-based methods for pathogen detection (Huang et al. 2011; Liu et al. 2013). FET biosensors detect pathogens via measured changes in source-drain channel conductivity that arise from the electric field of the sample environment. This is achieved by immobilizing biorecognition elements on the metal or polymer gate electrode of the device. He et al. showed that FETs based on PEDOT:PSS organic electrochemical transistor electrodes enabled the detection of E. coli in KCl solutions using Pt and Ag/AgCl gate electrodes (He et al. 2012). Wu et al. used a graphene-based FET to detect E. coli in nutrient broth diluted with phosphate buffered saline solution with amperometry using a Ag/AgCl gate electrode (Wu et al. 2016).\nFurther examples of amperometric sensing include the detection of human influenza A virus by Singh et al. using a reduced graphene oxide-based electrode and chronoamperometry using Fe(CN)6 3 - /4- at a LOD of 0.5 plaque-forming units (PFU)/mL (Singh et al. 2017b). Lee and Jun utilized wire-based electrodes for amperometric detection of E. coli and S. aureus (Lee and Jun 2016). A detailed list of studies that utilize amperometric methods for pathogen detection is provided in Table 1, Table 2\n\n3.3.2.1 Linear sweep and cyclic voltammetry\nLinear sweep voltammetry (LSV) methods are those in which a current is measured in response to an applied electrical potential that is swept at a constant rate across a range of electrical potentials (Bard and Faulkner, 2000). Cyclic voltammetry (CV) is a commonly used linear-sweep method in which the electrical potential is swept in both the forward and reverse directions in partial cycles, full cycles, or a series of cycles. CV is one of the most widely used voltammetric methods for pathogen detection.\nHong et al. used sweep voltammetry to detect norovirus in a sample solution with Fe(CN)6 3 - /4- extracted from lettuce (Hong et al. 2015). A typical CV response using Fe(CN)6 3 - /4- associated with pathogen detection is shown in Fig. 5 a for various concentrations of E. coli binding to a polymer composite electrode (Güner et al. 2017). A detailed overview of pathogen detection studies based on CV is provided in Table 1, Table 2.\nFig. 5 Typical responses associated with the common electrochemical methods used for pathogen detection. a) Cyclic voltammetry (CV) data using Fe(CN)63-/4- for varying concentrations of E. coli (Güner et al. 2017). b) Differential pulse voltammetry (DPV) data using Fe(CN)63-/4- for varying concentrations of S. aureus (Bhardwaj et al. 2017). c) Electrochemical impedance spectroscopy (EIS) in 100 mM LiClO4 solution in the form of a Nyquist plot and corresponding equivalent circuit model associated with biorecognition element immobilization and detection of S. typhimurium (Sheikhzadeh et al. 2016). d) Conductometry data for varying concentrations of B. subtilis (Yoo et al. 2017).\n\n3.3.2.2 Pulse voltammetry\nPulse voltammetry is a type of voltammetry in which the electrical potential is applied in pulses. The technique has the advantage of improved speed and sensitivity relative to traditional voltammetric techniques (Bard and Faulkner, 2000; Molina and González, 2016). In staircase voltammetry, the electrical potential is pulsed in a series of stair steps and the current is measured following each step change, which reduces the effect of capacitive charging on the current signal. Square wave voltammetry (SWV) is a type of staircase voltammetry that applies a symmetric square-wave pulse superimposed on a staircase potential waveform. The forward pulse of the waveform coincides with the staircase step. In differential pulse voltammetry (DPV), the electrical potential is scanned with a series of fixed amplitude pulses and superimposed on a changing base potential. The current is measured before the pulse application and again at the end of the pulse, which allows for the decay of the nonfaradaic current (Scott, 2016).\nFor example, Iqbal et al. used SWV with AuNP-modified carbon electrodes for detection of C. parvum in samples taken from fruit (Iqbal et al. 2015). Kitajima et al. also used SWV with Au microelectrodes to detect norovirus at a LOD of 10 PFU/mL (Kitajima et al. 2016). Cheng et al. used DPV and a nanostructured alumina electrode for detection of dengue type 2 virus with a LOD of 1 PFU/mL (Cheng et al. 2012). As shown in Fig. 5b, Bhardwaj et al. used DPV with a carbon-based electrode to detect S. aureus (Bhardwaj et al. 2017). Additional studies that utilize pulse voltammetry methods forpathogen detection are listed in Table 1, Table 2.\n\n3.3.2.3 Stripping voltammetry\nMany of the previously described voltammetric methods can be modified to include a step that pre-concentrates the target on the electrode surface. Subsequently, the pre-concentrated target is stripped from the surface by application of an electrical potential. In anodic stripping voltammetry (ASV), a negative potential is used to pre-concentrate metal ions onto the electrode surface. These ions are then stripped from the surface by applied positive potentials. Although most commonly used to detect trace amounts of metals, this method has been adapted for pathogen detection by electrocatalytically coating metallic labels on bound targets for oxidative stripping and subsequently measuring the current response (Abbaspour et al. 2015).\nChen et al. used stripping voltammetry with a polymer-CNT composite-based electrode to detect E. coli at a LOD of 13 CFU/mL (Chen et al. 2014). In that study, the biosensor was first incubated with E. coli. Silica-coated Ag nanoparticles conjugated with anti-E.coli were subsequently introduced to the system, inducing a binding reaction between the bacteria and the nanoparticles. After rinsing non-specifically bound particles, acid was introduced to dissolve Ag(s), and the resulting Ag+-rich solution was characterized using DPV. Viswanathan et al. used ASV with screen-printed composite electrodes for multiplexed detection of Campylobacter, S. typhimurium, and E. coli with a LOD of 400 cells/mL, 400 cells/mL, and 800 cells/mL, respectively (Viswanathan et al. 2012). In that study, antibody-functionalized nanocrystalline bioconjugates were first introduced to biosensor-bound bacteria, the specifically bound particles were dissolved with acid, and the ions were then stripped using a square-wave voltammetric waveform. Additional studies using stripping voltammetry for electrochemical detection of pathogens can be found in Table 1, Table 2."}
LitCovid-PD-CLO
{"project":"LitCovid-PD-CLO","denotations":[{"id":"T610","span":{"begin":110,"end":111},"obj":"http://purl.obolibrary.org/obo/CLO_0001020"},{"id":"T611","span":{"begin":516,"end":517},"obj":"http://purl.obolibrary.org/obo/CLO_0001020"},{"id":"T612","span":{"begin":732,"end":737},"obj":"http://purl.obolibrary.org/obo/UBERON_0007688"},{"id":"T613","span":{"begin":1000,"end":1005},"obj":"http://purl.obolibrary.org/obo/UBERON_0007688"},{"id":"T614","span":{"begin":1136,"end":1142},"obj":"http://purl.obolibrary.org/obo/OBI_0000968"},{"id":"T615","span":{"begin":1190,"end":1197},"obj":"http://purl.obolibrary.org/obo/OBI_0100026"},{"id":"T616","span":{"begin":1190,"end":1197},"obj":"http://purl.obolibrary.org/obo/UBERON_0000468"},{"id":"T617","span":{"begin":1356,"end":1357},"obj":"http://purl.obolibrary.org/obo/CLO_0001020"},{"id":"T618","span":{"begin":1484,"end":1485},"obj":"http://purl.obolibrary.org/obo/CLO_0001020"},{"id":"T619","span":{"begin":1593,"end":1598},"obj":"http://purl.obolibrary.org/obo/NCBITaxon_9606"},{"id":"T620","span":{"begin":1609,"end":1610},"obj":"http://purl.obolibrary.org/obo/CLO_0001020"},{"id":"T621","span":{"begin":1611,"end":1616},"obj":"http://purl.obolibrary.org/obo/NCBITaxon_10239"},{"id":"T622","span":{"begin":1639,"end":1640},"obj":"http://purl.obolibrary.org/obo/CLO_0001020"},{"id":"T623","span":{"begin":1716,"end":1722},"obj":"http://purl.obolibrary.org/obo/CLO_0001302"},{"id":"T624","span":{"begin":1727,"end":1728},"obj":"http://purl.obolibrary.org/obo/CLO_0001020"},{"id":"T625","span":{"begin":1907,"end":1908},"obj":"http://purl.obolibrary.org/obo/CLO_0001020"},{"id":"T626","span":{"begin":2127,"end":2128},"obj":"http://purl.obolibrary.org/obo/CLO_0001020"},{"id":"T627","span":{"begin":2213,"end":2214},"obj":"http://purl.obolibrary.org/obo/CLO_0001020"},{"id":"T628","span":{"begin":2236,"end":2237},"obj":"http://purl.obolibrary.org/obo/CLO_0001020"},{"id":"T629","span":{"begin":2323,"end":2324},"obj":"http://purl.obolibrary.org/obo/CLO_0001020"},{"id":"T630","span":{"begin":2480,"end":2481},"obj":"http://purl.obolibrary.org/obo/CLO_0001020"},{"id":"T631","span":{"begin":2637,"end":2638},"obj":"http://purl.obolibrary.org/obo/CLO_0001020"},{"id":"T632","span":{"begin":2668,"end":2674},"obj":"http://purl.obolibrary.org/obo/CLO_0001302"},{"id":"T633","span":{"begin":2719,"end":2720},"obj":"http://purl.obolibrary.org/obo/CLO_0001020"},{"id":"T634","span":{"begin":2755,"end":2761},"obj":"http://purl.obolibrary.org/obo/CLO_0001302"},{"id":"T635","span":{"begin":2817,"end":2818},"obj":"http://purl.obolibrary.org/obo/CLO_0001020"},{"id":"T636","span":{"begin":2868,"end":2869},"obj":"http://purl.obolibrary.org/obo/CLO_0001020"},{"id":"T637","span":{"begin":2919,"end":2920},"obj":"http://purl.obolibrary.org/obo/CLO_0001020"},{"id":"T638","span":{"begin":3119,"end":3120},"obj":"http://purl.obolibrary.org/obo/CLO_0001020"},{"id":"T639","span":{"begin":3229,"end":3230},"obj":"http://purl.obolibrary.org/obo/CLO_0001021"},{"id":"T640","span":{"begin":3446,"end":3447},"obj":"http://purl.obolibrary.org/obo/CLO_0001020"},{"id":"T641","span":{"begin":3669,"end":3670},"obj":"http://purl.obolibrary.org/obo/CLO_0001021"},{"id":"T642","span":{"begin":3749,"end":3750},"obj":"http://purl.obolibrary.org/obo/CLO_0001020"},{"id":"T643","span":{"begin":3841,"end":3844},"obj":"http://purl.obolibrary.org/obo/CLO_0051582"},{"id":"T644","span":{"begin":4059,"end":4060},"obj":"http://purl.obolibrary.org/obo/CLO_0001020"},{"id":"T645","span":{"begin":4202,"end":4208},"obj":"http://purl.obolibrary.org/obo/SO_0000418"},{"id":"T646","span":{"begin":4243,"end":4244},"obj":"http://purl.obolibrary.org/obo/CLO_0001020"},{"id":"T647","span":{"begin":4288,"end":4289},"obj":"http://purl.obolibrary.org/obo/CLO_0001020"},{"id":"T648","span":{"begin":4334,"end":4335},"obj":"http://purl.obolibrary.org/obo/CLO_0001020"},{"id":"T649","span":{"begin":4517,"end":4518},"obj":"http://purl.obolibrary.org/obo/CLO_0001020"},{"id":"T650","span":{"begin":4572,"end":4573},"obj":"http://purl.obolibrary.org/obo/CLO_0001020"},{"id":"T651","span":{"begin":4981,"end":4982},"obj":"http://purl.obolibrary.org/obo/CLO_0001020"},{"id":"T652","span":{"begin":5050,"end":5051},"obj":"http://purl.obolibrary.org/obo/CLO_0001020"},{"id":"T653","span":{"begin":5116,"end":5121},"obj":"http://purl.obolibrary.org/obo/NCBITaxon_10239"},{"id":"T654","span":{"begin":5127,"end":5128},"obj":"http://purl.obolibrary.org/obo/CLO_0001020"},{"id":"T655","span":{"begin":5217,"end":5218},"obj":"http://purl.obolibrary.org/obo/CLO_0001020"},{"id":"T656","span":{"begin":5511,"end":5512},"obj":"http://purl.obolibrary.org/obo/CLO_0001020"},{"id":"T657","span":{"begin":5730,"end":5731},"obj":"http://purl.obolibrary.org/obo/CLO_0001020"},{"id":"T658","span":{"begin":5970,"end":5973},"obj":"http://purl.obolibrary.org/obo/CLO_0051582"},{"id":"T659","span":{"begin":6051,"end":6057},"obj":"http://purl.obolibrary.org/obo/CLO_0007225"},{"id":"T660","span":{"begin":6216,"end":6217},"obj":"http://purl.obolibrary.org/obo/CLO_0001020"},{"id":"T661","span":{"begin":6226,"end":6229},"obj":"http://purl.obolibrary.org/obo/CLO_0050251"},{"id":"T662","span":{"begin":6277,"end":6278},"obj":"http://purl.obolibrary.org/obo/CLO_0001020"},{"id":"T663","span":{"begin":6491,"end":6492},"obj":"http://purl.obolibrary.org/obo/CLO_0001020"},{"id":"T664","span":{"begin":6522,"end":6530},"obj":"http://purl.obolibrary.org/obo/NCBITaxon_2"},{"id":"T665","span":{"begin":6852,"end":6853},"obj":"http://purl.obolibrary.org/obo/CLO_0001020"},{"id":"T666","span":{"begin":6865,"end":6870},"obj":"http://purl.obolibrary.org/obo/GO_0005623"},{"id":"T667","span":{"begin":6879,"end":6884},"obj":"http://purl.obolibrary.org/obo/GO_0005623"},{"id":"T668","span":{"begin":6897,"end":6902},"obj":"http://purl.obolibrary.org/obo/GO_0005623"},{"id":"T669","span":{"begin":7057,"end":7065},"obj":"http://purl.obolibrary.org/obo/NCBITaxon_2"},{"id":"T670","span":{"begin":7164,"end":7165},"obj":"http://purl.obolibrary.org/obo/CLO_0001020"}],"text":"3.3.2 Voltammetry\nVoltammetric methods, also referred to as controlled-potential methods, are those in which a current is measured in response to an applied electrical potential that drives redox reactions (Bard and Faulkner, 2000). The measured current is indicative of electron transfer within the sample and at the electrode surface, and thus, the concentration of the analyte. In chronoamperometry, the electrical potential at the working electrode is applied in steps, and the resulting current is measured as a function of time. The applied electrical potential can also be held constant or varied with time as the current is measured.\nAlthough various types of biosensors are compatible with voltammetry-based measurements, field-effect transistor (FET)-based biosensors often utilize amperometric-based methods for pathogen detection (Huang et al. 2011; Liu et al. 2013). FET biosensors detect pathogens via measured changes in source-drain channel conductivity that arise from the electric field of the sample environment. This is achieved by immobilizing biorecognition elements on the metal or polymer gate electrode of the device. He et al. showed that FETs based on PEDOT:PSS organic electrochemical transistor electrodes enabled the detection of E. coli in KCl solutions using Pt and Ag/AgCl gate electrodes (He et al. 2012). Wu et al. used a graphene-based FET to detect E. coli in nutrient broth diluted with phosphate buffered saline solution with amperometry using a Ag/AgCl gate electrode (Wu et al. 2016).\nFurther examples of amperometric sensing include the detection of human influenza A virus by Singh et al. using a reduced graphene oxide-based electrode and chronoamperometry using Fe(CN)6 3 - /4- at a LOD of 0.5 plaque-forming units (PFU)/mL (Singh et al. 2017b). Lee and Jun utilized wire-based electrodes for amperometric detection of E. coli and S. aureus (Lee and Jun 2016). A detailed list of studies that utilize amperometric methods for pathogen detection is provided in Table 1, Table 2\n\n3.3.2.1 Linear sweep and cyclic voltammetry\nLinear sweep voltammetry (LSV) methods are those in which a current is measured in response to an applied electrical potential that is swept at a constant rate across a range of electrical potentials (Bard and Faulkner, 2000). Cyclic voltammetry (CV) is a commonly used linear-sweep method in which the electrical potential is swept in both the forward and reverse directions in partial cycles, full cycles, or a series of cycles. CV is one of the most widely used voltammetric methods for pathogen detection.\nHong et al. used sweep voltammetry to detect norovirus in a sample solution with Fe(CN)6 3 - /4- extracted from lettuce (Hong et al. 2015). A typical CV response using Fe(CN)6 3 - /4- associated with pathogen detection is shown in Fig. 5 a for various concentrations of E. coli binding to a polymer composite electrode (Güner et al. 2017). A detailed overview of pathogen detection studies based on CV is provided in Table 1, Table 2.\nFig. 5 Typical responses associated with the common electrochemical methods used for pathogen detection. a) Cyclic voltammetry (CV) data using Fe(CN)63-/4- for varying concentrations of E. coli (Güner et al. 2017). b) Differential pulse voltammetry (DPV) data using Fe(CN)63-/4- for varying concentrations of S. aureus (Bhardwaj et al. 2017). c) Electrochemical impedance spectroscopy (EIS) in 100 mM LiClO4 solution in the form of a Nyquist plot and corresponding equivalent circuit model associated with biorecognition element immobilization and detection of S. typhimurium (Sheikhzadeh et al. 2016). d) Conductometry data for varying concentrations of B. subtilis (Yoo et al. 2017).\n\n3.3.2.2 Pulse voltammetry\nPulse voltammetry is a type of voltammetry in which the electrical potential is applied in pulses. The technique has the advantage of improved speed and sensitivity relative to traditional voltammetric techniques (Bard and Faulkner, 2000; Molina and González, 2016). In staircase voltammetry, the electrical potential is pulsed in a series of stair steps and the current is measured following each step change, which reduces the effect of capacitive charging on the current signal. Square wave voltammetry (SWV) is a type of staircase voltammetry that applies a symmetric square-wave pulse superimposed on a staircase potential waveform. The forward pulse of the waveform coincides with the staircase step. In differential pulse voltammetry (DPV), the electrical potential is scanned with a series of fixed amplitude pulses and superimposed on a changing base potential. The current is measured before the pulse application and again at the end of the pulse, which allows for the decay of the nonfaradaic current (Scott, 2016).\nFor example, Iqbal et al. used SWV with AuNP-modified carbon electrodes for detection of C. parvum in samples taken from fruit (Iqbal et al. 2015). Kitajima et al. also used SWV with Au microelectrodes to detect norovirus at a LOD of 10 PFU/mL (Kitajima et al. 2016). Cheng et al. used DPV and a nanostructured alumina electrode for detection of dengue type 2 virus with a LOD of 1 PFU/mL (Cheng et al. 2012). As shown in Fig. 5b, Bhardwaj et al. used DPV with a carbon-based electrode to detect S. aureus (Bhardwaj et al. 2017). Additional studies that utilize pulse voltammetry methods forpathogen detection are listed in Table 1, Table 2.\n\n3.3.2.3 Stripping voltammetry\nMany of the previously described voltammetric methods can be modified to include a step that pre-concentrates the target on the electrode surface. Subsequently, the pre-concentrated target is stripped from the surface by application of an electrical potential. In anodic stripping voltammetry (ASV), a negative potential is used to pre-concentrate metal ions onto the electrode surface. These ions are then stripped from the surface by applied positive potentials. Although most commonly used to detect trace amounts of metals, this method has been adapted for pathogen detection by electrocatalytically coating metallic labels on bound targets for oxidative stripping and subsequently measuring the current response (Abbaspour et al. 2015).\nChen et al. used stripping voltammetry with a polymer-CNT composite-based electrode to detect E. coli at a LOD of 13 CFU/mL (Chen et al. 2014). In that study, the biosensor was first incubated with E. coli. Silica-coated Ag nanoparticles conjugated with anti-E.coli were subsequently introduced to the system, inducing a binding reaction between the bacteria and the nanoparticles. After rinsing non-specifically bound particles, acid was introduced to dissolve Ag(s), and the resulting Ag+-rich solution was characterized using DPV. Viswanathan et al. used ASV with screen-printed composite electrodes for multiplexed detection of Campylobacter, S. typhimurium, and E. coli with a LOD of 400 cells/mL, 400 cells/mL, and 800 cells/mL, respectively (Viswanathan et al. 2012). In that study, antibody-functionalized nanocrystalline bioconjugates were first introduced to biosensor-bound bacteria, the specifically bound particles were dissolved with acid, and the ions were then stripped using a square-wave voltammetric waveform. Additional studies using stripping voltammetry for electrochemical detection of pathogens can be found in Table 1, Table 2."}
LitCovid-PD-CHEBI
{"project":"LitCovid-PD-CHEBI","denotations":[{"id":"T164","span":{"begin":272,"end":280},"obj":"Chemical"},{"id":"T52473","span":{"begin":1106,"end":1113},"obj":"Chemical"},{"id":"T11372","span":{"begin":1272,"end":1275},"obj":"Chemical"},{"id":"T72146","span":{"begin":1292,"end":1294},"obj":"Chemical"},{"id":"T59306","span":{"begin":1299,"end":1301},"obj":"Chemical"},{"id":"T87943","span":{"begin":1302,"end":1306},"obj":"Chemical"},{"id":"T52525","span":{"begin":1358,"end":1366},"obj":"Chemical"},{"id":"T86894","span":{"begin":1398,"end":1406},"obj":"Chemical"},{"id":"T175","span":{"begin":1426,"end":1435},"obj":"Chemical"},{"id":"T15115","span":{"begin":1452,"end":1460},"obj":"Chemical"},{"id":"T45998","span":{"begin":1486,"end":1488},"obj":"Chemical"},{"id":"T87052","span":{"begin":1489,"end":1493},"obj":"Chemical"},{"id":"T90850","span":{"begin":1649,"end":1663},"obj":"Chemical"},{"id":"T43328","span":{"begin":1649,"end":1657},"obj":"Chemical"},{"id":"T185","span":{"begin":1658,"end":1663},"obj":"Chemical"},{"id":"T8294","span":{"begin":1708,"end":1710},"obj":"Chemical"},{"id":"T70449","span":{"begin":2646,"end":2654},"obj":"Chemical"},{"id":"T85200","span":{"begin":2660,"end":2662},"obj":"Chemical"},{"id":"T43448","span":{"begin":2747,"end":2749},"obj":"Chemical"},{"id":"T96091","span":{"begin":2870,"end":2877},"obj":"Chemical"},{"id":"T82540","span":{"begin":3157,"end":3159},"obj":"Chemical"},{"id":"T38279","span":{"begin":3280,"end":3282},"obj":"Chemical"},{"id":"T58192","span":{"begin":3400,"end":3403},"obj":"Chemical"},{"id":"T196","span":{"begin":3422,"end":3430},"obj":"Chemical"},{"id":"T197","span":{"begin":4583,"end":4587},"obj":"Chemical"},{"id":"T43582","span":{"begin":4640,"end":4651},"obj":"Chemical"},{"id":"T16502","span":{"begin":4796,"end":4800},"obj":"Chemical"},{"id":"T14779","span":{"begin":4810,"end":4816},"obj":"Chemical"},{"id":"T202","span":{"begin":4939,"end":4941},"obj":"Chemical"},{"id":"T27284","span":{"begin":5067,"end":5074},"obj":"Chemical"},{"id":"T73919","span":{"begin":5219,"end":5225},"obj":"Chemical"},{"id":"T39056","span":{"begin":5651,"end":5662},"obj":"Chemical"},{"id":"T72019","span":{"begin":5784,"end":5788},"obj":"Chemical"},{"id":"T208","span":{"begin":5823,"end":5827},"obj":"Chemical"},{"id":"T98783","span":{"begin":5950,"end":5956},"obj":"Chemical"},{"id":"T24327","span":{"begin":6218,"end":6225},"obj":"Chemical"},{"id":"T59459","span":{"begin":6226,"end":6229},"obj":"Chemical"},{"id":"T48374","span":{"begin":6386,"end":6409},"obj":"Chemical"},{"id":"T64821","span":{"begin":6393,"end":6409},"obj":"Chemical"},{"id":"T71115","span":{"begin":6393,"end":6395},"obj":"Chemical"},{"id":"T33477","span":{"begin":6396,"end":6409},"obj":"Chemical"},{"id":"T62254","span":{"begin":6539,"end":6552},"obj":"Chemical"},{"id":"T83615","span":{"begin":6602,"end":6606},"obj":"Chemical"},{"id":"T49406","span":{"begin":6634,"end":6636},"obj":"Chemical"},{"id":"T6395","span":{"begin":6659,"end":6661},"obj":"Chemical"},{"id":"T99406","span":{"begin":6668,"end":6676},"obj":"Chemical"},{"id":"T15447","span":{"begin":7002,"end":7015},"obj":"Chemical"},{"id":"T79726","span":{"begin":7120,"end":7124},"obj":"Chemical"},{"id":"T41307","span":{"begin":7134,"end":7138},"obj":"Chemical"}],"attributes":[{"id":"A94299","pred":"chebi_id","subj":"T164","obj":"http://purl.obolibrary.org/obo/CHEBI_10545"},{"id":"A961","pred":"chebi_id","subj":"T52473","obj":"http://purl.obolibrary.org/obo/CHEBI_33839"},{"id":"A93547","pred":"chebi_id","subj":"T52473","obj":"http://purl.obolibrary.org/obo/CHEBI_60027"},{"id":"A90573","pred":"chebi_id","subj":"T11372","obj":"http://purl.obolibrary.org/obo/CHEBI_32588"},{"id":"A52856","pred":"chebi_id","subj":"T72146","obj":"http://purl.obolibrary.org/obo/CHEBI_33364"},{"id":"A65811","pred":"chebi_id","subj":"T72146","obj":"http://purl.obolibrary.org/obo/CHEBI_75318"},{"id":"A43804","pred":"chebi_id","subj":"T59306","obj":"http://purl.obolibrary.org/obo/CHEBI_30512"},{"id":"A18589","pred":"chebi_id","subj":"T59306","obj":"http://purl.obolibrary.org/obo/CHEBI_9141"},{"id":"A4955","pred":"chebi_id","subj":"T87943","obj":"http://purl.obolibrary.org/obo/CHEBI_30341"},{"id":"A56444","pred":"chebi_id","subj":"T52525","obj":"http://purl.obolibrary.org/obo/CHEBI_36973"},{"id":"A6112","pred":"chebi_id","subj":"T86894","obj":"http://purl.obolibrary.org/obo/CHEBI_33284"},{"id":"A20775","pred":"chebi_id","subj":"T175","obj":"http://purl.obolibrary.org/obo/CHEBI_18367"},{"id":"A59351","pred":"chebi_id","subj":"T175","obj":"http://purl.obolibrary.org/obo/CHEBI_26020"},{"id":"A61248","pred":"chebi_id","subj":"T175","obj":"http://purl.obolibrary.org/obo/CHEBI_35780"},{"id":"A82627","pred":"chebi_id","subj":"T175","obj":"http://purl.obolibrary.org/obo/CHEBI_43474"},{"id":"A37968","pred":"chebi_id","subj":"T15115","obj":"http://purl.obolibrary.org/obo/CHEBI_75958"},{"id":"A76979","pred":"chebi_id","subj":"T45998","obj":"http://purl.obolibrary.org/obo/CHEBI_30512"},{"id":"A82968","pred":"chebi_id","subj":"T45998","obj":"http://purl.obolibrary.org/obo/CHEBI_9141"},{"id":"A23691","pred":"chebi_id","subj":"T87052","obj":"http://purl.obolibrary.org/obo/CHEBI_30341"},{"id":"A27222","pred":"chebi_id","subj":"T90850","obj":"http://purl.obolibrary.org/obo/CHEBI_132889"},{"id":"A34268","pred":"chebi_id","subj":"T43328","obj":"http://purl.obolibrary.org/obo/CHEBI_36973"},{"id":"A43866","pred":"chebi_id","subj":"T185","obj":"http://purl.obolibrary.org/obo/CHEBI_25741"},{"id":"A8417","pred":"chebi_id","subj":"T185","obj":"http://purl.obolibrary.org/obo/CHEBI_29356"},{"id":"A41116","pred":"chebi_id","subj":"T8294","obj":"http://purl.obolibrary.org/obo/CHEBI_18248"},{"id":"A91245","pred":"chebi_id","subj":"T70449","obj":"http://purl.obolibrary.org/obo/CHEBI_75958"},{"id":"A20579","pred":"chebi_id","subj":"T85200","obj":"http://purl.obolibrary.org/obo/CHEBI_18248"},{"id":"A42954","pred":"chebi_id","subj":"T43448","obj":"http://purl.obolibrary.org/obo/CHEBI_18248"},{"id":"A15594","pred":"chebi_id","subj":"T96091","obj":"http://purl.obolibrary.org/obo/CHEBI_33839"},{"id":"A53871","pred":"chebi_id","subj":"T96091","obj":"http://purl.obolibrary.org/obo/CHEBI_60027"},{"id":"A96162","pred":"chebi_id","subj":"T82540","obj":"http://purl.obolibrary.org/obo/CHEBI_18248"},{"id":"A80388","pred":"chebi_id","subj":"T38279","obj":"http://purl.obolibrary.org/obo/CHEBI_18248"},{"id":"A75311","pred":"chebi_id","subj":"T58192","obj":"http://purl.obolibrary.org/obo/CHEBI_73498"},{"id":"A74162","pred":"chebi_id","subj":"T196","obj":"http://purl.obolibrary.org/obo/CHEBI_75958"},{"id":"A23524","pred":"chebi_id","subj":"T197","obj":"http://purl.obolibrary.org/obo/CHEBI_22695"},{"id":"A57845","pred":"chebi_id","subj":"T43582","obj":"http://purl.obolibrary.org/obo/CHEBI_33232"},{"id":"A66247","pred":"chebi_id","subj":"T16502","obj":"http://purl.obolibrary.org/obo/CHEBI_50825"},{"id":"A71274","pred":"chebi_id","subj":"T14779","obj":"http://purl.obolibrary.org/obo/CHEBI_27594"},{"id":"A23660","pred":"chebi_id","subj":"T14779","obj":"http://purl.obolibrary.org/obo/CHEBI_33415"},{"id":"A10903","pred":"chebi_id","subj":"T202","obj":"http://purl.obolibrary.org/obo/CHEBI_29287"},{"id":"A29452","pred":"chebi_id","subj":"T27284","obj":"http://purl.obolibrary.org/obo/CHEBI_30187"},{"id":"A66597","pred":"chebi_id","subj":"T73919","obj":"http://purl.obolibrary.org/obo/CHEBI_27594"},{"id":"A16266","pred":"chebi_id","subj":"T73919","obj":"http://purl.obolibrary.org/obo/CHEBI_33415"},{"id":"A64348","pred":"chebi_id","subj":"T39056","obj":"http://purl.obolibrary.org/obo/CHEBI_33232"},{"id":"A18409","pred":"chebi_id","subj":"T72019","obj":"http://purl.obolibrary.org/obo/CHEBI_24870"},{"id":"A7284","pred":"chebi_id","subj":"T208","obj":"http://purl.obolibrary.org/obo/CHEBI_24870"},{"id":"A98618","pred":"chebi_id","subj":"T98783","obj":"http://purl.obolibrary.org/obo/CHEBI_33521"},{"id":"A4401","pred":"chebi_id","subj":"T24327","obj":"http://purl.obolibrary.org/obo/CHEBI_33839"},{"id":"A99460","pred":"chebi_id","subj":"T24327","obj":"http://purl.obolibrary.org/obo/CHEBI_60027"},{"id":"A97160","pred":"chebi_id","subj":"T59459","obj":"http://purl.obolibrary.org/obo/CHEBI_50594"},{"id":"A66562","pred":"chebi_id","subj":"T48374","obj":"http://purl.obolibrary.org/obo/CHEBI_82776"},{"id":"A86671","pred":"chebi_id","subj":"T64821","obj":"http://purl.obolibrary.org/obo/CHEBI_50826"},{"id":"A41281","pred":"chebi_id","subj":"T71115","obj":"http://purl.obolibrary.org/obo/CHEBI_30512"},{"id":"A24430","pred":"chebi_id","subj":"T71115","obj":"http://purl.obolibrary.org/obo/CHEBI_9141"},{"id":"A31301","pred":"chebi_id","subj":"T33477","obj":"http://purl.obolibrary.org/obo/CHEBI_50803"},{"id":"A84379","pred":"chebi_id","subj":"T62254","obj":"http://purl.obolibrary.org/obo/CHEBI_50803"},{"id":"A81725","pred":"chebi_id","subj":"T83615","obj":"http://purl.obolibrary.org/obo/CHEBI_37527"},{"id":"A89820","pred":"chebi_id","subj":"T49406","obj":"http://purl.obolibrary.org/obo/CHEBI_30512"},{"id":"A5244","pred":"chebi_id","subj":"T49406","obj":"http://purl.obolibrary.org/obo/CHEBI_9141"},{"id":"A78289","pred":"chebi_id","subj":"T6395","obj":"http://purl.obolibrary.org/obo/CHEBI_30512"},{"id":"A72683","pred":"chebi_id","subj":"T6395","obj":"http://purl.obolibrary.org/obo/CHEBI_9141"},{"id":"A46231","pred":"chebi_id","subj":"T99406","obj":"http://purl.obolibrary.org/obo/CHEBI_75958"},{"id":"A97166","pred":"chebi_id","subj":"T15447","obj":"http://purl.obolibrary.org/obo/CHEBI_64985"},{"id":"A15025","pred":"chebi_id","subj":"T79726","obj":"http://purl.obolibrary.org/obo/CHEBI_37527"},{"id":"A18201","pred":"chebi_id","subj":"T41307","obj":"http://purl.obolibrary.org/obo/CHEBI_24870"}],"text":"3.3.2 Voltammetry\nVoltammetric methods, also referred to as controlled-potential methods, are those in which a current is measured in response to an applied electrical potential that drives redox reactions (Bard and Faulkner, 2000). The measured current is indicative of electron transfer within the sample and at the electrode surface, and thus, the concentration of the analyte. In chronoamperometry, the electrical potential at the working electrode is applied in steps, and the resulting current is measured as a function of time. The applied electrical potential can also be held constant or varied with time as the current is measured.\nAlthough various types of biosensors are compatible with voltammetry-based measurements, field-effect transistor (FET)-based biosensors often utilize amperometric-based methods for pathogen detection (Huang et al. 2011; Liu et al. 2013). FET biosensors detect pathogens via measured changes in source-drain channel conductivity that arise from the electric field of the sample environment. This is achieved by immobilizing biorecognition elements on the metal or polymer gate electrode of the device. He et al. showed that FETs based on PEDOT:PSS organic electrochemical transistor electrodes enabled the detection of E. coli in KCl solutions using Pt and Ag/AgCl gate electrodes (He et al. 2012). Wu et al. used a graphene-based FET to detect E. coli in nutrient broth diluted with phosphate buffered saline solution with amperometry using a Ag/AgCl gate electrode (Wu et al. 2016).\nFurther examples of amperometric sensing include the detection of human influenza A virus by Singh et al. using a reduced graphene oxide-based electrode and chronoamperometry using Fe(CN)6 3 - /4- at a LOD of 0.5 plaque-forming units (PFU)/mL (Singh et al. 2017b). Lee and Jun utilized wire-based electrodes for amperometric detection of E. coli and S. aureus (Lee and Jun 2016). A detailed list of studies that utilize amperometric methods for pathogen detection is provided in Table 1, Table 2\n\n3.3.2.1 Linear sweep and cyclic voltammetry\nLinear sweep voltammetry (LSV) methods are those in which a current is measured in response to an applied electrical potential that is swept at a constant rate across a range of electrical potentials (Bard and Faulkner, 2000). Cyclic voltammetry (CV) is a commonly used linear-sweep method in which the electrical potential is swept in both the forward and reverse directions in partial cycles, full cycles, or a series of cycles. CV is one of the most widely used voltammetric methods for pathogen detection.\nHong et al. used sweep voltammetry to detect norovirus in a sample solution with Fe(CN)6 3 - /4- extracted from lettuce (Hong et al. 2015). A typical CV response using Fe(CN)6 3 - /4- associated with pathogen detection is shown in Fig. 5 a for various concentrations of E. coli binding to a polymer composite electrode (Güner et al. 2017). A detailed overview of pathogen detection studies based on CV is provided in Table 1, Table 2.\nFig. 5 Typical responses associated with the common electrochemical methods used for pathogen detection. a) Cyclic voltammetry (CV) data using Fe(CN)63-/4- for varying concentrations of E. coli (Güner et al. 2017). b) Differential pulse voltammetry (DPV) data using Fe(CN)63-/4- for varying concentrations of S. aureus (Bhardwaj et al. 2017). c) Electrochemical impedance spectroscopy (EIS) in 100 mM LiClO4 solution in the form of a Nyquist plot and corresponding equivalent circuit model associated with biorecognition element immobilization and detection of S. typhimurium (Sheikhzadeh et al. 2016). d) Conductometry data for varying concentrations of B. subtilis (Yoo et al. 2017).\n\n3.3.2.2 Pulse voltammetry\nPulse voltammetry is a type of voltammetry in which the electrical potential is applied in pulses. The technique has the advantage of improved speed and sensitivity relative to traditional voltammetric techniques (Bard and Faulkner, 2000; Molina and González, 2016). In staircase voltammetry, the electrical potential is pulsed in a series of stair steps and the current is measured following each step change, which reduces the effect of capacitive charging on the current signal. Square wave voltammetry (SWV) is a type of staircase voltammetry that applies a symmetric square-wave pulse superimposed on a staircase potential waveform. The forward pulse of the waveform coincides with the staircase step. In differential pulse voltammetry (DPV), the electrical potential is scanned with a series of fixed amplitude pulses and superimposed on a changing base potential. The current is measured before the pulse application and again at the end of the pulse, which allows for the decay of the nonfaradaic current (Scott, 2016).\nFor example, Iqbal et al. used SWV with AuNP-modified carbon electrodes for detection of C. parvum in samples taken from fruit (Iqbal et al. 2015). Kitajima et al. also used SWV with Au microelectrodes to detect norovirus at a LOD of 10 PFU/mL (Kitajima et al. 2016). Cheng et al. used DPV and a nanostructured alumina electrode for detection of dengue type 2 virus with a LOD of 1 PFU/mL (Cheng et al. 2012). As shown in Fig. 5b, Bhardwaj et al. used DPV with a carbon-based electrode to detect S. aureus (Bhardwaj et al. 2017). Additional studies that utilize pulse voltammetry methods forpathogen detection are listed in Table 1, Table 2.\n\n3.3.2.3 Stripping voltammetry\nMany of the previously described voltammetric methods can be modified to include a step that pre-concentrates the target on the electrode surface. Subsequently, the pre-concentrated target is stripped from the surface by application of an electrical potential. In anodic stripping voltammetry (ASV), a negative potential is used to pre-concentrate metal ions onto the electrode surface. These ions are then stripped from the surface by applied positive potentials. Although most commonly used to detect trace amounts of metals, this method has been adapted for pathogen detection by electrocatalytically coating metallic labels on bound targets for oxidative stripping and subsequently measuring the current response (Abbaspour et al. 2015).\nChen et al. used stripping voltammetry with a polymer-CNT composite-based electrode to detect E. coli at a LOD of 13 CFU/mL (Chen et al. 2014). In that study, the biosensor was first incubated with E. coli. Silica-coated Ag nanoparticles conjugated with anti-E.coli were subsequently introduced to the system, inducing a binding reaction between the bacteria and the nanoparticles. After rinsing non-specifically bound particles, acid was introduced to dissolve Ag(s), and the resulting Ag+-rich solution was characterized using DPV. Viswanathan et al. used ASV with screen-printed composite electrodes for multiplexed detection of Campylobacter, S. typhimurium, and E. coli with a LOD of 400 cells/mL, 400 cells/mL, and 800 cells/mL, respectively (Viswanathan et al. 2012). In that study, antibody-functionalized nanocrystalline bioconjugates were first introduced to biosensor-bound bacteria, the specifically bound particles were dissolved with acid, and the ions were then stripped using a square-wave voltammetric waveform. Additional studies using stripping voltammetry for electrochemical detection of pathogens can be found in Table 1, Table 2."}
LitCovid-PD-GO-BP
{"project":"LitCovid-PD-GO-BP","denotations":[{"id":"T39","span":{"begin":272,"end":289},"obj":"http://purl.obolibrary.org/obo/GO_0022904"}],"text":"3.3.2 Voltammetry\nVoltammetric methods, also referred to as controlled-potential methods, are those in which a current is measured in response to an applied electrical potential that drives redox reactions (Bard and Faulkner, 2000). The measured current is indicative of electron transfer within the sample and at the electrode surface, and thus, the concentration of the analyte. In chronoamperometry, the electrical potential at the working electrode is applied in steps, and the resulting current is measured as a function of time. The applied electrical potential can also be held constant or varied with time as the current is measured.\nAlthough various types of biosensors are compatible with voltammetry-based measurements, field-effect transistor (FET)-based biosensors often utilize amperometric-based methods for pathogen detection (Huang et al. 2011; Liu et al. 2013). FET biosensors detect pathogens via measured changes in source-drain channel conductivity that arise from the electric field of the sample environment. This is achieved by immobilizing biorecognition elements on the metal or polymer gate electrode of the device. He et al. showed that FETs based on PEDOT:PSS organic electrochemical transistor electrodes enabled the detection of E. coli in KCl solutions using Pt and Ag/AgCl gate electrodes (He et al. 2012). Wu et al. used a graphene-based FET to detect E. coli in nutrient broth diluted with phosphate buffered saline solution with amperometry using a Ag/AgCl gate electrode (Wu et al. 2016).\nFurther examples of amperometric sensing include the detection of human influenza A virus by Singh et al. using a reduced graphene oxide-based electrode and chronoamperometry using Fe(CN)6 3 - /4- at a LOD of 0.5 plaque-forming units (PFU)/mL (Singh et al. 2017b). Lee and Jun utilized wire-based electrodes for amperometric detection of E. coli and S. aureus (Lee and Jun 2016). A detailed list of studies that utilize amperometric methods for pathogen detection is provided in Table 1, Table 2\n\n3.3.2.1 Linear sweep and cyclic voltammetry\nLinear sweep voltammetry (LSV) methods are those in which a current is measured in response to an applied electrical potential that is swept at a constant rate across a range of electrical potentials (Bard and Faulkner, 2000). Cyclic voltammetry (CV) is a commonly used linear-sweep method in which the electrical potential is swept in both the forward and reverse directions in partial cycles, full cycles, or a series of cycles. CV is one of the most widely used voltammetric methods for pathogen detection.\nHong et al. used sweep voltammetry to detect norovirus in a sample solution with Fe(CN)6 3 - /4- extracted from lettuce (Hong et al. 2015). A typical CV response using Fe(CN)6 3 - /4- associated with pathogen detection is shown in Fig. 5 a for various concentrations of E. coli binding to a polymer composite electrode (Güner et al. 2017). A detailed overview of pathogen detection studies based on CV is provided in Table 1, Table 2.\nFig. 5 Typical responses associated with the common electrochemical methods used for pathogen detection. a) Cyclic voltammetry (CV) data using Fe(CN)63-/4- for varying concentrations of E. coli (Güner et al. 2017). b) Differential pulse voltammetry (DPV) data using Fe(CN)63-/4- for varying concentrations of S. aureus (Bhardwaj et al. 2017). c) Electrochemical impedance spectroscopy (EIS) in 100 mM LiClO4 solution in the form of a Nyquist plot and corresponding equivalent circuit model associated with biorecognition element immobilization and detection of S. typhimurium (Sheikhzadeh et al. 2016). d) Conductometry data for varying concentrations of B. subtilis (Yoo et al. 2017).\n\n3.3.2.2 Pulse voltammetry\nPulse voltammetry is a type of voltammetry in which the electrical potential is applied in pulses. The technique has the advantage of improved speed and sensitivity relative to traditional voltammetric techniques (Bard and Faulkner, 2000; Molina and González, 2016). In staircase voltammetry, the electrical potential is pulsed in a series of stair steps and the current is measured following each step change, which reduces the effect of capacitive charging on the current signal. Square wave voltammetry (SWV) is a type of staircase voltammetry that applies a symmetric square-wave pulse superimposed on a staircase potential waveform. The forward pulse of the waveform coincides with the staircase step. In differential pulse voltammetry (DPV), the electrical potential is scanned with a series of fixed amplitude pulses and superimposed on a changing base potential. The current is measured before the pulse application and again at the end of the pulse, which allows for the decay of the nonfaradaic current (Scott, 2016).\nFor example, Iqbal et al. used SWV with AuNP-modified carbon electrodes for detection of C. parvum in samples taken from fruit (Iqbal et al. 2015). Kitajima et al. also used SWV with Au microelectrodes to detect norovirus at a LOD of 10 PFU/mL (Kitajima et al. 2016). Cheng et al. used DPV and a nanostructured alumina electrode for detection of dengue type 2 virus with a LOD of 1 PFU/mL (Cheng et al. 2012). As shown in Fig. 5b, Bhardwaj et al. used DPV with a carbon-based electrode to detect S. aureus (Bhardwaj et al. 2017). Additional studies that utilize pulse voltammetry methods forpathogen detection are listed in Table 1, Table 2.\n\n3.3.2.3 Stripping voltammetry\nMany of the previously described voltammetric methods can be modified to include a step that pre-concentrates the target on the electrode surface. Subsequently, the pre-concentrated target is stripped from the surface by application of an electrical potential. In anodic stripping voltammetry (ASV), a negative potential is used to pre-concentrate metal ions onto the electrode surface. These ions are then stripped from the surface by applied positive potentials. Although most commonly used to detect trace amounts of metals, this method has been adapted for pathogen detection by electrocatalytically coating metallic labels on bound targets for oxidative stripping and subsequently measuring the current response (Abbaspour et al. 2015).\nChen et al. used stripping voltammetry with a polymer-CNT composite-based electrode to detect E. coli at a LOD of 13 CFU/mL (Chen et al. 2014). In that study, the biosensor was first incubated with E. coli. Silica-coated Ag nanoparticles conjugated with anti-E.coli were subsequently introduced to the system, inducing a binding reaction between the bacteria and the nanoparticles. After rinsing non-specifically bound particles, acid was introduced to dissolve Ag(s), and the resulting Ag+-rich solution was characterized using DPV. Viswanathan et al. used ASV with screen-printed composite electrodes for multiplexed detection of Campylobacter, S. typhimurium, and E. coli with a LOD of 400 cells/mL, 400 cells/mL, and 800 cells/mL, respectively (Viswanathan et al. 2012). In that study, antibody-functionalized nanocrystalline bioconjugates were first introduced to biosensor-bound bacteria, the specifically bound particles were dissolved with acid, and the ions were then stripped using a square-wave voltammetric waveform. Additional studies using stripping voltammetry for electrochemical detection of pathogens can be found in Table 1, Table 2."}
LitCovid-sentences
{"project":"LitCovid-sentences","denotations":[{"id":"T753","span":{"begin":0,"end":18},"obj":"Sentence"},{"id":"T754","span":{"begin":19,"end":233},"obj":"Sentence"},{"id":"T755","span":{"begin":234,"end":381},"obj":"Sentence"},{"id":"T756","span":{"begin":382,"end":535},"obj":"Sentence"},{"id":"T757","span":{"begin":536,"end":642},"obj":"Sentence"},{"id":"T758","span":{"begin":643,"end":856},"obj":"Sentence"},{"id":"T759","span":{"begin":857,"end":873},"obj":"Sentence"},{"id":"T760","span":{"begin":874,"end":880},"obj":"Sentence"},{"id":"T761","span":{"begin":881,"end":1032},"obj":"Sentence"},{"id":"T762","span":{"begin":1033,"end":1143},"obj":"Sentence"},{"id":"T763","span":{"begin":1144,"end":1333},"obj":"Sentence"},{"id":"T764","span":{"begin":1334,"end":1340},"obj":"Sentence"},{"id":"T765","span":{"begin":1341,"end":1519},"obj":"Sentence"},{"id":"T766","span":{"begin":1520,"end":1526},"obj":"Sentence"},{"id":"T767","span":{"begin":1527,"end":1783},"obj":"Sentence"},{"id":"T768","span":{"begin":1784,"end":1791},"obj":"Sentence"},{"id":"T769","span":{"begin":1792,"end":1906},"obj":"Sentence"},{"id":"T770","span":{"begin":1907,"end":2022},"obj":"Sentence"},{"id":"T771","span":{"begin":2024,"end":2068},"obj":"Sentence"},{"id":"T772","span":{"begin":2069,"end":2295},"obj":"Sentence"},{"id":"T773","span":{"begin":2296,"end":2499},"obj":"Sentence"},{"id":"T774","span":{"begin":2500,"end":2578},"obj":"Sentence"},{"id":"T775","span":{"begin":2579,"end":2711},"obj":"Sentence"},{"id":"T776","span":{"begin":2712,"end":2718},"obj":"Sentence"},{"id":"T777","span":{"begin":2719,"end":2911},"obj":"Sentence"},{"id":"T778","span":{"begin":2912,"end":2918},"obj":"Sentence"},{"id":"T779","span":{"begin":2919,"end":3013},"obj":"Sentence"},{"id":"T780","span":{"begin":3014,"end":3221},"obj":"Sentence"},{"id":"T781","span":{"begin":3222,"end":3349},"obj":"Sentence"},{"id":"T782","span":{"begin":3350,"end":3609},"obj":"Sentence"},{"id":"T783","span":{"begin":3610,"end":3692},"obj":"Sentence"},{"id":"T784","span":{"begin":3693,"end":3699},"obj":"Sentence"},{"id":"T785","span":{"begin":3701,"end":3727},"obj":"Sentence"},{"id":"T786","span":{"begin":3728,"end":3826},"obj":"Sentence"},{"id":"T787","span":{"begin":3827,"end":3994},"obj":"Sentence"},{"id":"T788","span":{"begin":3995,"end":4209},"obj":"Sentence"},{"id":"T789","span":{"begin":4210,"end":4365},"obj":"Sentence"},{"id":"T790","span":{"begin":4366,"end":4434},"obj":"Sentence"},{"id":"T791","span":{"begin":4435,"end":4598},"obj":"Sentence"},{"id":"T792","span":{"begin":4599,"end":4755},"obj":"Sentence"},{"id":"T793","span":{"begin":4756,"end":4896},"obj":"Sentence"},{"id":"T794","span":{"begin":4897,"end":4903},"obj":"Sentence"},{"id":"T795","span":{"begin":4904,"end":5016},"obj":"Sentence"},{"id":"T796","span":{"begin":5017,"end":5023},"obj":"Sentence"},{"id":"T797","span":{"begin":5024,"end":5158},"obj":"Sentence"},{"id":"T798","span":{"begin":5159,"end":5165},"obj":"Sentence"},{"id":"T799","span":{"begin":5166,"end":5278},"obj":"Sentence"},{"id":"T800","span":{"begin":5279,"end":5285},"obj":"Sentence"},{"id":"T801","span":{"begin":5286,"end":5397},"obj":"Sentence"},{"id":"T802","span":{"begin":5399,"end":5429},"obj":"Sentence"},{"id":"T803","span":{"begin":5430,"end":5576},"obj":"Sentence"},{"id":"T804","span":{"begin":5577,"end":5690},"obj":"Sentence"},{"id":"T805","span":{"begin":5691,"end":5816},"obj":"Sentence"},{"id":"T806","span":{"begin":5817,"end":5894},"obj":"Sentence"},{"id":"T807","span":{"begin":5895,"end":6164},"obj":"Sentence"},{"id":"T808","span":{"begin":6165,"end":6171},"obj":"Sentence"},{"id":"T809","span":{"begin":6172,"end":6308},"obj":"Sentence"},{"id":"T810","span":{"begin":6309,"end":6315},"obj":"Sentence"},{"id":"T811","span":{"begin":6316,"end":6378},"obj":"Sentence"},{"id":"T812","span":{"begin":6379,"end":6553},"obj":"Sentence"},{"id":"T813","span":{"begin":6554,"end":6705},"obj":"Sentence"},{"id":"T814","span":{"begin":6706,"end":6939},"obj":"Sentence"},{"id":"T815","span":{"begin":6940,"end":6946},"obj":"Sentence"},{"id":"T816","span":{"begin":6947,"end":7200},"obj":"Sentence"},{"id":"T817","span":{"begin":7201,"end":7324},"obj":"Sentence"}],"namespaces":[{"prefix":"_base","uri":"http://pubannotation.org/ontology/tao.owl#"}],"text":"3.3.2 Voltammetry\nVoltammetric methods, also referred to as controlled-potential methods, are those in which a current is measured in response to an applied electrical potential that drives redox reactions (Bard and Faulkner, 2000). The measured current is indicative of electron transfer within the sample and at the electrode surface, and thus, the concentration of the analyte. In chronoamperometry, the electrical potential at the working electrode is applied in steps, and the resulting current is measured as a function of time. The applied electrical potential can also be held constant or varied with time as the current is measured.\nAlthough various types of biosensors are compatible with voltammetry-based measurements, field-effect transistor (FET)-based biosensors often utilize amperometric-based methods for pathogen detection (Huang et al. 2011; Liu et al. 2013). FET biosensors detect pathogens via measured changes in source-drain channel conductivity that arise from the electric field of the sample environment. This is achieved by immobilizing biorecognition elements on the metal or polymer gate electrode of the device. He et al. showed that FETs based on PEDOT:PSS organic electrochemical transistor electrodes enabled the detection of E. coli in KCl solutions using Pt and Ag/AgCl gate electrodes (He et al. 2012). Wu et al. used a graphene-based FET to detect E. coli in nutrient broth diluted with phosphate buffered saline solution with amperometry using a Ag/AgCl gate electrode (Wu et al. 2016).\nFurther examples of amperometric sensing include the detection of human influenza A virus by Singh et al. using a reduced graphene oxide-based electrode and chronoamperometry using Fe(CN)6 3 - /4- at a LOD of 0.5 plaque-forming units (PFU)/mL (Singh et al. 2017b). Lee and Jun utilized wire-based electrodes for amperometric detection of E. coli and S. aureus (Lee and Jun 2016). A detailed list of studies that utilize amperometric methods for pathogen detection is provided in Table 1, Table 2\n\n3.3.2.1 Linear sweep and cyclic voltammetry\nLinear sweep voltammetry (LSV) methods are those in which a current is measured in response to an applied electrical potential that is swept at a constant rate across a range of electrical potentials (Bard and Faulkner, 2000). Cyclic voltammetry (CV) is a commonly used linear-sweep method in which the electrical potential is swept in both the forward and reverse directions in partial cycles, full cycles, or a series of cycles. CV is one of the most widely used voltammetric methods for pathogen detection.\nHong et al. used sweep voltammetry to detect norovirus in a sample solution with Fe(CN)6 3 - /4- extracted from lettuce (Hong et al. 2015). A typical CV response using Fe(CN)6 3 - /4- associated with pathogen detection is shown in Fig. 5 a for various concentrations of E. coli binding to a polymer composite electrode (Güner et al. 2017). A detailed overview of pathogen detection studies based on CV is provided in Table 1, Table 2.\nFig. 5 Typical responses associated with the common electrochemical methods used for pathogen detection. a) Cyclic voltammetry (CV) data using Fe(CN)63-/4- for varying concentrations of E. coli (Güner et al. 2017). b) Differential pulse voltammetry (DPV) data using Fe(CN)63-/4- for varying concentrations of S. aureus (Bhardwaj et al. 2017). c) Electrochemical impedance spectroscopy (EIS) in 100 mM LiClO4 solution in the form of a Nyquist plot and corresponding equivalent circuit model associated with biorecognition element immobilization and detection of S. typhimurium (Sheikhzadeh et al. 2016). d) Conductometry data for varying concentrations of B. subtilis (Yoo et al. 2017).\n\n3.3.2.2 Pulse voltammetry\nPulse voltammetry is a type of voltammetry in which the electrical potential is applied in pulses. The technique has the advantage of improved speed and sensitivity relative to traditional voltammetric techniques (Bard and Faulkner, 2000; Molina and González, 2016). In staircase voltammetry, the electrical potential is pulsed in a series of stair steps and the current is measured following each step change, which reduces the effect of capacitive charging on the current signal. Square wave voltammetry (SWV) is a type of staircase voltammetry that applies a symmetric square-wave pulse superimposed on a staircase potential waveform. The forward pulse of the waveform coincides with the staircase step. In differential pulse voltammetry (DPV), the electrical potential is scanned with a series of fixed amplitude pulses and superimposed on a changing base potential. The current is measured before the pulse application and again at the end of the pulse, which allows for the decay of the nonfaradaic current (Scott, 2016).\nFor example, Iqbal et al. used SWV with AuNP-modified carbon electrodes for detection of C. parvum in samples taken from fruit (Iqbal et al. 2015). Kitajima et al. also used SWV with Au microelectrodes to detect norovirus at a LOD of 10 PFU/mL (Kitajima et al. 2016). Cheng et al. used DPV and a nanostructured alumina electrode for detection of dengue type 2 virus with a LOD of 1 PFU/mL (Cheng et al. 2012). As shown in Fig. 5b, Bhardwaj et al. used DPV with a carbon-based electrode to detect S. aureus (Bhardwaj et al. 2017). Additional studies that utilize pulse voltammetry methods forpathogen detection are listed in Table 1, Table 2.\n\n3.3.2.3 Stripping voltammetry\nMany of the previously described voltammetric methods can be modified to include a step that pre-concentrates the target on the electrode surface. Subsequently, the pre-concentrated target is stripped from the surface by application of an electrical potential. In anodic stripping voltammetry (ASV), a negative potential is used to pre-concentrate metal ions onto the electrode surface. These ions are then stripped from the surface by applied positive potentials. Although most commonly used to detect trace amounts of metals, this method has been adapted for pathogen detection by electrocatalytically coating metallic labels on bound targets for oxidative stripping and subsequently measuring the current response (Abbaspour et al. 2015).\nChen et al. used stripping voltammetry with a polymer-CNT composite-based electrode to detect E. coli at a LOD of 13 CFU/mL (Chen et al. 2014). In that study, the biosensor was first incubated with E. coli. Silica-coated Ag nanoparticles conjugated with anti-E.coli were subsequently introduced to the system, inducing a binding reaction between the bacteria and the nanoparticles. After rinsing non-specifically bound particles, acid was introduced to dissolve Ag(s), and the resulting Ag+-rich solution was characterized using DPV. Viswanathan et al. used ASV with screen-printed composite electrodes for multiplexed detection of Campylobacter, S. typhimurium, and E. coli with a LOD of 400 cells/mL, 400 cells/mL, and 800 cells/mL, respectively (Viswanathan et al. 2012). In that study, antibody-functionalized nanocrystalline bioconjugates were first introduced to biosensor-bound bacteria, the specifically bound particles were dissolved with acid, and the ions were then stripped using a square-wave voltammetric waveform. Additional studies using stripping voltammetry for electrochemical detection of pathogens can be found in Table 1, Table 2."}
2_test
{"project":"2_test","denotations":[{"id":"32364936-27096467-7713124","span":{"begin":1900,"end":1904},"obj":"27096467"},{"id":"32364936-25254625-7713125","span":{"begin":2712,"end":2716},"obj":"25254625"},{"id":"32364936-28372186-7713126","span":{"begin":2912,"end":2916},"obj":"28372186"},{"id":"32364936-28372186-7713127","span":{"begin":3222,"end":3226},"obj":"28372186"},{"id":"32364936-26836649-7713128","span":{"begin":3610,"end":3614},"obj":"26836649"},{"id":"32364936-28226280-7713129","span":{"begin":3693,"end":3697},"obj":"28226280"},{"id":"32364936-22502614-7713130","span":{"begin":5159,"end":5163},"obj":"22502614"},{"id":"32364936-25562742-7713131","span":{"begin":6165,"end":6169},"obj":"25562742"},{"id":"32364936-24872904-7713132","span":{"begin":6309,"end":6313},"obj":"24872904"},{"id":"32364936-22608454-7713133","span":{"begin":6940,"end":6944},"obj":"22608454"}],"text":"3.3.2 Voltammetry\nVoltammetric methods, also referred to as controlled-potential methods, are those in which a current is measured in response to an applied electrical potential that drives redox reactions (Bard and Faulkner, 2000). The measured current is indicative of electron transfer within the sample and at the electrode surface, and thus, the concentration of the analyte. In chronoamperometry, the electrical potential at the working electrode is applied in steps, and the resulting current is measured as a function of time. The applied electrical potential can also be held constant or varied with time as the current is measured.\nAlthough various types of biosensors are compatible with voltammetry-based measurements, field-effect transistor (FET)-based biosensors often utilize amperometric-based methods for pathogen detection (Huang et al. 2011; Liu et al. 2013). FET biosensors detect pathogens via measured changes in source-drain channel conductivity that arise from the electric field of the sample environment. This is achieved by immobilizing biorecognition elements on the metal or polymer gate electrode of the device. He et al. showed that FETs based on PEDOT:PSS organic electrochemical transistor electrodes enabled the detection of E. coli in KCl solutions using Pt and Ag/AgCl gate electrodes (He et al. 2012). Wu et al. used a graphene-based FET to detect E. coli in nutrient broth diluted with phosphate buffered saline solution with amperometry using a Ag/AgCl gate electrode (Wu et al. 2016).\nFurther examples of amperometric sensing include the detection of human influenza A virus by Singh et al. using a reduced graphene oxide-based electrode and chronoamperometry using Fe(CN)6 3 - /4- at a LOD of 0.5 plaque-forming units (PFU)/mL (Singh et al. 2017b). Lee and Jun utilized wire-based electrodes for amperometric detection of E. coli and S. aureus (Lee and Jun 2016). A detailed list of studies that utilize amperometric methods for pathogen detection is provided in Table 1, Table 2\n\n3.3.2.1 Linear sweep and cyclic voltammetry\nLinear sweep voltammetry (LSV) methods are those in which a current is measured in response to an applied electrical potential that is swept at a constant rate across a range of electrical potentials (Bard and Faulkner, 2000). Cyclic voltammetry (CV) is a commonly used linear-sweep method in which the electrical potential is swept in both the forward and reverse directions in partial cycles, full cycles, or a series of cycles. CV is one of the most widely used voltammetric methods for pathogen detection.\nHong et al. used sweep voltammetry to detect norovirus in a sample solution with Fe(CN)6 3 - /4- extracted from lettuce (Hong et al. 2015). A typical CV response using Fe(CN)6 3 - /4- associated with pathogen detection is shown in Fig. 5 a for various concentrations of E. coli binding to a polymer composite electrode (Güner et al. 2017). A detailed overview of pathogen detection studies based on CV is provided in Table 1, Table 2.\nFig. 5 Typical responses associated with the common electrochemical methods used for pathogen detection. a) Cyclic voltammetry (CV) data using Fe(CN)63-/4- for varying concentrations of E. coli (Güner et al. 2017). b) Differential pulse voltammetry (DPV) data using Fe(CN)63-/4- for varying concentrations of S. aureus (Bhardwaj et al. 2017). c) Electrochemical impedance spectroscopy (EIS) in 100 mM LiClO4 solution in the form of a Nyquist plot and corresponding equivalent circuit model associated with biorecognition element immobilization and detection of S. typhimurium (Sheikhzadeh et al. 2016). d) Conductometry data for varying concentrations of B. subtilis (Yoo et al. 2017).\n\n3.3.2.2 Pulse voltammetry\nPulse voltammetry is a type of voltammetry in which the electrical potential is applied in pulses. The technique has the advantage of improved speed and sensitivity relative to traditional voltammetric techniques (Bard and Faulkner, 2000; Molina and González, 2016). In staircase voltammetry, the electrical potential is pulsed in a series of stair steps and the current is measured following each step change, which reduces the effect of capacitive charging on the current signal. Square wave voltammetry (SWV) is a type of staircase voltammetry that applies a symmetric square-wave pulse superimposed on a staircase potential waveform. The forward pulse of the waveform coincides with the staircase step. In differential pulse voltammetry (DPV), the electrical potential is scanned with a series of fixed amplitude pulses and superimposed on a changing base potential. The current is measured before the pulse application and again at the end of the pulse, which allows for the decay of the nonfaradaic current (Scott, 2016).\nFor example, Iqbal et al. used SWV with AuNP-modified carbon electrodes for detection of C. parvum in samples taken from fruit (Iqbal et al. 2015). Kitajima et al. also used SWV with Au microelectrodes to detect norovirus at a LOD of 10 PFU/mL (Kitajima et al. 2016). Cheng et al. used DPV and a nanostructured alumina electrode for detection of dengue type 2 virus with a LOD of 1 PFU/mL (Cheng et al. 2012). As shown in Fig. 5b, Bhardwaj et al. used DPV with a carbon-based electrode to detect S. aureus (Bhardwaj et al. 2017). Additional studies that utilize pulse voltammetry methods forpathogen detection are listed in Table 1, Table 2.\n\n3.3.2.3 Stripping voltammetry\nMany of the previously described voltammetric methods can be modified to include a step that pre-concentrates the target on the electrode surface. Subsequently, the pre-concentrated target is stripped from the surface by application of an electrical potential. In anodic stripping voltammetry (ASV), a negative potential is used to pre-concentrate metal ions onto the electrode surface. These ions are then stripped from the surface by applied positive potentials. Although most commonly used to detect trace amounts of metals, this method has been adapted for pathogen detection by electrocatalytically coating metallic labels on bound targets for oxidative stripping and subsequently measuring the current response (Abbaspour et al. 2015).\nChen et al. used stripping voltammetry with a polymer-CNT composite-based electrode to detect E. coli at a LOD of 13 CFU/mL (Chen et al. 2014). In that study, the biosensor was first incubated with E. coli. Silica-coated Ag nanoparticles conjugated with anti-E.coli were subsequently introduced to the system, inducing a binding reaction between the bacteria and the nanoparticles. After rinsing non-specifically bound particles, acid was introduced to dissolve Ag(s), and the resulting Ag+-rich solution was characterized using DPV. Viswanathan et al. used ASV with screen-printed composite electrodes for multiplexed detection of Campylobacter, S. typhimurium, and E. coli with a LOD of 400 cells/mL, 400 cells/mL, and 800 cells/mL, respectively (Viswanathan et al. 2012). In that study, antibody-functionalized nanocrystalline bioconjugates were first introduced to biosensor-bound bacteria, the specifically bound particles were dissolved with acid, and the ions were then stripped using a square-wave voltammetric waveform. Additional studies using stripping voltammetry for electrochemical detection of pathogens can be found in Table 1, Table 2."}