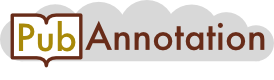
PMC:7143804 / 3449-9317
Annnotations
LitCovid-PubTator
{"project":"LitCovid-PubTator","denotations":[{"id":"69","span":{"begin":2024,"end":2032},"obj":"Chemical"},{"id":"70","span":{"begin":2584,"end":2589},"obj":"Chemical"},{"id":"71","span":{"begin":2620,"end":2622},"obj":"Chemical"},{"id":"76","span":{"begin":3832,"end":3852},"obj":"Chemical"},{"id":"77","span":{"begin":3854,"end":3858},"obj":"Chemical"},{"id":"78","span":{"begin":4269,"end":4292},"obj":"Chemical"},{"id":"79","span":{"begin":4294,"end":4297},"obj":"Chemical"},{"id":"86","span":{"begin":4769,"end":4774},"obj":"Chemical"},{"id":"87","span":{"begin":4908,"end":4913},"obj":"Chemical"},{"id":"88","span":{"begin":4966,"end":4969},"obj":"Chemical"},{"id":"89","span":{"begin":4978,"end":4983},"obj":"Chemical"},{"id":"90","span":{"begin":5103,"end":5108},"obj":"Chemical"},{"id":"91","span":{"begin":5766,"end":5771},"obj":"Chemical"}],"attributes":[{"id":"A69","pred":"tao:has_database_id","subj":"69","obj":"MESH:D011108"},{"id":"A70","pred":"tao:has_database_id","subj":"70","obj":"MESH:D008670"},{"id":"A71","pred":"tao:has_database_id","subj":"71","obj":"MESH:D003300"},{"id":"A76","pred":"tao:has_database_id","subj":"76","obj":"MESH:C013830"},{"id":"A77","pred":"tao:has_database_id","subj":"77","obj":"MESH:C013830"},{"id":"A86","pred":"tao:has_database_id","subj":"86","obj":"MESH:D008670"},{"id":"A87","pred":"tao:has_database_id","subj":"87","obj":"MESH:D008670"},{"id":"A89","pred":"tao:has_database_id","subj":"89","obj":"MESH:D008670"},{"id":"A90","pred":"tao:has_database_id","subj":"90","obj":"MESH:D008670"},{"id":"A91","pred":"tao:has_database_id","subj":"91","obj":"MESH:D008670"}],"namespaces":[{"prefix":"Tax","uri":"https://www.ncbi.nlm.nih.gov/taxonomy/"},{"prefix":"MESH","uri":"https://id.nlm.nih.gov/mesh/"},{"prefix":"Gene","uri":"https://www.ncbi.nlm.nih.gov/gene/"},{"prefix":"CVCL","uri":"https://web.expasy.org/cellosaurus/CVCL_"}],"text":"1.1. State-of-the-Art\nIn the past, several chip-based DNA and RNA amplification devices are reported. It goes beyond the scope of this paper to discuss the state-of-the-art of DNA amplification chips in-depth. There are several good review papers written on this topic [12,13,14,15,16,17,18,19,20]. Readers are referred to these for a comprehensive overview of the field. In this paper, the state-of-the-art is divided into several discussion points, i.e., the heating method, the temperature control method, and the substrate material and fabrication technique. These points will be discussed separately.\nWith respect to heat supply, different methods have been employed. Almassian et al. give a comprehensive overview of different possible heating methods in their review paper [12]. Not all of the mentioned methods are easy to implement in low-cost and portable lab-on-a-chip devices due to their bulkiness or implementation costs. Examples of these rather difficult methods are using heating via induction, infrared, or microwave radiation. Others are not useful due ot their challenging temperature control, like with heating up the system using exothermic reactions. Within the field of DNA amplification, different mechanisms of amplification exist. Some are based on thermo cycling processes, e.g., PCR, whereas others are isothermal. The use of an isothermal amplification technique puts less requirements on the heaters. Isothermal processes are either truly isothermal or consisting of three different temperatures, as they have a thermal denaturation step before and a termination step after the elongation step. The switching between these temperature steps does not have to be as fast as with thermal cycling steps in, for example, PCR amplification reactions. The use of less temperature variations makes it easier to maintain the set temperature as there is less heating an cooling involved. Furthermore, it eliminates the use of a continuous flow approach in systems with low thermal conductivities, e.g., polymers. Therefore, it is easier to implement within lab-on-a-chip devices [17,21]. Isothermal DNA amplification reactions can already be performed by putting the chip on a commercially available hotplate [22,23] or Peltier elements [24,25]. However, these heating systems are bulky and power-consuming. Therefore, they are not useful for portable equipment or operation at remote locations. Miniaturizing heaters lowers the bulkiness and power consumption. Miniaturized heaters can be integrated as integrated resistive heaters, e.g., as deposited thin-film metal [26,27,28,29] or as laminated Cu foil [30], or as micro-Peltier elements [31,32]. These miniaturized heaters can be implemented directly onto the microfluidic chip [28] or on a different substrate and leter incorporated onto the microfluidic chip [33,34,35,36]. The geometry of such a heater contributes significantly to the uniformity of the heat distribution within the chip [26,37]. One method to accurately control the temperature is the use of a proportional-integral-derivative (PID) controlled thermostat. These PID controllers are coupled to the electrical heaters and use a thermocouple as feedback-loop to the controller [22,23,24,25].\nThere are various materials that can be used to fabricate lab-on-a-chip devices for DNA or RNA amplification. In the past 15 years, more than ten polymers, ceramic materials, and metals have successfully been used to fabricate such devices [15]. The major property playing a role here is the biocompatibility of the material. The surface of the microfluidic structure should not inhibit the amplification reaction. This biocompatibility can be an intrinsic property of the material or the surface can be modified or coated to achieve this [12,13,14,15,16,17,18,19,20]. One often used material is polydimethylsiloxane (PDMS) [22,23,31,32,34,35,38], which can be processed using soft lithography [39]. However, this is a fabrication technology used in academia and is not suitable for upscaling to mass production [40]. Fabrication methods suitable for mass production are thermoforming/embossing or injection molding [41]. One of the materials which is biocompatible and suitable for both industrial scale fabrication technologies is cyclic olefin copolymer (COC) [42], which is one of the materials used in the past as well [28,36,43,44,45]. Guckenberger et al. estimates the costs of injection molding of only 50 simple microfluidic devices on $47, but this becomes cheaper when the mass production stage is reached [41]. Another benefit of COC is the possibility to shape it using micromilling. This technique is a rapid prototyping technology and therefore very useful within proof-of-concept projects [41].\nIntegrating resistive metal tracks onto a COC substrate have also been done in the past. Some papers describe the use of a surface modification step done before metal deposition in order to enhance adhesion between the COC and the metal layer, like a pretreatment with plasma [46] or an organic solvent [47]. Other papers describe the direct deposition of metal onto the COC surface [28,48]. Chung et al. specifically, fabricated an amplification chip in COC with integrated Au heaters [28]. However, their system required heating from both sides as the used grade of COC has a glass transition temperature (Tg) of 130 °C. This COC could not withstand the required heater temperatures to have enough heat flux into the system. They had to heat up the heater to temperatures above 130 °C, which caused cracking of the heater tracks due to deformation of the COC. With their double-sided heating they ensured that the reaction mixture had the desired PCR temperatures. However, double-sided heating doubles the amount of metal required, increases the amount of fabrication steps, and therefore increases the price per chip."}
LitCovid-PD-FMA-UBERON
{"project":"LitCovid-PD-FMA-UBERON","denotations":[{"id":"T13","span":{"begin":54,"end":57},"obj":"Body_part"},{"id":"T14","span":{"begin":62,"end":65},"obj":"Body_part"},{"id":"T15","span":{"begin":176,"end":179},"obj":"Body_part"},{"id":"T16","span":{"begin":1194,"end":1197},"obj":"Body_part"},{"id":"T17","span":{"begin":2120,"end":2123},"obj":"Body_part"},{"id":"T18","span":{"begin":3320,"end":3323},"obj":"Body_part"},{"id":"T19","span":{"begin":3327,"end":3330},"obj":"Body_part"},{"id":"T20","span":{"begin":5016,"end":5022},"obj":"Body_part"}],"attributes":[{"id":"A13","pred":"fma_id","subj":"T13","obj":"http://purl.org/sig/ont/fma/fma74412"},{"id":"A14","pred":"fma_id","subj":"T14","obj":"http://purl.org/sig/ont/fma/fma67095"},{"id":"A15","pred":"fma_id","subj":"T15","obj":"http://purl.org/sig/ont/fma/fma74412"},{"id":"A16","pred":"fma_id","subj":"T16","obj":"http://purl.org/sig/ont/fma/fma74412"},{"id":"A17","pred":"fma_id","subj":"T17","obj":"http://purl.org/sig/ont/fma/fma74412"},{"id":"A18","pred":"fma_id","subj":"T18","obj":"http://purl.org/sig/ont/fma/fma74412"},{"id":"A19","pred":"fma_id","subj":"T19","obj":"http://purl.org/sig/ont/fma/fma67095"},{"id":"A20","pred":"fma_id","subj":"T20","obj":"http://purl.org/sig/ont/fma/fma62970"}],"text":"1.1. State-of-the-Art\nIn the past, several chip-based DNA and RNA amplification devices are reported. It goes beyond the scope of this paper to discuss the state-of-the-art of DNA amplification chips in-depth. There are several good review papers written on this topic [12,13,14,15,16,17,18,19,20]. Readers are referred to these for a comprehensive overview of the field. In this paper, the state-of-the-art is divided into several discussion points, i.e., the heating method, the temperature control method, and the substrate material and fabrication technique. These points will be discussed separately.\nWith respect to heat supply, different methods have been employed. Almassian et al. give a comprehensive overview of different possible heating methods in their review paper [12]. Not all of the mentioned methods are easy to implement in low-cost and portable lab-on-a-chip devices due to their bulkiness or implementation costs. Examples of these rather difficult methods are using heating via induction, infrared, or microwave radiation. Others are not useful due ot their challenging temperature control, like with heating up the system using exothermic reactions. Within the field of DNA amplification, different mechanisms of amplification exist. Some are based on thermo cycling processes, e.g., PCR, whereas others are isothermal. The use of an isothermal amplification technique puts less requirements on the heaters. Isothermal processes are either truly isothermal or consisting of three different temperatures, as they have a thermal denaturation step before and a termination step after the elongation step. The switching between these temperature steps does not have to be as fast as with thermal cycling steps in, for example, PCR amplification reactions. The use of less temperature variations makes it easier to maintain the set temperature as there is less heating an cooling involved. Furthermore, it eliminates the use of a continuous flow approach in systems with low thermal conductivities, e.g., polymers. Therefore, it is easier to implement within lab-on-a-chip devices [17,21]. Isothermal DNA amplification reactions can already be performed by putting the chip on a commercially available hotplate [22,23] or Peltier elements [24,25]. However, these heating systems are bulky and power-consuming. Therefore, they are not useful for portable equipment or operation at remote locations. Miniaturizing heaters lowers the bulkiness and power consumption. Miniaturized heaters can be integrated as integrated resistive heaters, e.g., as deposited thin-film metal [26,27,28,29] or as laminated Cu foil [30], or as micro-Peltier elements [31,32]. These miniaturized heaters can be implemented directly onto the microfluidic chip [28] or on a different substrate and leter incorporated onto the microfluidic chip [33,34,35,36]. The geometry of such a heater contributes significantly to the uniformity of the heat distribution within the chip [26,37]. One method to accurately control the temperature is the use of a proportional-integral-derivative (PID) controlled thermostat. These PID controllers are coupled to the electrical heaters and use a thermocouple as feedback-loop to the controller [22,23,24,25].\nThere are various materials that can be used to fabricate lab-on-a-chip devices for DNA or RNA amplification. In the past 15 years, more than ten polymers, ceramic materials, and metals have successfully been used to fabricate such devices [15]. The major property playing a role here is the biocompatibility of the material. The surface of the microfluidic structure should not inhibit the amplification reaction. This biocompatibility can be an intrinsic property of the material or the surface can be modified or coated to achieve this [12,13,14,15,16,17,18,19,20]. One often used material is polydimethylsiloxane (PDMS) [22,23,31,32,34,35,38], which can be processed using soft lithography [39]. However, this is a fabrication technology used in academia and is not suitable for upscaling to mass production [40]. Fabrication methods suitable for mass production are thermoforming/embossing or injection molding [41]. One of the materials which is biocompatible and suitable for both industrial scale fabrication technologies is cyclic olefin copolymer (COC) [42], which is one of the materials used in the past as well [28,36,43,44,45]. Guckenberger et al. estimates the costs of injection molding of only 50 simple microfluidic devices on $47, but this becomes cheaper when the mass production stage is reached [41]. Another benefit of COC is the possibility to shape it using micromilling. This technique is a rapid prototyping technology and therefore very useful within proof-of-concept projects [41].\nIntegrating resistive metal tracks onto a COC substrate have also been done in the past. Some papers describe the use of a surface modification step done before metal deposition in order to enhance adhesion between the COC and the metal layer, like a pretreatment with plasma [46] or an organic solvent [47]. Other papers describe the direct deposition of metal onto the COC surface [28,48]. Chung et al. specifically, fabricated an amplification chip in COC with integrated Au heaters [28]. However, their system required heating from both sides as the used grade of COC has a glass transition temperature (Tg) of 130 °C. This COC could not withstand the required heater temperatures to have enough heat flux into the system. They had to heat up the heater to temperatures above 130 °C, which caused cracking of the heater tracks due to deformation of the COC. With their double-sided heating they ensured that the reaction mixture had the desired PCR temperatures. However, double-sided heating doubles the amount of metal required, increases the amount of fabrication steps, and therefore increases the price per chip."}
LitCovid-PD-UBERON
{"project":"LitCovid-PD-UBERON","denotations":[{"id":"T1","span":{"begin":4235,"end":4240},"obj":"Body_part"}],"attributes":[{"id":"A1","pred":"uberon_id","subj":"T1","obj":"http://purl.obolibrary.org/obo/UBERON_0002542"}],"text":"1.1. State-of-the-Art\nIn the past, several chip-based DNA and RNA amplification devices are reported. It goes beyond the scope of this paper to discuss the state-of-the-art of DNA amplification chips in-depth. There are several good review papers written on this topic [12,13,14,15,16,17,18,19,20]. Readers are referred to these for a comprehensive overview of the field. In this paper, the state-of-the-art is divided into several discussion points, i.e., the heating method, the temperature control method, and the substrate material and fabrication technique. These points will be discussed separately.\nWith respect to heat supply, different methods have been employed. Almassian et al. give a comprehensive overview of different possible heating methods in their review paper [12]. Not all of the mentioned methods are easy to implement in low-cost and portable lab-on-a-chip devices due to their bulkiness or implementation costs. Examples of these rather difficult methods are using heating via induction, infrared, or microwave radiation. Others are not useful due ot their challenging temperature control, like with heating up the system using exothermic reactions. Within the field of DNA amplification, different mechanisms of amplification exist. Some are based on thermo cycling processes, e.g., PCR, whereas others are isothermal. The use of an isothermal amplification technique puts less requirements on the heaters. Isothermal processes are either truly isothermal or consisting of three different temperatures, as they have a thermal denaturation step before and a termination step after the elongation step. The switching between these temperature steps does not have to be as fast as with thermal cycling steps in, for example, PCR amplification reactions. The use of less temperature variations makes it easier to maintain the set temperature as there is less heating an cooling involved. Furthermore, it eliminates the use of a continuous flow approach in systems with low thermal conductivities, e.g., polymers. Therefore, it is easier to implement within lab-on-a-chip devices [17,21]. Isothermal DNA amplification reactions can already be performed by putting the chip on a commercially available hotplate [22,23] or Peltier elements [24,25]. However, these heating systems are bulky and power-consuming. Therefore, they are not useful for portable equipment or operation at remote locations. Miniaturizing heaters lowers the bulkiness and power consumption. Miniaturized heaters can be integrated as integrated resistive heaters, e.g., as deposited thin-film metal [26,27,28,29] or as laminated Cu foil [30], or as micro-Peltier elements [31,32]. These miniaturized heaters can be implemented directly onto the microfluidic chip [28] or on a different substrate and leter incorporated onto the microfluidic chip [33,34,35,36]. The geometry of such a heater contributes significantly to the uniformity of the heat distribution within the chip [26,37]. One method to accurately control the temperature is the use of a proportional-integral-derivative (PID) controlled thermostat. These PID controllers are coupled to the electrical heaters and use a thermocouple as feedback-loop to the controller [22,23,24,25].\nThere are various materials that can be used to fabricate lab-on-a-chip devices for DNA or RNA amplification. In the past 15 years, more than ten polymers, ceramic materials, and metals have successfully been used to fabricate such devices [15]. The major property playing a role here is the biocompatibility of the material. The surface of the microfluidic structure should not inhibit the amplification reaction. This biocompatibility can be an intrinsic property of the material or the surface can be modified or coated to achieve this [12,13,14,15,16,17,18,19,20]. One often used material is polydimethylsiloxane (PDMS) [22,23,31,32,34,35,38], which can be processed using soft lithography [39]. However, this is a fabrication technology used in academia and is not suitable for upscaling to mass production [40]. Fabrication methods suitable for mass production are thermoforming/embossing or injection molding [41]. One of the materials which is biocompatible and suitable for both industrial scale fabrication technologies is cyclic olefin copolymer (COC) [42], which is one of the materials used in the past as well [28,36,43,44,45]. Guckenberger et al. estimates the costs of injection molding of only 50 simple microfluidic devices on $47, but this becomes cheaper when the mass production stage is reached [41]. Another benefit of COC is the possibility to shape it using micromilling. This technique is a rapid prototyping technology and therefore very useful within proof-of-concept projects [41].\nIntegrating resistive metal tracks onto a COC substrate have also been done in the past. Some papers describe the use of a surface modification step done before metal deposition in order to enhance adhesion between the COC and the metal layer, like a pretreatment with plasma [46] or an organic solvent [47]. Other papers describe the direct deposition of metal onto the COC surface [28,48]. Chung et al. specifically, fabricated an amplification chip in COC with integrated Au heaters [28]. However, their system required heating from both sides as the used grade of COC has a glass transition temperature (Tg) of 130 °C. This COC could not withstand the required heater temperatures to have enough heat flux into the system. They had to heat up the heater to temperatures above 130 °C, which caused cracking of the heater tracks due to deformation of the COC. With their double-sided heating they ensured that the reaction mixture had the desired PCR temperatures. However, double-sided heating doubles the amount of metal required, increases the amount of fabrication steps, and therefore increases the price per chip."}
LitCovid-PD-MONDO
{"project":"LitCovid-PD-MONDO","denotations":[{"id":"T17","span":{"begin":3075,"end":3078},"obj":"Disease"},{"id":"T18","span":{"begin":3109,"end":3112},"obj":"Disease"}],"attributes":[{"id":"A17","pred":"mondo_id","subj":"T17","obj":"http://purl.obolibrary.org/obo/MONDO_0000922"},{"id":"A18","pred":"mondo_id","subj":"T18","obj":"http://purl.obolibrary.org/obo/MONDO_0000922"}],"text":"1.1. State-of-the-Art\nIn the past, several chip-based DNA and RNA amplification devices are reported. It goes beyond the scope of this paper to discuss the state-of-the-art of DNA amplification chips in-depth. There are several good review papers written on this topic [12,13,14,15,16,17,18,19,20]. Readers are referred to these for a comprehensive overview of the field. In this paper, the state-of-the-art is divided into several discussion points, i.e., the heating method, the temperature control method, and the substrate material and fabrication technique. These points will be discussed separately.\nWith respect to heat supply, different methods have been employed. Almassian et al. give a comprehensive overview of different possible heating methods in their review paper [12]. Not all of the mentioned methods are easy to implement in low-cost and portable lab-on-a-chip devices due to their bulkiness or implementation costs. Examples of these rather difficult methods are using heating via induction, infrared, or microwave radiation. Others are not useful due ot their challenging temperature control, like with heating up the system using exothermic reactions. Within the field of DNA amplification, different mechanisms of amplification exist. Some are based on thermo cycling processes, e.g., PCR, whereas others are isothermal. The use of an isothermal amplification technique puts less requirements on the heaters. Isothermal processes are either truly isothermal or consisting of three different temperatures, as they have a thermal denaturation step before and a termination step after the elongation step. The switching between these temperature steps does not have to be as fast as with thermal cycling steps in, for example, PCR amplification reactions. The use of less temperature variations makes it easier to maintain the set temperature as there is less heating an cooling involved. Furthermore, it eliminates the use of a continuous flow approach in systems with low thermal conductivities, e.g., polymers. Therefore, it is easier to implement within lab-on-a-chip devices [17,21]. Isothermal DNA amplification reactions can already be performed by putting the chip on a commercially available hotplate [22,23] or Peltier elements [24,25]. However, these heating systems are bulky and power-consuming. Therefore, they are not useful for portable equipment or operation at remote locations. Miniaturizing heaters lowers the bulkiness and power consumption. Miniaturized heaters can be integrated as integrated resistive heaters, e.g., as deposited thin-film metal [26,27,28,29] or as laminated Cu foil [30], or as micro-Peltier elements [31,32]. These miniaturized heaters can be implemented directly onto the microfluidic chip [28] or on a different substrate and leter incorporated onto the microfluidic chip [33,34,35,36]. The geometry of such a heater contributes significantly to the uniformity of the heat distribution within the chip [26,37]. One method to accurately control the temperature is the use of a proportional-integral-derivative (PID) controlled thermostat. These PID controllers are coupled to the electrical heaters and use a thermocouple as feedback-loop to the controller [22,23,24,25].\nThere are various materials that can be used to fabricate lab-on-a-chip devices for DNA or RNA amplification. In the past 15 years, more than ten polymers, ceramic materials, and metals have successfully been used to fabricate such devices [15]. The major property playing a role here is the biocompatibility of the material. The surface of the microfluidic structure should not inhibit the amplification reaction. This biocompatibility can be an intrinsic property of the material or the surface can be modified or coated to achieve this [12,13,14,15,16,17,18,19,20]. One often used material is polydimethylsiloxane (PDMS) [22,23,31,32,34,35,38], which can be processed using soft lithography [39]. However, this is a fabrication technology used in academia and is not suitable for upscaling to mass production [40]. Fabrication methods suitable for mass production are thermoforming/embossing or injection molding [41]. One of the materials which is biocompatible and suitable for both industrial scale fabrication technologies is cyclic olefin copolymer (COC) [42], which is one of the materials used in the past as well [28,36,43,44,45]. Guckenberger et al. estimates the costs of injection molding of only 50 simple microfluidic devices on $47, but this becomes cheaper when the mass production stage is reached [41]. Another benefit of COC is the possibility to shape it using micromilling. This technique is a rapid prototyping technology and therefore very useful within proof-of-concept projects [41].\nIntegrating resistive metal tracks onto a COC substrate have also been done in the past. Some papers describe the use of a surface modification step done before metal deposition in order to enhance adhesion between the COC and the metal layer, like a pretreatment with plasma [46] or an organic solvent [47]. Other papers describe the direct deposition of metal onto the COC surface [28,48]. Chung et al. specifically, fabricated an amplification chip in COC with integrated Au heaters [28]. However, their system required heating from both sides as the used grade of COC has a glass transition temperature (Tg) of 130 °C. This COC could not withstand the required heater temperatures to have enough heat flux into the system. They had to heat up the heater to temperatures above 130 °C, which caused cracking of the heater tracks due to deformation of the COC. With their double-sided heating they ensured that the reaction mixture had the desired PCR temperatures. However, double-sided heating doubles the amount of metal required, increases the amount of fabrication steps, and therefore increases the price per chip."}
LitCovid-PD-CLO
{"project":"LitCovid-PD-CLO","denotations":[{"id":"T32","span":{"begin":80,"end":87},"obj":"http://purl.obolibrary.org/obo/OBI_0000968"},{"id":"T33","span":{"begin":333,"end":334},"obj":"http://purl.obolibrary.org/obo/CLO_0001020"},{"id":"T34","span":{"begin":365,"end":370},"obj":"http://purl.obolibrary.org/obo/UBERON_0007688"},{"id":"T35","span":{"begin":695,"end":696},"obj":"http://purl.obolibrary.org/obo/CLO_0001020"},{"id":"T36","span":{"begin":873,"end":874},"obj":"http://purl.obolibrary.org/obo/CLO_0001020"},{"id":"T37","span":{"begin":880,"end":887},"obj":"http://purl.obolibrary.org/obo/OBI_0000968"},{"id":"T38","span":{"begin":1185,"end":1190},"obj":"http://purl.obolibrary.org/obo/UBERON_0007688"},{"id":"T39","span":{"begin":1541,"end":1542},"obj":"http://purl.obolibrary.org/obo/CLO_0001020"},{"id":"T40","span":{"begin":1580,"end":1581},"obj":"http://purl.obolibrary.org/obo/CLO_0001020"},{"id":"T41","span":{"begin":1947,"end":1948},"obj":"http://purl.obolibrary.org/obo/CLO_0001020"},{"id":"T42","span":{"begin":2085,"end":2086},"obj":"http://purl.obolibrary.org/obo/CLO_0001020"},{"id":"T43","span":{"begin":2092,"end":2099},"obj":"http://purl.obolibrary.org/obo/OBI_0000968"},{"id":"T44","span":{"begin":2196,"end":2197},"obj":"http://purl.obolibrary.org/obo/CLO_0001020"},{"id":"T45","span":{"begin":2765,"end":2766},"obj":"http://purl.obolibrary.org/obo/CLO_0001020"},{"id":"T46","span":{"begin":2873,"end":2874},"obj":"http://purl.obolibrary.org/obo/CLO_0001020"},{"id":"T47","span":{"begin":3039,"end":3040},"obj":"http://purl.obolibrary.org/obo/CLO_0001020"},{"id":"T48","span":{"begin":3171,"end":3172},"obj":"http://purl.obolibrary.org/obo/CLO_0001020"},{"id":"T49","span":{"begin":3301,"end":3302},"obj":"http://purl.obolibrary.org/obo/CLO_0001020"},{"id":"T50","span":{"begin":3308,"end":3315},"obj":"http://purl.obolibrary.org/obo/OBI_0000968"},{"id":"T51","span":{"begin":3378,"end":3381},"obj":"http://purl.obolibrary.org/obo/CLO_0050884"},{"id":"T52","span":{"begin":3468,"end":3475},"obj":"http://purl.obolibrary.org/obo/OBI_0000968"},{"id":"T53","span":{"begin":3509,"end":3510},"obj":"http://purl.obolibrary.org/obo/CLO_0001020"},{"id":"T54","span":{"begin":3953,"end":3954},"obj":"http://purl.obolibrary.org/obo/CLO_0001020"},{"id":"T55","span":{"begin":4153,"end":4155},"obj":"http://purl.obolibrary.org/obo/CLO_0053794"},{"id":"T56","span":{"begin":4470,"end":4477},"obj":"http://purl.obolibrary.org/obo/OBI_0000968"},{"id":"T57","span":{"begin":4554,"end":4556},"obj":"http://purl.obolibrary.org/obo/CLO_0053794"},{"id":"T58","span":{"begin":4651,"end":4652},"obj":"http://purl.obolibrary.org/obo/CLO_0001020"},{"id":"T59","span":{"begin":4742,"end":4744},"obj":"http://purl.obolibrary.org/obo/CLO_0053794"},{"id":"T60","span":{"begin":4787,"end":4788},"obj":"http://purl.obolibrary.org/obo/CLO_0001020"},{"id":"T61","span":{"begin":4868,"end":4869},"obj":"http://purl.obolibrary.org/obo/CLO_0001020"},{"id":"T62","span":{"begin":4996,"end":4997},"obj":"http://purl.obolibrary.org/obo/CLO_0001020"},{"id":"T63","span":{"begin":5016,"end":5022},"obj":"http://purl.obolibrary.org/obo/UBERON_0001969"},{"id":"T64","span":{"begin":5034,"end":5041},"obj":"http://purl.obolibrary.org/obo/OBI_0100026"},{"id":"T65","span":{"begin":5034,"end":5041},"obj":"http://purl.obolibrary.org/obo/UBERON_0000468"},{"id":"T66","span":{"begin":5319,"end":5322},"obj":"http://purl.obolibrary.org/obo/CLO_0051582"},{"id":"T67","span":{"begin":5323,"end":5324},"obj":"http://purl.obolibrary.org/obo/CLO_0001020"}],"text":"1.1. State-of-the-Art\nIn the past, several chip-based DNA and RNA amplification devices are reported. It goes beyond the scope of this paper to discuss the state-of-the-art of DNA amplification chips in-depth. There are several good review papers written on this topic [12,13,14,15,16,17,18,19,20]. Readers are referred to these for a comprehensive overview of the field. In this paper, the state-of-the-art is divided into several discussion points, i.e., the heating method, the temperature control method, and the substrate material and fabrication technique. These points will be discussed separately.\nWith respect to heat supply, different methods have been employed. Almassian et al. give a comprehensive overview of different possible heating methods in their review paper [12]. Not all of the mentioned methods are easy to implement in low-cost and portable lab-on-a-chip devices due to their bulkiness or implementation costs. Examples of these rather difficult methods are using heating via induction, infrared, or microwave radiation. Others are not useful due ot their challenging temperature control, like with heating up the system using exothermic reactions. Within the field of DNA amplification, different mechanisms of amplification exist. Some are based on thermo cycling processes, e.g., PCR, whereas others are isothermal. The use of an isothermal amplification technique puts less requirements on the heaters. Isothermal processes are either truly isothermal or consisting of three different temperatures, as they have a thermal denaturation step before and a termination step after the elongation step. The switching between these temperature steps does not have to be as fast as with thermal cycling steps in, for example, PCR amplification reactions. The use of less temperature variations makes it easier to maintain the set temperature as there is less heating an cooling involved. Furthermore, it eliminates the use of a continuous flow approach in systems with low thermal conductivities, e.g., polymers. Therefore, it is easier to implement within lab-on-a-chip devices [17,21]. Isothermal DNA amplification reactions can already be performed by putting the chip on a commercially available hotplate [22,23] or Peltier elements [24,25]. However, these heating systems are bulky and power-consuming. Therefore, they are not useful for portable equipment or operation at remote locations. Miniaturizing heaters lowers the bulkiness and power consumption. Miniaturized heaters can be integrated as integrated resistive heaters, e.g., as deposited thin-film metal [26,27,28,29] or as laminated Cu foil [30], or as micro-Peltier elements [31,32]. These miniaturized heaters can be implemented directly onto the microfluidic chip [28] or on a different substrate and leter incorporated onto the microfluidic chip [33,34,35,36]. The geometry of such a heater contributes significantly to the uniformity of the heat distribution within the chip [26,37]. One method to accurately control the temperature is the use of a proportional-integral-derivative (PID) controlled thermostat. These PID controllers are coupled to the electrical heaters and use a thermocouple as feedback-loop to the controller [22,23,24,25].\nThere are various materials that can be used to fabricate lab-on-a-chip devices for DNA or RNA amplification. In the past 15 years, more than ten polymers, ceramic materials, and metals have successfully been used to fabricate such devices [15]. The major property playing a role here is the biocompatibility of the material. The surface of the microfluidic structure should not inhibit the amplification reaction. This biocompatibility can be an intrinsic property of the material or the surface can be modified or coated to achieve this [12,13,14,15,16,17,18,19,20]. One often used material is polydimethylsiloxane (PDMS) [22,23,31,32,34,35,38], which can be processed using soft lithography [39]. However, this is a fabrication technology used in academia and is not suitable for upscaling to mass production [40]. Fabrication methods suitable for mass production are thermoforming/embossing or injection molding [41]. One of the materials which is biocompatible and suitable for both industrial scale fabrication technologies is cyclic olefin copolymer (COC) [42], which is one of the materials used in the past as well [28,36,43,44,45]. Guckenberger et al. estimates the costs of injection molding of only 50 simple microfluidic devices on $47, but this becomes cheaper when the mass production stage is reached [41]. Another benefit of COC is the possibility to shape it using micromilling. This technique is a rapid prototyping technology and therefore very useful within proof-of-concept projects [41].\nIntegrating resistive metal tracks onto a COC substrate have also been done in the past. Some papers describe the use of a surface modification step done before metal deposition in order to enhance adhesion between the COC and the metal layer, like a pretreatment with plasma [46] or an organic solvent [47]. Other papers describe the direct deposition of metal onto the COC surface [28,48]. Chung et al. specifically, fabricated an amplification chip in COC with integrated Au heaters [28]. However, their system required heating from both sides as the used grade of COC has a glass transition temperature (Tg) of 130 °C. This COC could not withstand the required heater temperatures to have enough heat flux into the system. They had to heat up the heater to temperatures above 130 °C, which caused cracking of the heater tracks due to deformation of the COC. With their double-sided heating they ensured that the reaction mixture had the desired PCR temperatures. However, double-sided heating doubles the amount of metal required, increases the amount of fabrication steps, and therefore increases the price per chip."}
LitCovid-PD-CHEBI
{"project":"LitCovid-PD-CHEBI","denotations":[{"id":"T19","span":{"begin":54,"end":57},"obj":"Chemical"},{"id":"T20","span":{"begin":62,"end":65},"obj":"Chemical"},{"id":"T21","span":{"begin":176,"end":179},"obj":"Chemical"},{"id":"T22","span":{"begin":1194,"end":1197},"obj":"Chemical"},{"id":"T23","span":{"begin":2024,"end":2032},"obj":"Chemical"},{"id":"T24","span":{"begin":2120,"end":2123},"obj":"Chemical"},{"id":"T25","span":{"begin":2620,"end":2622},"obj":"Chemical"},{"id":"T26","span":{"begin":3075,"end":3078},"obj":"Chemical"},{"id":"T27","span":{"begin":3109,"end":3112},"obj":"Chemical"},{"id":"T28","span":{"begin":3320,"end":3323},"obj":"Chemical"},{"id":"T29","span":{"begin":3327,"end":3330},"obj":"Chemical"},{"id":"T30","span":{"begin":3382,"end":3390},"obj":"Chemical"},{"id":"T31","span":{"begin":3415,"end":3421},"obj":"Chemical"},{"id":"T32","span":{"begin":3832,"end":3852},"obj":"Chemical"},{"id":"T36","span":{"begin":3854,"end":3858},"obj":"Chemical"},{"id":"T38","span":{"begin":4269,"end":4282},"obj":"Chemical"},{"id":"T39","span":{"begin":4276,"end":4282},"obj":"Chemical"},{"id":"T41","span":{"begin":4283,"end":4292},"obj":"Chemical"},{"id":"T43","span":{"begin":4294,"end":4297},"obj":"Chemical"},{"id":"T44","span":{"begin":4578,"end":4581},"obj":"Chemical"},{"id":"T45","span":{"begin":4789,"end":4792},"obj":"Chemical"},{"id":"T46","span":{"begin":4966,"end":4969},"obj":"Chemical"},{"id":"T47","span":{"begin":5042,"end":5049},"obj":"Chemical"},{"id":"T48","span":{"begin":5118,"end":5121},"obj":"Chemical"},{"id":"T49","span":{"begin":5202,"end":5205},"obj":"Chemical"},{"id":"T50","span":{"begin":5222,"end":5224},"obj":"Chemical"},{"id":"T51","span":{"begin":5315,"end":5318},"obj":"Chemical"},{"id":"T52","span":{"begin":5355,"end":5357},"obj":"Chemical"},{"id":"T53","span":{"begin":5375,"end":5378},"obj":"Chemical"},{"id":"T54","span":{"begin":5604,"end":5607},"obj":"Chemical"},{"id":"T55","span":{"begin":5672,"end":5679},"obj":"Chemical"}],"attributes":[{"id":"A19","pred":"chebi_id","subj":"T19","obj":"http://purl.obolibrary.org/obo/CHEBI_16991"},{"id":"A20","pred":"chebi_id","subj":"T20","obj":"http://purl.obolibrary.org/obo/CHEBI_33697"},{"id":"A21","pred":"chebi_id","subj":"T21","obj":"http://purl.obolibrary.org/obo/CHEBI_16991"},{"id":"A22","pred":"chebi_id","subj":"T22","obj":"http://purl.obolibrary.org/obo/CHEBI_16991"},{"id":"A23","pred":"chebi_id","subj":"T23","obj":"http://purl.obolibrary.org/obo/CHEBI_33839"},{"id":"A24","pred":"chebi_id","subj":"T24","obj":"http://purl.obolibrary.org/obo/CHEBI_16991"},{"id":"A25","pred":"chebi_id","subj":"T25","obj":"http://purl.obolibrary.org/obo/CHEBI_28694"},{"id":"A26","pred":"chebi_id","subj":"T26","obj":"http://purl.obolibrary.org/obo/CHEBI_8066"},{"id":"A27","pred":"chebi_id","subj":"T27","obj":"http://purl.obolibrary.org/obo/CHEBI_8066"},{"id":"A28","pred":"chebi_id","subj":"T28","obj":"http://purl.obolibrary.org/obo/CHEBI_16991"},{"id":"A29","pred":"chebi_id","subj":"T29","obj":"http://purl.obolibrary.org/obo/CHEBI_33697"},{"id":"A30","pred":"chebi_id","subj":"T30","obj":"http://purl.obolibrary.org/obo/CHEBI_33839"},{"id":"A31","pred":"chebi_id","subj":"T31","obj":"http://purl.obolibrary.org/obo/CHEBI_33521"},{"id":"A32","pred":"chebi_id","subj":"T32","obj":"http://purl.obolibrary.org/obo/CHEBI_31498"},{"id":"A33","pred":"chebi_id","subj":"T32","obj":"http://purl.obolibrary.org/obo/CHEBI_48137"},{"id":"A34","pred":"chebi_id","subj":"T32","obj":"http://purl.obolibrary.org/obo/CHEBI_61466"},{"id":"A35","pred":"chebi_id","subj":"T32","obj":"http://purl.obolibrary.org/obo/CHEBI_61468"},{"id":"A36","pred":"chebi_id","subj":"T36","obj":"http://purl.obolibrary.org/obo/CHEBI_31498"},{"id":"A37","pred":"chebi_id","subj":"T36","obj":"http://purl.obolibrary.org/obo/CHEBI_61468"},{"id":"A38","pred":"chebi_id","subj":"T38","obj":"http://purl.obolibrary.org/obo/CHEBI_33642"},{"id":"A39","pred":"chebi_id","subj":"T39","obj":"http://purl.obolibrary.org/obo/CHEBI_32878"},{"id":"A40","pred":"chebi_id","subj":"T39","obj":"http://purl.obolibrary.org/obo/CHEBI_33641"},{"id":"A41","pred":"chebi_id","subj":"T41","obj":"http://purl.obolibrary.org/obo/CHEBI_53310"},{"id":"A42","pred":"chebi_id","subj":"T41","obj":"http://purl.obolibrary.org/obo/CHEBI_60804"},{"id":"A43","pred":"chebi_id","subj":"T43","obj":"http://purl.obolibrary.org/obo/CHEBI_53310"},{"id":"A44","pred":"chebi_id","subj":"T44","obj":"http://purl.obolibrary.org/obo/CHEBI_53310"},{"id":"A45","pred":"chebi_id","subj":"T45","obj":"http://purl.obolibrary.org/obo/CHEBI_53310"},{"id":"A46","pred":"chebi_id","subj":"T46","obj":"http://purl.obolibrary.org/obo/CHEBI_53310"},{"id":"A47","pred":"chebi_id","subj":"T47","obj":"http://purl.obolibrary.org/obo/CHEBI_46787"},{"id":"A48","pred":"chebi_id","subj":"T48","obj":"http://purl.obolibrary.org/obo/CHEBI_53310"},{"id":"A49","pred":"chebi_id","subj":"T49","obj":"http://purl.obolibrary.org/obo/CHEBI_53310"},{"id":"A50","pred":"chebi_id","subj":"T50","obj":"http://purl.obolibrary.org/obo/CHEBI_29287"},{"id":"A51","pred":"chebi_id","subj":"T51","obj":"http://purl.obolibrary.org/obo/CHEBI_53310"},{"id":"A52","pred":"chebi_id","subj":"T52","obj":"http://purl.obolibrary.org/obo/CHEBI_9516"},{"id":"A53","pred":"chebi_id","subj":"T53","obj":"http://purl.obolibrary.org/obo/CHEBI_53310"},{"id":"A54","pred":"chebi_id","subj":"T54","obj":"http://purl.obolibrary.org/obo/CHEBI_53310"},{"id":"A55","pred":"chebi_id","subj":"T55","obj":"http://purl.obolibrary.org/obo/CHEBI_60004"}],"text":"1.1. State-of-the-Art\nIn the past, several chip-based DNA and RNA amplification devices are reported. It goes beyond the scope of this paper to discuss the state-of-the-art of DNA amplification chips in-depth. There are several good review papers written on this topic [12,13,14,15,16,17,18,19,20]. Readers are referred to these for a comprehensive overview of the field. In this paper, the state-of-the-art is divided into several discussion points, i.e., the heating method, the temperature control method, and the substrate material and fabrication technique. These points will be discussed separately.\nWith respect to heat supply, different methods have been employed. Almassian et al. give a comprehensive overview of different possible heating methods in their review paper [12]. Not all of the mentioned methods are easy to implement in low-cost and portable lab-on-a-chip devices due to their bulkiness or implementation costs. Examples of these rather difficult methods are using heating via induction, infrared, or microwave radiation. Others are not useful due ot their challenging temperature control, like with heating up the system using exothermic reactions. Within the field of DNA amplification, different mechanisms of amplification exist. Some are based on thermo cycling processes, e.g., PCR, whereas others are isothermal. The use of an isothermal amplification technique puts less requirements on the heaters. Isothermal processes are either truly isothermal or consisting of three different temperatures, as they have a thermal denaturation step before and a termination step after the elongation step. The switching between these temperature steps does not have to be as fast as with thermal cycling steps in, for example, PCR amplification reactions. The use of less temperature variations makes it easier to maintain the set temperature as there is less heating an cooling involved. Furthermore, it eliminates the use of a continuous flow approach in systems with low thermal conductivities, e.g., polymers. Therefore, it is easier to implement within lab-on-a-chip devices [17,21]. Isothermal DNA amplification reactions can already be performed by putting the chip on a commercially available hotplate [22,23] or Peltier elements [24,25]. However, these heating systems are bulky and power-consuming. Therefore, they are not useful for portable equipment or operation at remote locations. Miniaturizing heaters lowers the bulkiness and power consumption. Miniaturized heaters can be integrated as integrated resistive heaters, e.g., as deposited thin-film metal [26,27,28,29] or as laminated Cu foil [30], or as micro-Peltier elements [31,32]. These miniaturized heaters can be implemented directly onto the microfluidic chip [28] or on a different substrate and leter incorporated onto the microfluidic chip [33,34,35,36]. The geometry of such a heater contributes significantly to the uniformity of the heat distribution within the chip [26,37]. One method to accurately control the temperature is the use of a proportional-integral-derivative (PID) controlled thermostat. These PID controllers are coupled to the electrical heaters and use a thermocouple as feedback-loop to the controller [22,23,24,25].\nThere are various materials that can be used to fabricate lab-on-a-chip devices for DNA or RNA amplification. In the past 15 years, more than ten polymers, ceramic materials, and metals have successfully been used to fabricate such devices [15]. The major property playing a role here is the biocompatibility of the material. The surface of the microfluidic structure should not inhibit the amplification reaction. This biocompatibility can be an intrinsic property of the material or the surface can be modified or coated to achieve this [12,13,14,15,16,17,18,19,20]. One often used material is polydimethylsiloxane (PDMS) [22,23,31,32,34,35,38], which can be processed using soft lithography [39]. However, this is a fabrication technology used in academia and is not suitable for upscaling to mass production [40]. Fabrication methods suitable for mass production are thermoforming/embossing or injection molding [41]. One of the materials which is biocompatible and suitable for both industrial scale fabrication technologies is cyclic olefin copolymer (COC) [42], which is one of the materials used in the past as well [28,36,43,44,45]. Guckenberger et al. estimates the costs of injection molding of only 50 simple microfluidic devices on $47, but this becomes cheaper when the mass production stage is reached [41]. Another benefit of COC is the possibility to shape it using micromilling. This technique is a rapid prototyping technology and therefore very useful within proof-of-concept projects [41].\nIntegrating resistive metal tracks onto a COC substrate have also been done in the past. Some papers describe the use of a surface modification step done before metal deposition in order to enhance adhesion between the COC and the metal layer, like a pretreatment with plasma [46] or an organic solvent [47]. Other papers describe the direct deposition of metal onto the COC surface [28,48]. Chung et al. specifically, fabricated an amplification chip in COC with integrated Au heaters [28]. However, their system required heating from both sides as the used grade of COC has a glass transition temperature (Tg) of 130 °C. This COC could not withstand the required heater temperatures to have enough heat flux into the system. They had to heat up the heater to temperatures above 130 °C, which caused cracking of the heater tracks due to deformation of the COC. With their double-sided heating they ensured that the reaction mixture had the desired PCR temperatures. However, double-sided heating doubles the amount of metal required, increases the amount of fabrication steps, and therefore increases the price per chip."}
LitCovid-PD-GO-BP
{"project":"LitCovid-PD-GO-BP","denotations":[{"id":"T3","span":{"begin":176,"end":193},"obj":"http://purl.obolibrary.org/obo/GO_0006277"},{"id":"T4","span":{"begin":1194,"end":1211},"obj":"http://purl.obolibrary.org/obo/GO_0006277"},{"id":"T5","span":{"begin":2120,"end":2137},"obj":"http://purl.obolibrary.org/obo/GO_0006277"}],"text":"1.1. State-of-the-Art\nIn the past, several chip-based DNA and RNA amplification devices are reported. It goes beyond the scope of this paper to discuss the state-of-the-art of DNA amplification chips in-depth. There are several good review papers written on this topic [12,13,14,15,16,17,18,19,20]. Readers are referred to these for a comprehensive overview of the field. In this paper, the state-of-the-art is divided into several discussion points, i.e., the heating method, the temperature control method, and the substrate material and fabrication technique. These points will be discussed separately.\nWith respect to heat supply, different methods have been employed. Almassian et al. give a comprehensive overview of different possible heating methods in their review paper [12]. Not all of the mentioned methods are easy to implement in low-cost and portable lab-on-a-chip devices due to their bulkiness or implementation costs. Examples of these rather difficult methods are using heating via induction, infrared, or microwave radiation. Others are not useful due ot their challenging temperature control, like with heating up the system using exothermic reactions. Within the field of DNA amplification, different mechanisms of amplification exist. Some are based on thermo cycling processes, e.g., PCR, whereas others are isothermal. The use of an isothermal amplification technique puts less requirements on the heaters. Isothermal processes are either truly isothermal or consisting of three different temperatures, as they have a thermal denaturation step before and a termination step after the elongation step. The switching between these temperature steps does not have to be as fast as with thermal cycling steps in, for example, PCR amplification reactions. The use of less temperature variations makes it easier to maintain the set temperature as there is less heating an cooling involved. Furthermore, it eliminates the use of a continuous flow approach in systems with low thermal conductivities, e.g., polymers. Therefore, it is easier to implement within lab-on-a-chip devices [17,21]. Isothermal DNA amplification reactions can already be performed by putting the chip on a commercially available hotplate [22,23] or Peltier elements [24,25]. However, these heating systems are bulky and power-consuming. Therefore, they are not useful for portable equipment or operation at remote locations. Miniaturizing heaters lowers the bulkiness and power consumption. Miniaturized heaters can be integrated as integrated resistive heaters, e.g., as deposited thin-film metal [26,27,28,29] or as laminated Cu foil [30], or as micro-Peltier elements [31,32]. These miniaturized heaters can be implemented directly onto the microfluidic chip [28] or on a different substrate and leter incorporated onto the microfluidic chip [33,34,35,36]. The geometry of such a heater contributes significantly to the uniformity of the heat distribution within the chip [26,37]. One method to accurately control the temperature is the use of a proportional-integral-derivative (PID) controlled thermostat. These PID controllers are coupled to the electrical heaters and use a thermocouple as feedback-loop to the controller [22,23,24,25].\nThere are various materials that can be used to fabricate lab-on-a-chip devices for DNA or RNA amplification. In the past 15 years, more than ten polymers, ceramic materials, and metals have successfully been used to fabricate such devices [15]. The major property playing a role here is the biocompatibility of the material. The surface of the microfluidic structure should not inhibit the amplification reaction. This biocompatibility can be an intrinsic property of the material or the surface can be modified or coated to achieve this [12,13,14,15,16,17,18,19,20]. One often used material is polydimethylsiloxane (PDMS) [22,23,31,32,34,35,38], which can be processed using soft lithography [39]. However, this is a fabrication technology used in academia and is not suitable for upscaling to mass production [40]. Fabrication methods suitable for mass production are thermoforming/embossing or injection molding [41]. One of the materials which is biocompatible and suitable for both industrial scale fabrication technologies is cyclic olefin copolymer (COC) [42], which is one of the materials used in the past as well [28,36,43,44,45]. Guckenberger et al. estimates the costs of injection molding of only 50 simple microfluidic devices on $47, but this becomes cheaper when the mass production stage is reached [41]. Another benefit of COC is the possibility to shape it using micromilling. This technique is a rapid prototyping technology and therefore very useful within proof-of-concept projects [41].\nIntegrating resistive metal tracks onto a COC substrate have also been done in the past. Some papers describe the use of a surface modification step done before metal deposition in order to enhance adhesion between the COC and the metal layer, like a pretreatment with plasma [46] or an organic solvent [47]. Other papers describe the direct deposition of metal onto the COC surface [28,48]. Chung et al. specifically, fabricated an amplification chip in COC with integrated Au heaters [28]. However, their system required heating from both sides as the used grade of COC has a glass transition temperature (Tg) of 130 °C. This COC could not withstand the required heater temperatures to have enough heat flux into the system. They had to heat up the heater to temperatures above 130 °C, which caused cracking of the heater tracks due to deformation of the COC. With their double-sided heating they ensured that the reaction mixture had the desired PCR temperatures. However, double-sided heating doubles the amount of metal required, increases the amount of fabrication steps, and therefore increases the price per chip."}
LitCovid-sentences
{"project":"LitCovid-sentences","denotations":[{"id":"T28","span":{"begin":0,"end":4},"obj":"Sentence"},{"id":"T29","span":{"begin":5,"end":21},"obj":"Sentence"},{"id":"T30","span":{"begin":22,"end":101},"obj":"Sentence"},{"id":"T31","span":{"begin":102,"end":209},"obj":"Sentence"},{"id":"T32","span":{"begin":210,"end":298},"obj":"Sentence"},{"id":"T33","span":{"begin":299,"end":371},"obj":"Sentence"},{"id":"T34","span":{"begin":372,"end":562},"obj":"Sentence"},{"id":"T35","span":{"begin":563,"end":605},"obj":"Sentence"},{"id":"T36","span":{"begin":606,"end":672},"obj":"Sentence"},{"id":"T37","span":{"begin":673,"end":785},"obj":"Sentence"},{"id":"T38","span":{"begin":786,"end":935},"obj":"Sentence"},{"id":"T39","span":{"begin":936,"end":1045},"obj":"Sentence"},{"id":"T40","span":{"begin":1046,"end":1173},"obj":"Sentence"},{"id":"T41","span":{"begin":1174,"end":1257},"obj":"Sentence"},{"id":"T42","span":{"begin":1258,"end":1343},"obj":"Sentence"},{"id":"T43","span":{"begin":1344,"end":1431},"obj":"Sentence"},{"id":"T44","span":{"begin":1432,"end":1625},"obj":"Sentence"},{"id":"T45","span":{"begin":1626,"end":1775},"obj":"Sentence"},{"id":"T46","span":{"begin":1776,"end":1908},"obj":"Sentence"},{"id":"T47","span":{"begin":1909,"end":2033},"obj":"Sentence"},{"id":"T48","span":{"begin":2034,"end":2108},"obj":"Sentence"},{"id":"T49","span":{"begin":2109,"end":2266},"obj":"Sentence"},{"id":"T50","span":{"begin":2267,"end":2328},"obj":"Sentence"},{"id":"T51","span":{"begin":2329,"end":2416},"obj":"Sentence"},{"id":"T52","span":{"begin":2417,"end":2482},"obj":"Sentence"},{"id":"T53","span":{"begin":2483,"end":2671},"obj":"Sentence"},{"id":"T54","span":{"begin":2672,"end":2851},"obj":"Sentence"},{"id":"T55","span":{"begin":2852,"end":2975},"obj":"Sentence"},{"id":"T56","span":{"begin":2976,"end":3102},"obj":"Sentence"},{"id":"T57","span":{"begin":3103,"end":3235},"obj":"Sentence"},{"id":"T58","span":{"begin":3236,"end":3345},"obj":"Sentence"},{"id":"T59","span":{"begin":3346,"end":3481},"obj":"Sentence"},{"id":"T60","span":{"begin":3482,"end":3561},"obj":"Sentence"},{"id":"T61","span":{"begin":3562,"end":3650},"obj":"Sentence"},{"id":"T62","span":{"begin":3651,"end":3804},"obj":"Sentence"},{"id":"T63","span":{"begin":3805,"end":3935},"obj":"Sentence"},{"id":"T64","span":{"begin":3936,"end":4053},"obj":"Sentence"},{"id":"T65","span":{"begin":4054,"end":4157},"obj":"Sentence"},{"id":"T66","span":{"begin":4158,"end":4377},"obj":"Sentence"},{"id":"T67","span":{"begin":4378,"end":4558},"obj":"Sentence"},{"id":"T68","span":{"begin":4559,"end":4632},"obj":"Sentence"},{"id":"T69","span":{"begin":4633,"end":4746},"obj":"Sentence"},{"id":"T70","span":{"begin":4747,"end":4835},"obj":"Sentence"},{"id":"T71","span":{"begin":4836,"end":5055},"obj":"Sentence"},{"id":"T72","span":{"begin":5056,"end":5138},"obj":"Sentence"},{"id":"T73","span":{"begin":5139,"end":5238},"obj":"Sentence"},{"id":"T74","span":{"begin":5239,"end":5369},"obj":"Sentence"},{"id":"T75","span":{"begin":5370,"end":5473},"obj":"Sentence"},{"id":"T76","span":{"begin":5474,"end":5608},"obj":"Sentence"},{"id":"T77","span":{"begin":5609,"end":5713},"obj":"Sentence"},{"id":"T78","span":{"begin":5714,"end":5868},"obj":"Sentence"}],"namespaces":[{"prefix":"_base","uri":"http://pubannotation.org/ontology/tao.owl#"}],"text":"1.1. State-of-the-Art\nIn the past, several chip-based DNA and RNA amplification devices are reported. It goes beyond the scope of this paper to discuss the state-of-the-art of DNA amplification chips in-depth. There are several good review papers written on this topic [12,13,14,15,16,17,18,19,20]. Readers are referred to these for a comprehensive overview of the field. In this paper, the state-of-the-art is divided into several discussion points, i.e., the heating method, the temperature control method, and the substrate material and fabrication technique. These points will be discussed separately.\nWith respect to heat supply, different methods have been employed. Almassian et al. give a comprehensive overview of different possible heating methods in their review paper [12]. Not all of the mentioned methods are easy to implement in low-cost and portable lab-on-a-chip devices due to their bulkiness or implementation costs. Examples of these rather difficult methods are using heating via induction, infrared, or microwave radiation. Others are not useful due ot their challenging temperature control, like with heating up the system using exothermic reactions. Within the field of DNA amplification, different mechanisms of amplification exist. Some are based on thermo cycling processes, e.g., PCR, whereas others are isothermal. The use of an isothermal amplification technique puts less requirements on the heaters. Isothermal processes are either truly isothermal or consisting of three different temperatures, as they have a thermal denaturation step before and a termination step after the elongation step. The switching between these temperature steps does not have to be as fast as with thermal cycling steps in, for example, PCR amplification reactions. The use of less temperature variations makes it easier to maintain the set temperature as there is less heating an cooling involved. Furthermore, it eliminates the use of a continuous flow approach in systems with low thermal conductivities, e.g., polymers. Therefore, it is easier to implement within lab-on-a-chip devices [17,21]. Isothermal DNA amplification reactions can already be performed by putting the chip on a commercially available hotplate [22,23] or Peltier elements [24,25]. However, these heating systems are bulky and power-consuming. Therefore, they are not useful for portable equipment or operation at remote locations. Miniaturizing heaters lowers the bulkiness and power consumption. Miniaturized heaters can be integrated as integrated resistive heaters, e.g., as deposited thin-film metal [26,27,28,29] or as laminated Cu foil [30], or as micro-Peltier elements [31,32]. These miniaturized heaters can be implemented directly onto the microfluidic chip [28] or on a different substrate and leter incorporated onto the microfluidic chip [33,34,35,36]. The geometry of such a heater contributes significantly to the uniformity of the heat distribution within the chip [26,37]. One method to accurately control the temperature is the use of a proportional-integral-derivative (PID) controlled thermostat. These PID controllers are coupled to the electrical heaters and use a thermocouple as feedback-loop to the controller [22,23,24,25].\nThere are various materials that can be used to fabricate lab-on-a-chip devices for DNA or RNA amplification. In the past 15 years, more than ten polymers, ceramic materials, and metals have successfully been used to fabricate such devices [15]. The major property playing a role here is the biocompatibility of the material. The surface of the microfluidic structure should not inhibit the amplification reaction. This biocompatibility can be an intrinsic property of the material or the surface can be modified or coated to achieve this [12,13,14,15,16,17,18,19,20]. One often used material is polydimethylsiloxane (PDMS) [22,23,31,32,34,35,38], which can be processed using soft lithography [39]. However, this is a fabrication technology used in academia and is not suitable for upscaling to mass production [40]. Fabrication methods suitable for mass production are thermoforming/embossing or injection molding [41]. One of the materials which is biocompatible and suitable for both industrial scale fabrication technologies is cyclic olefin copolymer (COC) [42], which is one of the materials used in the past as well [28,36,43,44,45]. Guckenberger et al. estimates the costs of injection molding of only 50 simple microfluidic devices on $47, but this becomes cheaper when the mass production stage is reached [41]. Another benefit of COC is the possibility to shape it using micromilling. This technique is a rapid prototyping technology and therefore very useful within proof-of-concept projects [41].\nIntegrating resistive metal tracks onto a COC substrate have also been done in the past. Some papers describe the use of a surface modification step done before metal deposition in order to enhance adhesion between the COC and the metal layer, like a pretreatment with plasma [46] or an organic solvent [47]. Other papers describe the direct deposition of metal onto the COC surface [28,48]. Chung et al. specifically, fabricated an amplification chip in COC with integrated Au heaters [28]. However, their system required heating from both sides as the used grade of COC has a glass transition temperature (Tg) of 130 °C. This COC could not withstand the required heater temperatures to have enough heat flux into the system. They had to heat up the heater to temperatures above 130 °C, which caused cracking of the heater tracks due to deformation of the COC. With their double-sided heating they ensured that the reaction mixture had the desired PCR temperatures. However, double-sided heating doubles the amount of metal required, increases the amount of fabrication steps, and therefore increases the price per chip."}
2_test
{"project":"2_test","denotations":[{"id":"32106462-24030680-69893274","span":{"begin":270,"end":272},"obj":"24030680"},{"id":"32106462-19327449-69893275","span":{"begin":279,"end":281},"obj":"19327449"},{"id":"32106462-21387067-69893276","span":{"begin":285,"end":287},"obj":"21387067"},{"id":"32106462-17553097-69893277","span":{"begin":288,"end":290},"obj":"17553097"},{"id":"32106462-22704369-69893278","span":{"begin":294,"end":296},"obj":"22704369"},{"id":"32106462-24030680-69893279","span":{"begin":781,"end":783},"obj":"24030680"},{"id":"32106462-21387067-69893280","span":{"begin":2101,"end":2103},"obj":"21387067"},{"id":"32106462-22592150-69893281","span":{"begin":2104,"end":2106},"obj":"22592150"},{"id":"32106462-21479543-69893282","span":{"begin":2231,"end":2233},"obj":"21479543"},{"id":"32106462-23610705-69893283","span":{"begin":2234,"end":2236},"obj":"23610705"},{"id":"32106462-21267083-69893284","span":{"begin":2259,"end":2261},"obj":"21267083"},{"id":"32106462-22038328-69893285","span":{"begin":2262,"end":2264},"obj":"22038328"},{"id":"32106462-15269812-69893286","span":{"begin":2838,"end":2840},"obj":"15269812"},{"id":"32106462-18975094-69893287","span":{"begin":2841,"end":2843},"obj":"18975094"},{"id":"32106462-21479543-69893288","span":{"begin":3222,"end":3224},"obj":"21479543"},{"id":"32106462-23610705-69893289","span":{"begin":3225,"end":3227},"obj":"23610705"},{"id":"32106462-21267083-69893290","span":{"begin":3228,"end":3230},"obj":"21267083"},{"id":"32106462-22038328-69893291","span":{"begin":3231,"end":3233},"obj":"22038328"},{"id":"32106462-19327449-69893292","span":{"begin":3477,"end":3479},"obj":"19327449"},{"id":"32106462-24030680-69893293","span":{"begin":3776,"end":3778},"obj":"24030680"},{"id":"32106462-19327449-69893294","span":{"begin":3785,"end":3787},"obj":"19327449"},{"id":"32106462-21387067-69893295","span":{"begin":3791,"end":3793},"obj":"21387067"},{"id":"32106462-17553097-69893296","span":{"begin":3794,"end":3796},"obj":"17553097"},{"id":"32106462-22704369-69893297","span":{"begin":3800,"end":3802},"obj":"22704369"},{"id":"32106462-21479543-69893298","span":{"begin":3861,"end":3863},"obj":"21479543"},{"id":"32106462-23610705-69893299","span":{"begin":3864,"end":3866},"obj":"23610705"},{"id":"32106462-18975094-69893300","span":{"begin":3873,"end":3875},"obj":"18975094"},{"id":"32106462-20218572-69893301","span":{"begin":3879,"end":3881},"obj":"20218572"},{"id":"32106462-28281349-69893302","span":{"begin":4049,"end":4051},"obj":"28281349"},{"id":"32106462-25906246-69893303","span":{"begin":4153,"end":4155},"obj":"25906246"},{"id":"32106462-20066496-69893304","span":{"begin":4367,"end":4369},"obj":"20066496"},{"id":"32106462-31277382-69893305","span":{"begin":4373,"end":4375},"obj":"31277382"},{"id":"32106462-25906246-69893306","span":{"begin":4554,"end":4556},"obj":"25906246"},{"id":"32106462-25906246-69893307","span":{"begin":4742,"end":4744},"obj":"25906246"},{"id":"32106462-24957952-69893308","span":{"begin":5134,"end":5136},"obj":"24957952"}],"text":"1.1. State-of-the-Art\nIn the past, several chip-based DNA and RNA amplification devices are reported. It goes beyond the scope of this paper to discuss the state-of-the-art of DNA amplification chips in-depth. There are several good review papers written on this topic [12,13,14,15,16,17,18,19,20]. Readers are referred to these for a comprehensive overview of the field. In this paper, the state-of-the-art is divided into several discussion points, i.e., the heating method, the temperature control method, and the substrate material and fabrication technique. These points will be discussed separately.\nWith respect to heat supply, different methods have been employed. Almassian et al. give a comprehensive overview of different possible heating methods in their review paper [12]. Not all of the mentioned methods are easy to implement in low-cost and portable lab-on-a-chip devices due to their bulkiness or implementation costs. Examples of these rather difficult methods are using heating via induction, infrared, or microwave radiation. Others are not useful due ot their challenging temperature control, like with heating up the system using exothermic reactions. Within the field of DNA amplification, different mechanisms of amplification exist. Some are based on thermo cycling processes, e.g., PCR, whereas others are isothermal. The use of an isothermal amplification technique puts less requirements on the heaters. Isothermal processes are either truly isothermal or consisting of three different temperatures, as they have a thermal denaturation step before and a termination step after the elongation step. The switching between these temperature steps does not have to be as fast as with thermal cycling steps in, for example, PCR amplification reactions. The use of less temperature variations makes it easier to maintain the set temperature as there is less heating an cooling involved. Furthermore, it eliminates the use of a continuous flow approach in systems with low thermal conductivities, e.g., polymers. Therefore, it is easier to implement within lab-on-a-chip devices [17,21]. Isothermal DNA amplification reactions can already be performed by putting the chip on a commercially available hotplate [22,23] or Peltier elements [24,25]. However, these heating systems are bulky and power-consuming. Therefore, they are not useful for portable equipment or operation at remote locations. Miniaturizing heaters lowers the bulkiness and power consumption. Miniaturized heaters can be integrated as integrated resistive heaters, e.g., as deposited thin-film metal [26,27,28,29] or as laminated Cu foil [30], or as micro-Peltier elements [31,32]. These miniaturized heaters can be implemented directly onto the microfluidic chip [28] or on a different substrate and leter incorporated onto the microfluidic chip [33,34,35,36]. The geometry of such a heater contributes significantly to the uniformity of the heat distribution within the chip [26,37]. One method to accurately control the temperature is the use of a proportional-integral-derivative (PID) controlled thermostat. These PID controllers are coupled to the electrical heaters and use a thermocouple as feedback-loop to the controller [22,23,24,25].\nThere are various materials that can be used to fabricate lab-on-a-chip devices for DNA or RNA amplification. In the past 15 years, more than ten polymers, ceramic materials, and metals have successfully been used to fabricate such devices [15]. The major property playing a role here is the biocompatibility of the material. The surface of the microfluidic structure should not inhibit the amplification reaction. This biocompatibility can be an intrinsic property of the material or the surface can be modified or coated to achieve this [12,13,14,15,16,17,18,19,20]. One often used material is polydimethylsiloxane (PDMS) [22,23,31,32,34,35,38], which can be processed using soft lithography [39]. However, this is a fabrication technology used in academia and is not suitable for upscaling to mass production [40]. Fabrication methods suitable for mass production are thermoforming/embossing or injection molding [41]. One of the materials which is biocompatible and suitable for both industrial scale fabrication technologies is cyclic olefin copolymer (COC) [42], which is one of the materials used in the past as well [28,36,43,44,45]. Guckenberger et al. estimates the costs of injection molding of only 50 simple microfluidic devices on $47, but this becomes cheaper when the mass production stage is reached [41]. Another benefit of COC is the possibility to shape it using micromilling. This technique is a rapid prototyping technology and therefore very useful within proof-of-concept projects [41].\nIntegrating resistive metal tracks onto a COC substrate have also been done in the past. Some papers describe the use of a surface modification step done before metal deposition in order to enhance adhesion between the COC and the metal layer, like a pretreatment with plasma [46] or an organic solvent [47]. Other papers describe the direct deposition of metal onto the COC surface [28,48]. Chung et al. specifically, fabricated an amplification chip in COC with integrated Au heaters [28]. However, their system required heating from both sides as the used grade of COC has a glass transition temperature (Tg) of 130 °C. This COC could not withstand the required heater temperatures to have enough heat flux into the system. They had to heat up the heater to temperatures above 130 °C, which caused cracking of the heater tracks due to deformation of the COC. With their double-sided heating they ensured that the reaction mixture had the desired PCR temperatures. However, double-sided heating doubles the amount of metal required, increases the amount of fabrication steps, and therefore increases the price per chip."}