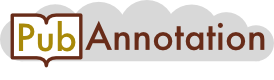
PMC:7031805 / 11897-18872
Annnotations
TEST0
{"project":"TEST0","denotations":[{"id":"31965829-94-100-28","span":{"begin":111,"end":113},"obj":"[\"30661434\"]"},{"id":"31965829-234-240-29","span":{"begin":283,"end":285},"obj":"[\"30347190\"]"},{"id":"31965829-234-240-30","span":{"begin":1160,"end":1162},"obj":"[\"30347190\"]"},{"id":"31965829-166-172-31","span":{"begin":1674,"end":1676},"obj":"[\"31371124\"]"},{"id":"31965829-236-242-32","span":{"begin":2402,"end":2404},"obj":"[\"28564577\"]"},{"id":"31965829-221-227-33","span":{"begin":2623,"end":2625},"obj":"[\"29589874\"]"},{"id":"31965829-224-230-34","span":{"begin":2626,"end":2628},"obj":"[\"30755487\"]"},{"id":"31965829-232-238-35","span":{"begin":4358,"end":4360},"obj":"[\"27839871\", \"28095420\", \"31504254\", \"31429998\", \"28377535\"]"},{"id":"31965829-229-235-36","span":{"begin":4613,"end":4615},"obj":"[\"28095420\"]"},{"id":"31965829-232-238-37","span":{"begin":4616,"end":4618},"obj":"[\"24002024\"]"},{"id":"31965829-235-241-38","span":{"begin":4619,"end":4621},"obj":"[\"29915537\"]"},{"id":"31965829-156-162-39","span":{"begin":4978,"end":4980},"obj":"[\"25990557\"]"},{"id":"31965829-192-198-40","span":{"begin":6070,"end":6072},"obj":"[\"29589874\"]"},{"id":"31965829-236-241-41","span":{"begin":6632,"end":6633},"obj":"[\"31327507\"]"}],"text":"Genetic Advances\nAn important consequence of the many genetic advances that are transforming clinical neurology39 is their ability to suggest new animal models to investigate the underlying disease mechanisms, including compensatory mechanisms that can contribute to a seizure focus.40 Such models are relevant to genetic human epilepsies and serve as an important complement to the acquired models of focal epilepsy (eg, pharmacologically induced seizure models) that have become the mainstay for development of in vivo models of chronic recurring seizures. Animal models of single-gene defects offer an opportunity to evaluate windows for therapeutic intervention in patients who have these specific variants, with the possibility that some therapies will be more broadly applicable to multiple epilepsies. In addition, such models offer a new opportunity to study common mechanisms that underlie maladaptive plasticity and can lead to generation of a seizure focus. Novel gene expression programs may be triggered by genetic deficiencies that engage similar mechanisms, and understanding these might allow better understanding of antiepileptic drug utility.40 In this respect, the intersection of gene expression data sets may inform key pathways that establish seizure foci regardless of the initial genetic defect driving seizures. In addition, genetic animal models can facilitate the evaluation and validation of strategies such as antisense oligonucleotides, gene replacement, and gene augmentation. The success of new genetic treatments of spinal muscular atrophy with intravenously delivered gene replacement via adeno-associated viral vector in very young infants41 has created hope for many patients that these therapies can correct other neurological conditions, stimulating work on this problem in academia and, importantly, in industry. Thus, there are actionable opportunities for genetic therapies for epilepsy on the horizon.\nIn addition to the value of new models suggested by genetic analysis, there are several opportunities to exploit advances in diagnostic and therapeutic genetic approaches. There are now multiple examples of strategies one could use to develop gene therapy employing viral vectors to treat focal and generalized epilepsies in animal models in which a missense variant or truncating mutation has modified the function of a target gene or reduced the gene dose.42 Other innovative uses of gene therapy include introduction of potassium channels that could reduce excitability, as well as engineering cells to release neuroactive molecules that can counteract excessive excitability.43,44 As more animal models are developed for different genes, there will be opportunities to test fundamental approaches that supplement underexpressed alleles or proteins with reduced function, as well as editing gene approaches to correct identified defects. These strategies will require demonstration of utility in animals with measurable defects, and the results will speak to the important question in epilepsy around whether symptoms are driven by the genetic defect, are a feature of maladaptive compensation, or reflect some combination of both. That is, there is a need for proof-of-concept data for oligonucleotide and antisense therapies for application in the treatment of genetically defined monogenic epilepsies, as well as data on effectiveness of the timing of treatment in the context of the development of a seizure focus. Advances are needed in genetic therapy using virus delivery vectors that are already approved for other payloads and access both brain and spinal cord following intrathecal administration. The rare genetic epilepsies might provide a test case for intervention, which can be evaluated in iPSC-based models in vitro, organoids derived from iPSC cells, and animal models now.\nOne opportunity that the accessibility of multiple new genetic models of human variants associated with epilepsy offers is evaluation of repurposed drugs. This requires a comprehensive functional evaluation of the effects of rare variants in vitro, which for ion channels is accessible. Functional evaluation of drug sensitivity of variant proteins will inform potential use of therapeutics, as will knowledge of the nature of the net functional effects as either gain of function or loss of function, or indeterminant.45-49 Genetic models—from cellular models to zebrafish and mouse models—harboring variants can then be screened for actions of Food and Drug Administration (FDA)-approved medications for efficacy in reducing electroencephalogram abnormalities and seizures46,50,51 as a step toward using pharmacological treatments. If the models capture patient-relevant features of epileptogenesis, early treatment within a vulnerable window might have long-lasting consequences.\nIn addition to these pharmacological approaches, bioinformatics coupled with large-scale data sets have driven the development of computational resources52,53 that can suggest candidate drugs in the FDA library from patterns of changes in gene expression. Moreover, evaluation of multiple drugs in multiple models might identify candidate drugs as add-on therapies that could be used more broadly than for just for rare genetic conditions. Indeed, a large number of epilepsy models have been or are being made from various genes identified in patients with rare epilepsies (eg, sodium channels, potassium channels, postsynaptic ligand-gated ion channels, synaptic proteins), which will provide patient-relevant models in which to assess new pharmacological strategies. These same models can be used to understand developmental compensation, transformation of the foci with time, and pharmacological sensitivity. It seems likely that some compensatory mechanisms will be shared across these different models and may inform treatment of refractory epilepsy. In addition to rodent models, use of companion models and organisms (fly, zebrafish, mice, iPSC-derived neuronal cultures, and organoids) could provide faster and more efficient drug screening43 as well as evaluation of compensatory mechanisms.\nThe advances in genetic analysis could also expand our understanding of acquired epilepsies and yield insight into whether persons with genetic predispositions may be at greater risk and merit more aggressive treatment and management. This will require concerted effort to capture genetic information from patients with acute events that lead to seizures or increase seizure risk. With a sufficient sample size, some common polygenic factors might emerge, suggesting genes or genetic patterns that imply risk.6 In some cases, one might consider treating the predisposition if it can be identified as the first step to gain adequate seizure control before considering, for example, epilepsy surgery. This same form of analyses could be applied to traumatic brain injury, stroke, hypoxia, and other insults that enhance the likelihood of future seizures."}
MyTest
{"project":"MyTest","denotations":[{"id":"31965829-30661434-28638388","span":{"begin":111,"end":114},"obj":"30661434"},{"id":"31965829-30347190-28638389","span":{"begin":283,"end":286},"obj":"30347190"},{"id":"31965829-30347190-28638390","span":{"begin":1160,"end":1163},"obj":"30347190"},{"id":"31965829-31371124-28638391","span":{"begin":1674,"end":1677},"obj":"31371124"},{"id":"31965829-28564577-28638392","span":{"begin":2402,"end":2405},"obj":"28564577"},{"id":"31965829-29589874-28638393","span":{"begin":2623,"end":2625},"obj":"29589874"},{"id":"31965829-30755487-28638394","span":{"begin":2626,"end":2629},"obj":"30755487"},{"id":"31965829-27839871-28638395","span":{"begin":4358,"end":4360},"obj":"27839871"},{"id":"31965829-28095420-28638395","span":{"begin":4358,"end":4360},"obj":"28095420"},{"id":"31965829-31504254-28638395","span":{"begin":4358,"end":4360},"obj":"31504254"},{"id":"31965829-31429998-28638395","span":{"begin":4358,"end":4360},"obj":"31429998"},{"id":"31965829-28377535-28638395","span":{"begin":4358,"end":4360},"obj":"28377535"},{"id":"31965829-28095420-28638396","span":{"begin":4613,"end":4615},"obj":"28095420"},{"id":"31965829-24002024-28638397","span":{"begin":4616,"end":4618},"obj":"24002024"},{"id":"31965829-29915537-28638398","span":{"begin":4619,"end":4622},"obj":"29915537"},{"id":"31965829-25990557-28638399","span":{"begin":4978,"end":4981},"obj":"25990557"},{"id":"31965829-29589874-28638400","span":{"begin":6070,"end":6073},"obj":"29589874"},{"id":"31965829-31327507-28638401","span":{"begin":6632,"end":6634},"obj":"31327507"}],"namespaces":[{"prefix":"_base","uri":"https://www.uniprot.org/uniprot/testbase"},{"prefix":"UniProtKB","uri":"https://www.uniprot.org/uniprot/"},{"prefix":"uniprot","uri":"https://www.uniprot.org/uniprotkb/"}],"text":"Genetic Advances\nAn important consequence of the many genetic advances that are transforming clinical neurology39 is their ability to suggest new animal models to investigate the underlying disease mechanisms, including compensatory mechanisms that can contribute to a seizure focus.40 Such models are relevant to genetic human epilepsies and serve as an important complement to the acquired models of focal epilepsy (eg, pharmacologically induced seizure models) that have become the mainstay for development of in vivo models of chronic recurring seizures. Animal models of single-gene defects offer an opportunity to evaluate windows for therapeutic intervention in patients who have these specific variants, with the possibility that some therapies will be more broadly applicable to multiple epilepsies. In addition, such models offer a new opportunity to study common mechanisms that underlie maladaptive plasticity and can lead to generation of a seizure focus. Novel gene expression programs may be triggered by genetic deficiencies that engage similar mechanisms, and understanding these might allow better understanding of antiepileptic drug utility.40 In this respect, the intersection of gene expression data sets may inform key pathways that establish seizure foci regardless of the initial genetic defect driving seizures. In addition, genetic animal models can facilitate the evaluation and validation of strategies such as antisense oligonucleotides, gene replacement, and gene augmentation. The success of new genetic treatments of spinal muscular atrophy with intravenously delivered gene replacement via adeno-associated viral vector in very young infants41 has created hope for many patients that these therapies can correct other neurological conditions, stimulating work on this problem in academia and, importantly, in industry. Thus, there are actionable opportunities for genetic therapies for epilepsy on the horizon.\nIn addition to the value of new models suggested by genetic analysis, there are several opportunities to exploit advances in diagnostic and therapeutic genetic approaches. There are now multiple examples of strategies one could use to develop gene therapy employing viral vectors to treat focal and generalized epilepsies in animal models in which a missense variant or truncating mutation has modified the function of a target gene or reduced the gene dose.42 Other innovative uses of gene therapy include introduction of potassium channels that could reduce excitability, as well as engineering cells to release neuroactive molecules that can counteract excessive excitability.43,44 As more animal models are developed for different genes, there will be opportunities to test fundamental approaches that supplement underexpressed alleles or proteins with reduced function, as well as editing gene approaches to correct identified defects. These strategies will require demonstration of utility in animals with measurable defects, and the results will speak to the important question in epilepsy around whether symptoms are driven by the genetic defect, are a feature of maladaptive compensation, or reflect some combination of both. That is, there is a need for proof-of-concept data for oligonucleotide and antisense therapies for application in the treatment of genetically defined monogenic epilepsies, as well as data on effectiveness of the timing of treatment in the context of the development of a seizure focus. Advances are needed in genetic therapy using virus delivery vectors that are already approved for other payloads and access both brain and spinal cord following intrathecal administration. The rare genetic epilepsies might provide a test case for intervention, which can be evaluated in iPSC-based models in vitro, organoids derived from iPSC cells, and animal models now.\nOne opportunity that the accessibility of multiple new genetic models of human variants associated with epilepsy offers is evaluation of repurposed drugs. This requires a comprehensive functional evaluation of the effects of rare variants in vitro, which for ion channels is accessible. Functional evaluation of drug sensitivity of variant proteins will inform potential use of therapeutics, as will knowledge of the nature of the net functional effects as either gain of function or loss of function, or indeterminant.45-49 Genetic models—from cellular models to zebrafish and mouse models—harboring variants can then be screened for actions of Food and Drug Administration (FDA)-approved medications for efficacy in reducing electroencephalogram abnormalities and seizures46,50,51 as a step toward using pharmacological treatments. If the models capture patient-relevant features of epileptogenesis, early treatment within a vulnerable window might have long-lasting consequences.\nIn addition to these pharmacological approaches, bioinformatics coupled with large-scale data sets have driven the development of computational resources52,53 that can suggest candidate drugs in the FDA library from patterns of changes in gene expression. Moreover, evaluation of multiple drugs in multiple models might identify candidate drugs as add-on therapies that could be used more broadly than for just for rare genetic conditions. Indeed, a large number of epilepsy models have been or are being made from various genes identified in patients with rare epilepsies (eg, sodium channels, potassium channels, postsynaptic ligand-gated ion channels, synaptic proteins), which will provide patient-relevant models in which to assess new pharmacological strategies. These same models can be used to understand developmental compensation, transformation of the foci with time, and pharmacological sensitivity. It seems likely that some compensatory mechanisms will be shared across these different models and may inform treatment of refractory epilepsy. In addition to rodent models, use of companion models and organisms (fly, zebrafish, mice, iPSC-derived neuronal cultures, and organoids) could provide faster and more efficient drug screening43 as well as evaluation of compensatory mechanisms.\nThe advances in genetic analysis could also expand our understanding of acquired epilepsies and yield insight into whether persons with genetic predispositions may be at greater risk and merit more aggressive treatment and management. This will require concerted effort to capture genetic information from patients with acute events that lead to seizures or increase seizure risk. With a sufficient sample size, some common polygenic factors might emerge, suggesting genes or genetic patterns that imply risk.6 In some cases, one might consider treating the predisposition if it can be identified as the first step to gain adequate seizure control before considering, for example, epilepsy surgery. This same form of analyses could be applied to traumatic brain injury, stroke, hypoxia, and other insults that enhance the likelihood of future seizures."}
2_test
{"project":"2_test","denotations":[{"id":"31965829-30661434-28638388","span":{"begin":111,"end":113},"obj":"30661434"},{"id":"31965829-30347190-28638389","span":{"begin":283,"end":285},"obj":"30347190"},{"id":"31965829-30347190-28638390","span":{"begin":1160,"end":1162},"obj":"30347190"},{"id":"31965829-31371124-28638391","span":{"begin":1674,"end":1676},"obj":"31371124"},{"id":"31965829-28564577-28638392","span":{"begin":2402,"end":2404},"obj":"28564577"},{"id":"31965829-29589874-28638393","span":{"begin":2623,"end":2625},"obj":"29589874"},{"id":"31965829-30755487-28638394","span":{"begin":2626,"end":2628},"obj":"30755487"},{"id":"31965829-27839871-28638395","span":{"begin":4358,"end":4360},"obj":"27839871"},{"id":"31965829-28095420-28638395","span":{"begin":4358,"end":4360},"obj":"28095420"},{"id":"31965829-31504254-28638395","span":{"begin":4358,"end":4360},"obj":"31504254"},{"id":"31965829-31429998-28638395","span":{"begin":4358,"end":4360},"obj":"31429998"},{"id":"31965829-28377535-28638395","span":{"begin":4358,"end":4360},"obj":"28377535"},{"id":"31965829-28095420-28638396","span":{"begin":4613,"end":4615},"obj":"28095420"},{"id":"31965829-24002024-28638397","span":{"begin":4616,"end":4618},"obj":"24002024"},{"id":"31965829-29915537-28638398","span":{"begin":4619,"end":4621},"obj":"29915537"},{"id":"31965829-25990557-28638399","span":{"begin":4978,"end":4980},"obj":"25990557"},{"id":"31965829-29589874-28638400","span":{"begin":6070,"end":6072},"obj":"29589874"},{"id":"31965829-31327507-28638401","span":{"begin":6632,"end":6633},"obj":"31327507"}],"text":"Genetic Advances\nAn important consequence of the many genetic advances that are transforming clinical neurology39 is their ability to suggest new animal models to investigate the underlying disease mechanisms, including compensatory mechanisms that can contribute to a seizure focus.40 Such models are relevant to genetic human epilepsies and serve as an important complement to the acquired models of focal epilepsy (eg, pharmacologically induced seizure models) that have become the mainstay for development of in vivo models of chronic recurring seizures. Animal models of single-gene defects offer an opportunity to evaluate windows for therapeutic intervention in patients who have these specific variants, with the possibility that some therapies will be more broadly applicable to multiple epilepsies. In addition, such models offer a new opportunity to study common mechanisms that underlie maladaptive plasticity and can lead to generation of a seizure focus. Novel gene expression programs may be triggered by genetic deficiencies that engage similar mechanisms, and understanding these might allow better understanding of antiepileptic drug utility.40 In this respect, the intersection of gene expression data sets may inform key pathways that establish seizure foci regardless of the initial genetic defect driving seizures. In addition, genetic animal models can facilitate the evaluation and validation of strategies such as antisense oligonucleotides, gene replacement, and gene augmentation. The success of new genetic treatments of spinal muscular atrophy with intravenously delivered gene replacement via adeno-associated viral vector in very young infants41 has created hope for many patients that these therapies can correct other neurological conditions, stimulating work on this problem in academia and, importantly, in industry. Thus, there are actionable opportunities for genetic therapies for epilepsy on the horizon.\nIn addition to the value of new models suggested by genetic analysis, there are several opportunities to exploit advances in diagnostic and therapeutic genetic approaches. There are now multiple examples of strategies one could use to develop gene therapy employing viral vectors to treat focal and generalized epilepsies in animal models in which a missense variant or truncating mutation has modified the function of a target gene or reduced the gene dose.42 Other innovative uses of gene therapy include introduction of potassium channels that could reduce excitability, as well as engineering cells to release neuroactive molecules that can counteract excessive excitability.43,44 As more animal models are developed for different genes, there will be opportunities to test fundamental approaches that supplement underexpressed alleles or proteins with reduced function, as well as editing gene approaches to correct identified defects. These strategies will require demonstration of utility in animals with measurable defects, and the results will speak to the important question in epilepsy around whether symptoms are driven by the genetic defect, are a feature of maladaptive compensation, or reflect some combination of both. That is, there is a need for proof-of-concept data for oligonucleotide and antisense therapies for application in the treatment of genetically defined monogenic epilepsies, as well as data on effectiveness of the timing of treatment in the context of the development of a seizure focus. Advances are needed in genetic therapy using virus delivery vectors that are already approved for other payloads and access both brain and spinal cord following intrathecal administration. The rare genetic epilepsies might provide a test case for intervention, which can be evaluated in iPSC-based models in vitro, organoids derived from iPSC cells, and animal models now.\nOne opportunity that the accessibility of multiple new genetic models of human variants associated with epilepsy offers is evaluation of repurposed drugs. This requires a comprehensive functional evaluation of the effects of rare variants in vitro, which for ion channels is accessible. Functional evaluation of drug sensitivity of variant proteins will inform potential use of therapeutics, as will knowledge of the nature of the net functional effects as either gain of function or loss of function, or indeterminant.45-49 Genetic models—from cellular models to zebrafish and mouse models—harboring variants can then be screened for actions of Food and Drug Administration (FDA)-approved medications for efficacy in reducing electroencephalogram abnormalities and seizures46,50,51 as a step toward using pharmacological treatments. If the models capture patient-relevant features of epileptogenesis, early treatment within a vulnerable window might have long-lasting consequences.\nIn addition to these pharmacological approaches, bioinformatics coupled with large-scale data sets have driven the development of computational resources52,53 that can suggest candidate drugs in the FDA library from patterns of changes in gene expression. Moreover, evaluation of multiple drugs in multiple models might identify candidate drugs as add-on therapies that could be used more broadly than for just for rare genetic conditions. Indeed, a large number of epilepsy models have been or are being made from various genes identified in patients with rare epilepsies (eg, sodium channels, potassium channels, postsynaptic ligand-gated ion channels, synaptic proteins), which will provide patient-relevant models in which to assess new pharmacological strategies. These same models can be used to understand developmental compensation, transformation of the foci with time, and pharmacological sensitivity. It seems likely that some compensatory mechanisms will be shared across these different models and may inform treatment of refractory epilepsy. In addition to rodent models, use of companion models and organisms (fly, zebrafish, mice, iPSC-derived neuronal cultures, and organoids) could provide faster and more efficient drug screening43 as well as evaluation of compensatory mechanisms.\nThe advances in genetic analysis could also expand our understanding of acquired epilepsies and yield insight into whether persons with genetic predispositions may be at greater risk and merit more aggressive treatment and management. This will require concerted effort to capture genetic information from patients with acute events that lead to seizures or increase seizure risk. With a sufficient sample size, some common polygenic factors might emerge, suggesting genes or genetic patterns that imply risk.6 In some cases, one might consider treating the predisposition if it can be identified as the first step to gain adequate seizure control before considering, for example, epilepsy surgery. This same form of analyses could be applied to traumatic brain injury, stroke, hypoxia, and other insults that enhance the likelihood of future seizures."}
testtesttest
{"project":"testtesttest","denotations":[{"id":"T24","span":{"begin":3595,"end":3600},"obj":"Body_part"},{"id":"T26","span":{"begin":3605,"end":3616},"obj":"Body_part"},{"id":"T27","span":{"begin":4905,"end":4910},"obj":"Body_part"},{"id":"T28","span":{"begin":5936,"end":5945},"obj":"Body_part"},{"id":"T29","span":{"begin":6879,"end":6884},"obj":"Body_part"}],"attributes":[{"id":"A24","pred":"uberon_id","subj":"T24","obj":"http://purl.obolibrary.org/obo/UBERON_0000955"},{"id":"A25","pred":"uberon_id","subj":"T24","obj":"http://purl.obolibrary.org/obo/UBERON_6110636"},{"id":"A26","pred":"uberon_id","subj":"T26","obj":"http://purl.obolibrary.org/obo/UBERON_0002240"},{"id":"A27","pred":"uberon_id","subj":"T27","obj":"http://purl.obolibrary.org/obo/UBERON_0002542"},{"id":"A28","pred":"uberon_id","subj":"T28","obj":"http://purl.obolibrary.org/obo/UBERON_0000468"},{"id":"A29","pred":"uberon_id","subj":"T29","obj":"http://purl.obolibrary.org/obo/UBERON_0000955"},{"id":"A30","pred":"uberon_id","subj":"T29","obj":"http://purl.obolibrary.org/obo/UBERON_6110636"}],"text":"Genetic Advances\nAn important consequence of the many genetic advances that are transforming clinical neurology39 is their ability to suggest new animal models to investigate the underlying disease mechanisms, including compensatory mechanisms that can contribute to a seizure focus.40 Such models are relevant to genetic human epilepsies and serve as an important complement to the acquired models of focal epilepsy (eg, pharmacologically induced seizure models) that have become the mainstay for development of in vivo models of chronic recurring seizures. Animal models of single-gene defects offer an opportunity to evaluate windows for therapeutic intervention in patients who have these specific variants, with the possibility that some therapies will be more broadly applicable to multiple epilepsies. In addition, such models offer a new opportunity to study common mechanisms that underlie maladaptive plasticity and can lead to generation of a seizure focus. Novel gene expression programs may be triggered by genetic deficiencies that engage similar mechanisms, and understanding these might allow better understanding of antiepileptic drug utility.40 In this respect, the intersection of gene expression data sets may inform key pathways that establish seizure foci regardless of the initial genetic defect driving seizures. In addition, genetic animal models can facilitate the evaluation and validation of strategies such as antisense oligonucleotides, gene replacement, and gene augmentation. The success of new genetic treatments of spinal muscular atrophy with intravenously delivered gene replacement via adeno-associated viral vector in very young infants41 has created hope for many patients that these therapies can correct other neurological conditions, stimulating work on this problem in academia and, importantly, in industry. Thus, there are actionable opportunities for genetic therapies for epilepsy on the horizon.\nIn addition to the value of new models suggested by genetic analysis, there are several opportunities to exploit advances in diagnostic and therapeutic genetic approaches. There are now multiple examples of strategies one could use to develop gene therapy employing viral vectors to treat focal and generalized epilepsies in animal models in which a missense variant or truncating mutation has modified the function of a target gene or reduced the gene dose.42 Other innovative uses of gene therapy include introduction of potassium channels that could reduce excitability, as well as engineering cells to release neuroactive molecules that can counteract excessive excitability.43,44 As more animal models are developed for different genes, there will be opportunities to test fundamental approaches that supplement underexpressed alleles or proteins with reduced function, as well as editing gene approaches to correct identified defects. These strategies will require demonstration of utility in animals with measurable defects, and the results will speak to the important question in epilepsy around whether symptoms are driven by the genetic defect, are a feature of maladaptive compensation, or reflect some combination of both. That is, there is a need for proof-of-concept data for oligonucleotide and antisense therapies for application in the treatment of genetically defined monogenic epilepsies, as well as data on effectiveness of the timing of treatment in the context of the development of a seizure focus. Advances are needed in genetic therapy using virus delivery vectors that are already approved for other payloads and access both brain and spinal cord following intrathecal administration. The rare genetic epilepsies might provide a test case for intervention, which can be evaluated in iPSC-based models in vitro, organoids derived from iPSC cells, and animal models now.\nOne opportunity that the accessibility of multiple new genetic models of human variants associated with epilepsy offers is evaluation of repurposed drugs. This requires a comprehensive functional evaluation of the effects of rare variants in vitro, which for ion channels is accessible. Functional evaluation of drug sensitivity of variant proteins will inform potential use of therapeutics, as will knowledge of the nature of the net functional effects as either gain of function or loss of function, or indeterminant.45-49 Genetic models—from cellular models to zebrafish and mouse models—harboring variants can then be screened for actions of Food and Drug Administration (FDA)-approved medications for efficacy in reducing electroencephalogram abnormalities and seizures46,50,51 as a step toward using pharmacological treatments. If the models capture patient-relevant features of epileptogenesis, early treatment within a vulnerable window might have long-lasting consequences.\nIn addition to these pharmacological approaches, bioinformatics coupled with large-scale data sets have driven the development of computational resources52,53 that can suggest candidate drugs in the FDA library from patterns of changes in gene expression. Moreover, evaluation of multiple drugs in multiple models might identify candidate drugs as add-on therapies that could be used more broadly than for just for rare genetic conditions. Indeed, a large number of epilepsy models have been or are being made from various genes identified in patients with rare epilepsies (eg, sodium channels, potassium channels, postsynaptic ligand-gated ion channels, synaptic proteins), which will provide patient-relevant models in which to assess new pharmacological strategies. These same models can be used to understand developmental compensation, transformation of the foci with time, and pharmacological sensitivity. It seems likely that some compensatory mechanisms will be shared across these different models and may inform treatment of refractory epilepsy. In addition to rodent models, use of companion models and organisms (fly, zebrafish, mice, iPSC-derived neuronal cultures, and organoids) could provide faster and more efficient drug screening43 as well as evaluation of compensatory mechanisms.\nThe advances in genetic analysis could also expand our understanding of acquired epilepsies and yield insight into whether persons with genetic predispositions may be at greater risk and merit more aggressive treatment and management. This will require concerted effort to capture genetic information from patients with acute events that lead to seizures or increase seizure risk. With a sufficient sample size, some common polygenic factors might emerge, suggesting genes or genetic patterns that imply risk.6 In some cases, one might consider treating the predisposition if it can be identified as the first step to gain adequate seizure control before considering, for example, epilepsy surgery. This same form of analyses could be applied to traumatic brain injury, stroke, hypoxia, and other insults that enhance the likelihood of future seizures."}