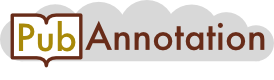
PMC:6194691 / 177170-187187
Annnotations
MyTest
{"project":"MyTest","denotations":[{"id":"30340614-696840-30706563","span":{"begin":3401,"end":3404},"obj":"696840"},{"id":"30340614-6786001-30706564","span":{"begin":3426,"end":3429},"obj":"6786001"},{"id":"30340614-6424869-30706565","span":{"begin":3459,"end":3462},"obj":"6424869"},{"id":"30340614-5555788-30706566","span":{"begin":3497,"end":3500},"obj":"5555788"},{"id":"30340614-3925086-30706567","span":{"begin":3528,"end":3531},"obj":"3925086"},{"id":"30340614-3098986-30706568","span":{"begin":3562,"end":3565},"obj":"3098986"},{"id":"30340614-696840-30706569","span":{"begin":3669,"end":3672},"obj":"696840"},{"id":"30340614-6786001-30706570","span":{"begin":3692,"end":3695},"obj":"6786001"},{"id":"30340614-6424869-30706571","span":{"begin":3723,"end":3726},"obj":"6424869"},{"id":"30340614-13814544-30706572","span":{"begin":3762,"end":3765},"obj":"13814544"},{"id":"30340614-14103562-30706573","span":{"begin":3797,"end":3800},"obj":"14103562"},{"id":"30340614-5371163-30706574","span":{"begin":3833,"end":3836},"obj":"5371163"},{"id":"30340614-3098986-30706575","span":{"begin":3867,"end":3870},"obj":"3098986"},{"id":"30340614-2460193-30706576","span":{"begin":3933,"end":3936},"obj":"2460193"},{"id":"30340614-11907803-30706577","span":{"begin":3975,"end":3978},"obj":"11907803"},{"id":"30340614-28779418-30706578","span":{"begin":4007,"end":4010},"obj":"28779418"},{"id":"30340614-28779418-30706579","span":{"begin":4052,"end":4055},"obj":"28779418"},{"id":"30340614-696840-30706580","span":{"begin":4128,"end":4131},"obj":"696840"},{"id":"30340614-6786001-30706581","span":{"begin":4157,"end":4160},"obj":"6786001"},{"id":"30340614-6424869-30706582","span":{"begin":4190,"end":4193},"obj":"6424869"},{"id":"30340614-14103562-30706583","span":{"begin":4225,"end":4228},"obj":"14103562"},{"id":"30340614-3925086-30706584","span":{"begin":4262,"end":4265},"obj":"3925086"},{"id":"30340614-2460193-30706585","span":{"begin":4284,"end":4287},"obj":"2460193"},{"id":"30340614-11907803-30706586","span":{"begin":4325,"end":4328},"obj":"11907803"},{"id":"30340614-8667222-30706587","span":{"begin":4356,"end":4359},"obj":"8667222"},{"id":"30340614-27799072-30706588","span":{"begin":6932,"end":6933},"obj":"27799072"},{"id":"30340614-25678956-30706589","span":{"begin":6935,"end":6937},"obj":"25678956"}],"namespaces":[{"prefix":"_base","uri":"https://www.uniprot.org/uniprot/testbase"},{"prefix":"UniProtKB","uri":"https://www.uniprot.org/uniprot/"},{"prefix":"uniprot","uri":"https://www.uniprot.org/uniprotkb/"}],"text":"Summary\nSubstances can be eliminated from the brain parenchyma either by metabolism or efflux. This review considers efflux, which can occur via perivascular routes or via the blood–brain barrier. The quantitative importance of these different mechanisms is assessed using clearance defined as the rate of elimination of the substance from interstitial fluid (ISF) divided by its ISF concentration (see Appendix A). If the rate of elimination and the concentration can both be measured, the clearance is calculated using this definition. Often, however, it is calculated from the half-life and volume of distribution of the substance as explained in Appendix A. The total clearance of a substance is the sum of its clearances by all mechanisms.\nTable 6 (see also Tables 1 and 7) lists a number of substances that are cleared by different mechanisms together with indication of the values of their clearances. The last row in the table indicates the clearance of markers for perivascular elimination. These are substances that are known to be neither transported across the blood–brain barrier nor metabolised at a significant rate but leave entirely by perivascular routes (see Appendix B). Each of these markers has a total clearance that is similar to the others. Every water soluble substance in ISF will have a total clearance at least this large, about 1 µL g−1 min−1, because the clearances by other mechanisms will be added on top of this basal value.\nTable 6 Overview of efflux routes showing clearance values for substances leaving the brain parenchyma from ISF\nSubstances Features Clearance/µL g−1 min−1\nPassive, non-specific transfer across the blood–brain barrier\n H2Oa, CO2b, O2c, NH3c Very small molecules 1000–7000a, \u003e 6500 largeb\n Methanol, ethanol, antipyrine, isopropanol Highly lipid soluble moleculesd \u003e 100\n Glycerol, ethylene glycol, butyric acid Moderately lipid solubled 100 \u003e CL \u003e 10\nTransfer across the blood–brain barrier by specific transporters\n Glucosee Via GLUT1 50–100\n Lactatef Via MCT1 60–100\n Many substratesg Via Slc22 and Slco transporters 11–364\n Amino acidsh Via L, A, ASC, N, y+, EAAT and others Seeh\n K+i Via several routes including the Na+ pump and NKCC1i 11.3\nEfflux via blood–brain barrier and perivascular fluxes\n Amyloid-βk Primarily across blood–brain barrier ~10k\n Na+ j, Cl− j mannitoll Via both blood–brain barrier and perivascular routes c. 1–2\nEfflux via perivascular routes only\n Sucrose, inulin, albumin, larger dextrans and PEGsm Used as markers for perivascular efflux c. 1\nValues substantially greater than ~ 1 µL g−1 min−1 imply that clearance is primarily across the blood–brain barrier rather than via perivascular efflux\naSee Sect. 5.1\nbSee Sect. 5.2\ncClearance known to be large but difficult to measure\ndSee Fig. 8\neSee Sect. 5.3 and Appendix D\nfSee Sect. 5.4\ngSee Table 1\nhNet fluxes at blood–brain barrier 1–20 nmol g−1 min−1, perivascular effluxes (except glutamine) ~ 0.1 nmol g−1 min−1, glutamine ~ 1 nmol g−1 min−1\niSee Appendix E, NKCC1 is the Na+, K+, 2Cl−—cotransporter; jsee Appendix E\nkSee Sect. 5.7.3\nlSee Appendix B\nmNegligible blood–brain barrier clearance, see Sect. 3 and Appendix B\nTable 7 Blood–brain permeability-surface area products (PS) from influx data and calculated blood–brain barrier efflux rate constants, keff,BBB for mannitol, sucrose and inulin\nPS/(10−2 µL g−1 min−1) keff,BBB/min−1\nMannitol\n Ohno et al. [591] 180 0.0090\n Amtorp [592] 121 0.0061\n Preston et al. [593] 72 0.0036\n Sisson and Oldendorf [594] 10 0.0005\n Daniel et al. [595] 49 0.0025\n Preston and Haas [531] as purchaseda, c 68 0.0034\n Purifiedc 44 0.0022\n Average 0.0040\n s.e.m 0.0013\nSucrose\n Ohno et al. [591] 40 0.002\n Amtorp [592] 20 0.001\n Preston et al. [593] 48 0.0024\n Davson and Spaziani # [596] 22 0.0011\n Reed and Woodbury [597] 5.3 0.00026\n Cameron et al. # [598] 33 0.0017\n Preston and Haas [531] as purchaseda, c 37 0.0019\n Purifiedc 15.3 0.00076\n Smith [599] 24 0.0012\n Preston and Webster 2002 [600] 11.8 0.00059\n Miah et al. [532] radiolabelleda 40 0.002\n Miah et al. [532] mass spec. 4 0.0002\n Average 0.0011\n s.e.m 0.0002\nInulin\n Ohno et al. [591] 1.44–2 0.00008\n Amtorp [592] 15 0.00075\n Preston et al. [593] 9 0.00045\n Reed and Woodbury [597] \u003c ~ 0.3 0.00002\n Daniel et al. [595] 6 0.0003\n Smith [599] \u003c 1.5 0.00006\n Preston and Webster [600] 3.9 0.0002\n Kakee et al. [601]b 30 0.0015\n Average 0.00027\n s.e.m 0.00010\nThe volume of distribution is assumed to be 0.2 mL g−1. Italic values are excluded from calculations because athey are for comparison with the accompanying values or bit is an outlier, more than 4 st. dev. from the mean of the other values for inulin. The values for mannitol and sucrose marked care reported for the radiolabelled substances both as purchased and following purification to remove impurities which might be transported more rapidly (see text). The average for sucrose is significantly different from than that for mannitol, p = 0.012, and that for inulin different from that for sucrose, p = 0.01. Data obtained with rats (except # in rabbits)\nPerivascular transport is a relatively non-selective flow-based process. The mechanism and detailed route of this transport have attracted a great deal of attention. Controversies still not finally resolved include: (i) the direction of solute movements and flows in periarterial and perivenous routes (Sects. 3.1 and 3.2); (ii) whether perivascular pathways are spaces containing free fluid or basement membranes (Sect. 3.1” section and Footnote 5); (iii) the driving force for the flows (Sect. 3.2); (iv) whether the immediate destination of perivascular efflux is CSF or lymph (Sect. 3.1, Fig. 6 and Footnote 6); and (v) whether there is enough flow either through the parenchyma or via perivascular routes along capillaries to allow there to be a net periarterial inflow and a net perivenous outflow as proposed in the glymphatic hypothesis (Sects. 3.2 and 3.2.1). The evidence for the involvement of flow or convection in perivascular transport of solutes is convincing but flow appears not to be important for transfers of solutes within the interstitial spaces of the parenchyma. Further work is required before it will be possible to reach a definite conclusion whether or not there is a net inward flow along arteries and net outward flow along veins as proposed in the glymphatic hypothesis.\nTransport across the blood–brain barrier can occur by a number of different mechanisms (see Fig. 3). The simplest of these, appropriate for small molecules that are lipid soluble, is diffusion across the lipid membranes and cytoplasm of brain endothelial cells (Sect. 4.1 and Appendix C). The blood–brain barrier is the main route for the large fluxes of water measured using tracers (Sect. 5.1), but it is almost certainly not the main route for the net inward flux of water into the brain because it is not the main route for net inward flux of Na+ or Cl− (see [4, 41]). The net inward flux of water, which occurs primarily at the choroid plexuses, together with the metabolic production of water, must balance the net outward flow of water as CSF and ISF are returned to blood and/or lymph. With the polar solutes Na+, Cl−, and mannitol the small fluxes that have been observed in tracer studies may be via a small “leak” through the tight junctions (Sects. 4.1 and 5.6 and Appendix B).\nFor many relatively small polar molecules there are specific transporters in the membranes (Sects. 4.2 and 5.2 through 5.6). Not surprisingly GLUT1, the transporter for glucose, is highly abundant in the endothelial cell membranes and glucose transport is rapid (Sect. 5.3 and Appendix D). The need for an increased glucose supply during periods of enhanced nervous activity is considered in Sect. 6.2. While lactate is also transported rapidly across the blood–brain barrier at low concentrations (Sect. 5.4), during nervous activity lactate must to some extent either be transferred to inactive regions within the brain or be effluxed to CSF or lymph. Amino acid transport (Sect. 5.5) is more complicated in that there are many different amino acids that are to some extent inter-convertible by transamination. Furthermore there are many different transporters with differing but overlapping substrate preferences (Sect. 5.5.6). The largest fluxes of amino acids across the blood–brain barrier measured using radiotracers are for the large neutral amino acids. However, these occur via a system that mediates obligatory exchanges of amino acids without resulting in an overall net flux (Sect. 5.5.4). The net inward flux of the large neutral amino acids is small compared to the rate at which they are used to allow synthesis of proteins and the neurotransmitters, glutamate and GABA. This implies substantial reuse of amino acids and the corresponding α-keto-acids when proteins, glutamate and GABA are catabolized within the cells (see Sect. 5.5.5).\nReceptor mediated transcytosis can transport large molecules across the blood–brain barrier. Amyloid-β is an important example (Sect. 5.7) of a substance primarily eliminated by this mechanism. Amyloid-β can also leave the brain parenchyma via perivascular efflux and this may be important as the route by which amyloid-β reaches arterial walls resulting in cerebral amyloid angiopathy.\nThe majority of substances listed in Table 6 have total clearances greater than those for inulin and sucrose, which implies that they are leaving the brain by routes in addition to the perivascular pathways. For those whose clearances are not much greater than 1 µL g−1 min−1, perivascular transport will still make a noticeable contribution. Na+ and Cl− enter and leave the brain parenchyma by perivascular and blood–brain barrier routes and both will be important in processes like the development and resolution of oedema (not considered in this review). Non-metabolized substances with clearances greater than about 10 µL g−1 min−1 leave the brain parenchyma primarily via transport across the blood–brain barrier."}
2_test
{"project":"2_test","denotations":[{"id":"30340614-696840-30706563","span":{"begin":3401,"end":3404},"obj":"696840"},{"id":"30340614-6786001-30706564","span":{"begin":3426,"end":3429},"obj":"6786001"},{"id":"30340614-6424869-30706565","span":{"begin":3459,"end":3462},"obj":"6424869"},{"id":"30340614-5555788-30706566","span":{"begin":3497,"end":3500},"obj":"5555788"},{"id":"30340614-3925086-30706567","span":{"begin":3528,"end":3531},"obj":"3925086"},{"id":"30340614-3098986-30706568","span":{"begin":3562,"end":3565},"obj":"3098986"},{"id":"30340614-696840-30706569","span":{"begin":3669,"end":3672},"obj":"696840"},{"id":"30340614-6786001-30706570","span":{"begin":3692,"end":3695},"obj":"6786001"},{"id":"30340614-6424869-30706571","span":{"begin":3723,"end":3726},"obj":"6424869"},{"id":"30340614-13814544-30706572","span":{"begin":3762,"end":3765},"obj":"13814544"},{"id":"30340614-14103562-30706573","span":{"begin":3797,"end":3800},"obj":"14103562"},{"id":"30340614-5371163-30706574","span":{"begin":3833,"end":3836},"obj":"5371163"},{"id":"30340614-3098986-30706575","span":{"begin":3867,"end":3870},"obj":"3098986"},{"id":"30340614-2460193-30706576","span":{"begin":3933,"end":3936},"obj":"2460193"},{"id":"30340614-11907803-30706577","span":{"begin":3975,"end":3978},"obj":"11907803"},{"id":"30340614-28779418-30706578","span":{"begin":4007,"end":4010},"obj":"28779418"},{"id":"30340614-28779418-30706579","span":{"begin":4052,"end":4055},"obj":"28779418"},{"id":"30340614-696840-30706580","span":{"begin":4128,"end":4131},"obj":"696840"},{"id":"30340614-6786001-30706581","span":{"begin":4157,"end":4160},"obj":"6786001"},{"id":"30340614-6424869-30706582","span":{"begin":4190,"end":4193},"obj":"6424869"},{"id":"30340614-14103562-30706583","span":{"begin":4225,"end":4228},"obj":"14103562"},{"id":"30340614-3925086-30706584","span":{"begin":4262,"end":4265},"obj":"3925086"},{"id":"30340614-2460193-30706585","span":{"begin":4284,"end":4287},"obj":"2460193"},{"id":"30340614-11907803-30706586","span":{"begin":4325,"end":4328},"obj":"11907803"},{"id":"30340614-8667222-30706587","span":{"begin":4356,"end":4359},"obj":"8667222"},{"id":"30340614-27799072-30706588","span":{"begin":6932,"end":6933},"obj":"27799072"},{"id":"30340614-25678956-30706589","span":{"begin":6935,"end":6937},"obj":"25678956"}],"text":"Summary\nSubstances can be eliminated from the brain parenchyma either by metabolism or efflux. This review considers efflux, which can occur via perivascular routes or via the blood–brain barrier. The quantitative importance of these different mechanisms is assessed using clearance defined as the rate of elimination of the substance from interstitial fluid (ISF) divided by its ISF concentration (see Appendix A). If the rate of elimination and the concentration can both be measured, the clearance is calculated using this definition. Often, however, it is calculated from the half-life and volume of distribution of the substance as explained in Appendix A. The total clearance of a substance is the sum of its clearances by all mechanisms.\nTable 6 (see also Tables 1 and 7) lists a number of substances that are cleared by different mechanisms together with indication of the values of their clearances. The last row in the table indicates the clearance of markers for perivascular elimination. These are substances that are known to be neither transported across the blood–brain barrier nor metabolised at a significant rate but leave entirely by perivascular routes (see Appendix B). Each of these markers has a total clearance that is similar to the others. Every water soluble substance in ISF will have a total clearance at least this large, about 1 µL g−1 min−1, because the clearances by other mechanisms will be added on top of this basal value.\nTable 6 Overview of efflux routes showing clearance values for substances leaving the brain parenchyma from ISF\nSubstances Features Clearance/µL g−1 min−1\nPassive, non-specific transfer across the blood–brain barrier\n H2Oa, CO2b, O2c, NH3c Very small molecules 1000–7000a, \u003e 6500 largeb\n Methanol, ethanol, antipyrine, isopropanol Highly lipid soluble moleculesd \u003e 100\n Glycerol, ethylene glycol, butyric acid Moderately lipid solubled 100 \u003e CL \u003e 10\nTransfer across the blood–brain barrier by specific transporters\n Glucosee Via GLUT1 50–100\n Lactatef Via MCT1 60–100\n Many substratesg Via Slc22 and Slco transporters 11–364\n Amino acidsh Via L, A, ASC, N, y+, EAAT and others Seeh\n K+i Via several routes including the Na+ pump and NKCC1i 11.3\nEfflux via blood–brain barrier and perivascular fluxes\n Amyloid-βk Primarily across blood–brain barrier ~10k\n Na+ j, Cl− j mannitoll Via both blood–brain barrier and perivascular routes c. 1–2\nEfflux via perivascular routes only\n Sucrose, inulin, albumin, larger dextrans and PEGsm Used as markers for perivascular efflux c. 1\nValues substantially greater than ~ 1 µL g−1 min−1 imply that clearance is primarily across the blood–brain barrier rather than via perivascular efflux\naSee Sect. 5.1\nbSee Sect. 5.2\ncClearance known to be large but difficult to measure\ndSee Fig. 8\neSee Sect. 5.3 and Appendix D\nfSee Sect. 5.4\ngSee Table 1\nhNet fluxes at blood–brain barrier 1–20 nmol g−1 min−1, perivascular effluxes (except glutamine) ~ 0.1 nmol g−1 min−1, glutamine ~ 1 nmol g−1 min−1\niSee Appendix E, NKCC1 is the Na+, K+, 2Cl−—cotransporter; jsee Appendix E\nkSee Sect. 5.7.3\nlSee Appendix B\nmNegligible blood–brain barrier clearance, see Sect. 3 and Appendix B\nTable 7 Blood–brain permeability-surface area products (PS) from influx data and calculated blood–brain barrier efflux rate constants, keff,BBB for mannitol, sucrose and inulin\nPS/(10−2 µL g−1 min−1) keff,BBB/min−1\nMannitol\n Ohno et al. [591] 180 0.0090\n Amtorp [592] 121 0.0061\n Preston et al. [593] 72 0.0036\n Sisson and Oldendorf [594] 10 0.0005\n Daniel et al. [595] 49 0.0025\n Preston and Haas [531] as purchaseda, c 68 0.0034\n Purifiedc 44 0.0022\n Average 0.0040\n s.e.m 0.0013\nSucrose\n Ohno et al. [591] 40 0.002\n Amtorp [592] 20 0.001\n Preston et al. [593] 48 0.0024\n Davson and Spaziani # [596] 22 0.0011\n Reed and Woodbury [597] 5.3 0.00026\n Cameron et al. # [598] 33 0.0017\n Preston and Haas [531] as purchaseda, c 37 0.0019\n Purifiedc 15.3 0.00076\n Smith [599] 24 0.0012\n Preston and Webster 2002 [600] 11.8 0.00059\n Miah et al. [532] radiolabelleda 40 0.002\n Miah et al. [532] mass spec. 4 0.0002\n Average 0.0011\n s.e.m 0.0002\nInulin\n Ohno et al. [591] 1.44–2 0.00008\n Amtorp [592] 15 0.00075\n Preston et al. [593] 9 0.00045\n Reed and Woodbury [597] \u003c ~ 0.3 0.00002\n Daniel et al. [595] 6 0.0003\n Smith [599] \u003c 1.5 0.00006\n Preston and Webster [600] 3.9 0.0002\n Kakee et al. [601]b 30 0.0015\n Average 0.00027\n s.e.m 0.00010\nThe volume of distribution is assumed to be 0.2 mL g−1. Italic values are excluded from calculations because athey are for comparison with the accompanying values or bit is an outlier, more than 4 st. dev. from the mean of the other values for inulin. The values for mannitol and sucrose marked care reported for the radiolabelled substances both as purchased and following purification to remove impurities which might be transported more rapidly (see text). The average for sucrose is significantly different from than that for mannitol, p = 0.012, and that for inulin different from that for sucrose, p = 0.01. Data obtained with rats (except # in rabbits)\nPerivascular transport is a relatively non-selective flow-based process. The mechanism and detailed route of this transport have attracted a great deal of attention. Controversies still not finally resolved include: (i) the direction of solute movements and flows in periarterial and perivenous routes (Sects. 3.1 and 3.2); (ii) whether perivascular pathways are spaces containing free fluid or basement membranes (Sect. 3.1” section and Footnote 5); (iii) the driving force for the flows (Sect. 3.2); (iv) whether the immediate destination of perivascular efflux is CSF or lymph (Sect. 3.1, Fig. 6 and Footnote 6); and (v) whether there is enough flow either through the parenchyma or via perivascular routes along capillaries to allow there to be a net periarterial inflow and a net perivenous outflow as proposed in the glymphatic hypothesis (Sects. 3.2 and 3.2.1). The evidence for the involvement of flow or convection in perivascular transport of solutes is convincing but flow appears not to be important for transfers of solutes within the interstitial spaces of the parenchyma. Further work is required before it will be possible to reach a definite conclusion whether or not there is a net inward flow along arteries and net outward flow along veins as proposed in the glymphatic hypothesis.\nTransport across the blood–brain barrier can occur by a number of different mechanisms (see Fig. 3). The simplest of these, appropriate for small molecules that are lipid soluble, is diffusion across the lipid membranes and cytoplasm of brain endothelial cells (Sect. 4.1 and Appendix C). The blood–brain barrier is the main route for the large fluxes of water measured using tracers (Sect. 5.1), but it is almost certainly not the main route for the net inward flux of water into the brain because it is not the main route for net inward flux of Na+ or Cl− (see [4, 41]). The net inward flux of water, which occurs primarily at the choroid plexuses, together with the metabolic production of water, must balance the net outward flow of water as CSF and ISF are returned to blood and/or lymph. With the polar solutes Na+, Cl−, and mannitol the small fluxes that have been observed in tracer studies may be via a small “leak” through the tight junctions (Sects. 4.1 and 5.6 and Appendix B).\nFor many relatively small polar molecules there are specific transporters in the membranes (Sects. 4.2 and 5.2 through 5.6). Not surprisingly GLUT1, the transporter for glucose, is highly abundant in the endothelial cell membranes and glucose transport is rapid (Sect. 5.3 and Appendix D). The need for an increased glucose supply during periods of enhanced nervous activity is considered in Sect. 6.2. While lactate is also transported rapidly across the blood–brain barrier at low concentrations (Sect. 5.4), during nervous activity lactate must to some extent either be transferred to inactive regions within the brain or be effluxed to CSF or lymph. Amino acid transport (Sect. 5.5) is more complicated in that there are many different amino acids that are to some extent inter-convertible by transamination. Furthermore there are many different transporters with differing but overlapping substrate preferences (Sect. 5.5.6). The largest fluxes of amino acids across the blood–brain barrier measured using radiotracers are for the large neutral amino acids. However, these occur via a system that mediates obligatory exchanges of amino acids without resulting in an overall net flux (Sect. 5.5.4). The net inward flux of the large neutral amino acids is small compared to the rate at which they are used to allow synthesis of proteins and the neurotransmitters, glutamate and GABA. This implies substantial reuse of amino acids and the corresponding α-keto-acids when proteins, glutamate and GABA are catabolized within the cells (see Sect. 5.5.5).\nReceptor mediated transcytosis can transport large molecules across the blood–brain barrier. Amyloid-β is an important example (Sect. 5.7) of a substance primarily eliminated by this mechanism. Amyloid-β can also leave the brain parenchyma via perivascular efflux and this may be important as the route by which amyloid-β reaches arterial walls resulting in cerebral amyloid angiopathy.\nThe majority of substances listed in Table 6 have total clearances greater than those for inulin and sucrose, which implies that they are leaving the brain by routes in addition to the perivascular pathways. For those whose clearances are not much greater than 1 µL g−1 min−1, perivascular transport will still make a noticeable contribution. Na+ and Cl− enter and leave the brain parenchyma by perivascular and blood–brain barrier routes and both will be important in processes like the development and resolution of oedema (not considered in this review). Non-metabolized substances with clearances greater than about 10 µL g−1 min−1 leave the brain parenchyma primarily via transport across the blood–brain barrier."}