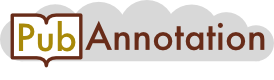
PMC:6194691 / 136879-141253
Annnotations
MyTest
{"project":"MyTest","denotations":[{"id":"30340614-5499038-30706431","span":{"begin":51,"end":54},"obj":"5499038"},{"id":"30340614-5124569-30706432","span":{"begin":56,"end":59},"obj":"5124569"},{"id":"30340614-27799072-30706433","span":{"begin":165,"end":166},"obj":"27799072"},{"id":"30340614-3468856-30706434","span":{"begin":195,"end":198},"obj":"3468856"},{"id":"30340614-6332899-30706435","span":{"begin":200,"end":203},"obj":"6332899"},{"id":"30340614-27799072-30706436","span":{"begin":335,"end":336},"obj":"27799072"},{"id":"30340614-27799072-30706437","span":{"begin":458,"end":459},"obj":"27799072"},{"id":"30340614-5124569-30706438","span":{"begin":556,"end":559},"obj":"5124569"},{"id":"30340614-3084708-30706439","span":{"begin":664,"end":667},"obj":"3084708"},{"id":"30340614-27799072-30706440","span":{"begin":1357,"end":1358},"obj":"27799072"},{"id":"30340614-25678956-30706441","span":{"begin":3335,"end":3337},"obj":"25678956"},{"id":"30340614-27799072-30706442","span":{"begin":3359,"end":3360},"obj":"27799072"},{"id":"30340614-5394498-30706443","span":{"begin":3593,"end":3596},"obj":"5394498"},{"id":"30340614-28969373-30706444","span":{"begin":4370,"end":4372},"obj":"28969373"}],"namespaces":[{"prefix":"_base","uri":"https://www.uniprot.org/uniprot/testbase"},{"prefix":"UniProtKB","uri":"https://www.uniprot.org/uniprot/"},{"prefix":"uniprot","uri":"https://www.uniprot.org/uniprotkb/"}],"text":"Na+ and Cl−\nIt has been known for almost 50 years [152, 417] that influx and efflux of Na+ and Cl− across the blood–brain barrier are much larger than the net flux [4]. It was proposed by Crone [151, 418] that these apparently passive fluxes might well be paracellular, a suggestion that is still in agreement with all available data [4]. (The partial inhibitions seen in some studies with amiloride derivatives are discussed in Sections 4.3.3 and 4.3.4 of [4]).\nThe permeability of the blood–brain barrier to Na+ was measured by Davson and Welch in 1971 [417] and subsequently using a different experimental and analytical approach by Smith and Rapoport in 1986 [419] (see Appendix E). Because the fluxes in and out across the barrier are nearly in balance and the potential difference across the barrier is small, the PS product measured for influx, ~ 1 µL min−1 g−1 for each ion, can be used as an estimate for that for efflux, i.e. for the clearance via the barrier (see Appendix A). This cannot be exactly true, because there is a component of active transport, but inhibition of the Na+-pump has little effect on the tracer fluxes. This is consistent with both passive influx and passive efflux being much larger than both active transport and the net flux, and with active transport making a major contribution to the net flux (see Section 4.3.5 in [4]).\nPerivascular transport does make a contribution to the clearances of Na+ and Cl−. Perhaps more importantly the net perivascular transport of each, the difference between influx and efflux, will be closely similar in size to the net transport across the blood–brain barrier, so that the volume, Na+ content and Cl− content of the parenchyma can be constant. The net transport of each across the blood–brain barrier is close to its concentration times the rate of fluid secretion across the blood–brain barrier in the steady-state. The controversy over whether perivascular influx and efflux occur along the same vessels or instead there is a glymphatic circulation with influx primarily by periarterial routes and efflux primarily by perivenular routes was considered in Sect. 4.2.\nThe net transports across the blood–brain barrier and via perivascular routes need not be exactly equal because there will be some component of diffusion between ISF and CSF at the brain surfaces, e.g. across the ependyma lining the ventricles and across the pia/glial layers. As indicated in Fig. 2 (see also [420], blood vessels enter and leave the parenchyma from subarachnoid spaces and cisterns and not from the ventricles. Thus transport from parenchyma to the ventricles will be primarily by diffusion probably with a component of flow in white matter (see Footnote 2) but it cannot be perivascular.\nThe possibility that there can be a small but significant net perivascular outflow from the parenchyma of Na+, Cl− and accompanying water may be the resolution of a long-standing difficulty. In non-communicating hydrocephalus, CSF production by the choroid plexuses continues at a nearly normal rate, but the normal route for CSF outflow from the IIIrd ventricle is blocked. Because after an initial period the ventricles do not continue to enlarge at a rate sufficient to accommodate the CSF production, CSF must be escaping via an alternative route (see Sections 4.2.2.1–4.2.2.2 in [41] and Section 4.1 in [4] for discussion and references). The periventricular parenchyma is oedematous which may allow flow of fluid from the ventricles, but the oedema only extends a small distance. In cats with kaolin induced hydrocephalus, Sahar et al. [421] observed penetration of serum albumin only up to about 2.5 mm which they took to mean that the albumin was being absorbed into the blood. There is no known mechanism by which this absorption could have occurred. It would be very interesting to know whether this distance corresponds instead to the distance from the ventricular surface to perivascular pathways that would allow sufficiently rapid removal of albumin to CSF in the subarachnoid spaces and/or to lymph that the concentrations observed deeper in the parenchyma would be small. The importance of fluid escape from the ventricles across the ependyma into the parenchyma in hydrocephalus has recently been given further support by observations of gadobutrol movements in normal pressure hydrocephalus in humans [15]."}
2_test
{"project":"2_test","denotations":[{"id":"30340614-5499038-30706431","span":{"begin":51,"end":54},"obj":"5499038"},{"id":"30340614-5124569-30706432","span":{"begin":56,"end":59},"obj":"5124569"},{"id":"30340614-27799072-30706433","span":{"begin":165,"end":166},"obj":"27799072"},{"id":"30340614-3468856-30706434","span":{"begin":195,"end":198},"obj":"3468856"},{"id":"30340614-6332899-30706435","span":{"begin":200,"end":203},"obj":"6332899"},{"id":"30340614-27799072-30706436","span":{"begin":335,"end":336},"obj":"27799072"},{"id":"30340614-27799072-30706437","span":{"begin":458,"end":459},"obj":"27799072"},{"id":"30340614-5124569-30706438","span":{"begin":556,"end":559},"obj":"5124569"},{"id":"30340614-3084708-30706439","span":{"begin":664,"end":667},"obj":"3084708"},{"id":"30340614-27799072-30706440","span":{"begin":1357,"end":1358},"obj":"27799072"},{"id":"30340614-25678956-30706441","span":{"begin":3335,"end":3337},"obj":"25678956"},{"id":"30340614-27799072-30706442","span":{"begin":3359,"end":3360},"obj":"27799072"},{"id":"30340614-5394498-30706443","span":{"begin":3593,"end":3596},"obj":"5394498"},{"id":"30340614-28969373-30706444","span":{"begin":4370,"end":4372},"obj":"28969373"}],"text":"Na+ and Cl−\nIt has been known for almost 50 years [152, 417] that influx and efflux of Na+ and Cl− across the blood–brain barrier are much larger than the net flux [4]. It was proposed by Crone [151, 418] that these apparently passive fluxes might well be paracellular, a suggestion that is still in agreement with all available data [4]. (The partial inhibitions seen in some studies with amiloride derivatives are discussed in Sections 4.3.3 and 4.3.4 of [4]).\nThe permeability of the blood–brain barrier to Na+ was measured by Davson and Welch in 1971 [417] and subsequently using a different experimental and analytical approach by Smith and Rapoport in 1986 [419] (see Appendix E). Because the fluxes in and out across the barrier are nearly in balance and the potential difference across the barrier is small, the PS product measured for influx, ~ 1 µL min−1 g−1 for each ion, can be used as an estimate for that for efflux, i.e. for the clearance via the barrier (see Appendix A). This cannot be exactly true, because there is a component of active transport, but inhibition of the Na+-pump has little effect on the tracer fluxes. This is consistent with both passive influx and passive efflux being much larger than both active transport and the net flux, and with active transport making a major contribution to the net flux (see Section 4.3.5 in [4]).\nPerivascular transport does make a contribution to the clearances of Na+ and Cl−. Perhaps more importantly the net perivascular transport of each, the difference between influx and efflux, will be closely similar in size to the net transport across the blood–brain barrier, so that the volume, Na+ content and Cl− content of the parenchyma can be constant. The net transport of each across the blood–brain barrier is close to its concentration times the rate of fluid secretion across the blood–brain barrier in the steady-state. The controversy over whether perivascular influx and efflux occur along the same vessels or instead there is a glymphatic circulation with influx primarily by periarterial routes and efflux primarily by perivenular routes was considered in Sect. 4.2.\nThe net transports across the blood–brain barrier and via perivascular routes need not be exactly equal because there will be some component of diffusion between ISF and CSF at the brain surfaces, e.g. across the ependyma lining the ventricles and across the pia/glial layers. As indicated in Fig. 2 (see also [420], blood vessels enter and leave the parenchyma from subarachnoid spaces and cisterns and not from the ventricles. Thus transport from parenchyma to the ventricles will be primarily by diffusion probably with a component of flow in white matter (see Footnote 2) but it cannot be perivascular.\nThe possibility that there can be a small but significant net perivascular outflow from the parenchyma of Na+, Cl− and accompanying water may be the resolution of a long-standing difficulty. In non-communicating hydrocephalus, CSF production by the choroid plexuses continues at a nearly normal rate, but the normal route for CSF outflow from the IIIrd ventricle is blocked. Because after an initial period the ventricles do not continue to enlarge at a rate sufficient to accommodate the CSF production, CSF must be escaping via an alternative route (see Sections 4.2.2.1–4.2.2.2 in [41] and Section 4.1 in [4] for discussion and references). The periventricular parenchyma is oedematous which may allow flow of fluid from the ventricles, but the oedema only extends a small distance. In cats with kaolin induced hydrocephalus, Sahar et al. [421] observed penetration of serum albumin only up to about 2.5 mm which they took to mean that the albumin was being absorbed into the blood. There is no known mechanism by which this absorption could have occurred. It would be very interesting to know whether this distance corresponds instead to the distance from the ventricular surface to perivascular pathways that would allow sufficiently rapid removal of albumin to CSF in the subarachnoid spaces and/or to lymph that the concentrations observed deeper in the parenchyma would be small. The importance of fluid escape from the ventricles across the ependyma into the parenchyma in hydrocephalus has recently been given further support by observations of gadobutrol movements in normal pressure hydrocephalus in humans [15]."}