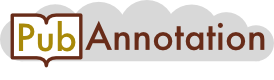
PMC:6085830 / 6892-27440
Annnotations
{"target":"http://pubannotation.org/docs/sourcedb/PMC/sourceid/6085830","sourcedb":"PMC","sourceid":"6085830","source_url":"https://www.ncbi.nlm.nih.gov/pmc/6085830","text":"RESULTS\n\nThe FUS prionlike domain is phosphorylated at numerous serines and threonines following calicheamicin treatment\nDeng and coworkers found several pharmacological agents caused a characteristic increase in the apparent molecular weight of the FUS protein band in Western blots (Deng et al., 2014): staurosporine (kinase inhibitor), calicheamicin (DNA damaging agent), and calyculin-A (phosphatase inhibitor). When they treated H4 neuroglioma cells with staurosporine, it yielded a high-molecular-weight FUS species that on phosphatase treatment returned to the untreated size, suggesting that the gel shift was due to phosphorylation. We reproduced their results showing an increase in FUS’s apparent molecular weight in multiple human cell lines treated with staurosporine, calicheamicin, and calyculin-A (Figure 1A and Supplemental Figure S1A). Likewise, when we subjected immunoprecipitated FUS from calicheamicin and calyculin-A–treated cells to phosphatase before Western blotting, the FUS bands returned to their original, untreated position (Figure 1B). Additionally, we expressed FUS in H4 cells with various numbers of phosphomimetic substitutions (replacing S or T with E; see Materials and Methods for specific sites) to simulate varying amounts of phosphorylation in the PrLD. The mimetic FUS Western blot bands migrated at increasingly higher apparent molecular weights (Figure 1C) with the number of phosphomimetic substitutions correlated to the increase in band shift. A stepwise change became prominent above the unmodified FUS band with ∼4 substitutions. The phosphomimetic with 12 substitutions (12E) migrated most similarly to FUS from staurosporine-, calicheamicin-, or calyculin-A–treated cells. From this, we concluded the large increase in apparent molecular weight in Western blots was consistent with multiple phosphorylation of FUS’s PrLD.\nFIGURE 1: The prionlike domain of cellular FUS contains numerous phosphorylation sites. (A) Lysates from multiple human cell lines treated with calicheamicin or calyculin-A showed FUS migrating with a larger apparent molecular weight in Western blot; HEK293T, n = 5; H4, n = 4; U-2 OS, n = 2. (B) Treatment of immunoprecipitated FUS with phosphatase caused FUS to return to its normal apparent size; *Heavy chain of the immunoglobulin G used for immunoprecipitation; n = 2. (C) Substituting glutamate at potential serine or threonine phosphorylation sites caused ectopic FUS in H4 cells to migrate similarly to endogenous FUS from cells treated with calicheamicin or calyculin-A; see Materials and Methods for exact substitution sites; n = 2. (D) Twenty-eight serines and threonines have been identified in this and other studies as putative sites of phosphorylation (bold and underlined; DNA-PK consensus sites are shown in red). Here sites were identified by mass spectrometry following immunoprecipitation of FUS from lysates of human cell lines treated with the DNA-damaging agents calicheamicin or camptothecin, or the phosphatase-inhibitor calyculin-A. Our previous work with recombinant DNA-PK indicated that all 12 S/TQ DNA-PK consensus motifs in the PrLD could be phosphorylated in vitro (Monahan et al., 2017). We preliminarily mapped three of these sites using mass spectrometry analysis of immunoprecipitated FUS from HEK293T cells treated with calyculin-A: Ser-26, Ser-30, and Ser-61. Here, using refinements of our previous proteomic approach to identify PTMs, we focused our attention on the DNA-damaging agents calicheamicin and camptothecin, due to the previously implicated link between DNA damage and FUS phosphorylation. Numerous unambiguous S and T sites were identified (Supplemental Figure S2A). Several ion fragments appeared to contain two phosphates, albeit with less certainty (Supplemental Figure S2B). Site combinations within individual peptides were as follows: Ser-26/Ser-30, Ser-30/Ser-42, Thr-109/Ser-115, and Ser-115/Ser-117. In total, we found seven DNA-PK consensus sites in the PrLD to be phosphorylated following DNA damage with Ser-26 and Ser-30 being the most prominent across multiple experiments (Figure 1D). In general, the PrLD appears to be highly susceptible to multiphosphorylation in cells with as many as 28 candidate sites found by us and others (Figure 1D).\n\nSer-26 and -30 in the FUS prionlike domain are phosphorylated following DNA-damaging stress\nOf the three drugs identified by Deng and colleagues to cause FUS phosphorylation, only calicheamicin has been established to directly cause DNA damage (Zein et al., 1988; Deng et al., 2014). Staurosporine is a promiscuous kinase inhibitor (Karaman et al., 2008), and calyculin-A is an inhibitor of protein phosphatases 1/2A (Ishihara et al., 1989). When HEK293T or H4 cells were treated with several DNA-damaging agents (e.g., etoposide, doxorubicin, camptothecin) or okadaic acid (phosphatase inhibitor similar to calyculin-A), we saw no characteristic band shift in FUS (Figure 2A). Phosphorylated ATM was used to confirm these treatments caused a DNA-damage response (Figure 2A). Even when dosage or treatments were extended and Western blot exposures were increased, there were no definitive FUS band shifts observed, although band shadowing suggested some FUS might be running at a slightly higher molecular weight (Supplemental Figure S1B).\nFIGURE 2: Custom anti-FUS(pSer26) and anti-FUS(pSer30) antibodies are specific to phosphorylated FUS. (A, B) Lysates of HEK293T cells treated with various reagents (DMSO, 50 nM calicheamicin, 100 nM calyculin-A, 200 μM etoposide, 10 μM camptothecin, 2 μM doxorubicin, or 100 nM okadaic acid) for 3 h at 37°C were analyzed by Western blotting with commercial FUS or with anti-FUS(pSer26) or anti-FUS(pSer30) antibodies, respectively; n = 2. (C) Phosphorylated and unphosphorylated synthetic peptides—corresponding to regions within FUS’s prionlike domain—were serially diluted, spotted on nitrocellulose, and immunoprobed with custom antibodies: anti-FUS(pSer26) or anti-FUS(pSer30); n = 2. (D) FUS was knocked down using siRNA in H4 cells then treated with calicheamicin (or DMSO; negative control) to induce phosphorylation. Western blots using anti-FUS(pSer26), anti-FUS(pSer30), and commercial FUS antibodies revealed specificity of the phosphoantibodies to the FUS protein; n = 4. (E) Densitometry analysis of the percentage of signal reduction with FUS knockdown compared with the control; error bars represent 95% confidence intervals. We concluded that calicheamicin, calyculin-A, and staurosporine were extreme in their effects on total cellular FUS and hypothesized that the other drugs might still cause FUS PrLD phosphorylation, but in a small subpopulation of FUS, or at lower frequencies on individual proteins, not revealed by a discernible band shift (Figure 2B). For these reasons, we generated polyclonal antibodies against FUS PrLD peptides encompassing phosphorylated Ser-26 or Ser-30 (Figure 2C). These peptides were chosen because phospho-Ser-26 and phospho-Ser-30 were repeatedly identified by our mass spectrometry experiments. Immunoblotting of phosphorylated and unphosphorylated synthetic peptides with the anti-FUS(pSer26) and anti-FUS(pSer30) antibodies indicated their specificity (Figure 2C). In Western blots with calyculin-A– or calicheamicin-treated HEK293T cell lysates, the antibodies recognized a protein species at the same position as commercial FUS antibody (Figure 2B). The phospho-specific antibodies did not recognize species from untreated controls. In all Western blots, there was a direct relationship between FUS protein band shift and phospho-specific antibody recognition (Figures 2 and 3 and Supplemental Figure S1, A, C, D, and F). Small interfering RNA (siRNA) knockdown was performed to ensure the custom antibodies were specific to FUS (Figure 2, D and E, and Supplemental Figure S3).\nBecause FUS-linked pathology presents in neurons, we chose to continue all experiments in the H4 cell type as it is neuronal in origin. Administering a dose series of calicheamicin to H4 cells revealed that at lower concentrations anti-FUS(pSer26) and anti-FUS(pSer30) recognize a subpopulation of FUS prior to an increase in apparent molecular weight by Western blot (Figure 3A). This confirmed that phosphorylation could occur at lower levels without an obvious band shift. We next looked specifically for low levels of phosphorylation after treating H4 cells with etoposide, camptothecin, doxorubicin, bleomycin, UV radiation, and ionizing radiation (IR). These treatments are known to induce DNA damage through different mechanisms (Povirk, 1996; Liu et al., 2000; Rastogi et al., 2010; Yang et al., 2014a; Montecucco et al., 2015; Xu and Her, 2015; Mavragani et al., 2017). However, each results in the production of double-strand DNA breaks that induce the DNA damage response pathway involving both ATM and DNA-PK (Shrivastav et al., 2008). All treatments caused FUS phosphorylation without a pronounced concomitant band shift (Figure 3, B and C; and in HEK293T cells shown in Supplemental Figure S1, C, D, and F). Of note, low concentrations of calyculin-A (5 vs. 100 nM) and UV radiation reproducibly caused observable phosphorylation at Ser-30 but not Ser-26. In control experiments with synthetic peptides, anti-FUS(pSer26) appeared more sensitive in epitope recognition than anti-FUS(pSer30), and its specificity was not diminished by diphosphorylation at both positions 26 and 30 (Figure 2C and Supplemental Figure S1E). For these reasons, we concluded differential phosphorylation is occurring in cells and is not a probing artifact.\nFIGURE 3: DNA-damaging conditions result in phosphorylation of Ser-26 and -30 of FUS. (A) Lysates of H4 cells treated with increasing amounts of calicheamicin were analyzed by Western blotting with commercial FUS and anti-FUS(pSer26) or anti-FUS(pSer30) antibodies; n = 2. (B, C) Lysates of H4 cells subjected to DNA-damaging conditions (0.5 nM calicheamicin, 5 nM calyculin-A, 200 μM etoposide, 10 μM camptothecin, 2 μM doxorubicin, 100 nM BLM, 90 mJ UV, or 20 Gy IR) were analyzed by Western blotting with commercial FUS and anti-FUS(pSer26) or anti-FUS(pSer30) antibodies; n = 3 for each treatment except IR in which n = 2. (D) Lysates of H4 cells treated with and without DNA-PK inhibitor (NU7441) prior to calicheamicin treatment were analyzed by Western blotting with commercial FUS and anti-FUS(pSer26) or anti-FUS(pSer30) antibodies; n = 3. As mentioned above, DNA-PK was previously implicated in the phosphorylation of FUS following DNA-damaging stress (Deng et al., 2014). When we treated cells with the DNA-PK inhibitor NU7441 followed by calicheamicin, the FUS band shift was completely eliminated, and the phospho-specific antibodies no longer recognized any FUS species (Figure 3D). This corroborates DNA-PK’s involvement in FUS PrLD phosphorylation.\n\nFUS remains nuclear following PrLD phosphorylation induced by DNA damage\nIt was previously suggested phosphorylation of the FUS PrLD following a DNA damage response could potentiate pathological aggregation by promoting FUS’s cytoplasmic localization (Deng et al., 2014). Alternatively, in previous work, we found phosphorylation of the PrLD might be protective since it disfavors LLPS and aggregation (Monahan et al., 2017). We used phospho-specific and non–phospho-specific FUS antibodies to evaluate the relationship between DNA damaging stress, FUS phosphorylation, and FUS localization.\nImmunofluorescence microscopy of H4 cells revealed total cellular FUS remained unchanged in its nuclear localization following treatment with the DNA-damaging agents calicheamicin, camptothecin, and etoposide (Figure 4A). Treatment caused nuclear phospho-FUS (at Ser-30) to appear within ∼15 min and persist in the nucleus for over an hour (Figure 4A). The same pattern was observed in U-2 OS and HEK293T cells (Supplemental Figure S4, A and B). To determine whether the extent of phosphorylation could alter FUS localization, we used low and high calicheamicin concentrations to cause lower and higher levels of phosphorylation, respectively. With both treatments, FUS remained nuclear like untreated controls (Figure 4A). Localization experiments were performed using anti-FUS(pSer30) because it revealed less nonspecific binding in siRNA immunofluorescence microscopy experiments (Figure 4, B and C).\nFIGURE 4: FUS remains nuclear following DNA-PK–dependent phosphorylation at Ser-30. (A) H4 cells were fixed for immunocytochemical analysis using commercial FUS and anti-FUS(pSer30) antibodies following treatment with DNA-damaging agents (DMSO, 50 or 5 nM calicheamicin, 60 μM camptothecin, 100 μM etoposide); quantification of nuclear and cytoplasmic fluorescence was used to determine the percentage of total FUS in the nucleus per cell; error bars represent 95% confidence intervals; the anti-FUS(pSer30) signal quantified in DMSO-treated cells resulting in ∼20% nuclear localization is due to background fluorescence; n = 3. (B, C) siRNA knockdown of FUS in H4 cells, using FUS-specific and scrambled-control nucleotides, showed a significant reduction in nuclear signal when cells were probed with both anti-FUS(pSer26) and anti-FUS(pSer30); error bars represent a 95% confidence interval; n = 3. Unlike the effects of the DNA-damaging agents, we observed calyculin-A and staurosporine to cause various levels of FUS phosphorylation and cytoplasmic localization (Supplemental Figure S4, A and C). However, both treatments dramatically altered cell morphology and caused adherent cells to disperse in culture. These effects were reproducible over multiple cell types and not observed with other treatments (Supplemental Figure S4, A and C). When we further evaluated FUS localization after staurosporine treatment, we observed phospho-FUS only in the nuclei and never in the cytoplasm (Supplemental Figure S4C).\nAs a positive control for FUS cytoplasmic localization, we subjected cells to osmotic stress, which was previously shown to cause an increase in cytoplasmic localization of FUS (Sama et al., 2013). Cytoplasmic localization was reported to depend on dimethylation of FUS arginine residues within its arginine-/glycine-rich domains. Using sorbitol (0.4 M), we confirmed osmotic stress causes FUS to rapidly localize to the cytoplasm (Supplmental Figure S5A). We also confirmed that FUS arginine dimethylation is required for cytoplasmic localization; when the methylase inhibitor adenosine-dialdehyde (Adox) was added, FUS remained nuclear following osmotic stress (Supplemental Figure S5, A and B). Theoretically, some of the DNA-damaging conditions we used could have simultaneously affected FUS arginine methylation, which would confound experiments that evaluate FUS localization. However, we found no evidence the experimental conditions that caused FUS phosphorylation were simultaneously affecting FUS’s arginine dimethylation (Supplemental Figure S5, C and D).\n\nDNA-PK–dependent phosphorylation of the PrLD does not promote FUS cytoplasmic localization\nWe asked whether PrLD multiphosphorylation has any effect on the change in localization of FUS induced by osmotic stress. H4 cells were treated with calicheamicin to induce phosphorylation and then subjected to osmotic stress with sorbitol. Using immunofluorescence microscopy, accumulation of total FUS in the cytoplasm appeared slightly inhibited in the calicheamicin-treated cells relative to control cells over time (Figure 5A). The FUS band shift in the Western blot suggested that FUS was multiphosphorylated under these conditions (Figure 5B). Next, using the phospho-specific anti-FUS(pSer30) antibody, the experiment was repeated with an extended end point. As before, the addition of calicheamicin prior to sorbitol resulted in a modest, but statistically significant, reduction of FUS in the cytoplasm (Figure 5, C and E). However, phosphorylated FUS was only observed in the nucleus of calicheamicin-treated cells—never in the cytoplasm. Likewise, we found that adding calicheamicin after sorbitol resulted in the rapid appearance of phosphorylated FUS only in the nucleus and not in the cytoplasm (Figure 5, C and E). This is consistent with DNA-PK’s nuclear localization and activity (Anderson and Lees-Miller, 1992). Western blot controls confirming FUS phosphorylation are shown in Figure 5D, and extended immunofluorescence control images are shown in Supplemental Figure S6. Similar nuclear-only localization of phospho-FUS was observed using the anti-FUS(pSer26) antibody (Supplemental Figure S7).\nFIGURE 5: Calicheamicin-induced phosphorylated FUS is maintained in the nucleus following osmotic stress. (A) H4 cells treated with 0.4 M sorbitol, with and without 50 nM calicheamicin pretreatment, were fixed for immunocytochemical analysis with a commercial FUS antibody; nuclear and cytoplasmic fluorescence signals at the 45-min time point were quantified to determine the percentage of total FUS in the cytoplasm per cell (right panel); error bars represent 95% confidence intervals; n = 3. (B) Lysates were analyzed by Western blotting; n = 2. (C) H4 cells were fixed for immunocytochemical analysis with commercial FUS and anti-FUS(pSer30) antibodies following sequential addition of sorbitol and/or calicheamicin (60 min sorbitol before 60 min calicheamicin, or 60 min calicheamcin before 60 min sorbitol, without removal of the first treatment); n = 3. (D) Lysates were analyzed by Western blotting; n = 3. (E) Quantification of nuclear and cytoplasmic fluorescence signals was done to determine the percentage of total FUS in the cytoplasm per cell and the percentage of total phospho-FUS in the nucleus per cell (N.D. = not determined); error bars represent 95% confidence intervals. We next asked how inhibition of DNA-PK could alter the balance between nuclear and cytoplasmic FUS. When H4 cells were treated with the DNA-PK inhibitor NU7441, we observed no effect on FUS localization. However, if cells were subjected to calicheamicin after treatment with the inhibitor, the majority of FUS localized to the cytoplasm (Figure 6, A and B). Figure 6C shows Western blots confirming the efficacy of calicheamicin and inhibitor. The data suggest that neither DNA-PK activity nor PrLD phosphorylation at positions 26 and 30 is sufficient to drive FUS cytoplasmic localization.\nFIGURE 6: Phosphorylation of the FUS prionlike domain is not linked to cytoplasmic localization. (A) H4 cells following calicheamicin treatment, with and without DNA-PK inhibitor (NU7441), were fixed for immunocytochemical analysis using commercial FUS and anti-FUS(pSer30) antibodies; n = 3. (B) Quantification of nuclear and cytoplasmic fluorescence signals was done to determine the percentage of cytoplasmic localization; error bars represent 95% confidence interval. (C) Lysates were analyzed by Western blotting; n = 3. (D) H4 cells expressing ectopic PM FUS (6 or 12 glutamate substitutions in the prionlike domain) were visualized by immunofluorescence microscopy; n = 3. (E) Quantification of nuclear and cytoplasmic fluorescence signal was done to determine the percentage of cytoplasmic localization of FUS-wt and FUS-12E from C; error bars represent 95% confidence intervals. (F) U-2 OS cells expressing GFP-fused ectopic phosphomimetic FUS were visualized by live-cell imaging; n = 2. Because the entire FUS PTM repertoire that follows calicheamicin treatment is unknown and is not necessarily limited to PrLD modifications, it is possible that PrLD phosphorylation favors cytoplasmic localization but is masked by other PTMs. We used H4 cells and a dual-expression plasmid system that expresses GFP and FUS phosphomimetic variants as separate proteins. The FUS variants were specifically modified at DNA-PK sites within the PrLD to determine their effects on FUS subcellular localization (phosphomimetics discussed in Figure 1C and Supplemental Figure S8; see Materials and Methods for sites). GFP fluorescence was used to determine which transfected cells expressed ectopic proteins at similar levels. The localization of ectopic FUS, FUS-6E, or FUS-12E was evaluated by immunofluorescence microscopy. We found that FUS, FUS-6E, and FUS-12E were all mostly nuclear (Figure 6D). Quantification yielded no significant differences in localization (Figure 6E). A similar experiment was performed in live U-2 OS cells expressing ectopic GFP-FUS fusion proteins. Again, no difference in localization was observed by introducing phosphomimetic substitutions (Figure 6F).\n","divisions":[{"label":"Title","span":{"begin":0,"end":7}},{"label":"Section","span":{"begin":9,"end":4286}},{"label":"Title","span":{"begin":9,"end":120}},{"label":"Figure caption","span":{"begin":1874,"end":3035}},{"label":"Section","span":{"begin":4288,"end":10884}},{"label":"Title","span":{"begin":4288,"end":4379}},{"label":"Figure caption","span":{"begin":5328,"end":6472}},{"label":"Figure caption","span":{"begin":9617,"end":10468}},{"label":"Section","span":{"begin":10886,"end":14967}},{"label":"Title","span":{"begin":10886,"end":10958}},{"label":"Figure caption","span":{"begin":12382,"end":13286}},{"label":"Section","span":{"begin":14969,"end":20547}},{"label":"Title","span":{"begin":14969,"end":15059}},{"label":"Figure caption","span":{"begin":16577,"end":17774}},{"label":"Figure caption","span":{"begin":18366,"end":19366}}],"tracks":[{"project":"2_test","denotations":[{"id":"29897835-24899704-70504538","span":{"begin":298,"end":302},"obj":"24899704"},{"id":"29897835-28790177-70504539","span":{"begin":3191,"end":3195},"obj":"28790177"},{"id":"29897835-24899704-70504540","span":{"begin":4565,"end":4569},"obj":"24899704"},{"id":"29897835-18183025-70504541","span":{"begin":4637,"end":4641},"obj":"18183025"},{"id":"29897835-2539153-70504542","span":{"begin":4723,"end":4727},"obj":"2539153"},{"id":"29897835-21209706-70504543","span":{"begin":8654,"end":8658},"obj":"21209706"},{"id":"29897835-26600742-70504544","span":{"begin":8699,"end":8703},"obj":"26600742"},{"id":"29897835-26287259-70504545","span":{"begin":8717,"end":8721},"obj":"26287259"},{"id":"29897835-28718816-70504546","span":{"begin":8741,"end":8745},"obj":"28718816"},{"id":"29897835-18157161-70504547","span":{"begin":8910,"end":8914},"obj":"18157161"},{"id":"29897835-24899704-70504548","span":{"begin":10596,"end":10600},"obj":"24899704"},{"id":"29897835-24899704-70504549","span":{"begin":11151,"end":11155},"obj":"24899704"},{"id":"29897835-28790177-70504550","span":{"begin":11305,"end":11309},"obj":"28790177"},{"id":"29897835-23625794-70504551","span":{"begin":14092,"end":14096},"obj":"23625794"},{"id":"29897835-1486241-70504552","span":{"begin":16285,"end":16289},"obj":"1486241"}],"attributes":[{"subj":"29897835-24899704-70504538","pred":"source","obj":"2_test"},{"subj":"29897835-28790177-70504539","pred":"source","obj":"2_test"},{"subj":"29897835-24899704-70504540","pred":"source","obj":"2_test"},{"subj":"29897835-18183025-70504541","pred":"source","obj":"2_test"},{"subj":"29897835-2539153-70504542","pred":"source","obj":"2_test"},{"subj":"29897835-21209706-70504543","pred":"source","obj":"2_test"},{"subj":"29897835-26600742-70504544","pred":"source","obj":"2_test"},{"subj":"29897835-26287259-70504545","pred":"source","obj":"2_test"},{"subj":"29897835-28718816-70504546","pred":"source","obj":"2_test"},{"subj":"29897835-18157161-70504547","pred":"source","obj":"2_test"},{"subj":"29897835-24899704-70504548","pred":"source","obj":"2_test"},{"subj":"29897835-24899704-70504549","pred":"source","obj":"2_test"},{"subj":"29897835-28790177-70504550","pred":"source","obj":"2_test"},{"subj":"29897835-23625794-70504551","pred":"source","obj":"2_test"},{"subj":"29897835-1486241-70504552","pred":"source","obj":"2_test"}]}],"config":{"attribute types":[{"pred":"source","value type":"selection","values":[{"id":"2_test","color":"#93ece7","default":true}]}]}}