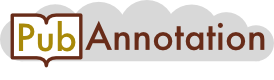
PMC:6085830 / 27441-35897
Annnotations
2_test
{"project":"2_test","denotations":[{"id":"29897835-21847013-70504553","span":{"begin":1355,"end":1359},"obj":"21847013"},{"id":"29897835-21452073-70504554","span":{"begin":1502,"end":1506},"obj":"21452073"},{"id":"29897835-26455390-70504555","span":{"begin":1575,"end":1579},"obj":"26455390"},{"id":"29897835-28942918-70504556","span":{"begin":2054,"end":2058},"obj":"28942918"},{"id":"29897835-17170131-70504557","span":{"begin":2191,"end":2195},"obj":"17170131"},{"id":"29897835-28790177-70504558","span":{"begin":2722,"end":2726},"obj":"28790177"},{"id":"29897835-26455390-70504559","span":{"begin":3115,"end":3119},"obj":"26455390"},{"id":"29897835-28942918-70504560","span":{"begin":3316,"end":3320},"obj":"28942918"},{"id":"29897835-28790177-70504561","span":{"begin":3632,"end":3636},"obj":"28790177"},{"id":"29897835-28924037-70504562","span":{"begin":3957,"end":3961},"obj":"28924037"},{"id":"29897835-24899704-70504563","span":{"begin":4238,"end":4242},"obj":"24899704"},{"id":"29897835-11313863-70504564","span":{"begin":4650,"end":4654},"obj":"11313863"},{"id":"29897835-26506362-70504565","span":{"begin":4671,"end":4675},"obj":"26506362"},{"id":"29897835-22968170-70504566","span":{"begin":5270,"end":5274},"obj":"22968170"},{"id":"29897835-23625794-70504567","span":{"begin":5289,"end":5293},"obj":"23625794"},{"id":"29897835-23620769-70504568","span":{"begin":5316,"end":5320},"obj":"23620769"},{"id":"29897835-12964758-70504569","span":{"begin":8045,"end":8049},"obj":"12964758"}],"text":"DISCUSSION\nThe results of our mass spectrometry experiments suggest that extensive FUS PrLD phosphorylation can occur in cells: Twenty-eight putative sites identified to date, which represents ∼17% of the approximately 160-amino-acid domain. In combination with the characteristic molecular weight shift of the FUS protein band in Western blots, it can be inferred that multiphosphorylation of the PrLD is occurring under certain cellular conditions. Here we confirmed phosphorylation of two of the predicted DNA-PK consensus sites (Ser-26 and Ser-30) within the PrLD using human cell lines under DNA-damaging stress. Previously, Gardiner et al. (2008) identified Ser-42 phosphorylation under similar conditions; thus, three of the 12 DNA-PK motifs in the PrLD have been confirmed as sites of phosphorylation in cells. This suggests many of the other predicted sites may also be phosphorylated in cells.\nIf a moderate fraction of the predicted phosphorylation sites within the FUS PrLD are phosphorylated, then the PrLD will fail—by definition—to preserve its prionlike character. Canonical prion domains are inherently low-complexity, consisting of mostly nonaliphatic and noncharged amino acids. All PrLD’s share these compositional features. Moreover, they are frequently found in proteins that pathologically aggregate in neurons (Gitler and Shorter, 2011) or undergo LLPS. The FUS PrLD contributes to both phenomena: it strongly enhances FUS’s aggregation propensity in cells (Kryndushkin et al., 2011) and it is critical to FUS’s ability to undergo LLPS (Burke et al., 2015). Both phenomena depend on protein self-association and, in this regard, are reminiscent of yeast prion behavior. Since FUS’s PrLD is considered integral to both LLPS and solid aggregate formation, the decrease in prionlike character through multiphosphorylation may have dramatic effects on FUS’s cellular behavior.\nMurray and coworkers discovered, in vitro, that FUS PrLD forms archetypical amyloid fibers with parallel in-register cross-beta architecture (Murray et al., 2017). This type of structure explains how aggregated proteins can pathologically propagate similarly to yeast prions (Shewmaker et al., 2006). Eleven of the identified putative phosphorylation sites are located within the core of Murray’s predicted FUS amyloid fibers (amino acids 39–95). Because the individual proteins align in-register to form the amyloid core, the introduction of multiple charges via phosphates would be structurally unfavorable due to electrostatic repulsion. Consistent with this idea, we previously found dramatic qualitative effects on FUS aggregation and toxicity in cell models with ∼4–6 PrLD phosphomimetic substitutions (Monahan et al., 2017). Based on our observations here, manipulation of cellular phosphatases and kinases could provide a realistic mechanism to combat FUS aggregation through multiphosphorylation.\nMultiphosphorylation may also regulate FUS incorporation into phase-separated ribonucleoprotein granules in cells. Recombinant FUS PrLD remains structurally disordered in the phase-separated state (Burke et al., 2015). However, the cross-beta in-register interactions found in the solid state were hypothesized to be similar to the dynamic interactions that may occur in the phase-separated state (Murray et al., 2017). In this scenario, multiphosphorylation may hinder LLPS similarly to how it disfavors solid aggregate formation: primarily through electrostatic repulsion. We previously observed dramatic qualitative effects on LLPS in vitro with six phosphomimetic substitutions, though fewer were not tested (Monahan et al., 2017). In agreement with our findings, Lin and coworkers modeled phase separation using an experimental system with SH3 domain protein. When the FUS PrLD was fused to SH3, the system underwent LLPS at lower concentration. However, DNA-PK treatment (i.e., phosphorylation) resulted in dissolution of the droplets (Lin et al., 2017). These results suggest a mechanism by which FUS function, interactions, and localization could be regulated rapidly under physiologic and stress conditions.\nPreviously, DNA-PK–dependent phosphorylation of the PrLD was suggested to facilitate FUS nuclear export (Deng et al., 2014). This was a significant finding because cytoplasmic accumulation may potentiate pathology. However, this observed cytoplasmic localization could be due to particularities of the drugs used in the study: calyculin-A and staurosporine. Calyculin-A is a phosphatase-inhibitor and staurosporine is a kinase inhibitor. Neither are specifically DNA-damaging agents and both induce apoptosis (Stepczynska et al., 2001; Kleppe et al., 2015), so for these reasons we did not further pursue their effects here. However, since both drugs were previously used to characterize FUS localization, they could have influenced conclusions. Understandably, we and others use these potent drugs because they easily enable the study of FUS PrLD phosphorylation, but their full individual effects on cellular mechanisms and other FUS domains may yield different experimental results.\nUnder osmotic stress conditions, we recapitulated previous observations that arginine dimethylation is critical to cytoplasmic localization of FUS (Dormann et al., 2012; Sama et al., 2013; Scaramuzzino et al., 2013). We determined the treatments that induce FUS phosphorylation do not appear to impact arginine dimethylation, which is a conceivable mechanism by which they could indirectly govern nucleocytoplasmic localization. To determine whether PrLD phosphorylation alone could cause cytoplasmic localization, we examined the localization of ectopically expressed phosphomimetic FUS. Assuming the S/T-to-E substitutions mimic the effects of phosphorylation, we concluded that phosphorylation of DNA-PK consensus sites in the PrLD is not sufficient to cause FUS cytoplasmic localization. Thus, FUS’s arginine dimethylation status is directly linked to its subcellular localization, while no similar link currently exists for FUS PrLD phosphorylation.\nDNA-PK is activated by double-strand DNA breaks. When cells are treated with calicheamicin, the DNA-PK–dependent phosphorylation of FUS is robust. However, extensive phosphorylation (or phosphomimetic substitution) of the PrLD does not change FUS’s subcellular localization. Retention of FUS in the nucleus following DNA damage would be consistent with FUS having a critical nuclear activity. Thus, DNA-PK–dependent FUS phosphorylation following DNA damage, corroborates FUS’s proposed function in the DNA damage response pathway. This is supported by the repertoire of DNA damaging treatments we confirmed to induce low levels of phosphorylation and still maintain nuclear localization. The change in localization that occurs when DNA-PK is inhibited prior to calicheamicin treatment suggests PrLD phosphorylation may be causing a retention of FUS to the nucleus. Validation of this prediction and elucidation of the mechanism by which this may be occurring is undetermined and necessitates further research.\nThe generation of phospho-specific FUS antibodies confirmed Ser-26 and Ser-30 phosphorylation following DNA damaging stress but generally only in a small percentage of total cellular FUS. Since FUS is implicated in many diverse functions, the entire cellular population may not require identical PTMs, which is consistent with our observation that subpopulations of FUS show differential levels of phosphorylation. For example, we observed situations in which Ser-30 phosphorylation appeared in the absence of Ser-26 phosphorylation. This was especially pronounced with UV radiation. For comparison, Gardiner et al. found phosphorylation of Ser-42 was noticeably stronger following IR stress compared with other DNA-damaging conditions (Gardiner et al., 2008). Thus, different responses and activities may require a specific pattern of PTMs. Likewise, 20 or more arginine residues of FUS are proposed to be mono- or dimethylated under various conditions (Rappsilber et al., 2003); how precisely this governs specific subcellular localization remains unknown, but a huge mathematical combination of potential PTMs exists. We observed rapid relocalization of FUS after calicheamicin treatment when DNA-PK was inhibited, despite no evidence of PrLD phosphorylation. Again, specific PTMs beyond DNA-PK phosphorylation may underlie this localization response and will require further study."}