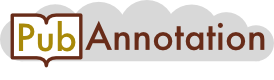
PMC:5767236 / 53175-77959
Annnotations
MyTest
{"project":"MyTest","denotations":[{"id":"29375564-15514167-34933940","span":{"begin":849,"end":852},"obj":"15514167"},{"id":"29375564-18566299-34933941","span":{"begin":854,"end":857},"obj":"18566299"},{"id":"29375564-15514167-34933942","span":{"begin":1032,"end":1035},"obj":"15514167"},{"id":"29375564-19041276-34933943","span":{"begin":1037,"end":1040},"obj":"19041276"},{"id":"29375564-18772495-34933944","span":{"begin":1284,"end":1287},"obj":"18772495"},{"id":"29375564-18772495-34933945","span":{"begin":1436,"end":1439},"obj":"18772495"},{"id":"29375564-1556191-34933946","span":{"begin":1527,"end":1530},"obj":"1556191"},{"id":"29375564-19041276-34933947","span":{"begin":1689,"end":1692},"obj":"19041276"},{"id":"29375564-20675591-34933948","span":{"begin":1694,"end":1697},"obj":"20675591"},{"id":"29375564-10845873-34933949","span":{"begin":1792,"end":1795},"obj":"10845873"},{"id":"29375564-8486772-34933950","span":{"begin":1797,"end":1800},"obj":"8486772"},{"id":"29375564-10845873-34933951","span":{"begin":1832,"end":1835},"obj":"10845873"},{"id":"29375564-20675591-34933952","span":{"begin":2062,"end":2065},"obj":"20675591"},{"id":"29375564-18487233-34933953","span":{"begin":2165,"end":2168},"obj":"18487233"},{"id":"29375564-10969071-34933954","span":{"begin":2423,"end":2426},"obj":"10969071"},{"id":"29375564-12777468-34933955","span":{"begin":2594,"end":2597},"obj":"12777468"},{"id":"29375564-19032770-34933956","span":{"begin":2636,"end":2639},"obj":"19032770"},{"id":"29375564-19189937-34933957","span":{"begin":2727,"end":2730},"obj":"19189937"},{"id":"29375564-16492740-34933958","span":{"begin":2928,"end":2931},"obj":"16492740"},{"id":"29375564-19913791-34933959","span":{"begin":2933,"end":2936},"obj":"19913791"},{"id":"29375564-18487233-34933960","span":{"begin":3020,"end":3023},"obj":"18487233"},{"id":"29375564-10625669-34933961","span":{"begin":3178,"end":3181},"obj":"10625669"},{"id":"29375564-10749696-34933962","span":{"begin":3183,"end":3186},"obj":"10749696"},{"id":"29375564-17056295-34933963","span":{"begin":3329,"end":3332},"obj":"17056295"},{"id":"29375564-11352993-34933964","span":{"begin":3438,"end":3441},"obj":"11352993"},{"id":"29375564-11742878-34933965","span":{"begin":3460,"end":3463},"obj":"11742878"},{"id":"29375564-15755745-34933966","span":{"begin":3709,"end":3712},"obj":"15755745"},{"id":"29375564-16601234-34933967","span":{"begin":3752,"end":3755},"obj":"16601234"},{"id":"29375564-20007839-34933968","span":{"begin":3905,"end":3908},"obj":"20007839"},{"id":"29375564-16336952-34933969","span":{"begin":4429,"end":4432},"obj":"16336952"},{"id":"29375564-17689273-34933970","span":{"begin":4519,"end":4522},"obj":"17689273"},{"id":"29375564-19776020-34933971","span":{"begin":4795,"end":4798},"obj":"19776020"},{"id":"29375564-20354139-34933972","span":{"begin":4800,"end":4803},"obj":"20354139"},{"id":"29375564-19234137-34933973","span":{"begin":5370,"end":5373},"obj":"19234137"},{"id":"29375564-22777293-34933974","span":{"begin":5375,"end":5378},"obj":"22777293"},{"id":"29375564-20651288-34933975","span":{"begin":5799,"end":5802},"obj":"20651288"},{"id":"29375564-25828120-34933976","span":{"begin":6107,"end":6110},"obj":"25828120"},{"id":"29375564-19910578-34933977","span":{"begin":6112,"end":6115},"obj":"19910578"},{"id":"29375564-25828120-34933978","span":{"begin":6425,"end":6428},"obj":"25828120"},{"id":"29375564-22161142-34933979","span":{"begin":6577,"end":6580},"obj":"22161142"},{"id":"29375564-25261478-34933980","span":{"begin":7339,"end":7342},"obj":"25261478"},{"id":"29375564-23596310-34933981","span":{"begin":7581,"end":7584},"obj":"23596310"},{"id":"29375564-22942426-34933982","span":{"begin":7874,"end":7877},"obj":"22942426"},{"id":"29375564-22161142-34933983","span":{"begin":8759,"end":8762},"obj":"22161142"},{"id":"29375564-24935258-34933984","span":{"begin":9324,"end":9327},"obj":"24935258"},{"id":"29375564-25261478-34933985","span":{"begin":9565,"end":9568},"obj":"25261478"},{"id":"29375564-22302786-34933986","span":{"begin":10092,"end":10095},"obj":"22302786"},{"id":"29375564-20007582-34933986","span":{"begin":10092,"end":10095},"obj":"20007582"},{"id":"29375564-20368519-34933986","span":{"begin":10092,"end":10095},"obj":"20368519"},{"id":"29375564-23601906-34933987","span":{"begin":11049,"end":11051},"obj":"23601906"},{"id":"29375564-20068232-34933988","span":{"begin":11297,"end":11300},"obj":"20068232"},{"id":"29375564-18302942-34933989","span":{"begin":11658,"end":11661},"obj":"18302942"},{"id":"29375564-28258700-34933990","span":{"begin":11725,"end":11728},"obj":"28258700"},{"id":"29375564-27665459-34933991","span":{"begin":11758,"end":11761},"obj":"27665459"},{"id":"29375564-22514659-34933992","span":{"begin":12691,"end":12694},"obj":"22514659"},{"id":"29375564-23720442-34933993","span":{"begin":13279,"end":13282},"obj":"23720442"},{"id":"29375564-17056121-34933994","span":{"begin":13446,"end":13449},"obj":"17056121"},{"id":"29375564-9698510-34933995","span":{"begin":13607,"end":13610},"obj":"9698510"},{"id":"29375564-15358667-34933996","span":{"begin":13999,"end":14002},"obj":"15358667"},{"id":"29375564-25104800-34933997","span":{"begin":14313,"end":14316},"obj":"25104800"},{"id":"29375564-28377486-34933998","span":{"begin":14760,"end":14763},"obj":"28377486"},{"id":"29375564-20067761-34933999","span":{"begin":15358,"end":15361},"obj":"20067761"},{"id":"29375564-20668222-34934000","span":{"begin":15513,"end":15516},"obj":"20668222"},{"id":"29375564-17360992-34934001","span":{"begin":15948,"end":15951},"obj":"17360992"},{"id":"29375564-23535595-34934002","span":{"begin":17384,"end":17387},"obj":"23535595"},{"id":"29375564-16814729-34934003","span":{"begin":17518,"end":17521},"obj":"16814729"},{"id":"29375564-27667687-34934004","span":{"begin":17782,"end":17785},"obj":"27667687"},{"id":"29375564-26926996-34934005","span":{"begin":18065,"end":18068},"obj":"26926996"},{"id":"29375564-27926861-34934006","span":{"begin":18282,"end":18285},"obj":"27926861"},{"id":"29375564-24315620-34934007","span":{"begin":18349,"end":18352},"obj":"24315620"},{"id":"29375564-17592090-34934008","span":{"begin":18675,"end":18678},"obj":"17592090"},{"id":"29375564-25779542-34934009","span":{"begin":19041,"end":19044},"obj":"25779542"},{"id":"29375564-25814686-34934009","span":{"begin":19041,"end":19044},"obj":"25814686"},{"id":"29375564-27340270-34934009","span":{"begin":19041,"end":19044},"obj":"27340270"},{"id":"29375564-21527743-34934010","span":{"begin":19359,"end":19362},"obj":"21527743"},{"id":"29375564-19200056-34934010","span":{"begin":19359,"end":19362},"obj":"19200056"},{"id":"29375564-14670831-34934010","span":{"begin":19359,"end":19362},"obj":"14670831"},{"id":"29375564-20703492-34934011","span":{"begin":20397,"end":20400},"obj":"20703492"},{"id":"29375564-20703492-34934012","span":{"begin":20580,"end":20583},"obj":"20703492"},{"id":"29375564-24485636-34934013","span":{"begin":20585,"end":20588},"obj":"24485636"},{"id":"29375564-17478729-34934014","span":{"begin":20771,"end":20774},"obj":"17478729"},{"id":"29375564-22842069-34934015","span":{"begin":20947,"end":20950},"obj":"22842069"},{"id":"29375564-21070867-34934016","span":{"begin":21108,"end":21111},"obj":"21070867"},{"id":"29375564-12086926-34934017","span":{"begin":21318,"end":21321},"obj":"12086926"},{"id":"29375564-27791119-34934018","span":{"begin":21698,"end":21701},"obj":"27791119"},{"id":"29375564-17307368-34934019","span":{"begin":23131,"end":23134},"obj":"17307368"},{"id":"29375564-16612592-34934020","span":{"begin":23136,"end":23139},"obj":"16612592"},{"id":"29375564-12788379-34934021","span":{"begin":23280,"end":23283},"obj":"12788379"},{"id":"29375564-28087471-34934022","span":{"begin":23470,"end":23472},"obj":"28087471"},{"id":"29375564-26294848-34934023","span":{"begin":24660,"end":24663},"obj":"26294848"}],"namespaces":[{"prefix":"_base","uri":"https://www.uniprot.org/uniprot/testbase"},{"prefix":"UniProtKB","uri":"https://www.uniprot.org/uniprot/"},{"prefix":"uniprot","uri":"https://www.uniprot.org/uniprotkb/"}],"text":"Signaling Pathways and Molecules Modulating Cardiovascular Immune Response\n\nCytokines and Chemokines\nMacrophages are major contributors to the inflammatory response in the setting of cardiovascular diseases. They secrete diverse pro-inflammatory mediators (including cytokines, chemokines, ROS and nitrogen species, among others), and MMPs and they eventually die by necrosis or apoptosis. Dying macrophages release their lipid contents, which lead to the development of a pro-thrombotic necrotic core. The necrotic core is, in turn, a clue component of unstable plaques and contributes to their rupture and the consequent intravascular blood clot that causes MI and stroke. The main effect of cytokines/chemokines released by macrophages is the recruitment of different innate and adaptive immune cell populations into the atherosclerotic lesions (120, 121), thereby amplifying the inflammatory environment. For example, different inflammatory mediators such as IL-1, TNF and IFN-γ increase the expression of MCP-1 in macrophages (120, 122). Also, under inflammatory conditions macrophages produce different enzymes such as MMPs, cathepsins and serine proteases that degrade the extracellular matrix of the atherosclerotic plaque contributing to the pathogenesis of atherosclerosis (123). In this sense, IL-1 and TNF increase the expression of numerous MMPs, such as MMP-9, and their activity contribute to unstable plaque morphology (123).\nWhile initial reports indicated that IFN-γ prevented macrophage foam cell formation (124), subsequent studies demonstrate that this cytokine promotes foam cell formation through diverse mechanisms: enhancing the uptake of oxLDL/AcLDL through SRs (122, 125), diminishing efflux of cholesterol via inhibition of ABCA-1 transporter and ApoE expression (126, 127) and increasing ACAT-1 levels (126) in macrophage-derived foam cells. For example, it was recently reported that the axis IFN-γ/STAT1 mediates the uptake of acLDL/oxLDL by human macrophages in an extracellular signal-regulated kinase (ERK)-dependent signaling (125). The activity of IFN-γ as a pro-foam cell mediator has been supported by several in vivo studies (128).\nSince IFN-γ was identified as a pro-foam cell cytokine, different authors evaluated the effect of other pro-inflammatory cytokines. Studies using a murine macrophage cell line have shown that TNF inhibits the expression of SRs and the uptake of oxLDL (129). Regarding additional mechanisms, more recent evidence has suggested that TNF promotes foam cell formation in vitro by reducing the mRNA expression of ABCA-1/ABCG-1 (130), and intracellular lipid catabolism (131), and by enhancing the expression of ACAT-1 and the accumulation of cholesteryl ester (132). However, the role for TNF remains controversial since other studies have shown that TNF enhances ABCA-1 expression and the efflux of cholesterol in peritoneal macrophages stimulated with ApoA–I (133, 134) and since evidences from studies performed in murine models are also contentious (128).\nRegarding anti-inflammatory cytokines, it has been demonstrated that TGF-β decreases CD36/SR-A expression and the uptake of oxLDL in human macrophages (135, 136). Additional data emphasized this anti-foam cell effect by showing that TGF-β downregulates CD36/SR-BI mRNA levels in peritoneal macrophages (137) and increases ApoA–I and HDL-stimulated cholesterol efflux and ABCA-1 gene transcription in foam cells (138). Argmann et al. (139) demonstrated that TGF-β1 decreases LPL expression/activity and cholesteryl ester accumulation with a coincident increase in the expression of ABCG-1 in murine macrophages. Recently, it was reported that TGF-β diminishes LPL gene transcription (140) and increases the expression of ApoE (141). Moreover, other group has shown that CD4+ CD25+ regulatory T cells use TGF-β1 to inhibit the uptake of oxLDL and the expression of CD36/SR-A mRNA (142). These mechanisms evidence an anti-foam cell role for TGF-β1, although it is important to stress that TGF-β may promote development either of anti-atherosclerotic regulatory T cells or of T-helper 17 (Th17) cells, depending on factors in the local milieu. Therefore, TGF-β signaling in T cells could promote stabilization of atherosclerotic plaques through an IL-17-dependent pathway.\nThe effect of IL-10 on plaque formation has also been reported. This anti-inflammatory mediator downregulates the CD36 SR expression (143) and, in consequence, decreases the accumulation of cholesterol in human macrophages (144). In addition, two independent groups have reported anti-foam cell activity of IL-10. Indeed, they found that IL-10 enhances the uptake and efflux of cholesterol by human macrophages in vitro and reduces foam cell formation and atherosclerotic plaque progression in vivo (145, 146). Altogether, the results suggest that IL-10 counteract atherogenesis.\nBesides M1 and M2 macrophage profiles, additional phenotypes have been described within atherosclerotic lesion and they have become a topic of increasing relevance. In areas of hemorrhage, macrophages respond adaptively to heme acquiring an activation status that was designated hemorrhage-associated macrophages (Mhem). In vitro human blood-derived monocytes are induced to Mhem by stimulation with hemoglobin–haptoglobin complexes and it is dependent on an autocrine action of secreted IL-10 (147, 148). This macrophage profile prevents foam cell formation and has antioxidant functions. Other study showed that oxPL products that accumulate in atherosclerotic lesions induce another phenotype designated as Mox. Data indicate that Mox macrophages are characterized by high expression of heme oxygenase-1 and it comprises approximately 30% of all macrophages in established atherosclerotic lesions in experimental models (149). In addition, CXCL4, a chemokine released by activated platelet, was demonstrated to prevent monocytes/macrophages apoptosis and promote its differentiation into a phenotype called “M4” (MMP7+ S100A8+ CD68+). M4 macrophage was described in human coronary atherosclerotic plaques ex vivo and postmortem (150, 151). The prevalence of this macrophage subset is higher in patients with severe coronary artery disease and there is a significant correlation between the accumulation of M4 macrophages within the intima and plaque destabilization, which is independent of the overall number of macrophages in the vascular wall (150).\nRecently, several groups have published that IL-17A-stimulated macrophages are suggested to constitute a new macrophage population. Barin et al. (152) showed that IL-17A induces activation of primary macrophages in a unique profile of cytokines and chemokines, including IL-12p70, GM-CSF, IL-3, IL-9, CCL4 and CCL5, that can be distinguished from previously characterized macrophage activation states. Moreover, another work showed that IL-17A induces a pro-inflammatory transcriptome in human monocytes/macrophages, including cytokines such as IL-1α and IL-6, chemokines like CCL2, CCL8, CCL20, CXCL1, CXCL2 and CXCL6, genes involved in oxidative stress, upregulation of CD14, CD163 levels and downregulation of genes associated with T cell costimulation. By comparing the entire transcriptomes of M1, M2 and M4 macrophages, authors confirm that IL-17A-treated macrophages are a unique polarization subset (153). On the other hand, it was reported that IL-17 also can strongly amplifies human monocytes/macrophages differentiation toward M2c profile, making it resistant to apoptosis and promoting its MerTK-dependent clearance of apoptotic bodies (154). M2c cells (CD14bright CD16+ CD163+ MerTK+) are a subset of alternatively activated macrophages induced by M-CSF plus IL-10 or glucocorticoids and they are involved in inflammation resolution through phagocytosis of early apoptotic neutrophils and anti-inflammatory cytokines production (155). In contrast with the mentioned effects of IL-17, authors demonstrated that both IFN-γ/Th1 environment and IL-4-chronic exposure impair glucocorticoid and IL-10-induced M2c macrophage differentiation and also alters phagocytosis function of stablished M2c macrophages by reducing MerTK levels and promoting its apoptosis. Thus, this work indicates that Th17 environment orchestrates the resolution of innate inflammation through expansion of M2c regulatory macrophages.\nAn important point to note is that in a predominantly Th17 environment, monocytes/macrophages have a key role in the IL-17-induced inflammatory responses. In an experimental murine autoimmune myocarditis (EAM) model, the genetic ablation of IL-17RA alters the recruitment of monocytes/macrophages, which is the most numerous component of the inflammatory infiltrate and it is implicated in the cardiac damage (152). In concordance, other work shows that IL-17A promotes cardiac infiltration of Ly6Chigh monocytes by inducing fibroblasts to produce high levels of cytokines and chemokines. Particularly, GM-CSF production by IL-17-stimulated cardiac fibroblasts drives differentiation of infiltrating monocytes into an even more inflammatory phenotype which further intensifies the myocarditis. Experiments performed in IL17RAKO mice or depletion of Ly6Chigh monocytes/macrophages demonstrates the involvement of these innate cells in the inflammatory dilated cardiomyopathy (156). On the other hand, in an advanced atherosclerotic model in ApoEKO mice, in vivo IL-17A blocking markedly prevents atherosclerotic lesion progression and improves its stability by reducing inflammatory burden and monocyte infiltration (153). Moreover, the CCL2 and CCL5 production were significantly reduced in those IL-17A mAb-treated mice. In vitro experiments demonstrated that IL-17A induces adhesion of human monocytes, adhesion and rolling of platelets on endothelial cells. Other studies performed in early stages of atherosclerosis demonstrate that IL-17/IL-17RA axis has a pro-atherogenic role by promoting early monocyte recruitment into plaque through CCL5 production, endothelial VCAM-1 expression and increasing CXCL1 expression on the vessel lumen (157–159).\n\nPurinergic Signaling\nPurinergic system has been recognized as a main pathway to regulate immune response and it is critical to prevent the collateral damage produced by the exacerbation of the immune response in several diseases including cardiac pathologies. In normal conditions, ATP is localized intracellularly where it is the main source of energy for cell functions and it accomplishes indispensable functions in cellular metabolism such as proliferation, migration, motility, biosynthesis and contraction functions within the cardiomyocytes. In addition to its metabolic function, ATP also acts as an important signaling molecule when it is released to the extracellular compartment. After it is released, the extracellular ATP hydrolysis is governed by the activity of two membrane ectoenzymes, the CD39 that catalyzes the phosphohydrolysis of ATP to ADO monophosphate (AMP) and CD73 that hydrolyzes AMP into ADO and inorganic phosphate (45). CD39 and CD73 expression on immune and vascular cells and their enzymatic activities play decisive role in the regulation of the magnitude and duration of purinergic signals that modulate cellular function in an autocrine or paracrine manner (160). These effects are mediated by two types of nucleotide/nucleoside receptors: P1 receptors activated by extracellular ADO and P2 receptors activated by ATP and other nucleotides such as ADP and AMP. The first group includes 4 ADO receptors (ADORA 1, ADORA 2A, ADORA 2B, and ADORA 3), and the second group includes several P2X and P2Y subtypes of receptors (161).\nPurinergic signaling is highly described in tumoral context (162) and in autoimmune diseases (163) but poorly studied in the context of cardiac pathologies. Given the high production rate of ATP and the turnover required to maintain its continuous mechanical work, disruption of heart tissue generates the release of high amounts of ATP to the extracellular space. Bonner et al. have reported that cardiomyocytes and erythrocytes do not detectably express the ATP catabolic machinery, but coronary endothelial cells were highly positive for CD39 under basal conditions and a low proportion of these cells express CD73. Furthermore, they stated that resident cardiac APCs and monocytes were the responsible of the first step of ATP degradation in the heart after ischemic injury because they were highly positive for the CD39 while CD73 was absent. Likewise, the dephosphorylating step of AMP to immunosuppressive ADO seems to take place on T lymphocytes and granulocytes because CD73 was mainly expressed in those populations (164).\nIn contrast to the pro-inflammatory action of ATP, ADO exhibits diverse immunoregulatory roles. Bonner and coworkers found that macrophages stimulated through ADORA 2A and ADORA 2B receptors with ADO undergo M2 phenotype in infarcted mice. In addition, in CD73KO mice there was augmented expression of microbicidal M1 genes, while the expression of M2 genes like arginase-1, IL-10, and TGF-β decreased. This monocyte imbalance toward inflammatory Ly6Chigh-expressing monocytes in mice lacking CD73 had been related to adverse myocardial healing and ventricular dilatation after MI (165). Furthermore, Haskó et al. reported that ADO diminish the oxidative burst by downregulating NO production and inhibit TNF release from monocytes and macrophages (166). A parallel mechanism has also been reported by Cain group in murine isquemia/reperfusion model where ADO pretreatment decreased myocardial TNF production (167). There is strong evidence that ADO can modulate leukocyte adhesion to vascular endothelium in vivo. In this sense, Koszalka et al. demonstrated that ADO is an important endogenous pathway to modulate the inflammatory vascular response. After a period of ischemia-reperfusion, they observed significant increase of leukocyte adherence to the vascular endothelium only in the CD73 mutant (168). It has also been suggested that purinergic signals play a role in cellular migration. Analysis of atherosclerotic lesions of P2Y6 KO mice revealed fewer macrophages, diminished RNA expression of IL-6 and VCAM-1, suggesting that deficiency in this ATP receptor limits atherosclerosis and plaque inflammation (169). Recently, it was reported that lack of P2X7 receptor resolute plaque destabilization by inhibiting inflammasome activation and consequently ameliorates experimental atherosclerosis. ATP binding to P2 receptors is crucial to NLRP3 assembly and the subsequent IL-1β release. P2X7 null mice had decreased amount of macrophages in the wound and, in consequence, smaller and less inflamed atherosclerotic lesions than respective control animals (170). Altogether, the data indicate that purinergic signaling can modulate macrophage polarization and infiltration; and that ADO is a key metabolite in suppressing inflammation following injury or infection.\nIn addition, ADO induces the production of vascular endothelial growth factor (VEGF) in human macrophages, associated with increased hypoxia inducible factor-1α (HIF-1α) expression, the main transcriptional inducer of VEGF in hypoxic milieu. VEGF promotes blood vessel formation, induces cell proliferation and initiates immune cell migration to stimulate vasculogenesis and angiogenesis (171). On the other hand, extracellular ATP contributes to tissue repair by the stimulation of P2X7 receptor and release of the pro-angiogenic factor VEGF (172). Summing up, activation of purinergic receptors in macrophages can promote wound healing and may be targeted to improve cardiac repair mechanisms.\n\nMetabolism\nThe heart changes its substrate preferences throughout the life, under physiological or pathological conditions. This metabolic flexibility allows it to adapt to environmental changes. However, shifts in fuel selectivity can become detrimental in the pathological heart (173).\nPathological cardiac hypertrophy is a common consequence of several cardiovascular diseases and is a maladaptive response to chronic stress, sometimes resulting in cardiac failure. Pathological hypertrophy involves a shift in fuel metabolism from fatty-acid oxidation to enhanced reliance on glucose. Increased glucose utilization is characterized by upregulation of glucose uptake and glycolysis concomitant with no change or a decrease in glucose oxidation, resulting in uncoupling of glucose uptake/oxidation metabolism. Nowadays, it is accepted that changes in mitochondrial function and cardiac metabolism precede heart dysfunction, indicating that metabolic remodeling is an early event in the development of cardiac diseases.\nRecent interest in understanding the impact of these fundamental metabolic changes on immune cell differentiation and function has yielded numerous studies examining the pathways and metabolites involved in driving protective immune responses. A key question that has emerged is whether differential metabolic activity (i.e., use of glycolysis versus mitochondrial respiration) is coincident with differentiation or if it is a direct driver of alterations in immune cell differentiation and function. Classically activated macrophages that promote inflammation utilize glycolysis and glutamine metabolism to generate large quantities of succinate which enhances inflammation and IL-1β production (174). In contrast, macrophages that play a role in tissue healing and inflammatory resolution increase mitochondrial lipid oxidation (175). A key differentiator between these metabolic phenotypes may arise in the mitochondria, where rather than engaging in conventional electron transport, altered electron flow through the succinate dehydrogenase complex promotes ROS generation and inflammation (176). Enhanced glycolysis in coronary artery disease patients drives mitochondrial ROS production, resulting in pyruvate kinase M2 (PKM2) assembly and translocation to the nucleus. It then phosphorylates and activates STAT3 to promote the production of the cytokines IL-1β and IL-6 (177). Indeed, the increased activation of glycolytic pathway was associated with epigenetic changes in monocyte-derived macrophages describing a new mechanism to explain trained immunity in human innate immune cells (178).\nDiabetes is a major risk factor for cardiovascular diseases (179). As such it may lead to heart failure indirectly, by promoting the development of coronary artery disease. However, it is now known that diabetes may cause heart failure also by eliciting a direct detrimental impact on the myocardium leading to the development of cardiac hypertrophy, diastolic and systolic dysfunction (180). The clinical condition associated with the spectrum of cardiac abnormalities induced by diabetes is termed diabetic cardiomyopathy. High glucose levels and dyslipidemia directly induce the upregulation and secretion of cytokines, chemokines and adhesion molecules in immune cells by modulating multiple signaling pathways that converge toward NF-κB signaling (181–183). Activation of the renin–angiotensin–aldosterone system and accumulation of advanced glycation end-products (AGE) and DAMPs molecules like extracellular high-mobility group box-1 protein (HMGB1), also represent key mechanisms that mediate inflammatory response in the diabetic heart primarily by acting TLRs (184–186). Following these initial molecular events, leukocytes infiltrate the myocardium and perpetuate the inflammatory process through secretion of cytokines and pro-fibrotic factors and by increasing the production of ROS. Mediators resulting from this inflammatory cascade, in turn, modulate specific intracellular signaling mechanisms in cardiac cells causing hypertrophy, mitochondrial dysfunction, ER stress and cell death fibroblast proliferation and collagen production. In addition, inflammatory factors may affect myocardial metabolic processes and interfere with cardiomyocyte contractile properties. These abnormalities together promote the development of diabetic cardiomyopathy.\nDiabetes-associated metabolic derangements can directly induce cytokine expression and release from cardiac cells. It was previously shown that hyperglycemia augmented the expression of HMGB1 in isolated cardiomyocytes, macrophages and cardiac fibroblasts, thereby activating the MAPK and NF-κB pathways and by inducing TNF and IL-6 secretion (187). Inhibition of HMGB1 decreased myocardial inflammation and fibrosis in a murine model of type 1 diabetes and protected diabetic animals in response to postinfarction remodeling (187, 188).\nAn increase in circulating lipids may also contribute to cardiac inflammation in diabetes. Fatty acids activate TLR4 that strongly promotes inflammation through the NF-κB pathway (189). Mice with a TLR4 gene deletion demonstrate improved cardiac function and reduced cardiac intracellular lipid accumulation in response to streptozotocin-induced diabetes (190). Fatty acids induce the subsequent expression of IL-6, TNF and IL-1, which can be reversed by peroxisome proliferator-activated receptor-γ, β/δ (PPAR-b/d) (191). Previous work showed that hyperglycemia and high circulating levels of lipids promote inflammation through the activation of protein kinase C (PKC), which activates MAPK pathway thereby inactivating IkB (192).\nThe challenge to improve cardiovascular disorders therapy by modulating metabolic pathways must take into account potential off-target effects on other cells and tissues. In these sense, by exploiting the intrinsic capacity of monocytes and macrophages to take up foreign particles, the use of nanoparticle formulations might be one option to achieve targeting specificity (193).\n\nOxidative Stress\nOxygen is the molecular source of the production of several molecules that damage vital tissues. Reactive oxygen and nitrogen species (RONS) are continuously produced as by-products of the reaction leading to energy production through the mitochondrial and microsomal electron-transport chains. In phagocytes, the oxidative bursts and enzyme systems such as xanthine oxidase and cytochrome P-450 oxidase are the endogenous sources of RONS. While physiological levels of RONS are crucial for cell function, excessive RONS levels trigger oxidative stress. Oxidative stress may damage clues molecules (proteins, lipids, DNA) and could, eventually, conducted to cell death. To prevent oxidative stress, antioxidants have evolved to protect biological systems against RONS-induced damage. Oxidative stress resulting from uncontrolled production of RONS that exceeds the antioxidant capacity, exacerbate atherosclerosis; since it induces endothelial dysfunction by impairing the bioactivity of endothelial NO and promotes leukocyte adhesion, enhances inflammation and thrombosis.\nNumerous reports demonstrated that increased oxidative stress is associated with disturbances in cardiovascular diseases. In this sense, it was reported an overproduction of superoxide anion by cardiac dysfunctional mitochondria or increased production of RONS from non-mitochondrial sources in experimental models of type 1 diabetes (194, 195). The augmented production of RONS induces maladaptive cardiac response causing cardiac cells death contributing to cardiovascular disease (196). An excessive oxidative stress has been associated with increased cardiac cell apoptosis, as was evidenced by TUNEL+ cells and caspase 3 activation in T. cruzi-infected cardiac tissue (44). In this sense, high oxidative state has been associated with amplified lipid and DNA damage and the consequent cardiac cell injury and death. Therapeutic treatment that promotes RONS regulation have been shown to be effective in reducing cardiovascular dysfunction.\nIt is known that increased oxidative stress induces modifications of LDL, generating DAMPs that are detected by TLRs on different immune cell populations, mainly monocytes/macrophages. A variety of mechanisms mediated by enzymatic (such as 12/15-lipoxygenase and myeloperoxidase) or no-enzymatic redox reaction (such as RONS) boost LDL oxidation in the artery wall. The level of LDL oxidation modulates the immune system, when cells were treated with low oxLDL, the expression level of CD86, a marker for M1 macrophages, increases compared with that in cells treated with high oxLDL. In contrast, the expression level of the marker for M2 macrophages, CD206, significantly increases in cells treated with high oxLDL compared with that in cells treated with native LDL or low oxLDL. These results indicate that the degree of LDL oxidation affects the differentiation of monocytes into different subtypes of macrophages (197). Further research is needed to assess the functional consequences of oxidation processes on human macrophage behavior.\n"}
TEST0
{"project":"TEST0","denotations":[{"id":"29375564-174-181-3353284","span":{"begin":849,"end":852},"obj":"[\"15514167\"]"},{"id":"29375564-179-186-3353285","span":{"begin":854,"end":857},"obj":"[\"18566299\"]"},{"id":"29375564-123-130-3353286","span":{"begin":1032,"end":1035},"obj":"[\"15514167\"]"},{"id":"29375564-128-135-3353287","span":{"begin":1037,"end":1040},"obj":"[\"19041276\"]"},{"id":"29375564-235-242-3353288","span":{"begin":1284,"end":1287},"obj":"[\"18772495\"]"},{"id":"29375564-146-153-3353289","span":{"begin":1436,"end":1439},"obj":"[\"18772495\"]"},{"id":"29375564-85-92-3353290","span":{"begin":1527,"end":1530},"obj":"[\"1556191\"]"},{"id":"29375564-233-240-3353291","span":{"begin":1689,"end":1692},"obj":"[\"19041276\"]"},{"id":"29375564-230-237-3353292","span":{"begin":1694,"end":1697},"obj":"[\"20675591\"]"},{"id":"29375564-228-235-3353293","span":{"begin":1792,"end":1795},"obj":"[\"10845873\"]"},{"id":"29375564-233-240-3353294","span":{"begin":1797,"end":1800},"obj":"[\"8486772\"]"},{"id":"29375564-235-242-3353295","span":{"begin":1832,"end":1835},"obj":"[\"10845873\"]"},{"id":"29375564-191-198-3353296","span":{"begin":2062,"end":2065},"obj":"[\"20675591\"]"},{"id":"29375564-97-104-3353297","span":{"begin":2165,"end":2168},"obj":"[\"18487233\"]"},{"id":"29375564-120-127-3353298","span":{"begin":2423,"end":2426},"obj":"[\"10969071\"]"},{"id":"29375564-165-172-3353299","span":{"begin":2594,"end":2597},"obj":"[\"12777468\"]"},{"id":"29375564-207-214-3353300","span":{"begin":2636,"end":2639},"obj":"[\"19032770\"]"},{"id":"29375564-230-237-3353301","span":{"begin":2727,"end":2730},"obj":"[\"19189937\"]"},{"id":"29375564-195-202-3353302","span":{"begin":2928,"end":2931},"obj":"[\"16492740\"]"},{"id":"29375564-200-207-3353303","span":{"begin":2933,"end":2936},"obj":"[\"19913791\"]"},{"id":"29375564-233-240-3353304","span":{"begin":3020,"end":3023},"obj":"[\"18487233\"]"},{"id":"29375564-152-159-3353305","span":{"begin":3178,"end":3181},"obj":"[\"10625669\"]"},{"id":"29375564-157-164-3353306","span":{"begin":3183,"end":3186},"obj":"[\"10749696\"]"},{"id":"29375564-140-147-3353307","span":{"begin":3329,"end":3332},"obj":"[\"17056295\"]"},{"id":"29375564-233-240-3353308","span":{"begin":3438,"end":3441},"obj":"[\"11352993\"]"},{"id":"29375564-16-23-3353309","span":{"begin":3460,"end":3463},"obj":"[\"11742878\"]"},{"id":"29375564-72-79-3353310","span":{"begin":3709,"end":3712},"obj":"[\"15755745\"]"},{"id":"29375564-115-122-3353311","span":{"begin":3752,"end":3755},"obj":"[\"16601234\"]"},{"id":"29375564-147-154-3353312","span":{"begin":3905,"end":3908},"obj":"[\"20007839\"]"},{"id":"29375564-70-77-3353313","span":{"begin":4429,"end":4432},"obj":"[\"16336952\"]"},{"id":"29375564-160-167-3353314","span":{"begin":4519,"end":4522},"obj":"[\"17689273\"]"},{"id":"29375564-186-193-3353315","span":{"begin":4795,"end":4798},"obj":"[\"19776020\"]"},{"id":"29375564-191-198-3353316","span":{"begin":4800,"end":4803},"obj":"[\"20354139\"]"},{"id":"29375564-174-181-3353317","span":{"begin":5370,"end":5373},"obj":"[\"19234137\"]"},{"id":"29375564-179-186-3353318","span":{"begin":5375,"end":5378},"obj":"[\"22777293\"]"},{"id":"29375564-209-216-3353319","span":{"begin":5799,"end":5802},"obj":"[\"20651288\"]"},{"id":"29375564-94-101-3353320","span":{"begin":6107,"end":6110},"obj":"[\"25828120\"]"},{"id":"29375564-99-106-3353321","span":{"begin":6112,"end":6115},"obj":"[\"19910578\"]"},{"id":"29375564-232-239-3353322","span":{"begin":6425,"end":6428},"obj":"[\"25828120\"]"},{"id":"29375564-14-21-3353323","span":{"begin":6577,"end":6580},"obj":"[\"22161142\"]"},{"id":"29375564-151-158-3353324","span":{"begin":7339,"end":7342},"obj":"[\"25261478\"]"},{"id":"29375564-236-243-3353325","span":{"begin":7581,"end":7584},"obj":"[\"23596310\"]"},{"id":"29375564-231-238-3353326","span":{"begin":7874,"end":7877},"obj":"[\"22942426\"]"},{"id":"29375564-236-243-3353327","span":{"begin":8759,"end":8762},"obj":"[\"22161142\"]"},{"id":"29375564-181-188-3353328","span":{"begin":9324,"end":9327},"obj":"[\"24935258\"]"},{"id":"29375564-235-242-3353329","span":{"begin":9565,"end":9568},"obj":"[\"25261478\"]"},{"id":"29375564-223-230-3353330","span":{"begin":10092,"end":10095},"obj":"[\"22302786\", \"20007582\", \"20368519\"]"},{"id":"29375564-233-239-3353331","span":{"begin":11049,"end":11051},"obj":"[\"23601906\"]"},{"id":"29375564-234-241-3353332","span":{"begin":11297,"end":11300},"obj":"[\"20068232\"]"},{"id":"29375564-158-165-3353333","span":{"begin":11658,"end":11661},"obj":"[\"18302942\"]"},{"id":"29375564-61-68-3353334","span":{"begin":11725,"end":11728},"obj":"[\"28258700\"]"},{"id":"29375564-94-101-3353335","span":{"begin":11758,"end":11761},"obj":"[\"27665459\"]"},{"id":"29375564-179-186-3353336","span":{"begin":12691,"end":12694},"obj":"[\"22514659\"]"},{"id":"29375564-179-186-3353337","span":{"begin":13279,"end":13282},"obj":"[\"23720442\"]"},{"id":"29375564-161-168-3353338","span":{"begin":13446,"end":13449},"obj":"[\"17056121\"]"},{"id":"29375564-155-162-3353339","span":{"begin":13607,"end":13610},"obj":"[\"9698510\"]"},{"id":"29375564-151-158-3353340","span":{"begin":13999,"end":14002},"obj":"[\"15358667\"]"},{"id":"29375564-222-229-3353341","span":{"begin":14313,"end":14316},"obj":"[\"25104800\"]"},{"id":"29375564-168-175-3353342","span":{"begin":14760,"end":14763},"obj":"[\"28377486\"]"},{"id":"29375564-147-154-3353343","span":{"begin":15358,"end":15361},"obj":"[\"20067761\"]"},{"id":"29375564-149-156-3353344","span":{"begin":15513,"end":15516},"obj":"[\"20668222\"]"},{"id":"29375564-86-93-3353345","span":{"begin":15948,"end":15951},"obj":"[\"17360992\"]"},{"id":"29375564-196-203-3353346","span":{"begin":17384,"end":17387},"obj":"[\"23535595\"]"},{"id":"29375564-128-135-3353347","span":{"begin":17518,"end":17521},"obj":"[\"16814729\"]"},{"id":"29375564-229-236-3353348","span":{"begin":17782,"end":17785},"obj":"[\"27667687\"]"},{"id":"29375564-102-109-3353349","span":{"begin":18065,"end":18068},"obj":"[\"26926996\"]"},{"id":"29375564-211-218-3353350","span":{"begin":18282,"end":18285},"obj":"[\"27926861\"]"},{"id":"29375564-61-68-3353351","span":{"begin":18349,"end":18352},"obj":"[\"24315620\"]"},{"id":"29375564-214-221-3353352","span":{"begin":18675,"end":18678},"obj":"[\"17592090\"]"},{"id":"29375564-228-235-3353353","span":{"begin":19041,"end":19044},"obj":"[\"25779542\", \"25814686\", \"27340270\"]"},{"id":"29375564-236-243-3353354","span":{"begin":19359,"end":19362},"obj":"[\"21527743\", \"19200056\", \"14670831\"]"},{"id":"29375564-229-236-3353355","span":{"begin":20397,"end":20400},"obj":"[\"20703492\"]"},{"id":"29375564-177-184-3353356","span":{"begin":20580,"end":20583},"obj":"[\"20703492\"]"},{"id":"29375564-182-189-3353357","span":{"begin":20585,"end":20588},"obj":"[\"24485636\"]"},{"id":"29375564-89-96-3353358","span":{"begin":20771,"end":20774},"obj":"[\"17478729\"]"},{"id":"29375564-170-177-3353359","span":{"begin":20947,"end":20950},"obj":"[\"22842069\"]"},{"id":"29375564-155-162-3353360","span":{"begin":21108,"end":21111},"obj":"[\"21070867\"]"},{"id":"29375564-204-211-3353361","span":{"begin":21318,"end":21321},"obj":"[\"12086926\"]"},{"id":"29375564-203-210-3353362","span":{"begin":21698,"end":21701},"obj":"[\"27791119\"]"},{"id":"29375564-213-220-3353363","span":{"begin":23131,"end":23134},"obj":"[\"17307368\"]"},{"id":"29375564-218-225-3353364","span":{"begin":23136,"end":23139},"obj":"[\"16612592\"]"},{"id":"29375564-138-145-3353365","span":{"begin":23280,"end":23283},"obj":"[\"12788379\"]"},{"id":"29375564-184-190-3353366","span":{"begin":23470,"end":23472},"obj":"[\"28087471\"]"},{"id":"29375564-137-144-3353367","span":{"begin":24660,"end":24663},"obj":"[\"26294848\"]"}],"text":"Signaling Pathways and Molecules Modulating Cardiovascular Immune Response\n\nCytokines and Chemokines\nMacrophages are major contributors to the inflammatory response in the setting of cardiovascular diseases. They secrete diverse pro-inflammatory mediators (including cytokines, chemokines, ROS and nitrogen species, among others), and MMPs and they eventually die by necrosis or apoptosis. Dying macrophages release their lipid contents, which lead to the development of a pro-thrombotic necrotic core. The necrotic core is, in turn, a clue component of unstable plaques and contributes to their rupture and the consequent intravascular blood clot that causes MI and stroke. The main effect of cytokines/chemokines released by macrophages is the recruitment of different innate and adaptive immune cell populations into the atherosclerotic lesions (120, 121), thereby amplifying the inflammatory environment. For example, different inflammatory mediators such as IL-1, TNF and IFN-γ increase the expression of MCP-1 in macrophages (120, 122). Also, under inflammatory conditions macrophages produce different enzymes such as MMPs, cathepsins and serine proteases that degrade the extracellular matrix of the atherosclerotic plaque contributing to the pathogenesis of atherosclerosis (123). In this sense, IL-1 and TNF increase the expression of numerous MMPs, such as MMP-9, and their activity contribute to unstable plaque morphology (123).\nWhile initial reports indicated that IFN-γ prevented macrophage foam cell formation (124), subsequent studies demonstrate that this cytokine promotes foam cell formation through diverse mechanisms: enhancing the uptake of oxLDL/AcLDL through SRs (122, 125), diminishing efflux of cholesterol via inhibition of ABCA-1 transporter and ApoE expression (126, 127) and increasing ACAT-1 levels (126) in macrophage-derived foam cells. For example, it was recently reported that the axis IFN-γ/STAT1 mediates the uptake of acLDL/oxLDL by human macrophages in an extracellular signal-regulated kinase (ERK)-dependent signaling (125). The activity of IFN-γ as a pro-foam cell mediator has been supported by several in vivo studies (128).\nSince IFN-γ was identified as a pro-foam cell cytokine, different authors evaluated the effect of other pro-inflammatory cytokines. Studies using a murine macrophage cell line have shown that TNF inhibits the expression of SRs and the uptake of oxLDL (129). Regarding additional mechanisms, more recent evidence has suggested that TNF promotes foam cell formation in vitro by reducing the mRNA expression of ABCA-1/ABCG-1 (130), and intracellular lipid catabolism (131), and by enhancing the expression of ACAT-1 and the accumulation of cholesteryl ester (132). However, the role for TNF remains controversial since other studies have shown that TNF enhances ABCA-1 expression and the efflux of cholesterol in peritoneal macrophages stimulated with ApoA–I (133, 134) and since evidences from studies performed in murine models are also contentious (128).\nRegarding anti-inflammatory cytokines, it has been demonstrated that TGF-β decreases CD36/SR-A expression and the uptake of oxLDL in human macrophages (135, 136). Additional data emphasized this anti-foam cell effect by showing that TGF-β downregulates CD36/SR-BI mRNA levels in peritoneal macrophages (137) and increases ApoA–I and HDL-stimulated cholesterol efflux and ABCA-1 gene transcription in foam cells (138). Argmann et al. (139) demonstrated that TGF-β1 decreases LPL expression/activity and cholesteryl ester accumulation with a coincident increase in the expression of ABCG-1 in murine macrophages. Recently, it was reported that TGF-β diminishes LPL gene transcription (140) and increases the expression of ApoE (141). Moreover, other group has shown that CD4+ CD25+ regulatory T cells use TGF-β1 to inhibit the uptake of oxLDL and the expression of CD36/SR-A mRNA (142). These mechanisms evidence an anti-foam cell role for TGF-β1, although it is important to stress that TGF-β may promote development either of anti-atherosclerotic regulatory T cells or of T-helper 17 (Th17) cells, depending on factors in the local milieu. Therefore, TGF-β signaling in T cells could promote stabilization of atherosclerotic plaques through an IL-17-dependent pathway.\nThe effect of IL-10 on plaque formation has also been reported. This anti-inflammatory mediator downregulates the CD36 SR expression (143) and, in consequence, decreases the accumulation of cholesterol in human macrophages (144). In addition, two independent groups have reported anti-foam cell activity of IL-10. Indeed, they found that IL-10 enhances the uptake and efflux of cholesterol by human macrophages in vitro and reduces foam cell formation and atherosclerotic plaque progression in vivo (145, 146). Altogether, the results suggest that IL-10 counteract atherogenesis.\nBesides M1 and M2 macrophage profiles, additional phenotypes have been described within atherosclerotic lesion and they have become a topic of increasing relevance. In areas of hemorrhage, macrophages respond adaptively to heme acquiring an activation status that was designated hemorrhage-associated macrophages (Mhem). In vitro human blood-derived monocytes are induced to Mhem by stimulation with hemoglobin–haptoglobin complexes and it is dependent on an autocrine action of secreted IL-10 (147, 148). This macrophage profile prevents foam cell formation and has antioxidant functions. Other study showed that oxPL products that accumulate in atherosclerotic lesions induce another phenotype designated as Mox. Data indicate that Mox macrophages are characterized by high expression of heme oxygenase-1 and it comprises approximately 30% of all macrophages in established atherosclerotic lesions in experimental models (149). In addition, CXCL4, a chemokine released by activated platelet, was demonstrated to prevent monocytes/macrophages apoptosis and promote its differentiation into a phenotype called “M4” (MMP7+ S100A8+ CD68+). M4 macrophage was described in human coronary atherosclerotic plaques ex vivo and postmortem (150, 151). The prevalence of this macrophage subset is higher in patients with severe coronary artery disease and there is a significant correlation between the accumulation of M4 macrophages within the intima and plaque destabilization, which is independent of the overall number of macrophages in the vascular wall (150).\nRecently, several groups have published that IL-17A-stimulated macrophages are suggested to constitute a new macrophage population. Barin et al. (152) showed that IL-17A induces activation of primary macrophages in a unique profile of cytokines and chemokines, including IL-12p70, GM-CSF, IL-3, IL-9, CCL4 and CCL5, that can be distinguished from previously characterized macrophage activation states. Moreover, another work showed that IL-17A induces a pro-inflammatory transcriptome in human monocytes/macrophages, including cytokines such as IL-1α and IL-6, chemokines like CCL2, CCL8, CCL20, CXCL1, CXCL2 and CXCL6, genes involved in oxidative stress, upregulation of CD14, CD163 levels and downregulation of genes associated with T cell costimulation. By comparing the entire transcriptomes of M1, M2 and M4 macrophages, authors confirm that IL-17A-treated macrophages are a unique polarization subset (153). On the other hand, it was reported that IL-17 also can strongly amplifies human monocytes/macrophages differentiation toward M2c profile, making it resistant to apoptosis and promoting its MerTK-dependent clearance of apoptotic bodies (154). M2c cells (CD14bright CD16+ CD163+ MerTK+) are a subset of alternatively activated macrophages induced by M-CSF plus IL-10 or glucocorticoids and they are involved in inflammation resolution through phagocytosis of early apoptotic neutrophils and anti-inflammatory cytokines production (155). In contrast with the mentioned effects of IL-17, authors demonstrated that both IFN-γ/Th1 environment and IL-4-chronic exposure impair glucocorticoid and IL-10-induced M2c macrophage differentiation and also alters phagocytosis function of stablished M2c macrophages by reducing MerTK levels and promoting its apoptosis. Thus, this work indicates that Th17 environment orchestrates the resolution of innate inflammation through expansion of M2c regulatory macrophages.\nAn important point to note is that in a predominantly Th17 environment, monocytes/macrophages have a key role in the IL-17-induced inflammatory responses. In an experimental murine autoimmune myocarditis (EAM) model, the genetic ablation of IL-17RA alters the recruitment of monocytes/macrophages, which is the most numerous component of the inflammatory infiltrate and it is implicated in the cardiac damage (152). In concordance, other work shows that IL-17A promotes cardiac infiltration of Ly6Chigh monocytes by inducing fibroblasts to produce high levels of cytokines and chemokines. Particularly, GM-CSF production by IL-17-stimulated cardiac fibroblasts drives differentiation of infiltrating monocytes into an even more inflammatory phenotype which further intensifies the myocarditis. Experiments performed in IL17RAKO mice or depletion of Ly6Chigh monocytes/macrophages demonstrates the involvement of these innate cells in the inflammatory dilated cardiomyopathy (156). On the other hand, in an advanced atherosclerotic model in ApoEKO mice, in vivo IL-17A blocking markedly prevents atherosclerotic lesion progression and improves its stability by reducing inflammatory burden and monocyte infiltration (153). Moreover, the CCL2 and CCL5 production were significantly reduced in those IL-17A mAb-treated mice. In vitro experiments demonstrated that IL-17A induces adhesion of human monocytes, adhesion and rolling of platelets on endothelial cells. Other studies performed in early stages of atherosclerosis demonstrate that IL-17/IL-17RA axis has a pro-atherogenic role by promoting early monocyte recruitment into plaque through CCL5 production, endothelial VCAM-1 expression and increasing CXCL1 expression on the vessel lumen (157–159).\n\nPurinergic Signaling\nPurinergic system has been recognized as a main pathway to regulate immune response and it is critical to prevent the collateral damage produced by the exacerbation of the immune response in several diseases including cardiac pathologies. In normal conditions, ATP is localized intracellularly where it is the main source of energy for cell functions and it accomplishes indispensable functions in cellular metabolism such as proliferation, migration, motility, biosynthesis and contraction functions within the cardiomyocytes. In addition to its metabolic function, ATP also acts as an important signaling molecule when it is released to the extracellular compartment. After it is released, the extracellular ATP hydrolysis is governed by the activity of two membrane ectoenzymes, the CD39 that catalyzes the phosphohydrolysis of ATP to ADO monophosphate (AMP) and CD73 that hydrolyzes AMP into ADO and inorganic phosphate (45). CD39 and CD73 expression on immune and vascular cells and their enzymatic activities play decisive role in the regulation of the magnitude and duration of purinergic signals that modulate cellular function in an autocrine or paracrine manner (160). These effects are mediated by two types of nucleotide/nucleoside receptors: P1 receptors activated by extracellular ADO and P2 receptors activated by ATP and other nucleotides such as ADP and AMP. The first group includes 4 ADO receptors (ADORA 1, ADORA 2A, ADORA 2B, and ADORA 3), and the second group includes several P2X and P2Y subtypes of receptors (161).\nPurinergic signaling is highly described in tumoral context (162) and in autoimmune diseases (163) but poorly studied in the context of cardiac pathologies. Given the high production rate of ATP and the turnover required to maintain its continuous mechanical work, disruption of heart tissue generates the release of high amounts of ATP to the extracellular space. Bonner et al. have reported that cardiomyocytes and erythrocytes do not detectably express the ATP catabolic machinery, but coronary endothelial cells were highly positive for CD39 under basal conditions and a low proportion of these cells express CD73. Furthermore, they stated that resident cardiac APCs and monocytes were the responsible of the first step of ATP degradation in the heart after ischemic injury because they were highly positive for the CD39 while CD73 was absent. Likewise, the dephosphorylating step of AMP to immunosuppressive ADO seems to take place on T lymphocytes and granulocytes because CD73 was mainly expressed in those populations (164).\nIn contrast to the pro-inflammatory action of ATP, ADO exhibits diverse immunoregulatory roles. Bonner and coworkers found that macrophages stimulated through ADORA 2A and ADORA 2B receptors with ADO undergo M2 phenotype in infarcted mice. In addition, in CD73KO mice there was augmented expression of microbicidal M1 genes, while the expression of M2 genes like arginase-1, IL-10, and TGF-β decreased. This monocyte imbalance toward inflammatory Ly6Chigh-expressing monocytes in mice lacking CD73 had been related to adverse myocardial healing and ventricular dilatation after MI (165). Furthermore, Haskó et al. reported that ADO diminish the oxidative burst by downregulating NO production and inhibit TNF release from monocytes and macrophages (166). A parallel mechanism has also been reported by Cain group in murine isquemia/reperfusion model where ADO pretreatment decreased myocardial TNF production (167). There is strong evidence that ADO can modulate leukocyte adhesion to vascular endothelium in vivo. In this sense, Koszalka et al. demonstrated that ADO is an important endogenous pathway to modulate the inflammatory vascular response. After a period of ischemia-reperfusion, they observed significant increase of leukocyte adherence to the vascular endothelium only in the CD73 mutant (168). It has also been suggested that purinergic signals play a role in cellular migration. Analysis of atherosclerotic lesions of P2Y6 KO mice revealed fewer macrophages, diminished RNA expression of IL-6 and VCAM-1, suggesting that deficiency in this ATP receptor limits atherosclerosis and plaque inflammation (169). Recently, it was reported that lack of P2X7 receptor resolute plaque destabilization by inhibiting inflammasome activation and consequently ameliorates experimental atherosclerosis. ATP binding to P2 receptors is crucial to NLRP3 assembly and the subsequent IL-1β release. P2X7 null mice had decreased amount of macrophages in the wound and, in consequence, smaller and less inflamed atherosclerotic lesions than respective control animals (170). Altogether, the data indicate that purinergic signaling can modulate macrophage polarization and infiltration; and that ADO is a key metabolite in suppressing inflammation following injury or infection.\nIn addition, ADO induces the production of vascular endothelial growth factor (VEGF) in human macrophages, associated with increased hypoxia inducible factor-1α (HIF-1α) expression, the main transcriptional inducer of VEGF in hypoxic milieu. VEGF promotes blood vessel formation, induces cell proliferation and initiates immune cell migration to stimulate vasculogenesis and angiogenesis (171). On the other hand, extracellular ATP contributes to tissue repair by the stimulation of P2X7 receptor and release of the pro-angiogenic factor VEGF (172). Summing up, activation of purinergic receptors in macrophages can promote wound healing and may be targeted to improve cardiac repair mechanisms.\n\nMetabolism\nThe heart changes its substrate preferences throughout the life, under physiological or pathological conditions. This metabolic flexibility allows it to adapt to environmental changes. However, shifts in fuel selectivity can become detrimental in the pathological heart (173).\nPathological cardiac hypertrophy is a common consequence of several cardiovascular diseases and is a maladaptive response to chronic stress, sometimes resulting in cardiac failure. Pathological hypertrophy involves a shift in fuel metabolism from fatty-acid oxidation to enhanced reliance on glucose. Increased glucose utilization is characterized by upregulation of glucose uptake and glycolysis concomitant with no change or a decrease in glucose oxidation, resulting in uncoupling of glucose uptake/oxidation metabolism. Nowadays, it is accepted that changes in mitochondrial function and cardiac metabolism precede heart dysfunction, indicating that metabolic remodeling is an early event in the development of cardiac diseases.\nRecent interest in understanding the impact of these fundamental metabolic changes on immune cell differentiation and function has yielded numerous studies examining the pathways and metabolites involved in driving protective immune responses. A key question that has emerged is whether differential metabolic activity (i.e., use of glycolysis versus mitochondrial respiration) is coincident with differentiation or if it is a direct driver of alterations in immune cell differentiation and function. Classically activated macrophages that promote inflammation utilize glycolysis and glutamine metabolism to generate large quantities of succinate which enhances inflammation and IL-1β production (174). In contrast, macrophages that play a role in tissue healing and inflammatory resolution increase mitochondrial lipid oxidation (175). A key differentiator between these metabolic phenotypes may arise in the mitochondria, where rather than engaging in conventional electron transport, altered electron flow through the succinate dehydrogenase complex promotes ROS generation and inflammation (176). Enhanced glycolysis in coronary artery disease patients drives mitochondrial ROS production, resulting in pyruvate kinase M2 (PKM2) assembly and translocation to the nucleus. It then phosphorylates and activates STAT3 to promote the production of the cytokines IL-1β and IL-6 (177). Indeed, the increased activation of glycolytic pathway was associated with epigenetic changes in monocyte-derived macrophages describing a new mechanism to explain trained immunity in human innate immune cells (178).\nDiabetes is a major risk factor for cardiovascular diseases (179). As such it may lead to heart failure indirectly, by promoting the development of coronary artery disease. However, it is now known that diabetes may cause heart failure also by eliciting a direct detrimental impact on the myocardium leading to the development of cardiac hypertrophy, diastolic and systolic dysfunction (180). The clinical condition associated with the spectrum of cardiac abnormalities induced by diabetes is termed diabetic cardiomyopathy. High glucose levels and dyslipidemia directly induce the upregulation and secretion of cytokines, chemokines and adhesion molecules in immune cells by modulating multiple signaling pathways that converge toward NF-κB signaling (181–183). Activation of the renin–angiotensin–aldosterone system and accumulation of advanced glycation end-products (AGE) and DAMPs molecules like extracellular high-mobility group box-1 protein (HMGB1), also represent key mechanisms that mediate inflammatory response in the diabetic heart primarily by acting TLRs (184–186). Following these initial molecular events, leukocytes infiltrate the myocardium and perpetuate the inflammatory process through secretion of cytokines and pro-fibrotic factors and by increasing the production of ROS. Mediators resulting from this inflammatory cascade, in turn, modulate specific intracellular signaling mechanisms in cardiac cells causing hypertrophy, mitochondrial dysfunction, ER stress and cell death fibroblast proliferation and collagen production. In addition, inflammatory factors may affect myocardial metabolic processes and interfere with cardiomyocyte contractile properties. These abnormalities together promote the development of diabetic cardiomyopathy.\nDiabetes-associated metabolic derangements can directly induce cytokine expression and release from cardiac cells. It was previously shown that hyperglycemia augmented the expression of HMGB1 in isolated cardiomyocytes, macrophages and cardiac fibroblasts, thereby activating the MAPK and NF-κB pathways and by inducing TNF and IL-6 secretion (187). Inhibition of HMGB1 decreased myocardial inflammation and fibrosis in a murine model of type 1 diabetes and protected diabetic animals in response to postinfarction remodeling (187, 188).\nAn increase in circulating lipids may also contribute to cardiac inflammation in diabetes. Fatty acids activate TLR4 that strongly promotes inflammation through the NF-κB pathway (189). Mice with a TLR4 gene deletion demonstrate improved cardiac function and reduced cardiac intracellular lipid accumulation in response to streptozotocin-induced diabetes (190). Fatty acids induce the subsequent expression of IL-6, TNF and IL-1, which can be reversed by peroxisome proliferator-activated receptor-γ, β/δ (PPAR-b/d) (191). Previous work showed that hyperglycemia and high circulating levels of lipids promote inflammation through the activation of protein kinase C (PKC), which activates MAPK pathway thereby inactivating IkB (192).\nThe challenge to improve cardiovascular disorders therapy by modulating metabolic pathways must take into account potential off-target effects on other cells and tissues. In these sense, by exploiting the intrinsic capacity of monocytes and macrophages to take up foreign particles, the use of nanoparticle formulations might be one option to achieve targeting specificity (193).\n\nOxidative Stress\nOxygen is the molecular source of the production of several molecules that damage vital tissues. Reactive oxygen and nitrogen species (RONS) are continuously produced as by-products of the reaction leading to energy production through the mitochondrial and microsomal electron-transport chains. In phagocytes, the oxidative bursts and enzyme systems such as xanthine oxidase and cytochrome P-450 oxidase are the endogenous sources of RONS. While physiological levels of RONS are crucial for cell function, excessive RONS levels trigger oxidative stress. Oxidative stress may damage clues molecules (proteins, lipids, DNA) and could, eventually, conducted to cell death. To prevent oxidative stress, antioxidants have evolved to protect biological systems against RONS-induced damage. Oxidative stress resulting from uncontrolled production of RONS that exceeds the antioxidant capacity, exacerbate atherosclerosis; since it induces endothelial dysfunction by impairing the bioactivity of endothelial NO and promotes leukocyte adhesion, enhances inflammation and thrombosis.\nNumerous reports demonstrated that increased oxidative stress is associated with disturbances in cardiovascular diseases. In this sense, it was reported an overproduction of superoxide anion by cardiac dysfunctional mitochondria or increased production of RONS from non-mitochondrial sources in experimental models of type 1 diabetes (194, 195). The augmented production of RONS induces maladaptive cardiac response causing cardiac cells death contributing to cardiovascular disease (196). An excessive oxidative stress has been associated with increased cardiac cell apoptosis, as was evidenced by TUNEL+ cells and caspase 3 activation in T. cruzi-infected cardiac tissue (44). In this sense, high oxidative state has been associated with amplified lipid and DNA damage and the consequent cardiac cell injury and death. Therapeutic treatment that promotes RONS regulation have been shown to be effective in reducing cardiovascular dysfunction.\nIt is known that increased oxidative stress induces modifications of LDL, generating DAMPs that are detected by TLRs on different immune cell populations, mainly monocytes/macrophages. A variety of mechanisms mediated by enzymatic (such as 12/15-lipoxygenase and myeloperoxidase) or no-enzymatic redox reaction (such as RONS) boost LDL oxidation in the artery wall. The level of LDL oxidation modulates the immune system, when cells were treated with low oxLDL, the expression level of CD86, a marker for M1 macrophages, increases compared with that in cells treated with high oxLDL. In contrast, the expression level of the marker for M2 macrophages, CD206, significantly increases in cells treated with high oxLDL compared with that in cells treated with native LDL or low oxLDL. These results indicate that the degree of LDL oxidation affects the differentiation of monocytes into different subtypes of macrophages (197). Further research is needed to assess the functional consequences of oxidation processes on human macrophage behavior.\n"}
2_test
{"project":"2_test","denotations":[{"id":"29375564-15514167-34933940","span":{"begin":849,"end":852},"obj":"15514167"},{"id":"29375564-18566299-34933941","span":{"begin":854,"end":857},"obj":"18566299"},{"id":"29375564-15514167-34933942","span":{"begin":1032,"end":1035},"obj":"15514167"},{"id":"29375564-19041276-34933943","span":{"begin":1037,"end":1040},"obj":"19041276"},{"id":"29375564-18772495-34933944","span":{"begin":1284,"end":1287},"obj":"18772495"},{"id":"29375564-18772495-34933945","span":{"begin":1436,"end":1439},"obj":"18772495"},{"id":"29375564-1556191-34933946","span":{"begin":1527,"end":1530},"obj":"1556191"},{"id":"29375564-19041276-34933947","span":{"begin":1689,"end":1692},"obj":"19041276"},{"id":"29375564-20675591-34933948","span":{"begin":1694,"end":1697},"obj":"20675591"},{"id":"29375564-10845873-34933949","span":{"begin":1792,"end":1795},"obj":"10845873"},{"id":"29375564-8486772-34933950","span":{"begin":1797,"end":1800},"obj":"8486772"},{"id":"29375564-10845873-34933951","span":{"begin":1832,"end":1835},"obj":"10845873"},{"id":"29375564-20675591-34933952","span":{"begin":2062,"end":2065},"obj":"20675591"},{"id":"29375564-18487233-34933953","span":{"begin":2165,"end":2168},"obj":"18487233"},{"id":"29375564-10969071-34933954","span":{"begin":2423,"end":2426},"obj":"10969071"},{"id":"29375564-12777468-34933955","span":{"begin":2594,"end":2597},"obj":"12777468"},{"id":"29375564-19032770-34933956","span":{"begin":2636,"end":2639},"obj":"19032770"},{"id":"29375564-19189937-34933957","span":{"begin":2727,"end":2730},"obj":"19189937"},{"id":"29375564-16492740-34933958","span":{"begin":2928,"end":2931},"obj":"16492740"},{"id":"29375564-19913791-34933959","span":{"begin":2933,"end":2936},"obj":"19913791"},{"id":"29375564-18487233-34933960","span":{"begin":3020,"end":3023},"obj":"18487233"},{"id":"29375564-10625669-34933961","span":{"begin":3178,"end":3181},"obj":"10625669"},{"id":"29375564-10749696-34933962","span":{"begin":3183,"end":3186},"obj":"10749696"},{"id":"29375564-17056295-34933963","span":{"begin":3329,"end":3332},"obj":"17056295"},{"id":"29375564-11352993-34933964","span":{"begin":3438,"end":3441},"obj":"11352993"},{"id":"29375564-11742878-34933965","span":{"begin":3460,"end":3463},"obj":"11742878"},{"id":"29375564-15755745-34933966","span":{"begin":3709,"end":3712},"obj":"15755745"},{"id":"29375564-16601234-34933967","span":{"begin":3752,"end":3755},"obj":"16601234"},{"id":"29375564-20007839-34933968","span":{"begin":3905,"end":3908},"obj":"20007839"},{"id":"29375564-16336952-34933969","span":{"begin":4429,"end":4432},"obj":"16336952"},{"id":"29375564-17689273-34933970","span":{"begin":4519,"end":4522},"obj":"17689273"},{"id":"29375564-19776020-34933971","span":{"begin":4795,"end":4798},"obj":"19776020"},{"id":"29375564-20354139-34933972","span":{"begin":4800,"end":4803},"obj":"20354139"},{"id":"29375564-19234137-34933973","span":{"begin":5370,"end":5373},"obj":"19234137"},{"id":"29375564-22777293-34933974","span":{"begin":5375,"end":5378},"obj":"22777293"},{"id":"29375564-20651288-34933975","span":{"begin":5799,"end":5802},"obj":"20651288"},{"id":"29375564-25828120-34933976","span":{"begin":6107,"end":6110},"obj":"25828120"},{"id":"29375564-19910578-34933977","span":{"begin":6112,"end":6115},"obj":"19910578"},{"id":"29375564-25828120-34933978","span":{"begin":6425,"end":6428},"obj":"25828120"},{"id":"29375564-22161142-34933979","span":{"begin":6577,"end":6580},"obj":"22161142"},{"id":"29375564-25261478-34933980","span":{"begin":7339,"end":7342},"obj":"25261478"},{"id":"29375564-23596310-34933981","span":{"begin":7581,"end":7584},"obj":"23596310"},{"id":"29375564-22942426-34933982","span":{"begin":7874,"end":7877},"obj":"22942426"},{"id":"29375564-22161142-34933983","span":{"begin":8759,"end":8762},"obj":"22161142"},{"id":"29375564-24935258-34933984","span":{"begin":9324,"end":9327},"obj":"24935258"},{"id":"29375564-25261478-34933985","span":{"begin":9565,"end":9568},"obj":"25261478"},{"id":"29375564-22302786-34933986","span":{"begin":10092,"end":10095},"obj":"22302786"},{"id":"29375564-20007582-34933986","span":{"begin":10092,"end":10095},"obj":"20007582"},{"id":"29375564-20368519-34933986","span":{"begin":10092,"end":10095},"obj":"20368519"},{"id":"29375564-23601906-34933987","span":{"begin":11049,"end":11051},"obj":"23601906"},{"id":"29375564-20068232-34933988","span":{"begin":11297,"end":11300},"obj":"20068232"},{"id":"29375564-18302942-34933989","span":{"begin":11658,"end":11661},"obj":"18302942"},{"id":"29375564-28258700-34933990","span":{"begin":11725,"end":11728},"obj":"28258700"},{"id":"29375564-27665459-34933991","span":{"begin":11758,"end":11761},"obj":"27665459"},{"id":"29375564-22514659-34933992","span":{"begin":12691,"end":12694},"obj":"22514659"},{"id":"29375564-23720442-34933993","span":{"begin":13279,"end":13282},"obj":"23720442"},{"id":"29375564-17056121-34933994","span":{"begin":13446,"end":13449},"obj":"17056121"},{"id":"29375564-9698510-34933995","span":{"begin":13607,"end":13610},"obj":"9698510"},{"id":"29375564-15358667-34933996","span":{"begin":13999,"end":14002},"obj":"15358667"},{"id":"29375564-25104800-34933997","span":{"begin":14313,"end":14316},"obj":"25104800"},{"id":"29375564-28377486-34933998","span":{"begin":14760,"end":14763},"obj":"28377486"},{"id":"29375564-20067761-34933999","span":{"begin":15358,"end":15361},"obj":"20067761"},{"id":"29375564-20668222-34934000","span":{"begin":15513,"end":15516},"obj":"20668222"},{"id":"29375564-17360992-34934001","span":{"begin":15948,"end":15951},"obj":"17360992"},{"id":"29375564-23535595-34934002","span":{"begin":17384,"end":17387},"obj":"23535595"},{"id":"29375564-16814729-34934003","span":{"begin":17518,"end":17521},"obj":"16814729"},{"id":"29375564-27667687-34934004","span":{"begin":17782,"end":17785},"obj":"27667687"},{"id":"29375564-26926996-34934005","span":{"begin":18065,"end":18068},"obj":"26926996"},{"id":"29375564-27926861-34934006","span":{"begin":18282,"end":18285},"obj":"27926861"},{"id":"29375564-24315620-34934007","span":{"begin":18349,"end":18352},"obj":"24315620"},{"id":"29375564-17592090-34934008","span":{"begin":18675,"end":18678},"obj":"17592090"},{"id":"29375564-25779542-34934009","span":{"begin":19041,"end":19044},"obj":"25779542"},{"id":"29375564-25814686-34934009","span":{"begin":19041,"end":19044},"obj":"25814686"},{"id":"29375564-27340270-34934009","span":{"begin":19041,"end":19044},"obj":"27340270"},{"id":"29375564-21527743-34934010","span":{"begin":19359,"end":19362},"obj":"21527743"},{"id":"29375564-19200056-34934010","span":{"begin":19359,"end":19362},"obj":"19200056"},{"id":"29375564-14670831-34934010","span":{"begin":19359,"end":19362},"obj":"14670831"},{"id":"29375564-20703492-34934011","span":{"begin":20397,"end":20400},"obj":"20703492"},{"id":"29375564-20703492-34934012","span":{"begin":20580,"end":20583},"obj":"20703492"},{"id":"29375564-24485636-34934013","span":{"begin":20585,"end":20588},"obj":"24485636"},{"id":"29375564-17478729-34934014","span":{"begin":20771,"end":20774},"obj":"17478729"},{"id":"29375564-22842069-34934015","span":{"begin":20947,"end":20950},"obj":"22842069"},{"id":"29375564-21070867-34934016","span":{"begin":21108,"end":21111},"obj":"21070867"},{"id":"29375564-12086926-34934017","span":{"begin":21318,"end":21321},"obj":"12086926"},{"id":"29375564-27791119-34934018","span":{"begin":21698,"end":21701},"obj":"27791119"},{"id":"29375564-17307368-34934019","span":{"begin":23131,"end":23134},"obj":"17307368"},{"id":"29375564-16612592-34934020","span":{"begin":23136,"end":23139},"obj":"16612592"},{"id":"29375564-12788379-34934021","span":{"begin":23280,"end":23283},"obj":"12788379"},{"id":"29375564-28087471-34934022","span":{"begin":23470,"end":23472},"obj":"28087471"},{"id":"29375564-26294848-34934023","span":{"begin":24660,"end":24663},"obj":"26294848"}],"text":"Signaling Pathways and Molecules Modulating Cardiovascular Immune Response\n\nCytokines and Chemokines\nMacrophages are major contributors to the inflammatory response in the setting of cardiovascular diseases. They secrete diverse pro-inflammatory mediators (including cytokines, chemokines, ROS and nitrogen species, among others), and MMPs and they eventually die by necrosis or apoptosis. Dying macrophages release their lipid contents, which lead to the development of a pro-thrombotic necrotic core. The necrotic core is, in turn, a clue component of unstable plaques and contributes to their rupture and the consequent intravascular blood clot that causes MI and stroke. The main effect of cytokines/chemokines released by macrophages is the recruitment of different innate and adaptive immune cell populations into the atherosclerotic lesions (120, 121), thereby amplifying the inflammatory environment. For example, different inflammatory mediators such as IL-1, TNF and IFN-γ increase the expression of MCP-1 in macrophages (120, 122). Also, under inflammatory conditions macrophages produce different enzymes such as MMPs, cathepsins and serine proteases that degrade the extracellular matrix of the atherosclerotic plaque contributing to the pathogenesis of atherosclerosis (123). In this sense, IL-1 and TNF increase the expression of numerous MMPs, such as MMP-9, and their activity contribute to unstable plaque morphology (123).\nWhile initial reports indicated that IFN-γ prevented macrophage foam cell formation (124), subsequent studies demonstrate that this cytokine promotes foam cell formation through diverse mechanisms: enhancing the uptake of oxLDL/AcLDL through SRs (122, 125), diminishing efflux of cholesterol via inhibition of ABCA-1 transporter and ApoE expression (126, 127) and increasing ACAT-1 levels (126) in macrophage-derived foam cells. For example, it was recently reported that the axis IFN-γ/STAT1 mediates the uptake of acLDL/oxLDL by human macrophages in an extracellular signal-regulated kinase (ERK)-dependent signaling (125). The activity of IFN-γ as a pro-foam cell mediator has been supported by several in vivo studies (128).\nSince IFN-γ was identified as a pro-foam cell cytokine, different authors evaluated the effect of other pro-inflammatory cytokines. Studies using a murine macrophage cell line have shown that TNF inhibits the expression of SRs and the uptake of oxLDL (129). Regarding additional mechanisms, more recent evidence has suggested that TNF promotes foam cell formation in vitro by reducing the mRNA expression of ABCA-1/ABCG-1 (130), and intracellular lipid catabolism (131), and by enhancing the expression of ACAT-1 and the accumulation of cholesteryl ester (132). However, the role for TNF remains controversial since other studies have shown that TNF enhances ABCA-1 expression and the efflux of cholesterol in peritoneal macrophages stimulated with ApoA–I (133, 134) and since evidences from studies performed in murine models are also contentious (128).\nRegarding anti-inflammatory cytokines, it has been demonstrated that TGF-β decreases CD36/SR-A expression and the uptake of oxLDL in human macrophages (135, 136). Additional data emphasized this anti-foam cell effect by showing that TGF-β downregulates CD36/SR-BI mRNA levels in peritoneal macrophages (137) and increases ApoA–I and HDL-stimulated cholesterol efflux and ABCA-1 gene transcription in foam cells (138). Argmann et al. (139) demonstrated that TGF-β1 decreases LPL expression/activity and cholesteryl ester accumulation with a coincident increase in the expression of ABCG-1 in murine macrophages. Recently, it was reported that TGF-β diminishes LPL gene transcription (140) and increases the expression of ApoE (141). Moreover, other group has shown that CD4+ CD25+ regulatory T cells use TGF-β1 to inhibit the uptake of oxLDL and the expression of CD36/SR-A mRNA (142). These mechanisms evidence an anti-foam cell role for TGF-β1, although it is important to stress that TGF-β may promote development either of anti-atherosclerotic regulatory T cells or of T-helper 17 (Th17) cells, depending on factors in the local milieu. Therefore, TGF-β signaling in T cells could promote stabilization of atherosclerotic plaques through an IL-17-dependent pathway.\nThe effect of IL-10 on plaque formation has also been reported. This anti-inflammatory mediator downregulates the CD36 SR expression (143) and, in consequence, decreases the accumulation of cholesterol in human macrophages (144). In addition, two independent groups have reported anti-foam cell activity of IL-10. Indeed, they found that IL-10 enhances the uptake and efflux of cholesterol by human macrophages in vitro and reduces foam cell formation and atherosclerotic plaque progression in vivo (145, 146). Altogether, the results suggest that IL-10 counteract atherogenesis.\nBesides M1 and M2 macrophage profiles, additional phenotypes have been described within atherosclerotic lesion and they have become a topic of increasing relevance. In areas of hemorrhage, macrophages respond adaptively to heme acquiring an activation status that was designated hemorrhage-associated macrophages (Mhem). In vitro human blood-derived monocytes are induced to Mhem by stimulation with hemoglobin–haptoglobin complexes and it is dependent on an autocrine action of secreted IL-10 (147, 148). This macrophage profile prevents foam cell formation and has antioxidant functions. Other study showed that oxPL products that accumulate in atherosclerotic lesions induce another phenotype designated as Mox. Data indicate that Mox macrophages are characterized by high expression of heme oxygenase-1 and it comprises approximately 30% of all macrophages in established atherosclerotic lesions in experimental models (149). In addition, CXCL4, a chemokine released by activated platelet, was demonstrated to prevent monocytes/macrophages apoptosis and promote its differentiation into a phenotype called “M4” (MMP7+ S100A8+ CD68+). M4 macrophage was described in human coronary atherosclerotic plaques ex vivo and postmortem (150, 151). The prevalence of this macrophage subset is higher in patients with severe coronary artery disease and there is a significant correlation between the accumulation of M4 macrophages within the intima and plaque destabilization, which is independent of the overall number of macrophages in the vascular wall (150).\nRecently, several groups have published that IL-17A-stimulated macrophages are suggested to constitute a new macrophage population. Barin et al. (152) showed that IL-17A induces activation of primary macrophages in a unique profile of cytokines and chemokines, including IL-12p70, GM-CSF, IL-3, IL-9, CCL4 and CCL5, that can be distinguished from previously characterized macrophage activation states. Moreover, another work showed that IL-17A induces a pro-inflammatory transcriptome in human monocytes/macrophages, including cytokines such as IL-1α and IL-6, chemokines like CCL2, CCL8, CCL20, CXCL1, CXCL2 and CXCL6, genes involved in oxidative stress, upregulation of CD14, CD163 levels and downregulation of genes associated with T cell costimulation. By comparing the entire transcriptomes of M1, M2 and M4 macrophages, authors confirm that IL-17A-treated macrophages are a unique polarization subset (153). On the other hand, it was reported that IL-17 also can strongly amplifies human monocytes/macrophages differentiation toward M2c profile, making it resistant to apoptosis and promoting its MerTK-dependent clearance of apoptotic bodies (154). M2c cells (CD14bright CD16+ CD163+ MerTK+) are a subset of alternatively activated macrophages induced by M-CSF plus IL-10 or glucocorticoids and they are involved in inflammation resolution through phagocytosis of early apoptotic neutrophils and anti-inflammatory cytokines production (155). In contrast with the mentioned effects of IL-17, authors demonstrated that both IFN-γ/Th1 environment and IL-4-chronic exposure impair glucocorticoid and IL-10-induced M2c macrophage differentiation and also alters phagocytosis function of stablished M2c macrophages by reducing MerTK levels and promoting its apoptosis. Thus, this work indicates that Th17 environment orchestrates the resolution of innate inflammation through expansion of M2c regulatory macrophages.\nAn important point to note is that in a predominantly Th17 environment, monocytes/macrophages have a key role in the IL-17-induced inflammatory responses. In an experimental murine autoimmune myocarditis (EAM) model, the genetic ablation of IL-17RA alters the recruitment of monocytes/macrophages, which is the most numerous component of the inflammatory infiltrate and it is implicated in the cardiac damage (152). In concordance, other work shows that IL-17A promotes cardiac infiltration of Ly6Chigh monocytes by inducing fibroblasts to produce high levels of cytokines and chemokines. Particularly, GM-CSF production by IL-17-stimulated cardiac fibroblasts drives differentiation of infiltrating monocytes into an even more inflammatory phenotype which further intensifies the myocarditis. Experiments performed in IL17RAKO mice or depletion of Ly6Chigh monocytes/macrophages demonstrates the involvement of these innate cells in the inflammatory dilated cardiomyopathy (156). On the other hand, in an advanced atherosclerotic model in ApoEKO mice, in vivo IL-17A blocking markedly prevents atherosclerotic lesion progression and improves its stability by reducing inflammatory burden and monocyte infiltration (153). Moreover, the CCL2 and CCL5 production were significantly reduced in those IL-17A mAb-treated mice. In vitro experiments demonstrated that IL-17A induces adhesion of human monocytes, adhesion and rolling of platelets on endothelial cells. Other studies performed in early stages of atherosclerosis demonstrate that IL-17/IL-17RA axis has a pro-atherogenic role by promoting early monocyte recruitment into plaque through CCL5 production, endothelial VCAM-1 expression and increasing CXCL1 expression on the vessel lumen (157–159).\n\nPurinergic Signaling\nPurinergic system has been recognized as a main pathway to regulate immune response and it is critical to prevent the collateral damage produced by the exacerbation of the immune response in several diseases including cardiac pathologies. In normal conditions, ATP is localized intracellularly where it is the main source of energy for cell functions and it accomplishes indispensable functions in cellular metabolism such as proliferation, migration, motility, biosynthesis and contraction functions within the cardiomyocytes. In addition to its metabolic function, ATP also acts as an important signaling molecule when it is released to the extracellular compartment. After it is released, the extracellular ATP hydrolysis is governed by the activity of two membrane ectoenzymes, the CD39 that catalyzes the phosphohydrolysis of ATP to ADO monophosphate (AMP) and CD73 that hydrolyzes AMP into ADO and inorganic phosphate (45). CD39 and CD73 expression on immune and vascular cells and their enzymatic activities play decisive role in the regulation of the magnitude and duration of purinergic signals that modulate cellular function in an autocrine or paracrine manner (160). These effects are mediated by two types of nucleotide/nucleoside receptors: P1 receptors activated by extracellular ADO and P2 receptors activated by ATP and other nucleotides such as ADP and AMP. The first group includes 4 ADO receptors (ADORA 1, ADORA 2A, ADORA 2B, and ADORA 3), and the second group includes several P2X and P2Y subtypes of receptors (161).\nPurinergic signaling is highly described in tumoral context (162) and in autoimmune diseases (163) but poorly studied in the context of cardiac pathologies. Given the high production rate of ATP and the turnover required to maintain its continuous mechanical work, disruption of heart tissue generates the release of high amounts of ATP to the extracellular space. Bonner et al. have reported that cardiomyocytes and erythrocytes do not detectably express the ATP catabolic machinery, but coronary endothelial cells were highly positive for CD39 under basal conditions and a low proportion of these cells express CD73. Furthermore, they stated that resident cardiac APCs and monocytes were the responsible of the first step of ATP degradation in the heart after ischemic injury because they were highly positive for the CD39 while CD73 was absent. Likewise, the dephosphorylating step of AMP to immunosuppressive ADO seems to take place on T lymphocytes and granulocytes because CD73 was mainly expressed in those populations (164).\nIn contrast to the pro-inflammatory action of ATP, ADO exhibits diverse immunoregulatory roles. Bonner and coworkers found that macrophages stimulated through ADORA 2A and ADORA 2B receptors with ADO undergo M2 phenotype in infarcted mice. In addition, in CD73KO mice there was augmented expression of microbicidal M1 genes, while the expression of M2 genes like arginase-1, IL-10, and TGF-β decreased. This monocyte imbalance toward inflammatory Ly6Chigh-expressing monocytes in mice lacking CD73 had been related to adverse myocardial healing and ventricular dilatation after MI (165). Furthermore, Haskó et al. reported that ADO diminish the oxidative burst by downregulating NO production and inhibit TNF release from monocytes and macrophages (166). A parallel mechanism has also been reported by Cain group in murine isquemia/reperfusion model where ADO pretreatment decreased myocardial TNF production (167). There is strong evidence that ADO can modulate leukocyte adhesion to vascular endothelium in vivo. In this sense, Koszalka et al. demonstrated that ADO is an important endogenous pathway to modulate the inflammatory vascular response. After a period of ischemia-reperfusion, they observed significant increase of leukocyte adherence to the vascular endothelium only in the CD73 mutant (168). It has also been suggested that purinergic signals play a role in cellular migration. Analysis of atherosclerotic lesions of P2Y6 KO mice revealed fewer macrophages, diminished RNA expression of IL-6 and VCAM-1, suggesting that deficiency in this ATP receptor limits atherosclerosis and plaque inflammation (169). Recently, it was reported that lack of P2X7 receptor resolute plaque destabilization by inhibiting inflammasome activation and consequently ameliorates experimental atherosclerosis. ATP binding to P2 receptors is crucial to NLRP3 assembly and the subsequent IL-1β release. P2X7 null mice had decreased amount of macrophages in the wound and, in consequence, smaller and less inflamed atherosclerotic lesions than respective control animals (170). Altogether, the data indicate that purinergic signaling can modulate macrophage polarization and infiltration; and that ADO is a key metabolite in suppressing inflammation following injury or infection.\nIn addition, ADO induces the production of vascular endothelial growth factor (VEGF) in human macrophages, associated with increased hypoxia inducible factor-1α (HIF-1α) expression, the main transcriptional inducer of VEGF in hypoxic milieu. VEGF promotes blood vessel formation, induces cell proliferation and initiates immune cell migration to stimulate vasculogenesis and angiogenesis (171). On the other hand, extracellular ATP contributes to tissue repair by the stimulation of P2X7 receptor and release of the pro-angiogenic factor VEGF (172). Summing up, activation of purinergic receptors in macrophages can promote wound healing and may be targeted to improve cardiac repair mechanisms.\n\nMetabolism\nThe heart changes its substrate preferences throughout the life, under physiological or pathological conditions. This metabolic flexibility allows it to adapt to environmental changes. However, shifts in fuel selectivity can become detrimental in the pathological heart (173).\nPathological cardiac hypertrophy is a common consequence of several cardiovascular diseases and is a maladaptive response to chronic stress, sometimes resulting in cardiac failure. Pathological hypertrophy involves a shift in fuel metabolism from fatty-acid oxidation to enhanced reliance on glucose. Increased glucose utilization is characterized by upregulation of glucose uptake and glycolysis concomitant with no change or a decrease in glucose oxidation, resulting in uncoupling of glucose uptake/oxidation metabolism. Nowadays, it is accepted that changes in mitochondrial function and cardiac metabolism precede heart dysfunction, indicating that metabolic remodeling is an early event in the development of cardiac diseases.\nRecent interest in understanding the impact of these fundamental metabolic changes on immune cell differentiation and function has yielded numerous studies examining the pathways and metabolites involved in driving protective immune responses. A key question that has emerged is whether differential metabolic activity (i.e., use of glycolysis versus mitochondrial respiration) is coincident with differentiation or if it is a direct driver of alterations in immune cell differentiation and function. Classically activated macrophages that promote inflammation utilize glycolysis and glutamine metabolism to generate large quantities of succinate which enhances inflammation and IL-1β production (174). In contrast, macrophages that play a role in tissue healing and inflammatory resolution increase mitochondrial lipid oxidation (175). A key differentiator between these metabolic phenotypes may arise in the mitochondria, where rather than engaging in conventional electron transport, altered electron flow through the succinate dehydrogenase complex promotes ROS generation and inflammation (176). Enhanced glycolysis in coronary artery disease patients drives mitochondrial ROS production, resulting in pyruvate kinase M2 (PKM2) assembly and translocation to the nucleus. It then phosphorylates and activates STAT3 to promote the production of the cytokines IL-1β and IL-6 (177). Indeed, the increased activation of glycolytic pathway was associated with epigenetic changes in monocyte-derived macrophages describing a new mechanism to explain trained immunity in human innate immune cells (178).\nDiabetes is a major risk factor for cardiovascular diseases (179). As such it may lead to heart failure indirectly, by promoting the development of coronary artery disease. However, it is now known that diabetes may cause heart failure also by eliciting a direct detrimental impact on the myocardium leading to the development of cardiac hypertrophy, diastolic and systolic dysfunction (180). The clinical condition associated with the spectrum of cardiac abnormalities induced by diabetes is termed diabetic cardiomyopathy. High glucose levels and dyslipidemia directly induce the upregulation and secretion of cytokines, chemokines and adhesion molecules in immune cells by modulating multiple signaling pathways that converge toward NF-κB signaling (181–183). Activation of the renin–angiotensin–aldosterone system and accumulation of advanced glycation end-products (AGE) and DAMPs molecules like extracellular high-mobility group box-1 protein (HMGB1), also represent key mechanisms that mediate inflammatory response in the diabetic heart primarily by acting TLRs (184–186). Following these initial molecular events, leukocytes infiltrate the myocardium and perpetuate the inflammatory process through secretion of cytokines and pro-fibrotic factors and by increasing the production of ROS. Mediators resulting from this inflammatory cascade, in turn, modulate specific intracellular signaling mechanisms in cardiac cells causing hypertrophy, mitochondrial dysfunction, ER stress and cell death fibroblast proliferation and collagen production. In addition, inflammatory factors may affect myocardial metabolic processes and interfere with cardiomyocyte contractile properties. These abnormalities together promote the development of diabetic cardiomyopathy.\nDiabetes-associated metabolic derangements can directly induce cytokine expression and release from cardiac cells. It was previously shown that hyperglycemia augmented the expression of HMGB1 in isolated cardiomyocytes, macrophages and cardiac fibroblasts, thereby activating the MAPK and NF-κB pathways and by inducing TNF and IL-6 secretion (187). Inhibition of HMGB1 decreased myocardial inflammation and fibrosis in a murine model of type 1 diabetes and protected diabetic animals in response to postinfarction remodeling (187, 188).\nAn increase in circulating lipids may also contribute to cardiac inflammation in diabetes. Fatty acids activate TLR4 that strongly promotes inflammation through the NF-κB pathway (189). Mice with a TLR4 gene deletion demonstrate improved cardiac function and reduced cardiac intracellular lipid accumulation in response to streptozotocin-induced diabetes (190). Fatty acids induce the subsequent expression of IL-6, TNF and IL-1, which can be reversed by peroxisome proliferator-activated receptor-γ, β/δ (PPAR-b/d) (191). Previous work showed that hyperglycemia and high circulating levels of lipids promote inflammation through the activation of protein kinase C (PKC), which activates MAPK pathway thereby inactivating IkB (192).\nThe challenge to improve cardiovascular disorders therapy by modulating metabolic pathways must take into account potential off-target effects on other cells and tissues. In these sense, by exploiting the intrinsic capacity of monocytes and macrophages to take up foreign particles, the use of nanoparticle formulations might be one option to achieve targeting specificity (193).\n\nOxidative Stress\nOxygen is the molecular source of the production of several molecules that damage vital tissues. Reactive oxygen and nitrogen species (RONS) are continuously produced as by-products of the reaction leading to energy production through the mitochondrial and microsomal electron-transport chains. In phagocytes, the oxidative bursts and enzyme systems such as xanthine oxidase and cytochrome P-450 oxidase are the endogenous sources of RONS. While physiological levels of RONS are crucial for cell function, excessive RONS levels trigger oxidative stress. Oxidative stress may damage clues molecules (proteins, lipids, DNA) and could, eventually, conducted to cell death. To prevent oxidative stress, antioxidants have evolved to protect biological systems against RONS-induced damage. Oxidative stress resulting from uncontrolled production of RONS that exceeds the antioxidant capacity, exacerbate atherosclerosis; since it induces endothelial dysfunction by impairing the bioactivity of endothelial NO and promotes leukocyte adhesion, enhances inflammation and thrombosis.\nNumerous reports demonstrated that increased oxidative stress is associated with disturbances in cardiovascular diseases. In this sense, it was reported an overproduction of superoxide anion by cardiac dysfunctional mitochondria or increased production of RONS from non-mitochondrial sources in experimental models of type 1 diabetes (194, 195). The augmented production of RONS induces maladaptive cardiac response causing cardiac cells death contributing to cardiovascular disease (196). An excessive oxidative stress has been associated with increased cardiac cell apoptosis, as was evidenced by TUNEL+ cells and caspase 3 activation in T. cruzi-infected cardiac tissue (44). In this sense, high oxidative state has been associated with amplified lipid and DNA damage and the consequent cardiac cell injury and death. Therapeutic treatment that promotes RONS regulation have been shown to be effective in reducing cardiovascular dysfunction.\nIt is known that increased oxidative stress induces modifications of LDL, generating DAMPs that are detected by TLRs on different immune cell populations, mainly monocytes/macrophages. A variety of mechanisms mediated by enzymatic (such as 12/15-lipoxygenase and myeloperoxidase) or no-enzymatic redox reaction (such as RONS) boost LDL oxidation in the artery wall. The level of LDL oxidation modulates the immune system, when cells were treated with low oxLDL, the expression level of CD86, a marker for M1 macrophages, increases compared with that in cells treated with high oxLDL. In contrast, the expression level of the marker for M2 macrophages, CD206, significantly increases in cells treated with high oxLDL compared with that in cells treated with native LDL or low oxLDL. These results indicate that the degree of LDL oxidation affects the differentiation of monocytes into different subtypes of macrophages (197). Further research is needed to assess the functional consequences of oxidation processes on human macrophage behavior.\n"}