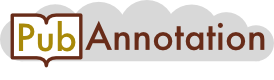
PMC:4996398 / 9392-24840
Annnotations
2_test
{"project":"2_test","denotations":[{"id":"27600217-16056655-69475892","span":{"begin":1600,"end":1602},"obj":"16056655"},{"id":"27600217-1698311-69475893","span":{"begin":2847,"end":2849},"obj":"1698311"},{"id":"27600217-15762675-69475894","span":{"begin":3371,"end":3373},"obj":"15762675"},{"id":"27600217-15762675-69475895","span":{"begin":3737,"end":3739},"obj":"15762675"},{"id":"27600217-15762675-69475896","span":{"begin":4344,"end":4346},"obj":"15762675"},{"id":"27600217-18600930-69475897","span":{"begin":4616,"end":4618},"obj":"18600930"},{"id":"27600217-18587846-69475898","span":{"begin":5647,"end":5649},"obj":"18587846"},{"id":"27600217-15762675-69475899","span":{"begin":6060,"end":6062},"obj":"15762675"},{"id":"27600217-11219714-69475900","span":{"begin":6066,"end":6068},"obj":"11219714"},{"id":"27600217-18316487-69475901","span":{"begin":6069,"end":6071},"obj":"18316487"},{"id":"27600217-14751730-69475902","span":{"begin":7126,"end":7128},"obj":"14751730"},{"id":"27600217-22125349-69475903","span":{"begin":7245,"end":7247},"obj":"22125349"},{"id":"27600217-19201462-69475904","span":{"begin":8460,"end":8462},"obj":"19201462"},{"id":"27600217-21193068-69475905","span":{"begin":8548,"end":8550},"obj":"21193068"},{"id":"27600217-11587588-69475906","span":{"begin":8713,"end":8715},"obj":"11587588"},{"id":"27600217-22336294-69475907","span":{"begin":8716,"end":8718},"obj":"22336294"},{"id":"27600217-22281421-69475908","span":{"begin":9011,"end":9013},"obj":"22281421"},{"id":"27600217-21334064-69475909","span":{"begin":9461,"end":9463},"obj":"21334064"},{"id":"27600217-18540674-69475910","span":{"begin":9464,"end":9466},"obj":"18540674"},{"id":"27600217-21112626-69475911","span":{"begin":9467,"end":9469},"obj":"21112626"},{"id":"27600217-9916770-69475912","span":{"begin":10088,"end":10090},"obj":"9916770"},{"id":"27600217-9916770-69475913","span":{"begin":10463,"end":10465},"obj":"9916770"},{"id":"27600217-12218178-69475914","span":{"begin":10466,"end":10468},"obj":"12218178"},{"id":"27600217-11857427-69475915","span":{"begin":11812,"end":11814},"obj":"11857427"},{"id":"27600217-11857427-69475916","span":{"begin":13155,"end":13157},"obj":"11857427"},{"id":"27600217-25067913-69475917","span":{"begin":13158,"end":13160},"obj":"25067913"},{"id":"27600217-25067913-69475918","span":{"begin":13293,"end":13295},"obj":"25067913"},{"id":"27600217-19126683-69475919","span":{"begin":13619,"end":13621},"obj":"19126683"},{"id":"27600217-15376189-69475920","span":{"begin":15442,"end":15444},"obj":"15376189"}],"text":"2. Alginate\nCommercially available alginates are extracted from harvested brown seaweeds. Significant amounts can also be produced by fermentation of bacteria, but this technology is not yet commercialized and will not be presented herein. The annual production of algal alginates is estimated to be approximately 38,000 tons worldwide and the largest volumes go to the food and pharmaceutical industry [16]. Alginates are also used as biomaterials in biomedical products for human use which are already on the market or in clinical trials. Such applications include wound healing, a bone graft substitute for spine fusion, cell therapy, and augmentation of the left ventricle wall for patients with dilated cardiomyopathy [17].\n\n2.1. Alginate Structure, Chemistry and Purity\nAlginates are polysaccharides which consist of linear (unbranched) 1,4 linked residues of β-d-mannuronic acid (M) and its C5-epimer α-l-guluronic acid (G) (Figure 1). The alginate molecular structure contains blocks of consecutive G or M monomers (-GGG- or -MMM-) or blocks of alternating monomers (-MGMG-). The G content of most algal alginates varies between 30% and 70%. The blocks vary considerably in length and distribution depending on from what species and part of the seaweed the alginate is extracted. The chemical composition and distribution of blocks in the alginate molecule play a major role in their capability of forming ionic gels.\nFigure 1 The structure of alginate shown as the segment of ..MMGG.. residues [18]. Epimerisation of the M residues changes the conformation of the sugar from 4C1 to 1C4 [19,20]. At neutral pH alginate has a polyanionic character due to the pKa values d-mannuronic and l‑gulronic acid of 3.38 and 3.65, respectively [21]. Hence, acidification below pKa leads to insoluble alginic acid, whereas alginate molecules in solution have an extended random coil conformation due to intramolecular electrostatic repulsion between neighboring negative charges. This results in highly viscous solutions of alginate even at low concentrations where the viscosity is influenced by the ionic strength, temperature and molecular weight [21].\nCommodity alginates, while having similar physicochemical properties, may contain contaminants inducing adverse cell reactions or undesired and uncontrolled cell to matrix interactions. Cells do not have receptors that recognize alginates and regular commercially available alginates can be considered as inert if they are of ultrapure quality. Impurities that should be considered and controlled in alginates for biomedical applications are presented in ASTM F 2067 and relate to the level of endotoxins, protein contaminants, elemental impurities and microbial bioburden [22]. The presence of residual endotoxins will, for example, interact with the liposolysaccharide (LPS) receptor CD14 [23]. CD14 is involved in different cell signaling pathways related to management of sepsis and can induce secretion of cytokines and upregulation of adhesion molecules. To ensure consistent cellular behavior in the presence of alginate biomaterials, the use of well-characterized and highly purified alginates is essential.\n\n2.2. Alginate Hydrogels\n\n2.2.1. Ionic Gelation\nAlginates have high affinity for alkaline earth metals and ionic hydrogels can be formed in the presence of divalent cations (except Mg2+) [21,24,25,26,27]. Chelation of the gel-forming ion occurs between two consecutive residues (Figure 2A) and an intermolecular gel network is formed as a result of a cooperative binding of consecutive residues in different alginate chains (Figure 2B). The G-blocks are the key structural elements in alginate hydrogels, but also alternating blocks may contribute to gel formation [27]. The different junction zones in an alginate gel are presented in Figure 2C. Alginates have different affinity for divalent cations in an increasing manner as Ca2+\u003cSr2+\u003cBa2+ [28]. The selection of type and amount of gel forming ion will influence the resulting properties of the hydrogels and can be utilized as an important tool to optimize elasticity, swelling and stability. Additionally, gel properties can be tuned by the selection of type and concentration of alginate. In general, gel elasticity, porosity and stability increases by increasing G-content, length of G-blocks and molecular weight [27,29].\nThe nanoscale porosity of an alginate gel network is tunable and in the range of 5–200 nm [29]. This will enable cellular access to nutrients and removal of waste products and synthesized products such as insulin, dopamine, endostatin and nerve growth factors [30,31].\nIonically gelled alginate can be dissolved by treatment with chelating agents for divalent cations such as citrate and ethylenediaminetetraacetic acid (EDTA) or hexametaphosphate [14]. This enables gentle and fast release of cells entrapped in alginate hydrogels for further downstream processing such as flow cytometry.\nIonic cross-linking between multivalent cations and alginate takes place instantaneously. Gels are, therefore, not easily prepared by mixing these two components directly. Two main techniques have been developed to provide controlled introduction of gelling ions to alginates with a subsequent formation of a gel. The techniques are referred to as diffusion gelation and internal gelation.\nDiffusion gelation is characterized by allowing gelling ions to diffuse from a large outer reservoir into an alginate solution. This is commonly used for preparation of hydrogel beads which will be formed instantaneously when an alginate solution is dripped into a solution containing gelling ions (usually CaCl2) [32]. This technique is described in more detail in Section 3.1.\nInternal gelation is characterized by a controlled release of gelling ions from an inert source that is dissolved or suspended within the alginate solution. Examples of sources of gel forming ions include carbonate salts (SrCO3 or CaCO3), calcium EDTA, calcium citrate, calcium sulfate (CaSO4), calcium alginate (Ca-alginate) and calcium gluconate [27,33,34,35,36]. Release of gel forming ions can be induced by a change in pH by, for example, incorporating the slowly hydrolyzing glucono-δ-lactone (GDL), limited solubility of a calcium salt source (Ca-alginate, CaSO4) and/or presence of chelating agents [37]. Compared to the method of diffusion gelation, the gelling ions are here released in a controlled fashion and over a period of time from sources distributed throughout the alginate. A molar ratio between GDL and SrCO3/CaCO3 of 2:1 will provide neutral gels [38]. Examples where this technique is utilized are described in more detail in Section 3.2 and Section 3.3.\nFigure 2 Four consecutive G-residues from two chain segments of one or two alginate molecules creating a binding site for divalent cations (A). Formation of an intermolecular network of alginate molecules formed in presence of gelling ions such as Ca2+ (B). Gelling ions organized in alternative junction zones (C) [18].\n\n2.2.2. Covalent Gelation\nCovalently cross-linked hydrogels can be prepared from chemically modified alginates [39,40]. To better control the physical properties of alginate gels, covalent cross-linking has been broadly investigated [41]. A covalently cross-linked hydrogel is chemically stable and can provide different modes of stress relaxation. By covalently conjugating methacrylate groups onto the alginate backbone, covalently cross-linked hydrogels can be prepared in the presence of a photoinitiator and UV light. Cells will be evenly distributed throughout the hydrogel if the cells are suspended in the alginate solution prior to photocrosslinking [17,42]. Photocrosslinking has a number of different advantages. By comparison with ionic gelation, photocrosslinking allows formation of more stable alginate hydrogels independent of the level of gel forming ions and non-gelling ions [42]. Mechanical properties and biodegradation rates of a hydrogel can be adjusted by varying the degree of alginate methacrylation [43].\n\n2.3. Alginate Derivatives\nAlginates can be chemically functionalized to alter physicochemical and biological characteristics and properties. One example is mentioned in Section 2.2.2 related to preparation of covalently gelled matrices. One approach for tuning degradation of alginate hydrogels includes the use of covalently cross-linked methacrylated alginates where the linkages are hydrolytically degradable [43,44], or inclusion of linkages that can be cleavable by matrix metalloproteinases (MMP) [45]. Another approach to enhance depolymerization is by partial periodate oxidation where some of the residues along the chain are made degradable by β-elimination [46,47,48]. Other tunable properties are solubility, hydrophobicity, bioactivity etc. Due to the free hydroxyl and carboxyl groups distributed along the backbone, alginate is a suitable candidate for chemical modification, and these are presented in reviews by Yang et al. [49] and Pawar and Edgar [50]. The most important modifications of alginate hydrogels for use in combination with cells are related to the ability to tailor and control the type and degree of cell interactions. This can be achieved by covalently conjugating alginate with heparin binding peptides (HBP) or peptide sequences found in ECM proteins. Cell matrix interactions can thereby be enabled via the non-integrin receptor syndecan for HBP or integrins for ECM peptides [51,52,53,54]. ECM peptide coupled alginates will be discussed in more detail below.\n\n2.3.1. Peptide-Coupled Alginates\nThe ability to modify the chemical and physical properties of alginate is a highly compelling incentive for using alginates in tissue engineering and regenerative medicine applications [55]. Cell attachment peptides, especially the sequence RGD (arginine-glycine-aspartic acid), have been shown to improve cellular adaptability to matrices, and such is also the case with alginate. Using aqueous carbodiimide chemistry, alginate can be modified by covalently grafting peptide sequences to the alginate molecule [56]. The interaction of cells with biomaterials is often mediated through cellular receptors that recognize adhesion molecules at material surfaces. One common example of such an adhesion ligand is the RGD peptide sequence, and it has been shown that RGD-coupled alginates (Figure 3) have the ability to initiate biological interactions between alginate hydrogels and cells [56,57].\nFigure 3 Chemical structure of RGD-alginate (arginine-glycine-aspartic acid conjugated to sodium alginate) (prepared using ISIS Draw). As cells do not have receptors that recognize alginate, proliferation and differentiation of some cells within an alginate hydrogel do require signaling molecules and matrix interaction. Studies using an alginate-based scaffold for 3D cell culture (NovaMatrix®-3D) have shown the importance of the presence of the RGD peptide sequence for cell proliferation. Table 1 lists various established cell lines grown in the NovaMatrix®-3D cell culture system and if RGD was required for cell proliferation. The commercial RGD-alginate NOVATACH MVG GRGDSP was used at a concentration of 120 µM RGD obtained by dilution with non-RGD alginate. (NOVATACH MVG GRGDSP contains 0.02–0.04 µmole RGD/mg alginate, and the exact value is reported on the certificate of analysis.). For example, the non‑tumorigenic cell lines C2C12 (mouse myoblast) and MDCK (canine kidney) did not proliferate in the alginate hydrogel during the first few weeks of culture. However, if RGD was available through incorporation of RGD-alginate, then cells displayed rapid proliferation. The data [58] on cell proliferation of C2C12 myoblast cells corroborated the importance of RGD peptide on cell proliferation found by Rowley and Mooney [59]. Moreover, after several weeks of culture, the C2C12 cells started to proliferate and fuse into multinucleated myofibrils as seen in Figure 4A. Figure 4 shows confocal images of the spatial organization of different cell types that were vital stained with Calcein AM (Molecular Probes) while inside the gel [60].\nFigure 4 Cellular organization within the alginate gel obtained by confocal microscopy of vital stained (Calcein AM) cells. Panels A–D and E–F show cells cultured without and with RGD-alginate, respectively. C2C12: murine myoblasts, HT-29: human colorectal adenocarcinoma cells, V79-379A: Chinese hamster lung fibroblasts, L1210: murine leukemia suspension cells, NIH:OVCAR-3: human ovarian adenocarcinoma cells, HCT 116: human colorectal carcinoma cells. Magnification ×100, scale bar 100 µm. Adapted from [60].\nmicroarrays-04-00133-t001_Table 1 Table 1 The importance of RGD (120 µM) as RGD-coupled alginate for cell proliferation in NovaMatrix®-3D (reproduced from www.novamatrix-3D.com) [58]. 1 Cell proliferation evaluated during three weeks of culture (NOVATACH is a tradename for RGD-alginate from FMC BioPolymer). 2 NOVATACH MVG GRGDSP influence on cell morphology. 3 Cell proliferation was accelerated in presence of NOVATACH MVG GRGDSP. Increased cell proliferation has been seen by increasing density of RGD [59,61]. Variations in nanoscale organization of RGD was shown to influence adhesion, proliferation and differentiation of preosteoblasts [61], which can be obtained by diluting RGD-alginates with different RGD densities along the chain with regular alginate. The peptide densities in alginate hydrogels can be optimized dependent on the cell culture system, but they are mainly comparable to the RGD density of commonly used biological matrices. Fischbach et al. [62] presented the number of RGD molecules in tumors associated ECM to be 8.6 × 1016 per mL matrix, which equals 143 µM. In the literature, the reported densities of peptides are presented in different ways such as µg peptide/mg polymer, µmole peptide/mg polymer, fmole/cm2 (2D), degree of substitution based on monomer or molecule (chain).\nThe presentation of the RGD peptide sequence has been shown to be an important cue regulating cellular behavior. This was demonstrated by, for example, Hsiong et al. [63] who compared the behavior of MC3T3-E1 preosteoblasts, human bone marrow stromal cells (hBMSCs) and D1 stem cells encapsulated in alginate hydrogels containing either linear G4RGDSP or cyclic G4CRGDSPC. The results showed that linear RGD densities promoted osteogenic differentiation of committed cells (MC3T3-E1 preosteoblasts), but not for the hBMSCs and the stem cells. However, osteoprogenitor differentiation of all cells was seen in the gels presenting the higher-affinity cyclic form of the adhesion ligand. As presented in a review of Perlin et al. [64], the affinity and selectivity for different types of integrin receptors varies among cell types and is dependent on the flanking amino acids of RGD, the conformation and the length of the peptide sequence. Some integrins are cell type specific, whereas others mediate different cellular behaviors such as adhesion and migration. Hence, the type of optimal RGD containing peptide sequence and RGD density will vary depending on the study, which must be seen in combination with the physical properties of the peptide-containing alginate. Other extracellular matrix peptide sequences in addition to RGD have been found to be of interest to couple to alginate such as REDV (found in fibronectin), YIGSR (found in laminin) and VAPG (found in elastin) [51,65].\n\n"}