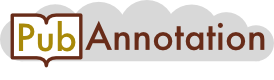
PMC:4996398 / 24840-45129
Annnotations
2_test
{"project":"2_test","denotations":[{"id":"27600217-19049902-69475921","span":{"begin":989,"end":991},"obj":"19049902"},{"id":"27600217-11857427-69475922","span":{"begin":1243,"end":1245},"obj":"11857427"},{"id":"27600217-20130138-69475923","span":{"begin":1246,"end":1248},"obj":"20130138"},{"id":"27600217-19800626-69475924","span":{"begin":1249,"end":1251},"obj":"19800626"},{"id":"27600217-20418863-69475925","span":{"begin":1252,"end":1254},"obj":"20418863"},{"id":"27600217-19705957-69475926","span":{"begin":1581,"end":1583},"obj":"19705957"},{"id":"27600217-22417620-69475927","span":{"begin":1754,"end":1756},"obj":"22417620"},{"id":"27600217-20574984-69475928","span":{"begin":1898,"end":1900},"obj":"20574984"},{"id":"27600217-25769043-69475929","span":{"begin":1901,"end":1903},"obj":"25769043"},{"id":"27600217-20418863-69475930","span":{"begin":2504,"end":2506},"obj":"20418863"},{"id":"27600217-19556500-69475931","span":{"begin":2507,"end":2509},"obj":"19556500"},{"id":"27600217-19539367-69475932","span":{"begin":2510,"end":2512},"obj":"19539367"},{"id":"27600217-16923388-69475933","span":{"begin":2513,"end":2515},"obj":"16923388"},{"id":"27600217-19556500-69475934","span":{"begin":2623,"end":2625},"obj":"19556500"},{"id":"27600217-20418863-69475935","span":{"begin":2923,"end":2925},"obj":"20418863"},{"id":"27600217-19556500-69475936","span":{"begin":2996,"end":2998},"obj":"19556500"},{"id":"27600217-6776628-69475937","span":{"begin":3205,"end":3207},"obj":"6776628"},{"id":"27600217-8516335-69475938","span":{"begin":8726,"end":8728},"obj":"8516335"},{"id":"27600217-16373911-69475939","span":{"begin":8729,"end":8731},"obj":"16373911"},{"id":"27600217-19549731-69475940","span":{"begin":8732,"end":8734},"obj":"19549731"},{"id":"27600217-14531571-69475941","span":{"begin":9324,"end":9326},"obj":"14531571"},{"id":"27600217-11135548-69475942","span":{"begin":9327,"end":9329},"obj":"11135548"},{"id":"27600217-11559558-69475943","span":{"begin":9330,"end":9332},"obj":"11559558"},{"id":"27600217-20842001-69475944","span":{"begin":9427,"end":9429},"obj":"20842001"},{"id":"27600217-24142904-69475945","span":{"begin":9430,"end":9432},"obj":"24142904"},{"id":"27600217-24626259-69475946","span":{"begin":9459,"end":9461},"obj":"24626259"},{"id":"27600217-20690708-69475947","span":{"begin":9462,"end":9464},"obj":"20690708"},{"id":"27600217-24880568-69475948","span":{"begin":10292,"end":10294},"obj":"24880568"},{"id":"27600217-11759015-69475949","span":{"begin":10316,"end":10318},"obj":"11759015"},{"id":"27600217-8021988-69475950","span":{"begin":10319,"end":10321},"obj":"8021988"},{"id":"27600217-20823504-69475951","span":{"begin":11725,"end":11728},"obj":"20823504"},{"id":"27600217-15247778-69475952","span":{"begin":11855,"end":11858},"obj":"15247778"},{"id":"27600217-18070820-69475953","span":{"begin":11969,"end":11972},"obj":"18070820"},{"id":"27600217-12182310-69475954","span":{"begin":11973,"end":11976},"obj":"12182310"},{"id":"27600217-16765436-69475955","span":{"begin":12293,"end":12296},"obj":"16765436"},{"id":"27600217-22095721-69475956","span":{"begin":12312,"end":12315},"obj":"22095721"},{"id":"27600217-12226863-69475957","span":{"begin":12337,"end":12340},"obj":"12226863"},{"id":"27600217-16889515-69475958","span":{"begin":12354,"end":12357},"obj":"16889515"},{"id":"27600217-19640157-69475959","span":{"begin":12358,"end":12361},"obj":"19640157"},{"id":"27600217-23349734-69475960","span":{"begin":12781,"end":12784},"obj":"23349734"},{"id":"27600217-25093879-69475961","span":{"begin":15100,"end":15103},"obj":"25093879"},{"id":"27600217-23719889-69475962","span":{"begin":15341,"end":15344},"obj":"23719889"},{"id":"27600217-23260439-69475963","span":{"begin":15452,"end":15455},"obj":"23260439"},{"id":"27600217-20353253-69475964","span":{"begin":16183,"end":16186},"obj":"20353253"},{"id":"27600217-25047630-69475965","span":{"begin":16296,"end":16299},"obj":"25047630"},{"id":"27600217-25383549-69475966","span":{"begin":16300,"end":16303},"obj":"25383549"},{"id":"27600217-20353253-69475967","span":{"begin":16489,"end":16492},"obj":"20353253"},{"id":"27600217-25047630-69475968","span":{"begin":16886,"end":16889},"obj":"25047630"},{"id":"27600217-25383549-69475969","span":{"begin":16890,"end":16893},"obj":"25383549"},{"id":"27600217-24722236-69475970","span":{"begin":17177,"end":17180},"obj":"24722236"},{"id":"27600217-22336294-69475971","span":{"begin":17692,"end":17695},"obj":"22336294"},{"id":"27600217-24998183-69475972","span":{"begin":17895,"end":17898},"obj":"24998183"},{"id":"27600217-25005170-69475973","span":{"begin":18336,"end":18339},"obj":"25005170"},{"id":"27600217-8745321-69475974","span":{"begin":18666,"end":18669},"obj":"8745321"},{"id":"27600217-22180835-69475975","span":{"begin":19925,"end":19928},"obj":"22180835"},{"id":"27600217-16285253-69475976","span":{"begin":20283,"end":20286},"obj":"16285253"}],"text":"3. 3D Cell Culture\nThere are several approaches and adaptations of 3D cell culture for cell immobilization. One system will likely not fit all types of experiments or cell types, but as can be seen in this section, alginate provides a great toolbox for design and optimization. There is one major difference to be aware of when comparing different techniques for formation and evaluation of multicellular spheroids. This relates to whether the spheroids that are formed are monoclonal or polyclonal in origin. Spheroids of monoclonal origin are formed from a single proliferating cell as will be the situation for cells in most hydrogel systems. Spheroids of polyclonal origin arise from an aggregating cell population and will be the situation when using low attachment plates or hanging drop techniques.\nTechnologies for formation of scaffolds as an alternative to cell culture on 2D plastics include beads, fibers, membranes, meshes, foams or hydrogels of different shapes and sizes [1,66]. Even 2D cell culture onto different types of scaffolds show benefits over monolayers on plastics, and valuable information about cellular responses to matrix stiffness and incorporated cell signaling factors has been obtained from such experiments [59,67,68,69]. Four technologies of alginate hydrogels are presented in this section and include beads, delayed gelation systems, macroporous scaffolds and 3D printed scaffolds. Other technologies providing 3D cell culture in alginate scaffolds are honeycomb alginate scaffolds with an aligned pore structure for improved vascularization [70], scaffolds impregnated with magnetically responsive nanoparticles for stimulation of cells and induced organization of endothelial cells into capillary-like structures [71], and alginate scaffolds with controlled nucleation of hydroxyapatite generating a composite scaffold promising for bone tissue engineering [72,73].\nAlginate scaffolds for 3D cell culture can be tuned and optimized for a wide range of applications and cell types. In addition to tuning the interaction of cells to their surrounding hydrogel via peptide‑coupled alginates as described in Section 2.3.1, the elasticity of the gels can be controlled. By optimizing alginate concentration, type of alginate and selection of cross-linking technology (ionic or covalent) in addition to cross-linking density, alginate hydrogels can be made to match the elasticity of most types of tissue (Figure 5) and can be used to control stem cell differentiation [69,74,75,76]. The elasticity of alginate hydrogels can be made to match the elasticity of all types of soft tissue [28,74,77]. Huebsch et al. reported that bond formation between mammalian mesenchymal stem cells (mMSCs) and RGD peptide was regulated by both the density of available peptides (7.5–150 µM RGD concentration) and matrix rigidity (2.5–110 kPa) thereby demonstrating the importance of these two parameters [69].\nFigure 5 Elasticity scale of soft tissues. Adapted from reference [74]. Reprinted with permission from AAAS.\n\n3.1. Beads\nThe technique to immobilize cells, particularly pancreatic islet cells, in calcium alginate matrices was developed by Lim and Sun at the end of the 1970s [13]. By coating the alginate gel bead with polycations like poly-L-lysine, poly-L-ornithine, or chitosan, the strength of the surface coating as well as the capsule porosity can be controlled (Figure 6) [78].\nFigure 6 Encapsulation of cells with alginate. (From FMC internal archive.) One important characteristic of alginates is their very limited inherent cell adhesion and cellular interaction. This is an advantage for cell encapsulation applications, but can be a disadvantage for tissue engineering applications. However, alginate can be modified by the addition of cell attachment peptides or other biologically active molecules (see Section 2.3.1).\nEncapsulation in an alginate hydrogel has been shown to be a rapid, non-toxic, and versatile method for immobilization of macromolecules and cells. The creation of artificial organs by encapsulating cells or tissue is under study for treatment of a variety of diseases such as Parkinson’s disease, liver failure, hypocalcemia as well as chronic pain and, perhaps the most well-known example, an artificial pancreas for the treatment of diabetes (encapsulated pancreatic islets).\nMost methods for encapsulation of cells or tissue in alginate gels basically involve two main steps. The first step is the formation of an internal phase where the alginate solution containing biological materials is dispersed into small droplets. In the second step, droplets are solidified by gelling, or by forming a membrane at the droplet surface.\nBead size is one of the most important parameters of alginate gel beads and capsules in biomedical applications. The appropriate size will often be a compromise. The bead itself must be large enough to contain the biological material. Larger beads are also easier to handle during washing or other treatments. In many applications involving cells, the cells should be homogeneously distributed within the internal capsular matrix. When generating beads the desired mean size and acceptable size distribution should be accounted for. The size of the beads is mainly controlled by regulating the formation of the droplet.\nDroplet size is dependent upon several factors: the size of the material to be immobilized or encapsulated (i.e., single cells or cell aggregates such as pancreatic islets), the technique used to generate droplets (i.e., pipette or syringe, coaxial air flow, electrostatic generator, jet-cutter, etc.), the viscosity of the alginate solution, and the rate of alginate flow. Generally, for biomedical applications, droplet size is regulated to give a gelled bead having a diameter of \u003c200–1000 µm. Per unit volume, smaller beads yield a larger surface area to transplant volume, a ratio that results in enhanced survival of tissue due to better nutritional and oxygen supply. Various techniques can be used to form droplets, as described in more detail by Dulieu et al. [79]. These include: • Extrusion through a needle: Beads can be made by dripping an alginate solution from a syringe with appropriate diameter needle directly into a gelling bath. While this method does not require any instrumentation, the size and size distribution of the produced beads are difficult to control.• Coaxial air or liquid flow: The coaxial air jet system is a simple way of generating small beads (down to around 400 µm), although the size distribution will normally be larger as compared to an electrostatic system. In this system, a coaxial air stream is used to pull droplets from a needle tip into a gelling bath (Figure 7).• Electrostatic potential: An electrostatic potential can be used to pull droplets from a needle tip into a gelling bath. The primary effect on droplet formation by the electrostatic potential is to direct charged molecules to the surface of the droplet to counteract surface tension. Using this type of instrument, beads below 200 µm and with a small size distribution may be generated. The desired bead size is obtained simply by adjusting the voltage (electrostatic potential) of the instrument. The principle for making smaller beads by electrostatic potential bead generators is shown in Figure 7.• Vibrating capillary jet breakage: A vibrating nozzle generates drops from a pressurized vessel.• Rotating capillary jet breakage: Bead generation is achieved by cutting a solid jet of fluid coming out of a nozzle by means of a rotating cutting device. The fluid is cut into cylindrical segments that then form beads due to surface tension while falling into a gelling bath.\nFigure 7 Principle of electrostatic (left) and coaxial air flow (right) bead generators [80]. Encapsulation of living cells, a form of cell immobilization, is one application for producing artificial organs and cell therapy constructs. Here, cells are mixed with alginate (osmolality is adjusted) and then the alginate + cell solution is dripped (or extruded) into a bath of calcium chloride (Figure 6). Since the ionic cross-linking reaction is instantaneous, living cells are entrapped inside an alginate hydrogel bead. The porosity of the alginate bead is such that oxygen and nutrients can diffuse into the gel while cell products, such as proteins, can diffuse out of the gel. The hydrogel is, however, porous barrier to antibodies and immune cells such as macrophages. Furthermore, it is possible to implant his alginate hydrogel ”biofactory” into an animal or man where the implant can act as a continuous production system for, for example, insulin. Implantation studies into animals and diabetic patients [81,82,83] have shown that long-term functionality.\nSome examples of encapsulated cells are shown in Figure 8. These photomicrographs are not of the same magnification. The panel on the left shows encapsulated genetically engineered murine 3T3 cells. Encapsulated Human Embryonal Kidney (HEK) 293 cells are shown in the center panel. Pancreatic islets encapsulated in alginate gel beads are shown in the right panel. Cells producing the anti‑angiogenic protein endostatin have been encapsulated in ultrapure alginate and implanted into the brains of dogs being treated for spontaneous brain cancer [84,85,86]. Many other cell types have been immobilized in alginate such as adipose-derived stem cells [87,88], mesenchymal stem cells [89,90], and chondrocytes [91,92].\nFigure 8 Examples of encapsulated cells in alginate. (From FMC internal archive.) Clinical cell therapy applications of alginate immobilized cells such as the treatment of Parkinson’s disease as well as diabetes are available at the web sites of Living Cell Technologies [93,94].\n\n3.2. Delayed Gelation Systems\nDelayed gelation systems where gels are formed inside the body (in situ) allow implantation with less invasive surgery which is easier to deliver since they will exactly fill tissue voids and defects. The mixture of gel forming ions suspended or dissolved in an alginate solution is, along with internal gelation principles, the basis of delayed gelation. Examples include mixing of an alginate solution with a suspension of calcium alginate particles [95], calcium carbonate and GDL [96] or calcium sulfate [97,98].\nA promising approach for tissue engineering of hyaline cartilage is utilization of human mesenchymal stem cells (hMSCs). The most sufficient chondrogenic differentiation is obtained in 3D culture systems. A novel model system for 3D chondrogenic differentiation of hMSCs has recently been described [95]. Here, cells were entrapped in an alginate hydrogel formed as calcium ions diffused from calcium alginate particles subsequent to mixture with a solution of sodium alginate (see principle in Figure 9). This technology, also known as “self-gelling” alginate, allows homogeneous distribution of the cells within a hydrogel of defined size and shape. The study demonstrated how hMSCs were differentiated resulting in up-regulation of a large number of genes associated with hyaline chondrogenesis which, for example, may be used to repair potential lesions of hyaline cartilage [95].\nFigure 9 Principle of delayed gelling of a hydrogel made from alginate only [99]. By mixing a sodium alginate solution with a dispersion of insoluble calcium alginate, using for example connected syringes, a homogeneous hydrogel can be made. Upon mixing, gel forming ions rearrange between insoluble and soluble alginate molecules resulting in gel formation.\n\n3.3. Macroporous Scaffolds\nMacroporous scaffolds may allow larger constructs as the mass transfer of nutrients, oxygen and waste removal will be enhanced [100]. The structure does also have predefined dimensions and they can better withstand deformation compared to compact hydrogels [101]. Also, the pore size and porosity can be tuned and optimized for the specific application and cell type [102,103,104]. However, a major challenge with such scaffolds may be cell seeding efficiency and cell distribution, as the pores are often either too small to let cells in or too large to retain cells inside. A variety of approaches have been investigated to overcome this challenge utilizing, for example, cell seeding devices [105], bioreactors [106], centrifugal force [107] and vacuum [108,109].\nTwo alginate based macroporous systems for cell culture are available, AlgiMatrix® and NovaMatrix®-3D from Thermo Fisher Scientific/Life Technologies (Carlsbad, CA, USA) and FMC BioPolymer/NovaMatrix (Sandvika, Norway), respectively. Both systems are based on ionically gelled and dried macroporous scaffolds and are available as sterile discs pre-filled in different formats of standard cell culture well plates [77,110]. Both scaffolds turn into hydrogels upon rehydration following cell seeding. Further, cells can be stained and microscopically evaluated while entrapped, the gels can be dissolved for easy retrieval of cells and multicellular structures and their gel elasticity is tunable. The main differences between these sponge‑like matrices are the seeding technique and ability to control cell to matrix interactions. A concentrated suspension of cells in culture media is absorbed by the dry AlgiMatrix® scaffold as it is applied on the top surface and cells will be entrapped inside the porous structure. Centrifugation may be utilized to ensure a more homogeneous distribution of cells throughout the scaffold. For NovaMatrix®-3D, cells are applied on top of the scaffold suspended in an alginate solution dissolved in cell culture media. As shown in Figure 10, the foam structure is utilized as a scaffold and source of gel forming ions to induce gelation of the applied alginate solution. When the pores are filled with the alginate solution, a hydrogel is subsequently formed in situ. This technique ensures a cell seeding efficiency of about 100% and an even distribution of cells throughout the thickness of the gel. NovaMatrix®-3D cell culture kits are also available with RGD-coupled alginate which is added to the dry sponge together with the cells. See Section 3.2 for examples of cellular responses with or without RGD-coupled alginate.\nFigure 10 Schematic presentation of the steps for in situ gelation in macroporous alginate scaffolds. (A) Am alginate solution with cells is applied on top of a dry scaffold containing calcium ions, (B) rehydration of the scaffold by the alginate solution filling its pores and diffusion of calcium ions from the foam lamellas to the absorbed alginate, and (C) formation of a calcium cross-linked alginate hydrogel inside the pores of the foam. From reference [77].\n\n3.4. Alginate as a Bioink and 3D Bioprinting\n3D printing as a technology is available in industrial and home-use applications. The ability to construct customized three dimensional structures on demand using relatively simple materials is leading to a boon in manufacturing sectors. The application of 3D printing technology in the fields of tissue engineering and regenerative medicine has already begun [111]. Bioprinting uses biocompatible materials and cells to form a variety of 3D formats where cell function and viability are preserved within the printed construct. Various 3D bioprinting technologies can already form vascular-like tubes [112], artificial skin [113], cartilage [114], and a wide range of tissue constructs also including stem cells [115]. A public workshop was hosted by the U.S. Food and Drug Administration (U.S. FDA) in October of 2014 under the title “Additive manufacturing of medical devices: An interactive discussion on the technical considerations of 3D printing”. The workshop agenda, participants and presentations held at this workshop are available at the U.S. FDA web sites [116].\n3D bioprinting techniques such as ink-jet and extrusion have the need for biocompatible “inks”. Alginate has shown particular relevance as a bioink due to its compatibility with cells, ease in forming cross-linked hydrogels, and the ability to control biodegradation. Khalil and Sun demonstrate bioprinting of 3D tissue constructs using alginate and endothelial cells [117] and alginate stabilized with gelatin was a suitable matrix for 3D bioprinting of bone-related SaOS-2 cells [118,119]. Common to these reports is a high (\u003e80%) cell viability following bioprinting. These reports also show two different approaches in the design of alginate as a bioink. Khalil and Sun [117] use a multinozzle system that prints alginate + cells and overlays with calcium chloride in order to induce gelation. The addition of a low-melting gelatin together with an alginate solution forms a gel when the solution printed at 37 °C cools. Moreover, addition of a calcium poly phosphate salt or bioglass to the cell‑containing hydrogel led to enhanced biomineralization by SaOS-2 cells [118,119].\nUsing 3D printing technology and alginate as a bioink, Zhao et al. show the advantage of printing Hela cells to form an in vitro cervical tumor model in order to study disease pathogenesis and enable new anti-cancer drug discovery with a more relevant physiological disease model [120]. This report used gelatin together with alginate to initiate gelation prior to printing. The printed construct was further strengthened after printing by subsequent addition of a calcium salt solution. The authors included fibrinogen in the gelatin/alginate formulation to mimic ECM components. Printed HeLa cells formed spheroids which were shown to be more resistant to paclitaxel treatment than HeLa cells grown as a 2D cell culture.\nBy oxidizing alginate, a known technique to “build in” biodegradability [121], Jia et al. demonstrate the interaction of alginate viscosity and density on printability while biodegradability of printed scaffolds containing human adipose-derived stem cells was also described [122].\nOptimization of alginate for use in different printing technologies is, however, necessary. For inc-jet types of printing, droplet formation is impacted by alginate molecular weight, solution viscosity, monomer composition (if ionic cross-linking is to be used to form a gel), and purity which impacts on biocompatibility. Xu et al. studied the characteristics of the droplet formation process using alginate viscosity and shear rate [123]. Furthermore, Gasperini et al. present a bioprinting techniques based on electrohydrodynamic processes to jet droplets of alginate containing cells [124].\n\n3.5. Cryopreservation\nSimple cell and tissue preservation techniques have disadvantages including limited shelf-life, high cost, risk of contamination or generic drift [125]. A more tangable option is cryopreservation where cells are preserved by cooling them to low temperatures typically in liquid nitrogen (−196 °C). At such low temperatures, biological activities of the cells are effectivly stopped. These includes the biochemical reactions that would lead to cell death under normal conditions and damage caused by the formation of ice crystals. Cryopreservation provides a valuable means for storing cells and tissues for future use. However, certain drawbacks exist, including damage that occurs to the cells during the freezing and/or thawing processes and the need to culture the cells after thawing to ensure that they have recovered properly. Such drawbacks limit the value of cryopreserved cells, particularly in situations where it is desirable to use the cryopreserved cells immediately or shortly after they have been thawed. There exist several methods to deal which such problems. One alternative is to suspend the cells in an alginate solution prior to cryopreservation. The cells can then be encapsulated (see Section 3.2) after thawing and used for their desired purpose without the need to culture the cryopreserved cells. Alternatively, cells are entrapped in hydrogels before they are cryopreserved [126,127]. This method is based on the discovery that cryopreserved cells that have been thawed, immediately suspended in alginate, and after encapsulation remain viable and are ready to be used [128]. To obtain off-the-shelf availability, distribution and storage of constructs, sterility testing and quality control, preservation of cells and tissues is vital [129].\n"}