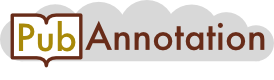
PMC:4996393 / 1234-7295
Annnotations
2_test
{"project":"2_test","denotations":[{"id":"27600215-23539594-69479488","span":{"begin":1488,"end":1489},"obj":"23539594"},{"id":"27600215-24608425-69479489","span":{"begin":1631,"end":1632},"obj":"24608425"},{"id":"27600215-18936473-69479490","span":{"begin":2830,"end":2831},"obj":"18936473"},{"id":"27600215-22365974-69479491","span":{"begin":3668,"end":3669},"obj":"22365974"},{"id":"27600215-24777629-69479492","span":{"begin":3670,"end":3672},"obj":"24777629"},{"id":"27600215-11360182-69479493","span":{"begin":5229,"end":5231},"obj":"11360182"},{"id":"27600215-20625992-69479494","span":{"begin":5508,"end":5510},"obj":"20625992"},{"id":"27600215-20534907-69479495","span":{"begin":5511,"end":5513},"obj":"20534907"},{"id":"27600215-19860827-69479496","span":{"begin":5514,"end":5516},"obj":"19860827"},{"id":"27600215-23913224-69479497","span":{"begin":5912,"end":5914},"obj":"23913224"},{"id":"27600215-19879857-69479498","span":{"begin":5915,"end":5917},"obj":"19879857"},{"id":"27600215-19396842-69479499","span":{"begin":5918,"end":5920},"obj":"19396842"},{"id":"27600215-24643969-69479500","span":{"begin":5927,"end":5929},"obj":"24643969"}],"text":"1. Introduction\nThe aim of future medicine is personalized treatment which is often characterized as providing the right patient with the right drug at the right dose at the right time. An increasing number of targeted adjuvant cancer therapies, i.e., any treatment given after primary therapy (e.g., surgery), is already available. An example for the success of such novel treatment strategies is the monoclonal antibody trastuzumab against the receptor tyrosine kinase HER2 [1]. Breast cancer and gastric cancer patients who will most likely respond to this therapy are identified before treatment by molecular tests, such as immunohistochemistry (IHC) and fluorescence in situ hybridization (FISH). In the case of a neoadjuvant setting, i.e., a treatment prior to surgery, patients who most likely will benefit from a treatment have to be identified using limited amounts of tissues, most often small biopsies with varying percentages of tumor cells. A tumor-specific treatment before surgery can increase the chance of tumor-free resection and therefore increase the overall survival [2]. As tiny biopsies are the most common available tumor samples in the neoadjuvant settings and on the other hand the number of predictive biomarkers for treatment decisions is increasing, highly multiplex methods for quantitative tumor analysis with low sample consumption are needed. \nWhile human cancers evolve from benign to malignant lesions by acquiring a series of gene mutations over time [3], the results of gene mutations translate to dysregulation or dysfunction of proteins, including kinases, which are attractive drug targets [4]. Currently, many cancer therapeutics are designed to target the malfunction of intracellular signaling pathways that rely on de- or phosphorylated proteins. Thus, to apply personalized medicine more efficiently it is necessary to analyze tumors at the protein level in addition to determining gene mutations or gene expression patterns. \nThere are several methods available for protein analysis. Each of them has advantages and disadvantages that are listed in Table 1. For example, Western Blot and Enzyme Linked Immunosorbent Assay (ELISA) require high amounts of protein lysates. Therefore, we think that despite their advantages (e.g., protein separation according to molecular weight and easy quantification, respectively) the use of Western Blot and ELISA is not reasonable in clinical routine for detection of deregulated signaling pathways when only biopsies are available for the analysis. Currently, for protein analysis of tumor tissues IHC is mainly used which gives a spatial resolution of the epitopes analyzed down to a single cell. Using IHC and the tissue microarray (TMA) technology, it is now possible to assay hundreds of patient tissues arrayed on a single microscope slide [5]. However, IHC can hardly provide information about the activation status of proteins as the detection limit of the method is often not sufficient for analyzing phosphorylated proteins. The importance of detecting phosphoproteins in tumor samples is illustrated by a recent study that revealed that patients with HER2 negative breast cancers (IHC/FISH) express a phosphorylated form of HER2 [6]. Thus, these phospho‑HER2 positive patients may benefit from anti-HER2 treatments in addition to patients showing HER2 gene amplification. Mass spectrometry (MS)-based technologies have rapidly advanced in recent years. Beside other advantages mentioned in Table 1, MS-based methods enable the identification of new biomarker candidates by comparing protein signals obtained from cancer and healthy tissues (de novo discovery platform) [7,8,9,10]. However, in our opinion, MS‑based technologies are not suitable for the use in clinical routine at the moment. First, due to the complex sample preparation and secondly due to the insufficient profiling of low‑abundance signaling proteins.\nmicroarrays-04-00098-t001_Table 1 Table 1 Advantages and disadvantages of commonly used protein analysis platforms for tissue samples. Abbreviations: IHC: Immunohistochemistry, ELISA: Enzyme Linked Immunosorbent Assay. Different kinds of very sensitive protein arrays have been developed to quantitatively measure protein levels in high-throughput and multiplex formats. Most of them either function on the basis of forward phase protein arrays or reverse phase protein arrays [11]. In the case of forward phase protein arrays numerous capture antibodies are printed on a solid phase and are exposed to a single protein lysate (Figure 1), allowing detection of multiple proteins in a single sample. The disadvantage is, however, that two highly specific antibodies are needed for protein detection and considerable amounts of starting material are required.\nFigure 1 Schematic presentation of forward phase protein array (A) and reverse phase protein array (B). Protein A: Protein of interest. B–G: Proteins of the lysate that are not recognized by the primary antibody. HRP: Horse radish peroxidase. The technology of Reverse Phase Protein Arrays (RPPA), a miniaturized dot blot, was first described in 2001 and is based on the simultaneous measurement of a single protein in multiple samples (Figure 1) [12]. RPPA has proven to be useful for efficient tissue protein quantification and for analysis of signaling cascades even on the basis of very low amounts of tumor sample (biopsy), enabling quantification of biomarkers in tumors in neoadjuvant settings and at early time points [13,14,15]. Using RPPA even phosphoproteins can be detected. Thus, RPPA represents a very powerful tool to identify patients that most likely will benefit from future targeted therapies, aiming to inhibit deregulated kinases. The power of RPPA is reflected by an increasing number of groups worldwide that study protein levels in various sample material including human and animal tissues and cell lines [16,17,18,19,20,21]. \nWe will now highlight the clinical use of RPPA and then discuss in detail recent developments for optimizing the RPPA procedure."}