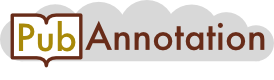
PMC:4502372 / 14261-35181
Annnotations
2_test
{"project":"2_test","denotations":[{"id":"25943524-18845848-43313263","span":{"begin":410,"end":414},"obj":"18845848"},{"id":"25943524-21490951-43313264","span":{"begin":416,"end":420},"obj":"21490951"},{"id":"25943524-15161972-43313265","span":{"begin":436,"end":440},"obj":"15161972"},{"id":"25943524-8978029-43313266","span":{"begin":1167,"end":1171},"obj":"8978029"},{"id":"25943524-2655926-43313267","span":{"begin":1194,"end":1198},"obj":"2655926"},{"id":"25943524-7545955-43313268","span":{"begin":1223,"end":1227},"obj":"7545955"},{"id":"25943524-22384331-43313269","span":{"begin":1439,"end":1443},"obj":"22384331"},{"id":"25943524-15126387-43313270","span":{"begin":2063,"end":2067},"obj":"15126387"},{"id":"25943524-10224249-43313271","span":{"begin":2079,"end":2083},"obj":"10224249"},{"id":"25943524-21454883-43313272","span":{"begin":2403,"end":2407},"obj":"21454883"},{"id":"25943524-22384331-43313274","span":{"begin":3118,"end":3122},"obj":"22384331"},{"id":"25943524-22384331-43313275","span":{"begin":3264,"end":3268},"obj":"22384331"},{"id":"25943524-22384331-43313276","span":{"begin":3358,"end":3362},"obj":"22384331"},{"id":"25943524-8477448-43313277","span":{"begin":3534,"end":3538},"obj":"8477448"},{"id":"25943524-17671506-43313278","span":{"begin":3555,"end":3559},"obj":"17671506"},{"id":"25943524-10567534-43313279","span":{"begin":3577,"end":3581},"obj":"10567534"},{"id":"25943524-8477448-43313280","span":{"begin":3877,"end":3881},"obj":"8477448"},{"id":"25943524-10567534-43313281","span":{"begin":3899,"end":3903},"obj":"10567534"},{"id":"25943524-10567534-43313282","span":{"begin":4037,"end":4041},"obj":"10567534"},{"id":"25943524-17671506-43313283","span":{"begin":4814,"end":4818},"obj":"17671506"},{"id":"25943524-20523895-43313284","span":{"begin":6174,"end":6178},"obj":"20523895"},{"id":"25943524-12191482-43313285","span":{"begin":9515,"end":9519},"obj":"12191482"},{"id":"25943524-17325030-43313286","span":{"begin":9661,"end":9665},"obj":"17325030"},{"id":"25943524-12667463-43313287","span":{"begin":9680,"end":9684},"obj":"12667463"},{"id":"25943524-12191482-43313288","span":{"begin":9698,"end":9702},"obj":"12191482"},{"id":"25943524-21454883-43313290","span":{"begin":14226,"end":14230},"obj":"21454883"},{"id":"25943524-23698095-43313291","span":{"begin":14251,"end":14255},"obj":"23698095"},{"id":"25943524-9759731-43313292","span":{"begin":14541,"end":14545},"obj":"9759731"},{"id":"25943524-10491387-43313293","span":{"begin":15120,"end":15124},"obj":"10491387"},{"id":"25943524-21454883-43313294","span":{"begin":15146,"end":15150},"obj":"21454883"},{"id":"25943524-9843498-43313295","span":{"begin":15167,"end":15171},"obj":"9843498"},{"id":"25943524-24142923-43313296","span":{"begin":17822,"end":17826},"obj":"24142923"},{"id":"25943524-25096760-43313297","span":{"begin":20013,"end":20017},"obj":"25096760"},{"id":"25943524-23457190-43313298","span":{"begin":20509,"end":20513},"obj":"23457190"}],"text":"Results\n\nSea3 impacts senescence progression and the formation of survivors in the absence of telomerase\nWe initially were interested in examining the impact of the SEA complex genes on telomere maintenance as both sea2∆ and sea3∆ deletions were identified as modifiers of growth of a cdc13-1 strain and the sea2∆ mutant was found to have short telomeres when examined in a genome-wide screen (Addinall et al. 2008, 2011; Askree et al. 2004). We found sea2∆, sea3∆, and sea4∆ haploid deletion strains had telomere lengths comparable with wild-type, whereas the iml1∆ strain had slightly longer telomeres (Figure 1B). Thus, in this directed analysis in the YPH274 strain background, deletion of the SEA complex genes had little, if any impact, on telomere length.\nWhereas wild-type strains, which constitutively express telomerase, maintain stable telomere length with propagation, strains deficient in telomerase, such as those lacking the telomerase regulatory subunits, Est1 or Est3, the telomerase catalytic subunit, Est2, or the telomerase RNA subunit, Tlc1, experience progressive telomere shortening, which leads to eventual cellular senescence (Lendvay et al. 1996; Lundblad and Szostak 1989; Singer and Gottschling 1994). Interestingly, the sea3∆ allele was reported to synthetically interact with the est1∆ deletion in a genome-wide screen for genes that affected telomere-driven senescence progression and recovery (Chang et al. 2011). Therefore, we examined directly the effect of a sea3Δ mutation on senescence progression in the absence of TLC1. To determine this, we investigated telomere length and growth potential of sea3∆ tlc1∆ strains compared with tlc1∆ strains derived from sea3Δ/SEA3tlc1∆/TLC1 (telomerase-proficient) diploids. The sea3Δ/SEA3tlc1∆/TLC1 diploids were sporulated and dissected, and individual meiotic segregants were inoculated into liquid culture. After 22 hr of growth, the cell concentration was determined and the cultures diluted back daily in a standard liquid culture senescence progression assay (Bertuch and Lundblad 2004; Le et al. 1999). Although the sea3∆ single-mutant strains exhibited a brief and slight decline in growth potential on early days of the experiment, they were otherwise largely indistinguishable from wild-type with respect to growth over the course of the experiment (Figure S1A), consistent with previous reports (Dokudovskaya et al. 2011; Panchaud et al. 2013a). Additionally, the single sea3Δ mutants, like wild-type, did not undergo progressive telomere shortening but maintained a stable telomere length over time (Figure S1B). We did find, however, that, with continued propagation, the sea3Δ tlc1Δ mutants had a more prolonged duration of restricted growth compared with tlc1Δ mutants alone, with a continued decline in cell numbers beyond day 9 and 10, until growth recovery on day 11 or 12 (Figure 1C). This finding was somewhat different than the genome-wide study, in which sea3Δ (mtc5∆) est1Δ mutants were found to have rad52Δ est1Δ mutant characteristics, with accelerated entry into senescence and lack of growth recovery (Chang et al. 2011). The differences in the growth patterns may have been attributable to differences in the media [liquid (this study) vs. solid (Chang et al. 2011)], media components, or strain background [YPH274 (this study) vs., BY4741 (Chang et al. 2011)].\nTwo types of telomerase-independent survivors have been described, both of which rely on BIR to elongate telomeres in the absence of telomerase (Lundblad and Blackburn 1993; Lydeard et al. 2007; Teng and Zakian 1999). Type I survivors amplify subtelomeric Y´ elements and have short terminal telomeric repeat tracts, whereas Type II survivors amplify telomeric sequence from the very end of another telomere, resulting in highly heterogeneous lengths of terminal telomeric repeat tracts (Lundblad and Blackburn 1993; Teng and Zakian 1999). Type II survivors predominant in serial liquid culture assays because of a growth advantage over Type I survivors (Teng and Zakian 1999). We found that sea3∆ tlc1Δ mutants still formed Type II survivors in liquid culture, but the survivors took approximately 1−2 d longer to be visualized than for tlc1∆ mutants (Figure 1D), indicating a possible delay in the execution of BIR or in growth post survivor formation. Thus, although the absence of Sea3 did not impact normal telomere length maintenance, it did impact growth and recovery of telomerase-independent survivors.\n\nAbsence of Sea3 slows colony formation after DSB induction\nAs the sea3∆ mutation slowed the appearance of BIR-dependent survivors in the absence of telomerase, we wanted to determine whether Sea3 specifically impacted BIR. To assess this, we deleted SEA3 in a strain created previously to analyze BIR at an induced DSB (Lydeard et al. 2007). In this strain, CAN1, which confers sensitivity to canavanine and is present on the nonessential telomere-proximal end of the recipient chromosome (Chr V), is truncated by the insertion of an HO endonuclease site flanked by a hygromycin resistance (HPH) reporter (Figure 2A). In addition, a 5′ truncation of CAN1 is inserted on the donor chromosome (Chr XI), resulting in 1157 bp of CAN1 homology between the donor and recipient chromosomes. The HO endonuclease is under the control of a galactose inducible promoter. Upon exposure to galactose, the DSB generated at the HO site on Chr V is repaired via BIR, resulting in a full-length CAN1 gene and loss of the hygromycin reporter. When we deleted SEA3 in this strain, we noted a consistent 2-d delay in the formation of quantifiable colonies on galactose compared with wild-type, whereas the sea3∆ mutants formed colonies comparably with wild-type on glucose (Figure 2B and Figure S2, uninduced). The overall viability on galactose, however, was equivalent to wild-type (Figure 2C). Importantly, the effect on galactose was attributable to loss of the SEA3 gene product as it was complemented by expression of SEA3 on a CEN plasmid (Figure S3A).\nFigure 2 Loss of Sea3 impacts colony formation in the break-induced replication (BIR) assay strain and on bleomycin. (A) BIR assay strain (Lydeard et al. 2010). An HO cut site (HO), marked with HPH, is integrated into the CAN1 gene (represented as CA) on chromosome V, deleting the 3′ portion of CAN1. The CAN1 donor (represented as AN1), which shares 1157 bp of homology to the CAN1 gene, is integrated into chromosome XI. Sites marked with A indicate AvaI sites used for monitoring BIR repair in Figure 3B. (B) Platings for single colonies of wild-type and sea3Δ mutants in the BIR assay strain on YPD and YPGal. (C) Percent viability of wild-type and sea3Δ mutants as a ratio of number of colonies on YPGal divided by the dilution factor and then divided by the number of colonies on YPD. Values represent average of two independent trials and error bars indicate standard error of the mean (SEM). (D) Platings for single colonies of wild-type and sea3Δ mutants in the YPH274 genetic background on YPD and YPD + 3.5 μg/mL bleomycin. To determine whether the growth delay was caused by a general sensitivity to galactose (rather than the DSB induced by galactose), we took sea3Δ mutants in the BIR strain background that had been plated previously on galactose, had undergone BIR repair of the DSB and, therefore, would not sustain another HO-induced DSB when replated on galactose, and compared their growth on galactose to sea3Δ mutants that had not been previously exposed to galactose and, therefore, would undergo DSB induction when plated on galactose. If the growth delay of the sea3Δ mutants on galactose were simply due to a general sensitivity to galactose, then the presence or absence of a cleavable HO cut site would not matter and both types of sea3Δ mutants would be equally sensitive to galactose. We found, however, that when the sea3Δ mutants that were originally plated on galactose were re-plated on galactose, quantifiable colonies appeared sooner (Figure S2; see also Figure 5A and Figure S8A), suggesting that the delay in colony formation in the sea3Δ mutant was not simply due to a sensitivity to galactose.\nTo further explore the growth delay in the absence of Sea3 after DSB induction, we examined the growth of sea3Δ mutants in the YPH274 genetic background on the DSB-inducing agent bleomycin. The sea3∆ mutant strain was sensitive bleomycin and, again, formed colonies more slowly than wild-type (Figure 2D). Growth of the sea3∆ mutant strain on galactose was comparable with the wild-type (Figure S3B), thus, clearly demonstrating a growth defect attributable to DSBs and independent of galactose. Additionally, like the delay in the sea3∆ BIR assay strain background when plated on galactose, the delay in colony formation in the sea3∆ mutant strain on bleomycin was complemented by expression of SEA3 on a CEN plasmid (Figure S3B). Therefore, absence Sea3 resulted in delayed growth upon DNA damage in two different genetic backgrounds—either via an inducible DSB in the BIR assay strain or by the DNA-damaging agent bleomycin in the YPH274 background.\n\nsea3Δ mutants are not classical recovery mutants\nThe delay in colony formation in the sea3∆ mutant BIR assay strain suggested that sea3∆ mutants may have a delay in recovery post-DNA damage. Recovery is typically defined as resumption of mitosis after repair is completed and the checkpoint is turned off; recovery mutants demonstrate sustained activation of the DDR despite repair of the DSB (Vaze et al. 2002). A variety of proteins have been implicated in recovery and most are associated with the DNA damage checkpoint or repair (Guillemain et al. 2007; Leroy et al. 2003; Vaze et al. 2002).\nWe, therefore, first determined whether the sea3Δ mutant BIR assay strain was able to repair the HO-induced DSB via BIR. Like wild-type, it repaired via BIR a majority of the time, as tracked by the sensitivity of the colonies to canavanine and hygromycin (Figure 3A). Additionally, the sea3Δ mutant repaired the DSB as rapidly as wild-type with repair products appearing in the interval between 8 and 10 hr after DSB induction (Figure 3B). Next we determined whether the mutant had a delay in terminating the DNA damage checkpoint postrepair, which is most frequently monitored by examining the pattern of Rad53 hyperphosphorylation after DSB induction. We found, however, that the sea3Δ mutant did not have prolonged Rad53 hyper-phosphorylation as compared to wild-type (Figure 3C). Taken together, as the sea3Δ mutant was able to repair the DSBs and extinguish the DNA damage checkpoint as proficiently as the wild-type, the delay in colony formation post DSB induction was distinct from a classical recovery defect.\nFigure 3 Loss of Sea3 does not delay break-induced replication (BIR) repair or extinction of the DNA damage checkpoint. (A) Percent repair types observed in wild-type and sea3Δ BIR assay strain mutants from (Figure 2B), determined by plating on media containing canavanine or hygromycin. Values represent average of two independent trials. (B) AvaI-digested genomic DNA collected from wild-type and sea3Δ BIR assay strain mutants at designated hours postgalactose induction blotted and probed with the CAN1 gene. (C) Rad53 western blot analysis of whole-cell extracts of an equivalent number of wild-type and sea3Δ mutant cells were prepared via the trichloroacetic acid method at designated hours postgalactose induction. Image is representative of two independent experiments.\n\nSea3 functions through the TORC1 pathway in response to DNA damage\nGiven Sea3′s role as a negative regulator of the Iml1 complex (SEACIT), which in turn negatively regulates TORC1 (Panchaud et al. 2013a,b), we reasoned that the delay in colony formation in the sea3∆ BIR assay strain might be the result of hyperrepression of TORC1 (Figure 4A). If so, then deletion of IML1 would rescue the delay. This is precisely what we observed (Figure 4B). Similarly, we found that deletion of IML1 rescued the sea3Δ mutant growth delay on bleomycin in the YPH274 strain background (Figure S4). Thus, these data are consistent with Sea3 functioning through TORC1 and this regulation of TORC1 impacting colony formation post-DNA repair in the BIR assay strain.\nFigure 4 Sea3 functions through TORC1, but not autophagy, in response to DNA damage. (A) Proposed effects on TORC1 if Sea3 functions as a negative regulator of the Iml1 complex/SEACIT. (B) Fivefold serial dilutions of wild-type and iml1Δ, sea3Δ iml1Δ, and sea3Δ BIR assay strain mutants plated on YPD and YPGal. (C) Fivefold serial dilutions of wild-type and atg5Δ, sea3Δ atg5Δ, and sea3Δ BIR assay strain mutants plated on YPD and YPGal. To determine whether Sea3 and, perhaps, TORC1 signaling were required for growth in response to other stress conditions, we plated sea3∆ mutants in both the YPH274 and BIR assay strain backgrounds on YPD containing a low concentration of glucose (0.25% compared to 2% in standard YPD), high salt (0.5 M NaCl added to standard YPD), or hydrogen peroxide (3 mM H2O2 added to standard YPD), and at high temperature (37°). We found the sea3Δ mutant in the YPH274 strain background had a growth delay on medium containing high salt, an effect that was mediated through TORC1 signaling as it was rescued by deletion of IML1 (Figure S5A). However, a growth delay was not observed on high salt with the sea3∆ mutant in the BIR assay strain background (Figure S5B), suggesting the phenotype was influenced by strain specific factors and not solely the absence of Sea3. In both strain backgrounds, we found that Sea3 was not required for growth in response to any of the other stresses tested (Figure S5). Taken together, although there are some strain specific differences, sea3Δ mutants experience a growth defect under conditions that induce DSBs and under conditions of high salt in a TORC1-dependent manner.\nWe next looked for possible targets downstream of TORC1 that might be responsible for the delay in colony formation phenotype. Likely candidates were factors mediating autophagy, which is negatively regulated by TORC1 signaling, and previously identified to be a pathway downstream of the yeast SEA complex (Dokudovskaya et al. 2011; Takahara and Maeda 2013). If Sea3 functioned to promote TORC1 repression of autophagy and, thereby, regulate growth post-DNA repair, then a block in autophagy would rescue the delay observed in the sea3∆ mutant. However, we found that deletion of ATG5, which encodes a core autophagy factor (Mizushima et al. 1998), had no impact on the delay in the BIR assay strain (Figure 4C). Thus, the delay in colony formation in the sea3∆ mutant was not due to aberrant up-regulation of autophagy but rather due to misregulation of another downstream TORC1 target.\n\nThe growth delay in sea3Δ mutants is rescued by the presence of wild-type TRP1\nBoth the SEA complex and TORC1 signaling have been linked to amino acid biosynthesis and internal trafficking of amino acid permeases. In the case of the SEA complex, the link has been inferred from genome-wide pairwise fitness screen data (Beck et al. 1999; Dokudovskaya et al. 2011; Schmidt et al. 1998). Therefore, it was possible that the delay in colony formation after DNA damage was attributable to a change in amino acid requirements. The parental BIR assay strain is auxotrophic for the amino acids tryptophan and leucine as well as the nucleobase uracil due to trp1, leu2, and ura3 mutations, respectively. Therefore, we asked whether addition of wild-type copies of these genes impacted the growth of wild-type and sea3Δ mutant strains. Introduction of a LEU2 CEN plasmid into the parental wild-type BIR assay strain did not result in a growth delay upon plating on galactose (Figure S6A), whereas deletion of SEA3 with a kanMX cassette did (Figure S6B), indicating that the growth delay of the sea3Δ::LEU2 mutant BIR strain on galactose was not due to LEU2 expression. Surprisingly, however, introduction of a TRP1 CEN plasmid rescued the delay of the sea3∆ mutant (Figure 5A). The rescue was specific to TRP1, as introduction of a URA3 CEN plasmid did not similarly rescue the delay (Figure S3A). Likewise, TRP1 rescued the growth delay observed when the sea3∆::HPH mutant in the YPH274 (trp1-) background was plated on bleomycin, although to a partial extent (Figure S7). These results suggested that the delay in colony formation observed in sea3∆ mutants was due to perturbations in internal levels of tryptophan.\nFigure 5 Expression of TRP1, but not exogenous tryptophan, rescues the delay in colony formation in sea3Δ mutants. (A) Fivefold serial dilutions of wild-type and sea3Δ mutants in the break-induced replication (BIR) assay strain with and without the addition of the TRP1 plasmid pRS414 on YPD and YPGal. The wild-type-in and sea3∆::LEU2-in strains have been previously experienced HO-induction with galactose and undergone a repair event, rendering them unable to undergo HO-mediated DSB induction upon replating on galactose. (B) Fivefold serial dilutions of the wild-type and sea3Δ BIR assay strain mutants plated on YPD, YPGal, YPD + 100 μM tryptophan (Trp) and YPGal + 100 μM Trp. (C) Western blots showing Tat2-3XFlag levels post-galactose induction at the indicated time points. Whole-cell extracts were prepared and blotted with α-Flag. Images are representative of three independent experiments. (D) Fivefold serial dilutions of the wild-type and sea3Δ mutants in the BIR assay strain were plated on YPD, YPGal and YPD and YPGal with either 2 or 4 μM quinolinic acid (QA) added. Plates were imaged on day 3, day 4, and day 5. Therefore, we examined whether tryptophan availability was contributing to the sea3∆ mutant phenotype. Even in rich media, the level of tryptophan is low (measured 26 µg/mL in Jarolim et al. 2013), so we reasoned that if tryptophan availability were the source of the delay in colony formation in the sea3Δ mutant, addition of an excessive amount of tryptophan to the media might rescue the delay. Although we found that both wild-type and sea3∆ mutant strains grew slightly more robustly on YPGal supplemented with an additional 100 μM tryptophan compared with YPGal, the sea3Δ mutant still displayed a growth delay relative to the wild-type (Figure 5B). Combined with the rescue of TRP1 expression, this suggested that after DNA damage, sea3Δ mutants are either unable to import tryptophan into the cell sufficiently or that tryptophan consumption was altered.\nTo address this further, we examined whether the levels of Tat2 changed in the absence of Sea3 upon galactose induction in the BIR assay strain. We found that Tat2 levels were comparable throughout the time course in wild-type and the sea3Δ mutant, with levels declining between 32 and 48 hr (Figure 5C and Figure S9). Additionally, deletion of TAT2 in the BIR assay strain did not result in a delay in colony formation post-DSB induction (Figure S10), suggesting that although the delay in colony formation in the sea3Δ mutant appeared to be attributable to insufficient internal tryptophan levels, loss of Tat2 protein was not responsible.\nOf note, we also found that the delay in colony formation in the sea3∆ BIR assay strain was only observed on rich media as, when the sea3∆ mutant was plated on synthetic defined media containing galactose and tryptophan, among the added amino acids (e.g., -Ura Gal or -Leu Gal minimal media; tryptophan concentration 40 µg/mL), it grew like the wild-type (Figure S6 and Figure S8). This indicated that, under those nutrient conditions, the cells were sufficient in tryptophan uptake.\nOne possibility for the delay in colony formation in the sea3Δ mutant might relate to the availability of the coenzyme nicotinamide adenosine dinucleotide (NAD+), which is synthesized either via a salvage pathway or de novo from tryptophan via the kynurenine pathway. Notably, NAD+ is used in a wide range of cellular pathways, including those involved in DNA repair and the DDR (Kato and Lin 2014). We hypothesized that the slow growth phenotype of the sea3Δ mutant post-DNA damage could be due to limiting NAD+ levels resulting from decreased internal tryptophan. Therefore, supplying an intermediate downstream from tryptophan in the de novo synthesis pathway might rescue the delay. Quinolinic acid is one such intermediate and, importantly, can be imported into cells via the high affinity nicotinic acid permease and, thereby, used to increase internal levels of NAD+ (Ohashi et al. 2013). When we plated wild-type and sea3Δ mutants in the BIR strain background on media containing galactose and varying amounts of quinolinic acid, we found the sea3Δ mutants grew markedly better than without quinolinic acid (Figure 5D). Therefore, we conclude tryptophan auxotrophy is synthetic with the sea3∆ mutation under DNA damage conditions partially due to a decline in the levels of the coenzyme NAD+."}