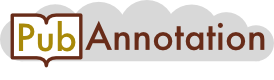
PMC:4103454 / 4441-19089
Annnotations
{"target":"https://pubannotation.org/docs/sourcedb/PMC/sourceid/4103454","sourcedb":"PMC","sourceid":"4103454","source_url":"https://www.ncbi.nlm.nih.gov/pmc/4103454","text":"Results\n\nProcessing of 5′ Flaps Is Conserved among FAN1 Orthologs\nOur initial aim was to crystallize and biochemically characterize FAN1, and to this end, we purified a range of FAN1 orthologs from human, mouse, and Pseudomonas (hFAN1, mFAN1, and pFAN1, respectively; Figure 1A), expressed in bacteria. To compare their nuclease activities, proteins were incubated with 5′ flap substrates labeled at either the 5′ (Figure 1B) or 3′ end (Figure 1C) of the flap strand. Figure 1B shows a similar cleavage pattern for hFAN1 and mFAN1, generating a long endonucleolytic product corresponding to cleavage 4 bp after the branchpoint and a short product (4 nt) corresponding to cleavage close to the 5′ end of the flap. This short product was the major band for hFAN1 but was less dominant for mFAN1. pFAN1 exhibited a different profile with strong cleavage 4 and 5 bp after the branchpoint but with no detectable cleavage at the 5′ end. However, all three enzymes displayed some 5′ to 3′ exonuclease activity following the initial cut (Figure 1C), suggesting that they differ in their ability to bind and/or cleave DNA ends, but not in their ability to process DNA branchpoints. As reported in MacKay et al. (2010), the FAN1 proteins do not have activity against Holliday junction (HJ) substrates (Figure S1A).\nThe apparent lack of pFAN1 activity at DNA ends suggested it as a good candidate for a more-detailed investigation of branchpoint processing. To this end, pFAN1 was incubated with 5′ flap substrates labeled at the 5′ end of either the flap strand (a) or the duplex strand (b) (Figures 1D and S1B). Whereas the a strand was cleaved almost to completion within the first minute, significant cleavage of the b strand only became apparent after 2 min, proceeding to completion in ∼40 min. The different kinetics of these two cleavage events suggests that the 5′ flap substrate is initially cleaved on the a strand and processed into a gapped duplex or a 5′ overhang, which is then targeted by another pFAN1 molecule in a slower reaction (Figure 1E).\n\nVRR-Nuc Domains Are Structurally Related to Holliday-Junction-Resolving Enzymes\nDespite considerable effort, we have thus far failed to crystallize FAN1 or isolate soluble VRR-Nuc domains from any of the FAN1 orthologs. However, we were able to solve the first VRR-Nuc domain structures using single-domain proteins derived from three bacteria and bacteriophage as surrogates: Psychrobacter (psNUC); Streptococcus equi bacteriophage P9 (stNUC); and Salmonella enteritidis typing phage 3 (saNUC) (Figure 2A). Crystallographic statistics are presented in Figure S2A.\nstNUC was solved by single-wavelength anomalous diffraction (SAD) methods on crystals of selenomethione-substituted protein and refined against native 1.3 Å resolution data (Figures 2A and S2B). One molecule is present per asymmetric unit (AU) and comprises a central four-stranded β sheet surrounded by three α helices in a αβββαβα topology (Figure 2B). The protein crystallized as a dimer with monomers related by a crystallographic 2-fold axis and interacting through a substantial hydrophobic interface formed by the central β sheets of opposing molecules and bounded on either side by the α2 helix from each partner. Electrostatic surface representations highlight the position of the negatively charged active sites on each monomer (Figure 2A), which bind a single magnesium ion in a geometry that is distorted from a classical octahedral arrangement toward a square pyramidal configuration (Figure 2C). K47 is presumably involved in positioning the catalytic water molecule as would be expected from homology with other members of the restriction endonuclease-like superfamily (Knizewski et al., 2007). Q57, a conserved residue within VRR-Nuc domains (http://pfam.xfam.org/family/vrr_nuc), makes a hydrogen bond to K47 that is absent in archaeal Holliday junction resolvase (HJR) structures. The active sites are surrounded by positively charged residues in a figure-of-eight pattern, as seen in Sulfolobus solfataricus Hjc (Protein Data Bank [PDB] ID 1HH1; Bond et al., 2001), consistent with a role in substrate DNA recognition and orientation.\nsaNUC was solved by molecular replacement using the stNUC structure as a search model (Figure 2A), revealing a dimeric arrangement of two molecules in the AU essentially identical to that seen in the stNUC crystals. Despite only having 34% sequence identity with stNUC, both have core structures that are remarkably similar (root-mean-square deviation = 1.3 Å over 86 Cα) and the orientation of active site residues is essentially identical to stNUC.\npsNUC was solved by SAD and refined against 2.0 Å resolution data. Here, the crystals contain six molecules per AU, comprising three dimers that, like those of saNUC and stNUC, form around a central hydrophobic β sheet interface. There are, nonetheless, several elaborations (Figure 2A). In particular, the linker between β1-β2 forms a partially structured extension over the active site surface. Such structures are common in Holliday-junction-resolving enzymes, including RecU (PDB ID 1ZP7), and likely play a role in the binding and orientation of substrate (Cañas et al., 2011). Hexacoordination of a magnesium ion is seen at each active site, involving the E-PD-EXK motif and two water molecules in a configuration stabilized by the conserved Q102 (Figure 2C). The active site surface is bounded by a ring of positively charged residues to allow substrate recognition (Figure 2A).\nDALI searches (Holm and Sander, 1995) show that the structures with greatest similarity to the VRR-Nuc domains are all Holliday-junction-resolving enzymes exemplified by Hjc (Figure 2B). This observation raised the possibility that dimeric VRR-Nuc domain proteins selectively process four-way DNA junctions, and we therefore investigated the nuclease activities of these three proteins.\n\nVRR-Nuc Domains Are Dimeric and Cleave Four-Way DNA Junctions\nA key characteristic of canonical HJRs is that they bind and process their substrate as dimers to achieve substrate recognition and productive resolution and predominantly form homodimers in solution in the absence of substrate (Lilley and White, 2001). We examined the quaternary structure of the VRR-Nuc domains in solution by performing analytical ultracentrifugation (AUC) experiments (Figure 3A). For each protein, the main peak in the sedimentation coefficient distribution analysis (C(S)) corresponded to a dimer. For psNUC, an additional minor peak corresponding to a tetrameric species was present at concentrations above 70 μM. This agrees well with our crystal structures and the known quaternary structure of HJRs.\nThe ability of VRR-Nuc domains to bind a range of DNA structures was examined using electrophoretic mobility-shift assays. As shown in Figure 3B, psNUC bound a four-way DNA junction stoichiometrically with an affinity indicative of a subnanomolar equilibrium dissociation constant (KD) typical of HJRs (∼1 nM; White et al., 1997) whereas stNUC bound the junction over 100 times more weakly (KD = 200 nM). Nevertheless, in both cases, the binding was structure-selective, with psNUC forming weaker complexes with a 5′ flap and a nicked three-way junction and stNUC binding the four-way junction only (Figure S3A). At high protein concentrations, higher-order complexes formed with equal efficiency on all substrates. By contrast to the other proteins, saNUC only formed nonspecific, higher-order complexes.\nWe next investigated the catalytic activity of the VRR-Nuc domains toward these DNA substrates. Figure 3C shows the cleavage products obtained with psNUC and Jbm5, a four-way junction that can undergo 12 steps of branch migration such that each possible dinucleotide can be accommodated at the branchpoint (Kvaratskhelia et al., 2001). This junction was cleaved symmetrically, and we observed only two discrete cleavage sites on each strand that occurred within or close to the homologous core of the junction (boxed, Figure 3D). This resulted in the formation of ligatable nicked duplex DNA, diagnostic of productive HJ resolution (Figure S3B). Under the same assay conditions, we observed no cleavage of linear double-stranded DNA substrate and very weak activity on most branched DNA structures with the exception of replication forks, which were cleaved to an intermediate degree (Figure S3C). Finally, psNUC cleaved a fixed HJ 3 nt 3′ to the branchpoint, and this activity was inhibited by the presence of a slow-cutting mutant of T7 endonuclease I, suggesting that they compete for the branchpoint (Figure S3D).\nstNUC and saNUC exhibited weaker nuclease activity against the Jbm5 HJ substrate, detectable only at protein concentrations above 1 μM and with increased reaction times (Figure 3E). Under these conditions, stNUC showed robust activity, whereas cleavage by saNUC was much reduced. This suggests that our saNUC preparations have a low active fraction potentially also explaining the lack of structure selectivity seen in the gel mobility shift experiments. In both cases, we observed a range of symmetrical cleavage sites within or close to the homologous core of the junction as well as some nonsymmetrical sites away from the branchpoint (Figure 3F). stNUC also selectively cleaved a nicked three-way junction (RF) and a 3′ flap structure (3′F) 2 bp 3′ to the branchpoint (Figure S3E). Both enzymes additionally exhibited a nonspecific nuclease activity producing a ladder of cleavage products (Figure S3F).\npsNUC therefore not only structurally resembles an archaeal HJR but also behaves as one, displaying binding to and symmetrical cutting of HJ substrates with affinities and cleavage patterns typical of a canonical HJC protein. Whether the levels of activity observed for stNUC and saNUC are representative of those in vivo is unclear, but it would appear unlikely given their close structural homology to psNUC. Crucially, however, both enzymes retain the ability to produce symmetric cleavages within a HJ substrate, an activity that is not characteristic of VRR-Nuc domains within the FAN1 orthologs.\n\nFAN1 Enzymes Are Monomeric in Solution\nThe isolated VRR-Nuc domains studied here form dimers, consistent with an important role in the recognition of four-way DNA junctions, whereas the FAN1 orthologs exhibited no activity on four-way DNA junctions, instead processing 5′ flaps very efficiently. This suggested that the FAN1 VRR-Nuc domains may act as monomeric enzymes, and this hypothesis was investigated further.\nUsing analytical gel filtration, column retention times of hFAN1 and mFAN1 were consistent with a molecular weight of approximately 200 kDa, raising the possibility of a dimeric interaction (Figure 4A). By contrast, the column retention time of pFAN1 was consistent with a monomer. PSIPRED secondary structure predictions (Jones, 1999) suggest that both hFAN1 and mFAN1 are essentially unstructured over the first ∼370 residues, potentially explaining the low retention times in gel filtration experiments. To test this, we removed the first 359 residues of hFAN1 leaving the region of homology to pFAN1. This protein (hFAN1_360) was fully functional in nuclease assays (not shown) and eluted from gel filtration with a retention time consistent with a monomer. Although it remained possible that the N-terminal regions within mouse and human FAN1 might be mediating self-association, sedimentation velocity analysis showed unambiguously that, like pFAN1, full-length mouse FAN1 is monomeric (Figure 4B). Finally, limited tryptic proteolysis of hFAN1_360 produced three protected fragments identified by mass spectrometry as amino acids 360–507, 515–790, and 795–1,012. Gel filtration performed on a preparative scale tryptic digest gave rise to a single eluted peak containing three species with a retention time almost identical to the undigested molecule (Figure 4C). Indeed, attempts to express constructs corresponding to the tryptic fragments either in isolation or as pairs were unsuccessful, suggesting an intimate association of all three domains. Together, these results support the hypothesis that eukaryotic FAN1 proteins comprise an unstructured N terminus and a compact C terminus comprised of three domains that broadly correspond to the SAP, TPR, and VRR-Nuc homology regions.\n\nModeling of FAN1 VRR-Nuc Domains Explains Their Oligomeric State and Substrate Specificity\nThe apparent inability of the VRR-Nuc domain of FAN1 to dimerize could in principle result from its interactions with other regions of the full-length protein or be an intrinsic property of the domain. To further investigate this and reconcile the apparent difference in substrate specificity and oligomeric state between bacterially derived VRR-Nuc domains and the FAN1 proteins, we examined sequence alignments of VRR-Nuc domains from each group prepared using the PRALINE multiple alignment server (Simossis and Heringa, 2005; Figures 5A and S4A). The majority of bacterially derived VRR-Nuc domains conform to the αβββαβα topology seen in the crystal structure of stNUC. A subset of these proteins additionally contain a predicted short α-helical section of 8–11 residues between β1-β2, in an equivalent position to the α-helical section of the stalk extension of psNUC. For the FAN1 proteins, however, this is replaced by two strongly predicted α helices approximately 10 and 18 residues long separated by a conserved I/L-G/P dipeptide, giving rise to an αβααββαβ topology that is likely unique among the restriction endonuclease-like superfamily (Knizewski et al., 2007). This difference is also apparent from phylogenetic analysis of VRR-Nuc sequences where FAN1-derived VRR-Nuc domains populate a clade distinct from the bacterial and phage examples (Figures 5B and S4B).\nTo understand how this two-helix insertion might affect the quaternary structure of the FAN1 VRR-Nuc domain, we generated models of the VRR-Nuc domains from hFAN1 and pFAN1, both of which resemble the bacterial VRR-Nuc domains with respect to the β sheet and position of the active site residues (Figures 5C and S4C). Helical wheel representations of the helical insertion suggest that the insertion forms an amphipathic helix-turn-helix motif that packs onto the hydrophobic surface of the central β sheet, effectively blocking the canonical VRR-Nuc domain dimerization interface (Figure 5D). In keeping with the VRR-Nuc domain structures described in this study, the negatively charged active site pocket is surrounded by a ring of positive charge, which also extends along the outer surface of the helical insertion in our models (Figure 5E).\n","divisions":[{"label":"Title","span":{"begin":0,"end":7}},{"label":"Section","span":{"begin":9,"end":2050}},{"label":"Title","span":{"begin":9,"end":65}},{"label":"Section","span":{"begin":2052,"end":5894}},{"label":"Title","span":{"begin":2052,"end":2131}},{"label":"Section","span":{"begin":5896,"end":10118}},{"label":"Title","span":{"begin":5896,"end":5957}},{"label":"Section","span":{"begin":10120,"end":12329}},{"label":"Title","span":{"begin":10120,"end":10158}},{"label":"Section","span":{"begin":12331,"end":14647}},{"label":"Title","span":{"begin":12331,"end":12421}}],"tracks":[{"project":"2_test","denotations":[{"id":"24981866-20603015-20021734","span":{"begin":1203,"end":1207},"obj":"20603015"},{"id":"24981866-17584917-20021735","span":{"begin":3720,"end":3724},"obj":"17584917"},{"id":"24981866-11331763-20021736","span":{"begin":4095,"end":4099},"obj":"11331763"},{"id":"24981866-21600217-20021737","span":{"begin":5198,"end":5202},"obj":"21600217"},{"id":"24981866-8578593-20021738","span":{"begin":5540,"end":5544},"obj":"8578593"},{"id":"24981866-11389467-20021739","span":{"begin":6205,"end":6209},"obj":"11389467"},{"id":"24981866-9223630-20021740","span":{"begin":7009,"end":7013},"obj":"9223630"},{"id":"24981866-11240135-20021741","span":{"begin":7820,"end":7824},"obj":"11240135"},{"id":"24981866-10493868-20021742","span":{"begin":10867,"end":10871},"obj":"10493868"},{"id":"24981866-15980472-20021743","span":{"begin":12946,"end":12950},"obj":"15980472"},{"id":"24981866-17584917-20021744","span":{"begin":13593,"end":13597},"obj":"17584917"}],"attributes":[{"subj":"24981866-20603015-20021734","pred":"source","obj":"2_test"},{"subj":"24981866-17584917-20021735","pred":"source","obj":"2_test"},{"subj":"24981866-11331763-20021736","pred":"source","obj":"2_test"},{"subj":"24981866-21600217-20021737","pred":"source","obj":"2_test"},{"subj":"24981866-8578593-20021738","pred":"source","obj":"2_test"},{"subj":"24981866-11389467-20021739","pred":"source","obj":"2_test"},{"subj":"24981866-9223630-20021740","pred":"source","obj":"2_test"},{"subj":"24981866-11240135-20021741","pred":"source","obj":"2_test"},{"subj":"24981866-10493868-20021742","pred":"source","obj":"2_test"},{"subj":"24981866-15980472-20021743","pred":"source","obj":"2_test"},{"subj":"24981866-17584917-20021744","pred":"source","obj":"2_test"}]}],"config":{"attribute types":[{"pred":"source","value type":"selection","values":[{"id":"2_test","color":"#ecde93","default":true}]}]}}