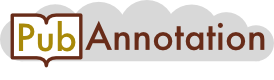
PMC:3681796 / 1423-5008
Annnotations
NEUROSES
{"project":"NEUROSES","denotations":[{"id":"T48","span":{"begin":131,"end":141},"obj":"PATO_0002261"},{"id":"T49","span":{"begin":265,"end":281},"obj":"CHEBI_25512"},{"id":"T50","span":{"begin":282,"end":295},"obj":"CHEBI_15355"},{"id":"T51","span":{"begin":332,"end":343},"obj":"PATO_0001818"},{"id":"T52","span":{"begin":344,"end":354},"obj":"PATO_0001510"},{"id":"T53","span":{"begin":467,"end":474},"obj":"PATO_0001193"},{"id":"T54","span":{"begin":524,"end":532},"obj":"PATO_0000380"},{"id":"T55","span":{"begin":724,"end":731},"obj":"PATO_0000006"},{"id":"T56","span":{"begin":756,"end":768},"obj":"PATO_0002037"},{"id":"T57","span":{"begin":2810,"end":2822},"obj":"PATO_0002037"},{"id":"T58","span":{"begin":756,"end":768},"obj":"PATO_0000639"},{"id":"T59","span":{"begin":2810,"end":2822},"obj":"PATO_0000639"},{"id":"T60","span":{"begin":855,"end":865},"obj":"PATO_0000990"},{"id":"T61","span":{"begin":1124,"end":1134},"obj":"CHEBI_18421"},{"id":"T62","span":{"begin":1184,"end":1190},"obj":"PATO_0001021"},{"id":"T63","span":{"begin":1295,"end":1301},"obj":"PATO_0001021"},{"id":"T64","span":{"begin":2463,"end":2469},"obj":"PATO_0001021"},{"id":"T65","span":{"begin":1208,"end":1217},"obj":"PATO_0001997"},{"id":"T66","span":{"begin":2486,"end":2493},"obj":"PATO_0001997"},{"id":"T67","span":{"begin":1388,"end":1397},"obj":"CHEBI_33284"},{"id":"T68","span":{"begin":1508,"end":1519},"obj":"PATO_0001624"},{"id":"T69","span":{"begin":1631,"end":1639},"obj":"PATO_0000428"},{"id":"T70","span":{"begin":1697,"end":1709},"obj":"CHEBI_15541"},{"id":"T71","span":{"begin":1902,"end":1914},"obj":"CHEBI_15541"},{"id":"T72","span":{"begin":1790,"end":1795},"obj":"CHEBI_36375"},{"id":"T73","span":{"begin":1798,"end":1806},"obj":"PATO_0000605"},{"id":"T74","span":{"begin":1798,"end":1806},"obj":"PATO_0000140"},{"id":"T75","span":{"begin":1876,"end":1889},"obj":"CHEBI_15525"},{"id":"T76","span":{"begin":1891,"end":1896},"obj":"CHEBI_15756"},{"id":"T77","span":{"begin":1916,"end":1921},"obj":"CHEBI_28842"},{"id":"T78","span":{"begin":1947,"end":1963},"obj":"CHEBI_53152"},{"id":"T79","span":{"begin":1965,"end":1970},"obj":"CHEBI_24548"},{"id":"T80","span":{"begin":1976,"end":1986},"obj":"CHEBI_15534"},{"id":"T81","span":{"begin":2079,"end":2086},"obj":"PATO_0001504"},{"id":"T82","span":{"begin":2087,"end":2093},"obj":"CHEBI_18059"},{"id":"T83","span":{"begin":2102,"end":2117},"obj":"CHEBI_18035"},{"id":"T84","span":{"begin":2119,"end":2132},"obj":"CHEBI_16247"},{"id":"T85","span":{"begin":2134,"end":2147},"obj":"CHEBI_17855"},{"id":"T86","span":{"begin":3070,"end":3077},"obj":"PATO_0000462"},{"id":"T87","span":{"begin":3192,"end":3201},"obj":"PATO_0002292"},{"id":"T88","span":{"begin":3251,"end":3261},"obj":"PATO_0000076"},{"id":"T89","span":{"begin":3402,"end":3406},"obj":"CHEBI_50906"},{"id":"T90","span":{"begin":3527,"end":3539},"obj":"PATO_0001869"},{"id":"T91","span":{"begin":888,"end":905},"obj":"PM6290"},{"id":"T92","span":{"begin":1476,"end":1493},"obj":"PM6290"},{"id":"T93","span":{"begin":455,"end":484},"obj":"PM2169"},{"id":"T94","span":{"begin":888,"end":905},"obj":"PM6290"},{"id":"T95","span":{"begin":1476,"end":1493},"obj":"PM6290"},{"id":"T96","span":{"begin":1631,"end":1643},"obj":"PM8995"}],"text":"Introduction\nIn mammals, the contraction of voluntary skeletal muscles is under the control of motor neurons whose cell bodies are located in the spinal cord and the brainstem. These so-called lower motor neurons directly communicate with muscle fibers through the neurotransmitter acetylcholine at the neuromuscular junctions. The progressive functional deterioration of this neuromuscular axis is typically found in neurodegenerative conditions such as amyotrophic lateral sclerosis (ALS). This disease, which is the most frequent adult-onset form of motor neuron disease, is characterised by motor neuron death, skeletal muscle atrophy and paralysis [1]. Studies conducted on genetic animal models of ALS showed that the process leading to motor neuron degeneration is not cell-autonomous but involves defects in other cell types than neurons [2]–[5]. Consistent with this notion, ALS neurodegeneration is also associated with systemic defects, including hypermetabolism and dyslipidemia, which are observed in both patients and animal models [6]–[8]. Particularly, transgenic mice overexpressing a mutated form of Cu/Zn superoxide dismutase (SOD1), linked to familial ALS, are in energy deficit and have decreased adipose tissue stores. These deficiencies appear to be elicited by increased energy expenditure (that is to say, hypermetabolism), and due to an increased consumption of nutrients by skeletal muscles [9], [10].\nTo gain insight into the relationships between neurodegeneration and metabolic dysfunction, we recently analyzed the gene expression profiles of skeletal muscles from mutant SOD1 mice and patients with sporadic ALS [11], [12]. We found a decrease in the expression of stearoyl-CoA desaturase-1 (SCD1), an enzyme that introduces the first cis double bond in the delta-9 position of saturated fatty acyl-CoA substrates. The preferred substrates are palmitoyl-CoA (C16:0) and stearoyl-CoA (C18:0), which are converted to palmitoleoyl-CoA (C16:1) and oleoyl-CoA (C18:1), respectively [13]. These monounsaturated fatty acids are the major constituents of complex lipids such as diacylglycerols, phospholipids, triglycerides, wax esters and cholesterol esters. Interestingly, the targeted disruption of the mouse SCD1 gene triggers an increase in the expression of genes involved in the β-oxidation of fatty acids and a decrease in the expression of genes involved in lipogenesis [14]. Therefore, these SCD1 knockout mice exhibit augmented energy expenditure and reduced body adiposity [14], a situation that is reminiscent of the metabolic phenotype of mutant SOD1 mice [9]. Despite sharing common features at the metabolic level, it is not known whether a decrease in SCD1 expression could be implicated by any means in the maintenance of muscle function and, perhaps, in motor neuron degeneration. In this work, first we characterized the expression of SCD1 in muscles from mutant SOD1 mice as well as in experimentally denervated muscles. Second, we studied the muscle phenotype of mice deficient in SCD1. Third, we analyzed the impact of the absence of SCD1, as obtained by both genetic and pharmacological means, on the recovery of muscle function in response to transient nerve lesion. We conclude that the systemic down-regulation of SCD1 promotes muscle oxidative metabolism and accelerates muscle function recovery after nerve injury, thus providing evidence for a new role of this enzyme in modulating the restorative potential of skeletal muscles. These findings therefore may be relevant to pathological conditions affecting the lower motor neurons."}
2_test
{"project":"2_test","denotations":[{"id":"23785402-21296405-91676649","span":{"begin":654,"end":655},"obj":"21296405"},{"id":"23785402-16741123-91676650","span":{"begin":847,"end":848},"obj":"16741123"},{"id":"23785402-22010221-91676651","span":{"begin":851,"end":852},"obj":"22010221"},{"id":"23785402-19306035-91676652","span":{"begin":1047,"end":1048},"obj":"19306035"},{"id":"23785402-21035400-91676653","span":{"begin":1051,"end":1052},"obj":"21035400"},{"id":"23785402-15263088-91676654","span":{"begin":1419,"end":1420},"obj":"15263088"},{"id":"23785402-17438338-91676655","span":{"begin":1424,"end":1426},"obj":"17438338"},{"id":"23785402-18000159-91676656","span":{"begin":1645,"end":1647},"obj":"18000159"},{"id":"23785402-21934272-91676657","span":{"begin":1651,"end":1653},"obj":"21934272"},{"id":"23785402-8453-91676658","span":{"begin":2010,"end":2012},"obj":"8453"},{"id":"23785402-12177411-91676659","span":{"begin":2404,"end":2406},"obj":"12177411"},{"id":"23785402-12177411-91676660","span":{"begin":2510,"end":2512},"obj":"12177411"},{"id":"23785402-15263088-91676661","span":{"begin":2595,"end":2596},"obj":"15263088"}],"text":"Introduction\nIn mammals, the contraction of voluntary skeletal muscles is under the control of motor neurons whose cell bodies are located in the spinal cord and the brainstem. These so-called lower motor neurons directly communicate with muscle fibers through the neurotransmitter acetylcholine at the neuromuscular junctions. The progressive functional deterioration of this neuromuscular axis is typically found in neurodegenerative conditions such as amyotrophic lateral sclerosis (ALS). This disease, which is the most frequent adult-onset form of motor neuron disease, is characterised by motor neuron death, skeletal muscle atrophy and paralysis [1]. Studies conducted on genetic animal models of ALS showed that the process leading to motor neuron degeneration is not cell-autonomous but involves defects in other cell types than neurons [2]–[5]. Consistent with this notion, ALS neurodegeneration is also associated with systemic defects, including hypermetabolism and dyslipidemia, which are observed in both patients and animal models [6]–[8]. Particularly, transgenic mice overexpressing a mutated form of Cu/Zn superoxide dismutase (SOD1), linked to familial ALS, are in energy deficit and have decreased adipose tissue stores. These deficiencies appear to be elicited by increased energy expenditure (that is to say, hypermetabolism), and due to an increased consumption of nutrients by skeletal muscles [9], [10].\nTo gain insight into the relationships between neurodegeneration and metabolic dysfunction, we recently analyzed the gene expression profiles of skeletal muscles from mutant SOD1 mice and patients with sporadic ALS [11], [12]. We found a decrease in the expression of stearoyl-CoA desaturase-1 (SCD1), an enzyme that introduces the first cis double bond in the delta-9 position of saturated fatty acyl-CoA substrates. The preferred substrates are palmitoyl-CoA (C16:0) and stearoyl-CoA (C18:0), which are converted to palmitoleoyl-CoA (C16:1) and oleoyl-CoA (C18:1), respectively [13]. These monounsaturated fatty acids are the major constituents of complex lipids such as diacylglycerols, phospholipids, triglycerides, wax esters and cholesterol esters. Interestingly, the targeted disruption of the mouse SCD1 gene triggers an increase in the expression of genes involved in the β-oxidation of fatty acids and a decrease in the expression of genes involved in lipogenesis [14]. Therefore, these SCD1 knockout mice exhibit augmented energy expenditure and reduced body adiposity [14], a situation that is reminiscent of the metabolic phenotype of mutant SOD1 mice [9]. Despite sharing common features at the metabolic level, it is not known whether a decrease in SCD1 expression could be implicated by any means in the maintenance of muscle function and, perhaps, in motor neuron degeneration. In this work, first we characterized the expression of SCD1 in muscles from mutant SOD1 mice as well as in experimentally denervated muscles. Second, we studied the muscle phenotype of mice deficient in SCD1. Third, we analyzed the impact of the absence of SCD1, as obtained by both genetic and pharmacological means, on the recovery of muscle function in response to transient nerve lesion. We conclude that the systemic down-regulation of SCD1 promotes muscle oxidative metabolism and accelerates muscle function recovery after nerve injury, thus providing evidence for a new role of this enzyme in modulating the restorative potential of skeletal muscles. These findings therefore may be relevant to pathological conditions affecting the lower motor neurons."}