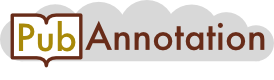
PMC:2938983 / 8981-13552
Annnotations
TEST0
{"project":"TEST0","denotations":[{"id":"20859447-180-188-266772","span":{"begin":397,"end":401},"obj":"[\"8941956\"]"},{"id":"20859447-204-212-266773","span":{"begin":421,"end":425},"obj":"[\"11283309\"]"},{"id":"20859447-227-235-266774","span":{"begin":444,"end":448},"obj":"[\"19110006\"]"},{"id":"20859447-69-77-266775","span":{"begin":520,"end":524},"obj":"[\"16107585\"]"},{"id":"20859447-102-110-266776","span":{"begin":553,"end":557},"obj":"[\"16960576\"]"},{"id":"20859447-120-128-266777","span":{"begin":571,"end":575},"obj":"[\"19634031\"]"},{"id":"20859447-55-63-266778","span":{"begin":943,"end":947},"obj":"[\"18874598\"]"},{"id":"20859447-65-73-266779","span":{"begin":1830,"end":1834},"obj":"[\"10818167\"]"},{"id":"20859447-98-106-266780","span":{"begin":1863,"end":1867},"obj":"[\"11853862\"]"},{"id":"20859447-119-127-266781","span":{"begin":1884,"end":1888},"obj":"[\"16842867\"]"},{"id":"20859447-144-152-266782","span":{"begin":1909,"end":1913},"obj":"[\"18971455\"]"},{"id":"20859447-79-87-266783","span":{"begin":2078,"end":2082},"obj":"[\"10818167\"]"},{"id":"20859447-114-122-266784","span":{"begin":2113,"end":2117},"obj":"[\"18971455\"]"},{"id":"20859447-230-238-266785","span":{"begin":2410,"end":2414},"obj":"[\"15998206\"]"},{"id":"20859447-231-239-266786","span":{"begin":4134,"end":4138},"obj":"[\"16380289\"]"},{"id":"20859447-226-234-266787","span":{"begin":4565,"end":4569},"obj":"[\"19576575\"]"}],"text":"Mouse Assays for Cognitive Flexibility\nExecutive functions subserve the selection and processing of information necessary to plan, control and direct behavior in a manner appropriate to current environmental demands. Across species, these processes are mediated by neural circuitry including the prefrontal cortex (PFC) and interconnected subcortical regions, such as the striatum (Goldman-Rakic, 1996; Miller and Cohen, 2001; Floresco et al., 2009). Pathology in these circuits is a hallmark of schizophrenia (Robbins, 2005; Lewis and Gonzalez-Burgos, 2006; Tan et al., 2009), and is specifically tied to the profound executive deficits exhibited by schizophrenic patients on measures of cognitive flexibility described below.\nCognitive flexibility is a critical executive function that can be broadly defined as the ability to adapt behaviors in response to changes in the environment. The Wisconsin Card Sorting Task (WCST; Grant and Berg, 1948) has been one of the more commonly employed assays for impaired cognitive flexibility in schizophrenic patients, and analogous versions have been developed for use in lower species, including mice (Table 2). In essence, these tasks involve the subject selecting between stimuli, which vary from one another in more than one perceptual dimension (or “set”), and being reinforced for choosing a stimulus based upon one specific dimension alone, e.g., odor. During an “intra-dimensional shift” (IDS), the form of the dimension the subject must choose is changed by the experimenter, e.g., from cinnamon to chocolate odor. In an “extra-dimensional shift” (EDS), the correct dimension is changed altogether, such that choices must be guided by the new dimension (texture) while ignoring the previously rewarded dimension.\nIn a rodent IDS/EDS analog of the WCST, rats (Birrell and Brown, 2000) or mice (Colacicco et al., 2002; Garner et al., 2006; Bissonette et al., 2008) dig in sand to make choices based on the dimension of texture or smell (Figure 2A). As in humans, this task is sensitive to PFC damage in rats (Birrell and Brown, 2000) and mice (Bissonette et al., 2008). One potentially salient difference with the human procedure is that the dimensions are in different sensory modalities [note: attempts to date to develop a IDS/EDS task using a single (visual) modality have not demonstrated the formation of an attentional set in the mouse (Brigman et al., 2005)].\nFigure 2 Mouse behavioral assays for cognitive flexibility. (A) Intra-dimensional/extra-dimensional digging task. Mice are trained to dig for food reward using either olfactory (digging medium odor) or tactile (digging medium texture) cues. The rewarded cue in the same dimension is switched to test for intra-dimensional shifting. The rewarded cue is changed to the different dimension to test for extra-dimensional shifting. (B) Spatial reversal in Morris water maze. After learning to locate a submerged platform using distal spatial cues (see Figure 1E), the platform location is moved to the opposite side of the pool to test for reversal. (C) Spatial reversal in T-maze. After learning to locate a food reward in one goal arm using distal spatial cues, the rewarded goal arm is switched to test for reversal. (D) Reversal of discriminated operant response in a bar-pressing system. After learning to press a lever for reward based on a visual (e.g., light) or spatial (lever location) cue, the rewarded cue is switched to test for reversal. (E) Reversal of discriminated operant response in a touchscreen-based system. Reversal of a discriminated operant response can also be tested using a touchscreen-based system (for details, see Figure 3). Reversal learning provides another measure of cognitive flexibility. Subjects first learn a stimulus-reward association, then the reward association is switched. Although reversal learning has been described as hierarchically less complex than set-shifting, it requires flexible switching between cues within the same perceptual dimension or modality, and taxes multiple executive functions, including attention, working memory and response inhibition (Roberts, 2006). In addition, reversal learning paradigms allow for a direct measure of perseverative responding to a previously rewarded stimuli or cue, as it is present, but unrewarded, during the reversal phase. This is particularly pertinent because perseveration, possibly caused by insensitivity to negative feedback (i.e., non-reward) following incorrect responding, is a feature of impaired reversal in schizophrenia (Leeson et al., 2009)."}
0_colil
{"project":"0_colil","denotations":[{"id":"20859447-8941956-266772","span":{"begin":397,"end":401},"obj":"8941956"},{"id":"20859447-11283309-266773","span":{"begin":421,"end":425},"obj":"11283309"},{"id":"20859447-19110006-266774","span":{"begin":444,"end":448},"obj":"19110006"},{"id":"20859447-16107585-266775","span":{"begin":520,"end":524},"obj":"16107585"},{"id":"20859447-16960576-266776","span":{"begin":553,"end":557},"obj":"16960576"},{"id":"20859447-19634031-266777","span":{"begin":571,"end":575},"obj":"19634031"},{"id":"20859447-18874598-266778","span":{"begin":943,"end":947},"obj":"18874598"},{"id":"20859447-10818167-266779","span":{"begin":1830,"end":1834},"obj":"10818167"},{"id":"20859447-11853862-266780","span":{"begin":1863,"end":1867},"obj":"11853862"},{"id":"20859447-16842867-266781","span":{"begin":1884,"end":1888},"obj":"16842867"},{"id":"20859447-18971455-266782","span":{"begin":1909,"end":1913},"obj":"18971455"},{"id":"20859447-10818167-266783","span":{"begin":2078,"end":2082},"obj":"10818167"},{"id":"20859447-18971455-266784","span":{"begin":2113,"end":2117},"obj":"18971455"},{"id":"20859447-15998206-266785","span":{"begin":2410,"end":2414},"obj":"15998206"},{"id":"20859447-16380289-266786","span":{"begin":4134,"end":4138},"obj":"16380289"},{"id":"20859447-19576575-266787","span":{"begin":4565,"end":4569},"obj":"19576575"}],"text":"Mouse Assays for Cognitive Flexibility\nExecutive functions subserve the selection and processing of information necessary to plan, control and direct behavior in a manner appropriate to current environmental demands. Across species, these processes are mediated by neural circuitry including the prefrontal cortex (PFC) and interconnected subcortical regions, such as the striatum (Goldman-Rakic, 1996; Miller and Cohen, 2001; Floresco et al., 2009). Pathology in these circuits is a hallmark of schizophrenia (Robbins, 2005; Lewis and Gonzalez-Burgos, 2006; Tan et al., 2009), and is specifically tied to the profound executive deficits exhibited by schizophrenic patients on measures of cognitive flexibility described below.\nCognitive flexibility is a critical executive function that can be broadly defined as the ability to adapt behaviors in response to changes in the environment. The Wisconsin Card Sorting Task (WCST; Grant and Berg, 1948) has been one of the more commonly employed assays for impaired cognitive flexibility in schizophrenic patients, and analogous versions have been developed for use in lower species, including mice (Table 2). In essence, these tasks involve the subject selecting between stimuli, which vary from one another in more than one perceptual dimension (or “set”), and being reinforced for choosing a stimulus based upon one specific dimension alone, e.g., odor. During an “intra-dimensional shift” (IDS), the form of the dimension the subject must choose is changed by the experimenter, e.g., from cinnamon to chocolate odor. In an “extra-dimensional shift” (EDS), the correct dimension is changed altogether, such that choices must be guided by the new dimension (texture) while ignoring the previously rewarded dimension.\nIn a rodent IDS/EDS analog of the WCST, rats (Birrell and Brown, 2000) or mice (Colacicco et al., 2002; Garner et al., 2006; Bissonette et al., 2008) dig in sand to make choices based on the dimension of texture or smell (Figure 2A). As in humans, this task is sensitive to PFC damage in rats (Birrell and Brown, 2000) and mice (Bissonette et al., 2008). One potentially salient difference with the human procedure is that the dimensions are in different sensory modalities [note: attempts to date to develop a IDS/EDS task using a single (visual) modality have not demonstrated the formation of an attentional set in the mouse (Brigman et al., 2005)].\nFigure 2 Mouse behavioral assays for cognitive flexibility. (A) Intra-dimensional/extra-dimensional digging task. Mice are trained to dig for food reward using either olfactory (digging medium odor) or tactile (digging medium texture) cues. The rewarded cue in the same dimension is switched to test for intra-dimensional shifting. The rewarded cue is changed to the different dimension to test for extra-dimensional shifting. (B) Spatial reversal in Morris water maze. After learning to locate a submerged platform using distal spatial cues (see Figure 1E), the platform location is moved to the opposite side of the pool to test for reversal. (C) Spatial reversal in T-maze. After learning to locate a food reward in one goal arm using distal spatial cues, the rewarded goal arm is switched to test for reversal. (D) Reversal of discriminated operant response in a bar-pressing system. After learning to press a lever for reward based on a visual (e.g., light) or spatial (lever location) cue, the rewarded cue is switched to test for reversal. (E) Reversal of discriminated operant response in a touchscreen-based system. Reversal of a discriminated operant response can also be tested using a touchscreen-based system (for details, see Figure 3). Reversal learning provides another measure of cognitive flexibility. Subjects first learn a stimulus-reward association, then the reward association is switched. Although reversal learning has been described as hierarchically less complex than set-shifting, it requires flexible switching between cues within the same perceptual dimension or modality, and taxes multiple executive functions, including attention, working memory and response inhibition (Roberts, 2006). In addition, reversal learning paradigms allow for a direct measure of perseverative responding to a previously rewarded stimuli or cue, as it is present, but unrewarded, during the reversal phase. This is particularly pertinent because perseveration, possibly caused by insensitivity to negative feedback (i.e., non-reward) following incorrect responding, is a feature of impaired reversal in schizophrenia (Leeson et al., 2009)."}
2_test
{"project":"2_test","denotations":[{"id":"20859447-8941956-38208213","span":{"begin":397,"end":401},"obj":"8941956"},{"id":"20859447-11283309-38208214","span":{"begin":421,"end":425},"obj":"11283309"},{"id":"20859447-19110006-38208215","span":{"begin":444,"end":448},"obj":"19110006"},{"id":"20859447-16107585-38208216","span":{"begin":520,"end":524},"obj":"16107585"},{"id":"20859447-16960576-38208217","span":{"begin":553,"end":557},"obj":"16960576"},{"id":"20859447-19634031-38208218","span":{"begin":571,"end":575},"obj":"19634031"},{"id":"20859447-18874598-38208219","span":{"begin":943,"end":947},"obj":"18874598"},{"id":"20859447-10818167-38208220","span":{"begin":1830,"end":1834},"obj":"10818167"},{"id":"20859447-11853862-38208221","span":{"begin":1863,"end":1867},"obj":"11853862"},{"id":"20859447-16842867-38208222","span":{"begin":1884,"end":1888},"obj":"16842867"},{"id":"20859447-18971455-38208223","span":{"begin":1909,"end":1913},"obj":"18971455"},{"id":"20859447-10818167-38208224","span":{"begin":2078,"end":2082},"obj":"10818167"},{"id":"20859447-18971455-38208225","span":{"begin":2113,"end":2117},"obj":"18971455"},{"id":"20859447-15998206-38208226","span":{"begin":2410,"end":2414},"obj":"15998206"},{"id":"20859447-16380289-38208227","span":{"begin":4134,"end":4138},"obj":"16380289"},{"id":"20859447-19576575-38208228","span":{"begin":4565,"end":4569},"obj":"19576575"}],"text":"Mouse Assays for Cognitive Flexibility\nExecutive functions subserve the selection and processing of information necessary to plan, control and direct behavior in a manner appropriate to current environmental demands. Across species, these processes are mediated by neural circuitry including the prefrontal cortex (PFC) and interconnected subcortical regions, such as the striatum (Goldman-Rakic, 1996; Miller and Cohen, 2001; Floresco et al., 2009). Pathology in these circuits is a hallmark of schizophrenia (Robbins, 2005; Lewis and Gonzalez-Burgos, 2006; Tan et al., 2009), and is specifically tied to the profound executive deficits exhibited by schizophrenic patients on measures of cognitive flexibility described below.\nCognitive flexibility is a critical executive function that can be broadly defined as the ability to adapt behaviors in response to changes in the environment. The Wisconsin Card Sorting Task (WCST; Grant and Berg, 1948) has been one of the more commonly employed assays for impaired cognitive flexibility in schizophrenic patients, and analogous versions have been developed for use in lower species, including mice (Table 2). In essence, these tasks involve the subject selecting between stimuli, which vary from one another in more than one perceptual dimension (or “set”), and being reinforced for choosing a stimulus based upon one specific dimension alone, e.g., odor. During an “intra-dimensional shift” (IDS), the form of the dimension the subject must choose is changed by the experimenter, e.g., from cinnamon to chocolate odor. In an “extra-dimensional shift” (EDS), the correct dimension is changed altogether, such that choices must be guided by the new dimension (texture) while ignoring the previously rewarded dimension.\nIn a rodent IDS/EDS analog of the WCST, rats (Birrell and Brown, 2000) or mice (Colacicco et al., 2002; Garner et al., 2006; Bissonette et al., 2008) dig in sand to make choices based on the dimension of texture or smell (Figure 2A). As in humans, this task is sensitive to PFC damage in rats (Birrell and Brown, 2000) and mice (Bissonette et al., 2008). One potentially salient difference with the human procedure is that the dimensions are in different sensory modalities [note: attempts to date to develop a IDS/EDS task using a single (visual) modality have not demonstrated the formation of an attentional set in the mouse (Brigman et al., 2005)].\nFigure 2 Mouse behavioral assays for cognitive flexibility. (A) Intra-dimensional/extra-dimensional digging task. Mice are trained to dig for food reward using either olfactory (digging medium odor) or tactile (digging medium texture) cues. The rewarded cue in the same dimension is switched to test for intra-dimensional shifting. The rewarded cue is changed to the different dimension to test for extra-dimensional shifting. (B) Spatial reversal in Morris water maze. After learning to locate a submerged platform using distal spatial cues (see Figure 1E), the platform location is moved to the opposite side of the pool to test for reversal. (C) Spatial reversal in T-maze. After learning to locate a food reward in one goal arm using distal spatial cues, the rewarded goal arm is switched to test for reversal. (D) Reversal of discriminated operant response in a bar-pressing system. After learning to press a lever for reward based on a visual (e.g., light) or spatial (lever location) cue, the rewarded cue is switched to test for reversal. (E) Reversal of discriminated operant response in a touchscreen-based system. Reversal of a discriminated operant response can also be tested using a touchscreen-based system (for details, see Figure 3). Reversal learning provides another measure of cognitive flexibility. Subjects first learn a stimulus-reward association, then the reward association is switched. Although reversal learning has been described as hierarchically less complex than set-shifting, it requires flexible switching between cues within the same perceptual dimension or modality, and taxes multiple executive functions, including attention, working memory and response inhibition (Roberts, 2006). In addition, reversal learning paradigms allow for a direct measure of perseverative responding to a previously rewarded stimuli or cue, as it is present, but unrewarded, during the reversal phase. This is particularly pertinent because perseveration, possibly caused by insensitivity to negative feedback (i.e., non-reward) following incorrect responding, is a feature of impaired reversal in schizophrenia (Leeson et al., 2009)."}