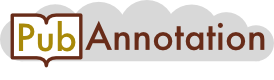
PMC:2726282 / 2841-23541
Annnotations
2_test
{"project":"2_test","denotations":[{"id":"18599074-16381895-62517914","span":{"begin":300,"end":301},"obj":"16381895"},{"id":"18599074-11967538-62517915","span":{"begin":462,"end":464},"obj":"11967538"},{"id":"18599074-15604458-62517915","span":{"begin":462,"end":464},"obj":"15604458"},{"id":"18599074-10734204-62517916","span":{"begin":794,"end":796},"obj":"10734204"},{"id":"18599074-12582243-62517916","span":{"begin":794,"end":796},"obj":"12582243"},{"id":"18599074-14572541-62517917","span":{"begin":1066,"end":1068},"obj":"14572541"},{"id":"18599074-14572541-62517918","span":{"begin":1151,"end":1153},"obj":"14572541"},{"id":"18599074-9670816-62517919","span":{"begin":2427,"end":2429},"obj":"9670816"},{"id":"18599074-10734204-62517920","span":{"begin":3013,"end":3015},"obj":"10734204"},{"id":"18599074-16772031-62517920","span":{"begin":3013,"end":3015},"obj":"16772031"},{"id":"18599074-10412974-62517920","span":{"begin":3013,"end":3015},"obj":"10412974"},{"id":"18599074-17675376-62517921","span":{"begin":4478,"end":4480},"obj":"17675376"},{"id":"18599074-15465042-62517922","span":{"begin":6238,"end":6240},"obj":"15465042"},{"id":"18599074-10672184-62517922","span":{"begin":6238,"end":6240},"obj":"10672184"},{"id":"18599074-15504398-62517922","span":{"begin":6238,"end":6240},"obj":"15504398"},{"id":"18599074-11967538-62517923","span":{"begin":7161,"end":7163},"obj":"11967538"},{"id":"18599074-14530388-62517923","span":{"begin":7161,"end":7163},"obj":"14530388"},{"id":"18599074-14530388-62517924","span":{"begin":7263,"end":7265},"obj":"14530388"},{"id":"18599074-14530388-62517925","span":{"begin":7664,"end":7666},"obj":"14530388"},{"id":"18599074-14530388-62517926","span":{"begin":7874,"end":7876},"obj":"14530388"},{"id":"18599074-17933780-62517926","span":{"begin":7874,"end":7876},"obj":"17933780"},{"id":"18599074-17510665-62517926","span":{"begin":7874,"end":7876},"obj":"17510665"},{"id":"18599074-17158705-62517927","span":{"begin":8849,"end":8851},"obj":"17158705"},{"id":"18599074-16406067-62517928","span":{"begin":9349,"end":9351},"obj":"16406067"},{"id":"18599074-16204860-62517928","span":{"begin":9349,"end":9351},"obj":"16204860"},{"id":"18599074-9891794-62517929","span":{"begin":9693,"end":9695},"obj":"9891794"},{"id":"18599074-15738412-62517929","span":{"begin":9693,"end":9695},"obj":"15738412"},{"id":"18599074-11934612-62517930","span":{"begin":9912,"end":9914},"obj":"11934612"},{"id":"18599074-16385110-62517930","span":{"begin":9912,"end":9914},"obj":"16385110"},{"id":"18599074-17114252-62517930","span":{"begin":9912,"end":9914},"obj":"17114252"},{"id":"18599074-11719226-62517930","span":{"begin":9912,"end":9914},"obj":"11719226"},{"id":"18599074-16348384-62517930","span":{"begin":9912,"end":9914},"obj":"16348384"},{"id":"18599074-15096544-62517930","span":{"begin":9912,"end":9914},"obj":"15096544"},{"id":"18599074-11408658-62517931","span":{"begin":12050,"end":12052},"obj":"11408658"},{"id":"18599074-15186773-62517931","span":{"begin":12050,"end":12052},"obj":"15186773"},{"id":"18599074-12208995-62517932","span":{"begin":12212,"end":12214},"obj":"12208995"},{"id":"18599074-9893020-62517933","span":{"begin":12352,"end":12354},"obj":"9893020"},{"id":"18599074-9287021-62517933","span":{"begin":12352,"end":12354},"obj":"9287021"},{"id":"18599074-12730325-62517933","span":{"begin":12352,"end":12354},"obj":"12730325"},{"id":"18599074-11018124-62517934","span":{"begin":13147,"end":13149},"obj":"11018124"},{"id":"18599074-12142477-62517934","span":{"begin":13147,"end":13149},"obj":"12142477"},{"id":"18599074-12730325-62517934","span":{"begin":13147,"end":13149},"obj":"12730325"},{"id":"18599074-16311037-62517935","span":{"begin":13283,"end":13284},"obj":"16311037"},{"id":"18599074-12142477-62517936","span":{"begin":14144,"end":14146},"obj":"12142477"},{"id":"18599074-10691740-62517936","span":{"begin":14144,"end":14146},"obj":"10691740"},{"id":"18599074-11807075-62517937","span":{"begin":14469,"end":14471},"obj":"11807075"},{"id":"18599074-14731270-62517938","span":{"begin":14775,"end":14777},"obj":"14731270"},{"id":"18599074-11398398-62517938","span":{"begin":14775,"end":14777},"obj":"11398398"},{"id":"18599074-11717281-62517938","span":{"begin":14775,"end":14777},"obj":"11717281"},{"id":"18599074-9287021-62517939","span":{"begin":15403,"end":15405},"obj":"9287021"},{"id":"18599074-16879983-62517939","span":{"begin":15403,"end":15405},"obj":"16879983"},{"id":"18599074-16963779-62517939","span":{"begin":15403,"end":15405},"obj":"16963779"},{"id":"18599074-15345459-62517940","span":{"begin":16190,"end":16192},"obj":"15345459"},{"id":"18599074-15716448-62517941","span":{"begin":16584,"end":16586},"obj":"15716448"},{"id":"18599074-16776655-62517942","span":{"begin":16650,"end":16652},"obj":"16776655"},{"id":"18599074-17185552-62517942","span":{"begin":16650,"end":16652},"obj":"17185552"},{"id":"18599074-16239477-62517943","span":{"begin":17353,"end":17355},"obj":"16239477"},{"id":"18599074-16554821-62517943","span":{"begin":17353,"end":17355},"obj":"16554821"},{"id":"18599074-15889097-62517943","span":{"begin":17353,"end":17355},"obj":"15889097"},{"id":"18599074-11891111-62517943","span":{"begin":17353,"end":17355},"obj":"11891111"},{"id":"18599074-15063859-62517943","span":{"begin":17353,"end":17355},"obj":"15063859"},{"id":"18599074-10742912-62517943","span":{"begin":17353,"end":17355},"obj":"10742912"},{"id":"18599074-9654035-62517943","span":{"begin":17353,"end":17355},"obj":"9654035"},{"id":"18599074-14708014-62517943","span":{"begin":17353,"end":17355},"obj":"14708014"},{"id":"18599074-15129285-62517944","span":{"begin":18004,"end":18006},"obj":"15129285"},{"id":"18599074-17187058-62517944","span":{"begin":18004,"end":18006},"obj":"17187058"},{"id":"18599074-15705577-62517944","span":{"begin":18004,"end":18006},"obj":"15705577"},{"id":"18599074-18421347-62517945","span":{"begin":18973,"end":18975},"obj":"18421347"},{"id":"18599074-16840530-62517946","span":{"begin":19304,"end":19306},"obj":"16840530"},{"id":"18599074-16840530-62517947","span":{"begin":19653,"end":19655},"obj":"16840530"},{"id":"18599074-16530225-62517947","span":{"begin":19653,"end":19655},"obj":"16530225"},{"id":"18599074-17617412-62517947","span":{"begin":19653,"end":19655},"obj":"17617412"}],"text":"Results and Discussion\n\nTopology of E. coli cross-regulatory transcriptional network\nAvailable experimental data point to more than 3000 regulatory interactions between TFs and their regulated genes in E. coli. This information is integrated and documented in a specialised database called RegulonDB.8 Global analyses of this huge network have already been published, emphasising a hierarchical organisation and statistically overrepresented regulatory motifs.9–11 Here, our aim is to analyse the flow of regulatory information within the network of transcriptional interactions among TFs and sigmas (E. coli transcriptional cross-regulatory network). This network encompasses 115 TFs and 7 sigma factors, i.e., around one-third of the total predicted TF proteins in this bacterium (Fig. 1).12,13 On average, every TF is connected to two other TFs (i.e., more technically, the mean degree of the regulatory graph is 2.74). However, the connectivity distribution of TFs is not uniform, with a small fraction of global TFs with high out-degrees dominating the network.15 Seven global regulators were defined previously based on a collection of criteria:15 (i) number of regulated genes; (ii) number of regulated genes encoding for TFs; (iii) propensity of cooperative regulation of targets with the aid of other TFs; (iv) ability to directly affect the expression of a variety of promoters that use different sigma factors; (v) belonging to evolutionary families with few paralogs; and (vi) heterogeneity of the functional classes of the regulated genes.\nIn order to better visualise the informational flow through the network, the following graphical conventions have been used in Fig. 1 (see also legend): (i) the size of the nodes representing TFs is proportional to the number of genes they regulate [e.g., cAMP receptor protein (CRP) regulates 413 genes and is represented by the second biggest node, after the housekeeping sigma factor rpoD]; (ii) arrows and colours refer to the direction and sign of the regulatory interaction; (iii) arrow thickness is proportional to the impact of the interaction, computed as the number of genes thereby (in)directly regulated.\nThe majority of the TFs in this network are autoregulated (∼ 70%), of which about two-thirds account for negative loops (see Table 1). This finding is consistent with the results of an analysis performed with a much smaller number of TFs about 10 years ago.17 This predominance of negative autoregulatory loops contrasts with the predominance of positive arcs between different TFs (about 54%, see Table 1). The dominance of positive regulatory interactions in the regulatory network of E. coli is not limited to those among TFs, as when we compute the regulation of all the target genes (3017 arcs) we found that about 54% (1630) are positively regulated, 40% (1206) are repressed, while about 6% (171) are dual regulated. This is especially interesting because a majority of the TFs in bacteria have been reported to act as repressors.12,18,19 The conventions used in Fig. 1 clearly display the hierarchical organisation of the network, with master regulators such as CRP, fumarate and nitrate regulatory protein (FNR) or integration host factor (IHF) each (in)directly regulating a large number of other TFs. Furthermore, the layout emphasises important variations regarding the length of the transcriptional cascades.\nAlthough functional annotations on TFs are still limited, it is possible to classify the cross-regulating TFs into broad categories according to the physiological functions of the target structural genes: carbohydrate initial catabolism, respiration, biofilm formation and chemotaxis, etc. As shown in Fig. 1, these broad classes correspond to different local network topologies. Due to their contrasting topologies, in what follows, we will focus our discussion on short regulatory cascades observed in the case of carbohydrate catabolism as opposed to long regulatory cascades seen in the case of biofilm and chemotaxis pathways (Fig. 2a). CRP resides at the top of both subnetworks. CRP is the only global TF acting hierarchically over local TFs for the usage of carbohydrates, whereas CRP's activity is comparable to the activity of other global regulators in the rest of the network. Note that the concentration of its effector metabolite, cyclic adenosine monophosphate (cAMP), is at par with that of adenosine triphosphate (ATP), which acts as the energetic currency of the cell.20 This suggests that CRP not only regulates the use of these substrates for producing ATP, but also senses the energetic status of the cell to decide the execution of other cellular programs.\nThis study aims at understanding the network structure in relation to physiological roles played by the different modules, focusing on differences in the topologies of the subnetworks controlling metabolism versus motility and chemotaxis (cf. the following sections).\nHowever, other subnetworks are also worth mentioning. In particular, all nine TFs controlling the expression of genes for amino acid biosynthesis seem to be expressed constitutively by sigma 70. Each TF regulates the transcription of the required genes for producing different amino acids. At high concentrations of the amino acids, allosteric modifications of TFs follow binding to their respective amino acids, resulting in TF autorepression as well as to the repression of the corresponding biosynthetic genes. Interestingly, the logic behind negative autoregulation in this case is different from that of the catabolism of carbohydrates. While in the latter case TFs are autorepressed until the substrate is available, in the case of amino acids, TFs are autorepressed only in the presence of an excess of the synthesized final product. Another interesting subnetwork is that for alleviating the stresses by drugs, solvents and weak organic acids. The regulatory logic in this complex subnetwork is peculiar, as their components form multi-element circuits (see Fig. 3) and their inputs are directed by Rob and SoxR, two small proteins constitutively expressed but with very short half-lives (1–2 min). Their stability/degradation depends on the presence/absence of their effector signals.21,22,23\n\nMultiple parallel feed-forward loops regulate the use of different carbon sources\nCellular feeding, which includes the uptake of carbon and energy sources and their metabolism, can be considered as one of the main physiological processes in bacterial systems. The regulation of these processes directly affects cellular fitness. The selection of carbon sources is regulated by CRP and about 20 more specific TFs (Fig. 2a). The hierarchical organisation of the corresponding subnetwork is characterized by a short average path length (cf. Table 1). Regulatory interactions between CRP and the specific TFs result in the occurrence of multiple feed-forward loops (FFLs) for the use of alternative sugar sources. FFL is a network motif recurrently found in transcriptional networks and is defined as a three-gene pattern composed of two input TFs, one of which regulates the other, both jointly regulating a target gene.9,24 Based on the mode of regulation of each TF, this motif is subdivided into eight different subtypes.24 Coherent FFL type 1 corresponds to all the regulatory interactions in the motif being positive; in incoherent type 1 FFL, the first TF regulates positively both the targets, although the second TF represses the expression of the target gene thereby reversing the final effect. The majority of the FFLs present in the subnetwork for carbon catabolism belong to coherent and incoherent type 1 groups,24 with both TFs working together, as a result of a persistent signal affecting the global TF (in this case, cAMP) and the presence of a signal affecting a TF corresponding to a sugar alternative to glucose.24–26 This motif structure enables the filtering of short pulses of the signal affecting the global TF (cAMP) in case of transient glucose deprivation. Consequently, the target structural genes are activated only in the persistent absence of glucose and in the presence of an alternative carbon source.\nThe phosphotransferase system typically transports and phosphorylates certain sugars, including glucose, a preferred carbon source for E. coli, and this condition ultimately results in low levels of cAMP. Consequently, CRP does not activate the transcription of the genes responsible for the degradation of alternative sugars. Note that most structural genes involved in the transport and initial catabolism of alternative carbon sources are encoded in operons, each specifically repressed in the absence of the inducing sugar.\nWhen glucose is lacking, cAMP level increases and CRP can activate the transcription of genes responsible for degrading alternative carbon sources.27 Simultaneously, sugars (or a processed variant thereof) present in the cell bind their specific TF; allosteric interactions then result in TF unbinding from DNA, alleviating the repression and permitting the transcription of the corresponding target genes. This organisation involving multiple parallel FFLs coupled to phosphotransferase activity appears optimal for enabling rapid transcriptional responses to sudden lack of glucose in the presence of alternative carbon sources in the milieu.28,29\n\nLong hierarchical cascades regulate developmental processes\nBiofilm formation and bacterial mobility can be seen as the outcome of specialised cell differentiation pathways. Biofilm formation involves subsequent cellular changes at the morphological and physiological levels resulting in bacterial populations with multiple phenotypes.4,30,31 Furthermore, bacteria living in biofilm communities are present in different developmental stages (at least four defined stages) as has been observed in Cryptoccocus, Pseudomonas, Staphylococcus, Xanthomonas, etc.32–37\nThe part of E. coli transcriptional cross-regulatory network involved in the control of biofilm formation and motility exhibits a relatively complex topology with several long cascades from CRP, IHF and FNR to downstream specialised TFs (see Table 1 and Fig. 2a). Several of these cascades converge on the master regulators for motility and biofilm formation (FhlCD and CsgD, respectively).\nFurthermore, a relatively high proportion of the downstream specialised TFs autoactivate themselves (see below), a feature that is rare at the level of the whole transcriptional network. In addition, we could identify 105 multi-element circuits in the transcriptional regulatory network involving 2 to 14 different elements (including transcription and sigma factors, see Fig. 3 and supplementary material for the complete list of circuits). Interestingly, the information fluxes inside these circuits follow a frequent route in the network with the order CsgD \u003e Cspa \u003e HNS \u003e GadX \u003e RpoS \u003e IHF. From IHF the regulatory flow diverge in two main directions: IHF \u003e RpoH \u003e RpoD and IHF \u003e FIS \u003e CRP. In line with this observation, CRP and RpoD are the major distributors, whereas CsgD and GadX are the main collectors of information. These multi-element circuits inside the cross-regulatory network are novel observations. The functional relevance of these regulatory structures remains to be assessed experimentally. Tentatively, these circuits may implement a feedback between the presence of different stresses and the basic machinery for replication and growth.\nCsgD, the master regulator for biofilm formation, is directly involved in 28 of these long circuits, suggesting a particular tight coupling of CsgD activity with the intracellular status. In contrast, FlhCD, the master compound regulator for motility and chemotaxis, is known to be regulated by nine other TFs but has not yet been reported to regulate any other TF.\nNote that the motility module has its own sigma factor, FliA, regulated by FlhCD. FliA is required for the transcription of the genes required for the last part of flagella development and for chemotaxis machinery.38,39 In contrast, the genes for biofilm development are transcribed by the housekeeping sigma 70 and RpoS, the sigma factor expressed in response to general stress.40\nThe execution of such long regulatory cascades requires time. Indeed, complete flagella assembly may take a generation time or longer.41–43 The occurrence of positively autoregulated TFs at several intermediate steps enables informed decisions about the cellular/environmental condition. In some conditions, cellular duplication might be faster than the conclusion of a long regulatory cascade. This implies that bacterial populations likely consist of mixtures of bacteria with transcriptional programs at different levels in long regulatory cascades.\n\nMultiple conditions regulate the first steps of developmental processes in E. coli\nBoth biofilm and motility are regulated by multiple, long regulatory cascades (Fig. 2a). This organisation is congruent with the observation that these developmental processes are regulated by multiple environmental conditions, ranging from nutritional deprivation to environmental stresses.6,7,43 In E. coli, several global TFs sit at the top in these regulatory cascades, namely, CRP, IHF and FNR, all sensing endogenous signals.2 Additional regulatory inputs are provided by more specialised TFs such as ArcA (aerobic respiration regulatory protein), EvgA (environmentally responsive activator) and TorR (trimethylamine N-oxide reductase regulator) involved in exogenous sensing. Remarkably, downstream players are enriched in two-component system partners: RcsAB (capsule biosynthesis regulator), OmpR (outer membrane protein regulator), CpxR (regulator of cell envelope proteins folding and degradation) and QseB (quorum sensing regulator) (see the next section). Consequently, the first developmental steps likely occur in most of the cellular population in response to nutritional (CRP) or respiration (FNR-ArcA) stresses, yet depending on nucleoid structure status (sensed through IHF), while the final steps critically depend on exogenous conditions, as observed in other bacteria.7,44 For instance, in Pseudomonas aeruginosa, the initial steps for biofilm development (motility and reversible attachment to solid surfaces) are independent of quorum sensing, whereas the final steps (irreversible attachment and biofilm maturation) are strongly dependent on quorum-sensing regulation, an exogenous condition.45 Both processes (motility and biofilm) must be tightly interconnected, since motility is important for the concerted movement of groups of bacteria over solid surfaces, followed by cell membrane modification, excretion of polysaccharides, causing them to be less motile and smaller in mature biofilms.46–48 Interestingly, in E. coli, the activation of nucleoid-associated proteins such as histone-like protein (HNS) occurs relatively early along both biofilm and chemotaxis developmental pathways. The expression of these nucleoid-associated proteins is known to be growth-phase dependent (HNS is mostly expressed in the exponential phase, whereas IHF and factor for inversion stimulation (FIS) are expressed in arrested growing cells and during the early exponential phase, respectively), pointing to an influence of nucleoid structure and specific bacterial growth phases on the first steps of these developmental processes.42,49,50\n\nExternal input information defines the last steps of the developmental processes\nDownstream of biofilm and chemotaxis developmental cascades, transcriptional autoactivations and cross-inhibitions become more frequent (Figs. 1 and 2a). This part of the network comprises several two-component systems, enabling the sensing of external information. In particular, OmpR is responsible for switching the phenotype between biofilm and motility (activating the first and repressing the latter) depending on external stresses. During stressing conditions as well as in mature biofilms, cells tend to be smaller compared to rapidly growing cells. This phenotypic transition depends on the expression of BolA (cell morphogenetic regulator), which is transcribed by RpoS and repressed by OmpR.51\nThe activation of the master gene for motility, FlhCD, is further controlled by the quorum-sensing detector QseB, while the activity of the master regulator for biolfilm formation, CsgD, is repressed in the presence of extracellular stressing conditions, detected by CpxR. In addition, the BaeSR system controls the expression of export complexes, conferring multidrug resistance phenotypes,52 while RcsB mediates the glutamate-dependent acid resistance.53,54 QseB, CpxR, BaeR, OmpR and RcsAB are all members of two-component systems that are modified by protein–protein phosphorylation, enabling quick response in comparison to de novo protein production along transcriptional cascades. Strikingly, almost all of these two-component TFs (except OmpR) autoactivate their expression, an observation fitting the contention that positive feedback circuits are necessarily found at the core of all differentiation switches. Indeed, such TF autoactivations likely enable persistent TF expression in two stable states: “on” or “off”. Thus, they constitute a potentially robust machinery for all-or-nothing output response depending on transient exogenous signals.55–62\n\nExpression of master regulators\nIn the preceding sections, we have emphasised the hierarchical organisation as well as important local structural variations in E. coli transcriptional network. In the subsequent section, we consider the expression of a TF as a function of its position in the regulatory hierarchy. Although it is difficult to address this at the protein level because of the current relative scarcity of proteomic data, this question can be readily addressed at the level of transcription. Therefore, we have analysed the levels of mRNA reported by two independent experiments on E. coli cells grown on minimal medium + glucose.63–65 In summary, the mean mRNA levels of global TFs such as CRP, FNR, ArcA and IHF are significantly higher than those of more specialised regulators (Fig. 2b). This result supports the contention that the master regulators are continuously required at high levels to bind to numerous DNA sites across the genome, whereas downstream, specialised TFs can be expressed or not depending on the environmental conditions. Indeed, in conditions favourable to planktonic growth (as in the condition for mRNA quantification mentioned above), TFs controlling the final stages of biofilm/motility development are lowly expressed. Similarly, the TFs controlling specific sugar catabolism regulons show much lower mean concentration compared to CRP, suggesting that these TFs are sporadically required, depending on the metabolic state of the cell. A similar tendency has been recently observed by measuring the fluorescence of 15 different TF–green fluorescent protein gene fusions.66\n\nConservation of the transcriptional cross-regulatory network in bacteria\nTo what extent can our observations on E. coli transcriptional network be generalised to other bacteria? To address this question, we have compiled orthologs of E. coli TFs across 216 non-redundant bacterial genomes (using the approach described in Ref. 67) and analysed their conservation. As a general observation, global regulators (and negatively autoregulated TFs) of E. coli tend to be more conserved than more specialised regulators (see Fig. 4). In spite of this observation, previous studies indicated that even the TFs found at the top of regulatory hierarchies vary across bacterial phyla.67–69 Intriguingly, orthologs of CsgD and FlhCD are found in less than 25 genomes, whereas orthologs for OmpR and FliA are found in more than 75 genomes. More precisely, FliA appears to be conserved in many organisms lacking FlhCD orthologs, although FlhCD is involved in the transcriptional regulation of FliA in E. coli. Tentatively, in these organisms, a different TF might regulate FliA. Alternatively, FliA alone might control the transcription of genes required in the final stages of motility and chemotaxis, or FliA might be directing the transcription of genes for unrelated functions in these bacteria. This set of regulatory components and the corresponding network structure are likely to be conserved in a relatively small group of bacteria (less than 25 bacteria closely related to E. coli). However, the general network organisation (long regulatory cascades versus multiple FFLs, and endogenous versus exogenous sensing) apparently constitute a common theme for the regulation of physiological and developmental processes in bacteria."}