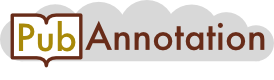
PMC:1702488 / 31129-31132
Evidence of the neuron-restrictive silencer factor (NRSF) interaction with Sp3 and its synergic repression to the mu opioid receptor (MOR) gene
Abstract
Previously, we reported that the neuron-restrictive silencer element (NRSE) of mu opioid receptor (MOR) functions as a critical regulator to repress the MOR transcription in specific neuronal cells, depending on neuron-restriction silence factor (NRSF) expression levels [C.S.Kim, C.K.Hwang, H.S.Choi, K.Y.Song, P.Y.Law, L.N.Wei and H.H.Loh (2004) J. Biol. Chem., 279, 46464–46473]. Herein, we identify a conserved GC sequence next to NRSE region in the mouse MOR gene. The inhibition of Sp family factors binding to this GC box by mithramycin A led to a significant increase in the endogenous MOR transcription. In the co-immunoprecipitation experiment, NRSF interacted with the full-length Sp3 factor, but not with Sp1 or two short Sp3 isoforms. The sequence specific and functional binding by Sp3 at this GC box was confirmed by in vitro gel-shift assays using either in vitro translated proteins or nuclear extract, and by in vivo chromatin immunoprecipitation assays. Transient transfection assays showed that Sp3-binding site of the MOR gene is a functionally synergic repressor element with NRSE in NS20Y cells, but not in the NRSF negative PC12 cells. The results suggest that the synergic interaction between NRSF and Sp3 is required to negatively regulate MOR gene transcription and that transcription of MOR gene would be governed by the context of available transcription factors rather than by a master regulator.
INTRODUCTION
The mu opioid receptor (MOR) plays an important role in mediating the actions of morphine and morphine-like drugs. Based largely on pharmacological and clinical observations, MOR has traditionally been considered the main site of interaction of the major clinically used analgesics, particularly morphine (1). Three major types of opioid receptors, μ, δ and κ, have been cloned and shown to belong to the G-protein-coupled receptor superfamily (2). Regulation of the opioid receptor gene expression may be in response to fluctuating levels of various agents in certain brain regions. Thus, study of the mechanism underlying the transcriptional regulation of opioid receptor genes may facilitate elucidation of the spatial and temporal expressions and the modulation of expression in different physiological states.
The expression of mouse MOR gene is known to be regulated by various cis-acting elements and trans-acting factors, which are important for promoter activity (3–9). It has been reported that a major species of mRNA and polyadenylation signal of MOR has promoter-dependent functional activity (10). We have also reported that MOR transcription is suppressed by the neuron-restrictive silencer element (NRSE) in the mouse MOR promoter through binding of neuron-restriction silence factor (NRSF) (11).
In both non-neuronal and neuronal cells, NRSF silences the expression of its target genes by its two independently acting repressor domains (12,13). The N-terminal repressor domain of NRSF has been shown to recruit co-repressors, such as mSIN3 and histone deacetylases (HDACs), into the vicinity of the promoter. Histone deacetylation leads to a more compact chromatin that prevents accessibility of transcription factors. The C-terminal repressor domain (CTRD) of NRSF has been shown to interact with at least one factor, the transcriptional co-repressor 2 (CoREST) that may serve as a platform protein for the recruitment of molecular machinery that imposes silencing across a chromosomal interval (14,15). CoREST is able to interact with HDAC1/2, indicating that the CTRD also contributes to deacetylation of histones (16).
Some studies have revealed the existence of additional repression mechanisms mediated by NRSF. This has been postulated because HDAC inhibitors fail to derepress the CTRD repression of some NRSF target genes, such as GluR2 receptor and SCG10 (17,18). In addition, overexpression of the dominant-negative NRSF and knock-out of NRSF failed to release the repression of its target gene completely, even though NRSF was originally defined as a repressor for neuronal-specific gene in non-neuronal cells. Therefore, from recent studies, it has become clear that genetic context is extremely important in determining the function of NRSE/NRSF repression mechanisms.
In this study, we have identified a conserved GC box (Sp family binding site) sequence downstream of NRSE region in the mouse MOR gene. Our results have showed that Sp3 specifically binds to this mouse GC box and interacts with NRSF to synergistically repress the MOR expression.
MATERIALS AND METHODS
Cell culture and reporter gene constructs
NS20Y and HeLa cells were routinely grown in DMEM supplemented with 10% heat-inactivated fetal bovine serum (FBS) at 37°C in a humidified atmosphere of 5% CO2. PC12 cells were cultured in 10% CO2 in DMEM with 10% donor horse serum and 5% FBS (7).
The pGL4.7 (−4744 to +1, the translation start site was designated as +1) was generated by ligation of the PCR product (−249 to +1) with the BamHI and NcoI digested pL4.7K (−4744 to −249) (19). PCR was performed using genomic DNA from mouse NS20Y cells as a template and an upstream sense oligonucleotide (5′-GCCTCTGGATCCCTCACAGCCCAT-3′), containing a BamHI site, and a downstream antisense oligonucleotide (5′-GGCGCTGCTGTCCATGGTTCTGAA-3′) containing a NcoI site. The pGL4.7NRSP, pGL4.7preNRmSP, pGL4.7NRSPm and pGL4.7preNRmSPm constructs were generated by ligation of the pGL4.7 DNA digested with NcoI and the double-strand oligomers (pGL4.7NRSP; wild type of NRSE and Sp-binding sequence, pGL4.7NRmSP; mutated NRSE and wild type of Sp-binding sequence, pGL4.7NRSPm; wild type of NRSE and mutated Sp-binding sequence, pGL4.7NRmSPm; mutated NRSE and mutated Sp-binding sequence) containing NcoI site at both 5′ and 3′ ends (for pGL4.7NRSP: 5′-TTCAGAACCATGGACAGCAGCGCGCCGGCCCATGGATTCTTC-3′; for pGL4.7preNRmSP: 5′-TTCAGA ACCATGGA ATAGTTGCGCGCCGGCCCATGGATTCTTC-3′; for pGL4.7NRSPm: 5′-TTCAGAACCATGGACAGCAGCGCATATGCCCATGGATTCTTC -3′; for pGL4.7preNRmSPm: 5′-TTCAGAACCATGGAATAGTTGCGCATATGCCCATGGATTCTTC-3′) (The underlines indicate mutated nucleotides for NRSE and Sp3 core binding sites).
The pGL4.7NRmSP and pGL4.7NRmSPm constructs were finally generated by PCR site-directed mutagenesis using high-fidelity Pfu DNA polymerase according to the manufacturer's protocol (Quikchange TM; Stratagene). In vitro mutagenesis was carried out on MOR promoter linked to luciferase gene reporter (pGL4.7preNRmSP and pGL4.7preNRmSPm) using primers as follows: for pGL4.7NRmSP: 5′-TTCAGAACCATAAAATAGTTGCGCGCCGGCCCATGGATTCTTC-3′; 5′-GAAGAATCCATGGGCCGGCGCGCAACTATTTTATGGTTCTGAA-3′; for pGL4.7NRmSPm: 5′-TTCAGAACCATAAAATAGTTGCGCATATGCCCATGGATTCTTC-3′; 5′-GAAGAATCCATGGGCATATGCGCAACTATTTTATGGTTCTGAA-3′). The mutated nucleotides are underlined.
Total RNA preparation and RT–PCR analysis
Total RNA was isolated according to the supplier's protocol (TRI Reagent; Molecular Research Center, Inc.). For RT–PCR, 2 μg of total RNA was reverse transcribed and PCRs were carried out with MOR-specific primers at the same tube using one-step RT–PCR reagent (Qiagen) in a GeneAmp 9600 PCR machine (Perkin-Elmer). The PCR cycle conditions for MOR consisted of 95°C for 45 s, 60°C for 45 s and 72°C for 45 s, followed by a 10 min extension at 72°C (37 cycles for NS20Y, 33 cycles for PC12 cell). PCR products were separated in a 2.0% agarose gel with TBE buffer. The mouse MOR transcript was amplified with primer set P2Ss (5′-CTCCGTGTACTTCTAAGGTGGGAG-3′) and TM2as (5′-GGCTAAGGCATCTGCCAGAGCAAG-3′). Similar reactions were carried out using primers for β-actin as an internal control. Quantitative analyses were carried out using ImageQuant 5.2 (Amersham) software.
Transient transfection and reporter gene assay
For luciferase assays, 1 × 106 cells/well were cultured overnight before transfection. Various reporter constructs at equimolar concentrations were transfected using Effectene transfection reagent (Qiagen, Valencia, CA) as described previously (11). After 48 h of transfection, cells were washed twice with phosphate-buffered saline (PBS) and lysed with lysis buffer (Promega). For all the assays, pCH110 (β-galactosidase; Amersham Bioscience Inc.) was also co-transfected and measured to normalize transfection efficiency. The luciferase and β-galactosidase activities were determined according to the manufacturer's instructions (Promega and Tropics, Madison, WI).
Real-time PCR
Total RNA was extracted using the TRIzol reagent (Molecular Research Center, Inc.) from NS20Y cells. DNase I-treated RNA (2 μg) was subjected to RT (Roche) using oligo(dT) primer. One-fortieth of this reaction was used for real-time PCR analysis using SYBR Green I dye chemistry. PCR product accumulation was monitored using a iCycler iQ Real-Time detection system (Bio-Rad). The mean cycle threshold value (Ct) from triplicate samples was used to calculate gene expression level. PCR products were normalized to levels of β-actin. Relative gene expression levels were determined as described in User's resource guide from Bio-Rad.
Co-immunoprecipitation and western blots
Cells were lysed in a buffer containing 1% Triton X-100, 10 mM Tris, 5 mM EDTA, 50 mM NaCl, 50 mM NaF, supplemented with 1 mg/ml antipain, 1 mg/ml leupeptin, 100 nM PMSF and 100 nM sodium orthovanadate. Approximately 1 mg of the clarified cell lysate was incubated overnight at 4°C with an anti-Sp1, anti-Sp3 or anti-NRSF monoclonal antibody. Immunoprecipitates were recovered on protein G–Sepharose beads, washed extensively and separated on SDS–PAGE. Proteins were transferred on to PVDF membrane (Amersham Biosciences). Membranes were incubated with anti-Sp3 (sc-644; Santa Cruz Biotechnology), anti-Sp1 (sc-59; Santa Cruz Biotechnology) and anti-NRSF antibody (12C11; kindly provided by Dr David Anderson) and signals were detected using a Storm 840 PhosphorImager system (Amersham Biosciences).
Electrophoretic mobility shift assay
In vitro translation of NRSF and Sp3 was performed using the TNT SP6 and T7 Quick Coupled Transcription/Translation System as per the manufacturer's protocol (Promega) and nuclear extracts from NS20Y cells were prepared as described previously (11). Double-stranded oligonucleotides containing a copy of the MOR NRSE/GC box (5′-CAGCAAGCATTCAGAACCATGGACAGCAGCGCCGGCCCAGGGA-3′) were synthesized and end-labeled using the Klenow fragment of DNA polymerase I in the presence of [γ-32P]dATP. Free nucleotides were separated by centrifugation through a G-25 column (Roche). The end-labeled DNA probes were incubated with in vitro translated NRSF or Sp3 or both and with nuclear extract from NS20Y cells. For competition analysis, a 100-fold molar excess of cold competitor oligonucleotide was added to the mixture before adding the probe. For supershift assay, antibodies or pre-immune serum were pre-incubated for 30 min on ice before adding the labeled probe. Radiolabeled probe was then added and the mixture was incubated for further 20 min on ice. Samples were subjected to electrophoresis on a native 4% polyacrylamide gel run in 0.5× TBE buffer for 2 h at 180 V. Monoclonal antibody against NRSF was obtained from Dr D. J. Anderson. Anti-IRF4 antibody was purchased from Santa Cruz Biotechnology.
Chromatin immunoprecipitation (ChIP) and re-precipitation (re-ChIP)
ChIP assay was performed as described by Kim et al. (11). Briefly, cells were incubated for 15 min in a medium containing 1% formaldehyde at room temperature and cross-linking was quenched by adding glycine to 125 mM. Cells were washed three times with ice-cold Tris-buffered saline (150 mM NaCl and 20 mM Tris–HCl, pH 7.6), resuspended in lysis buffer and disrupted on ice with a sonicator followed by centrifugation at 15 000 g for 10 min to remove cell debris. Gel electrophoresis indicated that a substantial fraction of DNA fragments at this stage are from 0.3 to 3.0 kb in length. ChIP was performed by incubating the cell lysate overnight at 4°C with 4 μg of anti-Sp1, anti-Sp3, anti-HDAC1, anti-HDAC2 or anti-IRF4 (as a non-specific antibody) followed by incubation with protein G Plus–agarose (Santa Cruz Biotechnology) for 2 h. The beads were rinsed four times sequentially with lysis buffer 500 (0.1% deoxycholic acid, 1 mM EDTA, 50 mM HEPES, pH 7.5, 500 mM NaCl and 1% Triton X-100), LiCl/detergent solution (0.5% deoxycholic acid, 1 mM EDTA, 250 mM LiCl, 0.5% NP-40 and 10 mM Tris–HCl, pH 8.0) and TE buffer (10 mM Tris–HCl, pH 8.0 and 1 mM EDTA, pH 8.0). The beads were then incubated for 10 min at 65°C with elution buffer (10 mM EDTA, 1% SDS and 50 mM Tris–HCl, pH 8.0).
For re-ChIP, re-ChIP elution buffer (10 mM EDTA, 50 mM Tris–HCl, pH 8.0 and 0.7 M NaCl) was used. To reverse the cross-linking and purify the DNA, the precipitates were incubated at 65°C overnight and then treated with proteinase K solution for 2 h at 37°C. DNA samples were recovered and resuspended in TE buffer. PCRs were performed using 4 μl of immunoprecipitated chromatin sample with the primers spanning mouse MOR promoter region in 50 μl of total volume. Primers used were NSCF forward, 5′-CTGTGAGAGGAAGAGGCTG-3′ and NSCR reverse, 5′-AAGTTGAGCCAGGAGCCAGGT-3′, which produces 188 bp PCR products.
RESULTS
Mithramycin A up-regulates the endogenous MOR transcription
Previously, we reported that the MOR transcription is suppressed by NRSE in the mouse MOR promoter through binding of NRSF (11). In the current study, we have studied the role of G/C box sequences (GGGGGCGGGGC), identified in mouse, rat and human in adjacent to NRSE, which is a consensus nucleotide-binding sites for the Sp transcription factors (Figure 1A). To assess the functionality of the putative Sp factor binding site on the expression of the MOR genes, NS20Y cells were treated with mithramycin A, a DNA-binding blocking drug that inhibits the binding of transcription factor to G/C-specific regions of DNA. Mithramycin A is a cell-permeable agent that binds GC-rich DNA sequences and is frequently used to explore the sequence dependency of DNA-binding factors (20). As shown in Figure 1B and D, RT–PCR and real-time RT–PCR revealed that the mRNA levels of the MOR were increased by mithramycin A treatment in a dose-dependent manner in the NRSF positive NS20Y cells. However, in the NRSF negative PC12 cells, MOR transcript level was not affected by mithramycin A treatment (Figure 1C). In accordance with other studies, mithramycin A did not change mRNA levels of β-actin (21). These results indicated that the putative Sp-binding site may mainly act as a negative element for the expression of MOR gene in NS20Y cells.
The Sp-binding site and NRSE synergistically repress the expression of MOR gene
Independent studies have shown that Sp1 has functional implication in expression of some of NRSF target genes including N-methyl-d-aspartate receptors, dynamin I, synaptophysin and glutamate receptor ionotropic kainate-5 genes (22–24). For MOR gene regulation, Sp1 factor acts as a major positive regulator by binding two cis-elements (distal and proximal promoters) located at −340 through −300 residues from the translation start site while Sp3 has a minor effect by binding to one of these elements (25). As mentioned above, a computer search identified a G/C box sequence at the downstream of NRSE region. To verify the functionality of this motif in MOR transcription, we generated various MOR/luciferase reporter constructs containing pGL4.7NRSP (wild type), pGL4.7NRmSP (mutated NRSE and wild-type Sp-binding site), pGL4.7NRSPm (wild-type NRSE and mutated Sp-binding site), pGL4.7NRmSPm (mutated both in NRSE and Sp-binding site) (Figure 2A). All the constructs had been generated in more than 4.7 kb MOR promoter region containing distal and proximal elements and possibly other unidentified elements that might have roles in the promoter activity. Thus, the promoter assay in the current study may accurately reveal the role of newly identified GC box in the MOR gene regulation.
The constructs were transiently transfected in NS20Y and PC12 cells separately and the promoter activity was examined by measuring the signal of the reporter gene. As expected, the mutation in either NRSE or GC box sequence increased the expression of reporter gene in NS20Y cells (Figure 2B). Interestingly, when both elements were mutated at the same time, the promoter activity (14-fold in NS20Y cell) was repeatedly greater than the sum of activities from the constructs where each element was mutated singly (4-fold in Sp site mutation and 6-fold in NRSE mutation). Therefore, these data indicate that NRSE and Sp-binding site may cooperate to repress the MOR promoter activity. Meanwhile, the activities of the same reporter constructs in the NRSF negative PC12 cells (judged from the western blot in Figure 2C) had little effect (Figure 2B) on the promoter activity. These data suggest that a putative Sp-binding site acts as a synergic repressor with NRSE in NS20Y cells and NRSF and NRSE plays an important role to decide the function of the newly identified Sp-binding site as a repressive cis-element.
In addition, we also investigated whether the transcriptional repression activity of the putative Sp-binding site in the MOR promoter can be relieved in the NS20Y cells after mithramycin A treatment. NS20Y cells were transiently transfected either with pGL4.7NRSP or pNRSPm constructs (Figure 3A). Twenty-four hours after transfection, the cells were treated with either 100 or 400 nM mithramycin A. When the wild-type promoter sequence (pGL4.7NRSP) was transfected, a dose-dependent increase in the promoter activity was observed (Figure 3B). In contrast, when the putative Sp-binding site was mutated (pGL4.7NRSPm), the high promoter activity was detected even without treatment of mithramycin A strongly supporting the notion that this putative Sp-binding site downregulates expression of the MOR gene. Interestingly, at the highest concentration, mithramycin A started to decrease the promoter activity. At this condition, mithramycin A could interfere the binding of positive transcriptional regulators such as Sp1 within the promoter region.
Sp3 interacts with NRSF and binds G/C box adjacent to NRSE to repress transcription of MOR gene
Co-immunoprecipitation was performed to identify a subclass of Sp factors responsible for the observed repressor activity in the transcription of MOR gene. The lysates from HeLa cells were immunoprecipitated with Sp1, Sp3, NRSF antibody and pre-immune serum (PI) (as a negative control) and subjected to SDS–PAGE followed by western blot analysis with NRSF and Sp3 antibody. The endogenous Sp3 factor and NRSF were co-immunoprecipitated with NRSF and Sp3 antibody, respectively. In contrast, Sp1 did not co-precipitate with NRSF antibody (Figure 4A). The same result was obtained using c-Myc-tagged NRSF expressed in NS20Y cells implying that Sp3 binds the GC box and a direct interaction between Sp3 and NRSF is required for a synergistic repression of MOR gene expression (Figure 4B). In addition, immunoprecipitation experiment revealed that only the full-length Sp3 factor, other than two short Sp3 isoforms (M1 and M2), interact with NRSF (Figure 4C).
To confirm the binding of Sp3 to the putative GC box, we performed a supershift assay with in vitro translated proteins (myc-NRSF, Sp3) and nuclear extract from NS20Y and PC12 cells. In the electrophoretic mobility shift assay (EMSA) with in vitro translated proteins, both NRSF (Figure 5A) and Sp3 (Figure 5B) formed a binary complex with the labeled probe (from −18 to +24 of MOR). In both cases, cold competitor completely eliminated the binary complex indicating the sequence-specific binding of NRSF and Sp3 to NRSE/GC box. Moreover, the super shift of the binary complex were observed when incubated with anti-c-myc or anti-Sp3 antibody demonstrating the specific binding of two transcription factors to the probed DNA sequences. The incubation with PI or IRF-4 (non-specific antibody as a negative control) had no effect in either NRSF or Sp3 bound complex.
Since the binding site in DNA for both NRSF and Sp3 are slightly overlapped and co-immunoprecipitation experiment suggests that NRSF and Sp3 interact with each other, we tested whether both NRSF and Sp3 can bind the labeled probe at the same time without any interference. As shown in Figure 5C, incubation of increased amount of Sp3 to the fixed amount of NRSF generated a new ternary complex indicating that NRSF and Sp3 bind this short MOR promoter sequence. The fact that the NRSF binary complex was remained at the highest amount of Sp3 tested may indicate that either an additional protein(s) is needed for the efficient binding in vivo or the two short isoforms of Sp3 produced during in vitro translation (data not shown) decreased the actual concentration of the full-length Sp3.
As an additional test of specific binding of NRSF and Sp3 to NRSE/GC box of MOR gene, we performed EMSA with NS20Y nuclear extracts. As shown in Figure 6, three major DNA bound complexes were observed (lane 2). The upper complex was eliminated in the presence of monoclonal NRSF or Sp3 antibody (lanes 5 and 6, respectively), whereas incubation with PI had no effect on the complex (lane 4). The two lower complexes were not affected (lanes 5 and 6) by both antibodies, suggesting that these are not related to NRSF or Sp3. Competitive binding experiments were also conducted in this EMSA using a 100-fold molar excess of a self NRSP cold competitor, NRmSP and NRSPm competitor (lanes 7 and 8, respectively). The self NRSP competitor competed for protein–DNA interaction efficiently (lane 3) and also mutated competitor NRmSP and NRSPm significantly competed for upper complex indicating the specific binding of NRSF and Sp3 (lanes 7 and 8). The signal left after the treatment of the mutated competitor represents the complex with either NRSF (lane 7) or Sp3 (lane 8). In PC12 cells (NRSF negative but Sp3 positive), the NRSF-associated complex (the upper complex in lane 7) was not detected (data not shown). The subtle shift in mobility (lane 7) or no apparent mobility shift (land 8) may be due to the presence of various nuclear proteins that were recruited by both NRSF and Sp3 and/or due to the binding of other nuclear proteins which have affinity for the probe DNA sequences that could now be available after the displacement of NRSF or Sp3 by the mutated competitors.
Interestingly, two lower complexes were dramatically decreased only when NRSPm competitor was used, suggesting that the bound proteins have specificity to NRSE region. Studies investigating the identity of this complex are underway.
Taken altogether, the co-immunoprecipitation and EMSA studies demonstrate that NRSF and Sp3 factor interact with each other and physically bind to the repressive element of the MOR promoter, NRSE/GC box.
ChIP and re-ChIP assay revealed that Sp3 and NRSF were presented together on 188 bp MOR DNA segment for synergic downregulation of MOR expression
To validate the functional Sp3 binding to MOR promoter in vivo, we carried out the in vivo ChIP assay using several antibodies. After cross-linking the proteins and DNAs with formaldehyde, cell lysates from NS20Y cells were subjected to immunoprecipitation with NRSF, HDAC1, HDAC2, Sp1, Sp3 and IRF-4 (as a negative control). The precipitated DNA fragments were PCR-amplified with specific primers design to generate a 188 bp fragment covering the NRSE and Sp3-binding GC box in the MOR gene. As shown in Figure 7A and B, ChIP PCR products were detected with HDAC2, NRSF and Sp3 antibody, but were not detected when PI, no antibody or IRF-4 antibody was tested. In addition, we performed the Re-ChIP assay to verify the Sp3 and NRSF are specifically co-localized on MOR DNA (Figure 7C). First, ChIP was performed using anti-NRSF antibody. Then, prior to reversal of protein–DNA cross-linking, the chromatin fragments were subjected to re-precipitation using anti-Sp1 or anti-Sp3 antibody. During subsequent PCR only those MOR DNA sequences that are simultaneously bound to both NRSF and Sp3 proteins should be amplified. As shown in Figure 7C, Sp3, but not Sp1 and NRSF are specifically co-localized on the 188 bp MOR promoter sequence containing NRSE and a GC box. The results clearly demonstrate that NRSF and Sp3 factor bind to the short MOR promoter segment with close proximity in vivo.
DISCUSSION
Combinatorial interactions between cis-elements and trans-acting factors are required for the regulation of gene expression. One of the best-characterized transcription factors for controlling neuronal gene expression is the repressor element 1 (RE-1)–silencing transcription factor/neuron-restrictive silencer factor (REST/NRSF) repressor protein that is involved in suppressing several neuronal gene expressions in non-neuronal cells (11,12,26–28). We have previously demonstrated that the NRSE of MOR functions as a critical regulator to repress MOR gene expression in specific neuronal cells depending on NRSF expression level (11). In this study, we explored the role of the putative Sp transcription factor binding element (G/C box) in MOR promoter region located 3′ end of NRSE and demonstrated that Sp3 transcription factor represses the expression of MOR gene by binding to this element and by interacting with NRSF.
From the sequence data analysis, we initially found the conserved Sp family binding site adjacent to NRSE in mouse, rat and human MOR gene that could regulate MOR gene expression. When treated with mithramycin A, a G/C box specific binding inhibitor, the mRNA level of MOR gene was increased in NRSF containing NS20Y cells but not in NRSF negative PC12 cells (Figure 1). The result suggested that this GC box element may mainly act as a repressor in MOR gene regulation and explained why the MOR transcription level is very low in NS20Y cell line.
To explore the repression mechanism by this GC box in MOR gene regulation, several MOR-luciferase constructs with mutations in either NRSE or the G/C box or both were made and tested for their promoter activities. Mutations in both NRSE and Sp-binding site showed 14-fold increase in the activity whereas either NRSE or G/C box mutation had 4- to 5-fold increased activity compared with that of wild-type sequences in NS20Y cells (Figure 2). Therefore, the negative effect of the putative Sp-binding element for the MOR gene expression could be considered as additive to or preferably synergic with NRSE. A recent study reported that the transcription levels of the several NRSF target genes containing GC-rich regions were decreased with mithramycin A in a dose-dependent manner in both βTC3 and PC12 cells indicating that Sp1 acts as an activator for the expression of NRSF target genes (29). Two positive Sp family binding elements in MOR promoter were previously identified which is located at ∼300 bp upstream from the GC box identified in the current study (25). In those upstream elements, Sp1 acts as a major activator by binding to both elements whereas Sp3 has minor activator effect.
The identity of the transcriptional repressor that binds to the newly identified GC box was first hinted by co-immunoprecipitation of the Sp3 factor with NRSF in both HeLa and NS20Y nuclear extracts (Figure 4). Then, the specific and functional binding of Sp3 factor to the GC box was confirmed by gel-shift assays using either in vitro translated NRSF and Sp3 factor (Figure 5) or nuclear extract (Figure 6), and in vivo ChIP assay (Figure 7). The results clearly demonstrated that Sp3 factor binds the G/C motif and interacts with NRSF in MOR promoter region, suggesting that transcription factor Sp3 is required for silencing MOR expression together with NRSF.
Within the Sp family transcription factors, Sp1 and Sp3 are ubiquitously expressed in mammalian cells. Sp3 is structurally similar to Sp1, with similar affinities for Sp1-binding site. Although Sp1 and Sp3 share >90% sequence homology in the DNA-binding domain and they bind to the same cognate DNA element, they have strikingly different functions (30). Although Sp1 mainly acts as transcriptional activator, Sp3 can repress activity of the promoter by recruiting HDAC1 or HDAC2. HDAC1 and HDAC2 have been shown to potently repress luteinizing hormone receptor gene transcription. The mSin3A, a component of the Sin3–HDAC complex, potentiates the inhibition mediated by HDAC1 (31,32). It has been well established that the Zn2+-finger-containing protein REST/NRSF binds the 21 bp NRSE DNA element found in many neuronal genes including MOR and then nucleates the formation of a multiprotein complex that represses gene transcription by deacetylating histones, methylating both DNA and histone H3, and dephosphorylation of RNA polymerase II (15,18,33). In conjunction with our current data, especially from the co-immunoprecipitation of Sp3 with NRSF, it can be postulated that the involvement of Sp3 is needed for the full repression of MOR gene in addition to NRSF.
An interesting feature of the Sp3 protein family is that, in addition to a full-length isoform, Sp3 has two short isoforms which are products of differential translational initiation. It has been suggested that the short Sp3 isoforms may be responsible for the transcriptional repression because they do not have any of the transactivation domains located at the N-terminus of Sp3 factor (34). However, our current and previous data strongly suggest that the regulatory action of Sp3 factors is promoter- and cellular context dependent at least in the MOR gene expression because (i) only the full-length Sp3 can interact with NRSF in co-immunoprecipitation experiment whereas all three isoforms of Sp3 are expressed in NS20Y cells (Figure 4C), (ii) two short isoforms of Sp3 act as repressors by binding to the promoter element located at −219 to −189 of MOR gene (4), (iii) Sp3 acts as a weak positive element by binding to Sp1 sites at the proximal region (−450 to −249) of MOR promoter (25).
To our knowledge, this is the first report showing that the sequence-specific transcription factor (NRSF) has a differential interaction among Sp3 isoforms to regulate gene expression. Because two short isoforms are generated by internal start codon, it can be speculated that the N-terminal region (112 amino acid residues) of Sp3, which is absent from the two short isoforms, takes responsibility for the interaction of the full-length Sp3 with NRSF and for determining the role of Sp3 factors for MOR gene expression. In addition, differential post-translational modification states among Sp3 factors, such as sumoylation, phosphorylation and acetylation, may have an important role in determining the fate of Sp3 factors in the regulation of gene expression (35,36).
Although the exact mechanism of interdependency (or cooperativity) between NRSF and Sp3 for binding to NRSE/GC box remains to be elucidated, it is tempting to speculate that the function of Sp3 could be determined by the interaction with NRSF. In other words, if NRSF is abundant in the nucleus, Sp3 interacts with NRSF mainly acting as a repressor while, when NRSF is depleted in the nucleus or inactive, Sp3 might bind to the positive elements and act as a part of Sp1/Sp3 activator complex.
Many researchers eagerly awaited the results of NRSF loss-of-function experiments in the hope that they would shed light on the role of NRSF in the regulation of neural induction. Previous studies clearly indicated that knock-out of NRSF function is not sufficient to induce either precocious neurogenesis in neural precursors or transformation of non-neural cells into neurons (26). Our data may suggest that NRSF is not a master regulator of neural induction and that its function is orchestrated by a symphony of transcription factors for the control of target genes. A recent report identified that huntingtin protein is mutated in Huntington's disease (37). Wild-type huntingtin acts as a positive transcriptional regulator for many NRSE-containing genes involved in the maintenance of the neuronal phenotype (38). Consistently, loss of expression of NRSE-controlled neuronal genes is shown in cells, mice and human brain with Huntington's disease. In Huntington's disease, it has been proposed that mutant huntingtin led to accumulate the NRSF in nucleus, resulting in the strong repression of the BDNF which is controlled by NRSE (39). It is probable that the silencing of NRSF target genes in Huntinton's disease could also be the result of additive or synergic repression through increasing interaction with Sp3 or other transcriptional factors resulting from abnormal presence of NRSF in neuronal cells.
Emerging evidence indicates that the genetic context is extremely important in determining the function of NRSE/NRSF-dependent repression mechanism. In this study, we demonstrated that Sp3 transcription factor represses the MOR gene expression by binding to a GC box adjacent to NRSE and by interacting with NRSF providing evidence that there is another level of complexity in regulating the MOR transcription. Detailed elucidation of such synergic repression mechanism will provide an insight understanding about the complexity of the transcriptional regulation of MOR gene as their expression varies in density in different regions of the central nervous system.
|