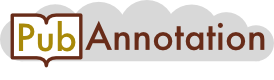
PMC:1044833 / 8532-14830
Annnotations
{"target":"https://pubannotation.org/docs/sourcedb/PMC/sourceid/1044833","sourcedb":"PMC","sourceid":"1044833","source_url":"https://www.ncbi.nlm.nih.gov/pmc/1044833","text":"Model Results Are Robust to Parameter Variation\nAn alternate hypothesis to explain the discordance between estimated sulfide supply and uptake rates is the presence of locally elevated seepage rates. Sensitivity analyses were carried out to determine the potential effects of uncertainty in seepage rate on supply estimated for aggregations without root sulfate release. A 10% increase in seepage rate resulted in a 5.6% increase in sulfide supply to aggregations 200 y old and older. This corresponds to only 16.4% of the sulfide required, which does not serve to extend aggregation survivorship (average, 39 y; range, 21 to 79 y) beyond that determined for lower flow rates. To supply the sulfide flux required by older aggregations, seepage rate would have to be at least 363 mm · y−1. This is over ten times greater than the rate used in the model (32 mm · y−1), which is the highest region-wide estimate for the Gulf of Mexico [24]. A rate of over 300 mm · y−1 approaches rates reported for active venting of fluids (Table 1). Active venting would result in the visual manifestation of seepage in the form of methane bubbles and oil droplets, which are generally restricted to mussel (Bathymodiolus childressi) beds at these sites [25]. In addition, larger, and therefore older [26], aggregations have lower epibenthic sulfide concentrations [8,9,25] suggesting that seepage becomes less vigorous over time and is not in the form of active venting in larger tubeworm aggregations. While difficult to obtain, in situ measures of advection rate of fluids at Gulf of Mexico seeps could be used to test these assumptions and may lend insight into the relationship between variability in tubeworm growth rate and sulfide availability.\nTable 1 Reported Seepage Rates for Hydrocarbon and Methane Seeps The high degree of variability in growth rate and recruitment rate could also affect the ratio of supply and demand in the model. In an aggregation exhibiting anomalously low recruitment, the size of the rhizosphere would increase more rapidly than the biomass of the aggregation. This would lead to high rates of sulfide delivery and generation and low rates of sulfide uptake by tubeworm roots. When initial recruitment rate (a in equations 1 and 2) is decreased by 10%, the length of time that supply exceeds demand increases by 3.7%. This effect appears to be linear, with a 20% decrease in initial recruitment rate resulting in a 7.4% increase in persistence. If growth rate is increased, thereby increasing the rate of rhizosphere growth in terms of surface area for diffusion and advection, there appears to be little effect of the ratio of supply to demand (20% increase in growth—0% change in persistence time). In fact, increasing growth to the upper limits of the error term (equation 5) lowers the amount of time that the aggregation can be supported since biomass and sulfide demand increase more rapidly than increases in supply resulting from additional surface area. By decreasing growth rate, aggregations may be supported for longer periods of time, with a 20% decrease leading to a 6.3% increase in persistence time and a decrease of 88% leading to persistence for over 250 y. While an 88% lower growth rate lies outside of the range of existing growth data, this could be accomplished by ceasing growth for extended periods of time in a quiescent stage. This possibility remains to be investigated in L. luymesi. By utilizing a variable recruitment rate in the model, both between realized aggregations and between years within a model run, along with a growth error term encompassing the full range of observed growth data, the model is capable of generating aggregations within the range of the 10%–20% variability tested in this analysis. Even these outlying aggregations (presented as maxima and minima in Figure 1) support the qualitative conclusions drawn from model results.\nWhile the model was based on empirical data to the greatest degree possible, estimates of many of the parameters necessary to resolve the model were not available or are extremely difficult to measure in deep water with existing technology. Uptake rates were measured in the laboratory [8] for relatively small individuals (\u003c50 cm). While we attempted to approximate metabolic scaling by covarying uptake and growth rates, it is possible that large individuals require even lower sulfide flux. Model predictions are not overly sensitive to variability in this parameter. A reduction by 10% of the overall sulfide uptake rate results in a 5.2% increase in persistence time. To maintain an aggregation for over 250 y, mass-specific uptake rate would have to be reduced 6-fold. While this could also be accomplished by entering a period of quiescence as mentioned before, there is no existing evidence for this ability in vestimentiferans.\nThe second version of the model is based on the assumption that L. luymesi is capable of releasing sulfate through its roots. It should be noted that in the model, sulfate release is constrained by the rate of sulfate generation by the tubeworm's sulfide-oxidizing symbionts, resulting in the near 1:1 ratio of supply and demand in Figure 1. Though modeled sulfate flux across the roots into the rhizosphere may exceed 20 mmol · h−1 in older aggregations, the roots provide an ample respiratory surface such that rates of sulfate flux per unit root surface area do not exceed 0.4 μmol · h−1· cm−2 in the model. It remains possible that a proportion of the sulfate could be released through the plume of the tubeworms, though the energy required to pump sulfate against a concentration gradient (seawater [SO4] = 29 mM) [13] suggests that it would be more energetically favorable for the sulfate to passively diffuse out of the roots. It is also possible that sulfate flux could be increased by active bioirrigation delivering seawater to deeper sediment layers through the tubeworm tubes. This could allow the sulfide-oxidizing symbionts to store some of the oxidized sulfide as elemental sulfur rather than releasing it as sulfate, while maintaining sufficient sulfate flux to deeper sediment layers for sulfide generation. These mechanisms remain hypothetical and require further experimental investigations to evaluate their potential role in this system.","divisions":[{"label":"title","span":{"begin":0,"end":47}},{"label":"p","span":{"begin":48,"end":1734}},{"label":"table-wrap","span":{"begin":1735,"end":1800}},{"label":"label","span":{"begin":1735,"end":1742}},{"label":"caption","span":{"begin":1744,"end":1800}},{"label":"title","span":{"begin":1744,"end":1800}},{"label":"p","span":{"begin":1801,"end":3902}},{"label":"p","span":{"begin":3903,"end":4839}}],"tracks":[{"project":"2_test","denotations":[{"id":"15736979-10676948-84746028","span":{"begin":1284,"end":1286},"obj":"10676948"},{"id":"T98413","span":{"begin":1284,"end":1286},"obj":"10676948"}],"attributes":[{"subj":"15736979-10676948-84746028","pred":"source","obj":"2_test"},{"subj":"T98413","pred":"source","obj":"2_test"}]}],"config":{"attribute types":[{"pred":"source","value type":"selection","values":[{"id":"2_test","color":"#93d9ec","default":true}]}]}}