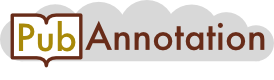
PMC:1044833 / 14832-22169
Annnotations
{"target":"https://pubannotation.org/docs/sourcedb/PMC/sourceid/1044833","sourcedb":"PMC","sourceid":"1044833","source_url":"https://www.ncbi.nlm.nih.gov/pmc/1044833","text":"Tubeworms Impact Seep Biogeochemistry\nTubeworm sulfate release, in conjunction with high sulfide uptake rates, could contribute to the observation of declining advection rate in older aggregations. By increasing sulfate flux to deeper sediments, L. luymesi increases integrated rates of anaerobic methane oxidation and hydrocarbon degradation, which would enhance authigenic calcium carbonate precipitation within the rhizosphere. Under the conditions of root sulfate release in the model, calcium carbonate precipitation is rapid (0.109 to 0.316 μmol · l−1 · sec−1) in the first 53 y, with rates declining exponentially thereafter. By creating a barrier to fluid advection [4], this could result in the observed decrease in epibenthic sulfide concentration in older aggregations [8,9] and the predicted cessation of tubeworm recruitment around this time [12,23].\nIn order to prevent the precipitation of carbonate directly on the root surface, L. luymesi individuals may release hydrogen ions as well as sulfate through their roots. While hydrogen ion flux through the roots has not yet been empirically demonstrated, none of the nearly 5,000 tubeworms examined as part of this study were observed to have carbonate formed directly on their roots, suggesting that this form of precipitation is inhibited in some manner. In the model, diffusion of hydrogen ions across the root surface (the only form of release explicitly modeled) accounts for less than 40% of ion generation when carbonate precipitation is most vigorous. We speculate that L. luymesi may utilize the excess hydrogen ions generated by their sulfide-oxidizing symbionts to periodically raise the rate of hydrogen ion flux from their roots. This would not only supply additional hydrogen ions to sulfate-reducing bacteria, but could inhibit carbonate precipitation on the tubes and subsequent reduction of the root area utilizable as a respiratory surface. Further pH reduction could dissolve existing carbonate in sediments beneath the rhizosphere, thereby opening seepage pathways and allowing further root growth. This possibility is corroborated by the observation of young tubeworms that had apparently bored through bivalve shells in an experimental system (R. Carney, personal communication). Empirical measurements of hydrogen ion flux across the root tissue of L. luymesi are required to test these hypothetical mechanisms.\nThe release of sulfate by tubeworm roots potentially explains the frequent observation of highly degraded hydrocarbons in the vicinity of large tubeworm aggregations [27]. The majority of sulfate supplied by tubeworm roots is utilized for microbial hydrocarbon degradation in the model (Figure2). This process alone accounts for over 60% of the sulfide available to aggregations after approximately 80 y. In the absence of liquid and solid phase hydrocarbons, methane flux would have to be approximately four times the rate in the model in order to fuel sufficient sulfate reduction to support an aggregation for over 200 y. This could occur in sediments overlying rapidly sublimating gas hydrates, and hydrate abundance has been previously suggested as a potential factor influencing the distribution of chemosynthetic communities in the Gulf of Mexico [10]. However, model results indicate that large chain hydrocarbons are the most significant energy source for sulfate reduction in tubeworm-dominated sediments. Increased integrated rates of hydrocarbon degradation would lead to highly biologically altered hydrocarbon pools among the roots of tubeworm aggregations. Hydrocarbon oxidation has been implicated as one of the dominant processes in the carbon cycle at ULS seeps, accounting for over 90% of the carbon in carbonates collected in the vicinity of tubeworm aggregations [19]. Model analysis indicates that the minimum amount of organic carbon (including hydrocarbons as well as buried organic material) in sediments required to supply sulfide at rates matching aggregation demand (1:1 supply:uptake ratio) is 1.03% by weight, remarkably close to the lowest value found in any of the seep sediment core samples (1.2%) [13,28], and greater than that found in ULS sediments away from seeps (0.71%) [29]. Determination of organic carbon concentration in sediments beneath tubeworm aggregations is necessary to test the prediction that elevated carbon content at seeps, primarily resulting from oil seepage, provides the energy source required to generate sufficient sulfide for tubeworm aggregations.\nFigure 2 Sources of Sulfide Available to Tubeworm Aggregations over Time in the Model\nSources of sulfide include advection and diffusion of sulfide from deep sources (yellow) or sulfate reduction using methane (blue), buried organic carbon (green), or C6+ hydrocarbons (dark grey) as electron donors. Sulfate is provided by diffusion from sediments surrounding the rhizosphere, diffusion at the sediment–water interface, and release from tubeworm roots. Additional sulfate flux from tubeworm roots could also explain the high apparent sulfate diffusion coefficients determined for tubeworm-impacted sediments [13]. Anomalous sulfate fluxes have been proposed to be a result of bioturbation and bioirrigation by macrofauna [3,5], and recycling by microbial mats [13]. The results of the model presented here provide evidence for macrofaunal sulfur recycling, an additional component to be considered in future investigations of cold seep biogeochemistry. The hypothesized release of sulfate by tubeworm roots potentially explains numerous, apparently disparate observations, hinting at the great impact that L. luymesi aggregations may have on their abiotic environment.\nWhile the proposed interactions between symbiotic tubeworms and sulfate-reducing bacteria are essential for the persistence of L. luymesi aggregations in the model, we suggest that there are significant effects on the microbial community as well. This syntrophy will increase the abundance of sulfate-reducing bacteria and therefore increase the rates of anaerobic methane oxidation and hydrocarbon degradation carried out by microbial consortia that rely on sulfate as an oxidant. Tubeworm-generated sulfate supplies a more energetically favorable electron acceptor below the normal depth of sulfate penetration at seeps, relaxing the limitation on anaerobic oxidative processes at these sediment depths. Deeper sediment layers then become habitable to sulfate reducers, significantly altering the microbial community structure within the rhizosphere. Model configurations neglect the potential role of bioirrigation of seawater sulfate through L. luymesi tubes, which could further increase sulfate supply to deeper sediment layers. The possible role of tubeworm roots as substrata for the growth of microbial consortia, analogous to the habitat afforded mycorrhizal symbionts of higher plants, remains another possible benefit for the microbes. These predictions may be tested by determination of the relative abundance of microbial consortia at different depths of sediments both impacted by and isolated from tubeworms. Localization of the microbes on the root surface would provide evidence for a more intricate relationship. It is our hope that the results of this model may provide the impetus for future rigorous experimental tests of these ideas.","divisions":[{"label":"title","span":{"begin":0,"end":37}},{"label":"p","span":{"begin":38,"end":863}},{"label":"p","span":{"begin":864,"end":2398}},{"label":"p","span":{"begin":2399,"end":4509}},{"label":"figure","span":{"begin":4510,"end":4964}},{"label":"label","span":{"begin":4510,"end":4518}},{"label":"caption","span":{"begin":4520,"end":4964}},{"label":"title","span":{"begin":4520,"end":4596}},{"label":"p","span":{"begin":4597,"end":4964}},{"label":"p","span":{"begin":4965,"end":5680}}],"tracks":[]}