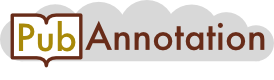
FA10@AikoHIRAKI:FA1115 / 0-10
The Matrix Protein hnRNP U Is Required for Chromosomal Localization of Xist RNA
Summary
In XX female mammals, one of the two X chromosomes is epigenetically inactivated to equalize gene expression with XY males. The formation of the inactive X chromosome (Xi) is regulated by an X-linked long noncoding RNA Xist, which accumulates on the entire length of the chromosome in cis and induces heterochromatin formation. However, the mechanism by which Xist RNA “paints” the Xi has long remained elusive. Here, we show that a matrix protein hnRNP U/SP120/SAF-A is required for the accumulation of Xist RNA on the Xi. Xist RNA and hnRNP U interact and upon depletion of hnRNP U, Xist RNA is detached from the Xi and diffusely localized into the nucleoplasm. In addition, ES cells lacking hnRNP U expression fail to form the Xi. We propose that the association with matrix proteins is an essential step in the epigenetic regulation of gene expression by Xist RNA.
Introduction
Since it was first proposed by Lyon in 1961 (Lyon, 1961), X chromosome inactivation has provided an excellent model system to study the epigenetic regulation of gene expression (for review, see Brockdorff, 2002, Lee, 2003, Masui and Heard, 2006, Ng et al., 2007). In female mammals, one of the two X chromosomes is transcriptionally repressed during early development to equalize the expression of X-linked genes in females and males (Lyon, 1961). Central to this process is a long noncoding RNA, Xist. This RNA was identified as a novel transcript expressed from a specific cis-acting locus called the X inactivation center, which is required for the establishment of the inactive X chromosome (Xi) (Borsani et al., 1991, Brockdorff et al., 1991, Brown et al., 1991). The functional importance of Xist in the establishment of X inactivation has been demonstrated in a series of experiments. First, embryonic stem (ES) cells lacking one Xist allele fail to establish random X inactivation upon differentiation (Penny et al., 1996). Second, exogenous induction of Xist expression during the differentiation of ES cells results in the inactivation of the chromosome expressing the transgene, leading to progressive cell death (Lee et al., 1996, Wutz and Jaenisch, 2000). Third, female mice show an embryonic lethal phenotype when they paternally inherit a deficient X chromosome, due to a failure in stabilizing the inactive state of the paternally imprinted Xi (Marahrens et al., 1997). Once established, however, the inactive state of the Xi is no longer dependent on the expression of Xist, since the monoallelic expression of X-linked genes is maintained in mouse embryonic fibroblasts (MEFs) upon conditional deletion of a large part of the Xist gene (Csankovszki et al., 1999). These studies suggest that the expression of Xist is necessary and sufficient to initiate the formation of the Xi but not for its maintenance.
One of the remarkable features of Xist is the subcellular and subnuclear localization of its transcripts. In the case of protein-coding mRNAs, post-transcriptional modifications such as 5′ capping, intron removal, and 3′ end processing promote the assembly of nuclear export factors, leading to rapid transport of mature transcripts into the cytoplasm (for review, see Dreyfuss et al., 2002). Despite its mRNA-like characteristics (e.g., being spliced and polyadenylated), Xist RNA escapes nuclear export and is retained within the nucleus (Brockdorff et al., 1992, Brown et al., 1992). More strikingly, Xist RNA accumulates at the site of its transcription and spreads along the entire length of the chromosome, coating the Xi (Clemson et al., 1996). This chromosomal “painting” by Xist RNA is closely associated with the accumulation of polycomb repressor complex 2 (PRC2) on the X chromosome and subsequent deposition of the inactive histone marker H3K27me3 (Silva et al., 2003). In addition, a variety of proteins are known to be localized to the Xi during and following the initiation of X inactivation; these include another polycomb complex, PRC1 (Plath et al., 2004); structural maintenance of chromosomes hinge domain-containing 1 protein (SmcHD1) (Blewitt et al., 2008); a histone variant called macro histone H2A1 (Costanzi and Pehrson, 1998); a trithorax group protein Ash2l (Pullirsch et al., 2010) and a nuclear matrix protein, heterogeneous nuclear ribonucleoprotein U (hnRNP U; also known as SP120 and SAF-A) (Helbig and Fackelmayer, 2003, Pullirsch et al., 2010). Although many of these proteins are functionally required for the establishment and maintenance of the Xi, none of them has been shown to regulate the unique localization of Xist RNA on the Xi itself (for review, see Brockdorff, 2002, Masui and Heard, 2006). BRCA1 has previously been proposed to regulate the chromosomal accumulation of Xist RNA (Ganesan et al., 2002, Silver et al., 2007). However, the localization of BRCA1 protein as well as its requirement for X inactivation remains largely controversial (Pageau et al., 2007, Xiao et al., 2007).
In this study, we show that hnRNP U is required for the association of Xist RNA with the Xi. RNAi-mediated knockdown of hnRNP U leads to lack of localization of Xist RNA on the Xi, and the accumulation of H3K27me3 also disappears in RNAi-treated cells. In addition, ES cells that do not express hnRNP U fail to form inactive X chromosomes, resulting in frequent biallelic expression of X-linked genes. We propose that hnRNP U is the Xist-interacting protein that tethers Xist RNA on the Xi through its DNA- and RNA-binding properties, which are necessary for the initiation of X inactivation.
Results and Discussion
hnRNP U Is Required for the Chromosomal Localization of Xist RNA
To identify factors that control Xist RNA localization on the Xi, we screened a small scale, custom RNAi library designed for 174 of abundant RNA-binding proteins (see Table S1 available online). We used a mouse neuroblastoma cultured cell line Neuro2a, in which we could efficiently knockdown target transcripts following transfection of synthesized siRNA (Figure 1A). In the control Neuro2a, Xist RNA was observed in three discrete foci in the nucleus (Figure 1B). Various RNA-binding protein knockdowns showed no obvious effect on this pattern (Figures S1A and S1B) but knockdown of a single protein, hnRNP U (Kiledjian and Dreyfuss, 1992) (also known as SP120 [Tsutsui et al., 1993] or SAF-A [Romig et al., 1992]) led to a dramatic change in which Xist RNA became diffusely distributed throughout the nucleus (Figure 1B). Visualization of the X chromosome territories in the hnRNP U-depleted cells revealed no obvious change in the number or shape of the X chromosome (Figure 1D), suggesting that a change in packaging or organization of the interphase X chromosome was not the reason for the observed effects. The amount of total Xist RNA was decreased by the hnRNP U knockdown (Figures S1C and S1D); however, similar decrease of Xist RNA by transcriptional inhibition did not lead to the delocalization of Xist RNA from the chromosome (Figures S1E and S1F).
A hypomorphic mutation in hnRNP U results in early embryonic lethality in mice (Roshon and Ruley, 2005), and it is therefore possible hnRNP U knockdown is cell toxic and that changed Xist RNA localization is a nonspecific consequence of this. We thus analyzed the cell cycle distribution by FACS of cells 72 hr after the siRNA treatment, at the time when Xist RNA was almost completely delocalized from the X chromosomes. The analysis revealed that the distribution of cell cycle stages was unchanged upon hnRNP U knockdown (Figure 1C), suggesting that at least cell cycle progression was not affected by the siRNA treatment. We also examined the effect of various stresses on the Xist RNA localization in the Neuro2a cells, including transcriptional inhibition by α-amanitin, heat shock, osmotic shock, and UV irradiation. None of these treatments changed the condensed localization of Xist RNA (Figure 1E), suggesting that the delocalization of Xist RNA was not caused by secondary nonspecific stress resulting from depletion of hnRNP U.
hnRNP U Directly Interacts with Xist RNA via RGG RNA-Binding Domain
hnRNP U contains three conserved domains: a SAF-Box (Kipp et al., 2000) that binds to AT-rich chromosomal region known as a scaffold- or matrix-attachment region (S/MAR) (Mirkovitch et al., 1987), SPRY (Spla and Ryanodine receptor) domain of unknown function (Ponting et al., 1997), and RGG (arginine-glycine-glycine) RNA-binding domain (Helbig and Fackelmayer, 2003). Presence of the latter suggested hnRNP U could directly associate with Xist RNA. To test this, we carried out a coimmunoprecipitation experiment. Xist RNA is fractionated into the nuclear matrix (Clemson et al., 1996) and its insoluble nature has hampered biochemical analyses (for review, see Masui and Heard, 2006, Ng et al., 2007). We thus initially examined various conditions that enable efficient solubilization of Xist RNA. We found that the majority of Xist RNA becomes soluble by mild sonication in the presence of 1% SDS (Figure 2A), and these conditions were used to test if hnRNP U interacts with Xist RNA. We then established Neuro2a cells that stably express FLAG-tagged hnRNP U as well as mutant hnRNP U lacking the SAF-Box or RGG domain, which enabled efficient and specific immunoprecipitation of the tagged proteins. The introduction of these exogenous genes mildly changed the expression level of Xist RNA (Figure S2); however, the chromosomal localization of Xist RNA was not affected (Figure 2H). Using these cells, Xist RNA was specifically coimmunoprecipitated with hnRNP U in a region specific manner when the complex was crosslinked by UV irradiation (Figures 2C and 2D). This interaction was greatly reduced in the absence of the UV-crosslink under the stringent buffer condition (Figures 2C and 2D), suggesting that hnRNP U interacts with Xist RNA at close proximity. We also found that the RGG RNA-binding domain of hnRNP U was required for the interaction with Xist RNA, while the SAF-Box was dispensable for it (Figures 2C and 2D).
Both Matrix- and RNA-Binding Domain of hnRNP U Are Necessary for Proper Xist RNA Localization
To determine which domains of hnRNP U are required for the accumulation of Xist RNA, we transiently transfected the cells with a series of FLAG-tagged deletion mutants containing silent point mutations in the target region of the siRNA (Figures 2E and 2F). Under these experimental conditions, 86% of the cells possessed condensed Xist RNA signals in the control Neuro2a cells. This frequency decreased markedly to 20% after siRNA treatment (Figures 2G and 2H). The introduction of exogenous full-length hnRNP U rescued the RNAi phenotype, and 66% of the cells restored the condensed Xist RNA localization (Figures 2G and 2H). The mutant hnRNP U lacking the SPRY domain also rescued the phenotype to a similar ratio comparable to the full-length molecule (63%). In contrast, hnRNP U lacking the SAF or RGG domains showed no increase in Xist RNA localization (19% for ΔSAF and 26% for ΔRGG), suggesting that both DNA- and RNA-binding activity of hnRNP U are necessary for the localization of Xist RNA on the X chromosomes (Figures 2G and 2H).
hnRNP U Is Required for the Formation of Condensed H3K27me3 Marks on the Xi
In the course of these experiments, we noticed that histone H3 lysine 27 tri-methylation (H3K27me3), a marker for Xi (Plath et al., 2003, Silva et al., 2003), did not accumulate on the X chromosome in the Neuro2a cells (data not shown), suggesting that the inactivation state of the X chromosome is unusual in this particular cell line. We therefore re-examined the effect of hnRNP U knockdown on Xist RNA localization using primary cultures of mouse embryonic fibroblast derived from female embryos. The RNAi treatment efficiently downregulated the expression of hnRNP U (Figure 3A), and the localization of Xist RNA was decondensed in these cells (Figure 3B). In addition, focal expression of H3K27me3 also disappeared upon hnRNPU knockdown (Figures 3C and 3E), whereas total H3K27me3 was not significantly affected (Figure 3D). In contrast, monoallelic expression of X-linked genes was not affected after hnRNP U knockdown (Figure 3F). H3K27me3 is catalyzed by the polycomb repressor complex 2 (PRC2) and recruitment of this complex to the inactive X is Xist RNA dependent (Plath et al., 2003, Silva et al., 2003, Kohlmaier et al., 2004). We conclude that in the absence of localized Xist RNA PRC2 recruitment and H3K27me3 accumulation on the inactive X do not occur. That loss of H3K27me3 and other Xist-dependent modifications is insufficient to cause reactivation of the inactive X, which is consistent with previous studies demonstrating that continued Xist RNA expression is not required for the maintenance of X inactivation in differentiated somatic cells (Csankovszki et al., 1999).
Delocalized Xist RNA Contains Putative Chromosome-Localizing Elements
The chromosomal localization of Xist RNA is regulated by cis-elements separately distributed in 5′ half of the molecule (Wutz et al., 2002). It was thus theoretically possible that downregulation of hnRNP U induced cleavage or alternative splicing of Xist RNA, resulting in the release of partial RNA fragments lacking the localization signals. To exclude this possibility, we carried out Northern blot analysis using multiple probes that detect different regions of the Xist RNA (Figure 3G). As previously described (Ma and Strauss, 2005), two isoforms of Xist RNA, the L- and S-isoforms, were detected as discrete bands in the RNA derived from the control MEF (Figure 3H). The S-isoform remained intact after the knockdown of hnRNP U (Figure 3H), and this form was detected by all the probes designed for the elements necessary for X chromosome localization (Figures 3G and 3H). On the other hand, the L-isoform completely disappeared after the RNAi treatment, suggesting that this isoform becomes unstable upon detachment from the X chromosome, presumably due to the lack of a poly A tail in this specific isoform (Memili et al., 2001). These observations were also confirmed by RNA FISH analysis (Figure 3I).
hnRNP U Is Essential for the Establishment of Xi during the ES Cell Differentiation
We finally investigated if hnRNP U is functionally required for the initiation of X chromosome inactivation using female ES cell, which undergo random X-inactivation upon differentiation (Norris et al., 1994, Penny et al., 1996). The siRNA efficiently downregulated expression of hnRNP U in 2 days, and expression then recovered progressively during subsequent culture (Figure 4A). Upon induction of differentiation (Figure S3A), both control and hnRNP U-knockdown ES cells downregulated pluripotent cell markers Oct3/4 and Nanog (Figure 4B), showed flattened morphology (Figure 4C), and upregulated mesoendodermal cell marker N-cadherin, neural marker Nestin, and endodermal marker GATA4 (Figures 4B and 4C). These observations suggested that the basic process of differentiation was not affected by the hnRNP knockdown. On the other hand, cellular proliferation was decreased by the depletion of hnRNP U (Figure S3E), suggesting that hnRNP U is also required for normal proliferation of ES cells. Focal Xist RNA signals were observed in 33% of control cells 4 days after the induction of differentiation (Figure S3D), and 82% of these signals overlapped with intense H3K27me3 domains (Figures 4D–4F). On the other hand, the ratio of Xist-expressing cells decreased to 18% upon treatment with the hnRNP U siRNA (Figure S3D), and the Xist RNA signals were diffusely localized in these cells (Figure 4D). The accumulation of H3K27me3 was observed only in 7% of the total cells upon knockdown of hnRNP U (Figures 4E and 4F). In addition, accumulation of Ezh2, a component of PRC2 complex, was also decreased in these cells (Figures S3B and S3C). These observations suggested that hnRNP U is required for the establishment of X inactivation in addition to the localization of the Xist RNA on the X chromosome. To confirm this, we examined if hnRNP U is required for the monoallelic expression of X-linked genes in differentiated ES cells using probes that detect pre-mRNA of PGK1 and MeCP2, which are located proximal and distal to the X chromosome inactivation center, respectively. For this experiment, we restricted our analysis to the cells with a single Xist RNA domain to exclude polyploid or X chromosome aneuploid cells. The ratio of biallelic expression increased from 8% to 22% and 22% to 39% for PGK1 and MeCP2 (Figures 4G and 4H), respectively, suggesting that hnRNP U is required for the establishment of the monoallelic expression of X-linked genes.
Insight into the Molecular Mechanism of Xist RNA Localization and X Chromosome Inactivation
We have thus demonstrated that hnRNP U is required for the localization of Xist RNA on the X chromosome and also for the subsequent establishment of the Xi. hnRNP U binds both DNA and RNA in a noncompetitive manner (Fackelmayer et al., 1994) and forms multimers in the presence of nucleic acids (Fackelmayer et al., 1994). We speculate that nascent Xist RNA is trapped by hnRNP U, and that the complex then forms multimers on the chromosomal DNA. Importantly, Fackelmayer and colleagues have reported that GFP-tagged hnRNP U is concentrated in the territories of the Xi (Helbig and Fackelmayer, 2003, Fackelmayer, 2005, Pullirsch et al., 2010), an observation that strongly supports our conclusion. Considering that the enrichment of hnRNP U occurs relatively late during the Xi formation at the maintenance stage of the gene silencing (Pullirsch et al., 2010), Xist-localizing function of hnRNP U might be separable from its function to establish stable chromatin structures that would require accumulation of Xist RNA on the Xi (Pullirsch et al., 2010). We did not detect concentrated hnRNP U signal on the Xi by immunostaining, possibly indicating that the epitope of hnRNP U or FLAG tag detected by our antibody is masked by other proteins or RNA due to the condensed nature of Xi.
hnRNPU has been identified as a component of the nuclear matrix that binds S/MAR (Romig et al., 1992, Tsutsui et al., 1993). It should be noted that a recent study also identified a matrix protein SATB1 (special AT-rich binding protein 1) as a protein factor that is required to make cells competent to initiate Xist-dependent transcriptional repression (Agrelo et al., 2009). There is thus increasing evidence that the nuclear matrix plays a key role in the formation of Xi, and future studies on the insoluble components in the matrix may advance our understanding on the process of epigenetic modification of gene expression.
Experimental Procedures
Plasmid Transfection and RNAi-Mediated Knockdown
All primer information is provided as Supplemental Experimental Procedures. Details of vector construction are available on request. siRNA transfections were performed with lipofectamine RNAi max (Invitrogen) and synthesized siRNAs (Ambion). For knockdown of Neuro2a and MEF, 2 × 104 cells were plated in an 8-well chamber to which siRNA-lipofectamine complexes were immediately added. After 72 hr, cells were assayed for each experiment. In ES cells, 1.0 × 105 cells were plated on a 35 mm dish (Nunc) with siRNA-lipofectamine complexes. Cells were then cultured in ES cell medium or differentiation medium for appropriate days. Plasmid transfections into the Neuro2a were carried out with FuGENE HD (Roche). One day before transfection, 4 × 105 cells were plated on 35 mm dish and allowed to grow for 24 hr. Transfections were performed with 2 μg of plasmid DNA. In the case of cotransfection with siRNA, plasmid DNA were transfected 1 day before siRNA transfection. Then, cells were trypsinized and plated in the 8-well chamber with siRNA-lipofectamine complexes.
In Situ Hybridization and Immunohistochemistry
Fluorescent in situ hybridization was performed according to a protocol described previously (Sone et al., 2007). Neuro2a or MEF cells were cultured in a well of 8-well chamber slide (IWAKI) and fixed overnight in 4% paraformaldehyde at 4°C. ES cells were cultured on collagen-coated dishes (IWAKI) and fixed with 4% paraformaldehyde for 20 min at room temperature. The antibodies used are described in the Supplemental Information.
Drug and Stress Treatment
α-Amanitin (A2263, Sigma) was added to the cell culture medium at 10 μg/ml, and cells were incubated for 8 hr. Heat stress treatment was at 45°C for 30 min. For osmotic stress, cells were treated with 400 mM sorbitol for 2 hr. DNA damage response was induced by 200 J/m2 UV irradiation, and then cells were recovered in culture medium at 37°C for 1 hr.
Immunoprecipitation of Xist RNA
For immunoprecipitation experiments, we first established Neuro2a cells stably expressing FLAG-tagged hnRNP U and the molecule lacking the RGG domain or SAF domain. We could not establish stable cell lines expressing hnRNPU lacking the SPRY domain after repeated trials. For UV crosslinking, 1 × 107 cells were washed twice with HEPES-buffered saline (HBSS; HEPES = 10 mM [pH 7.4]) and irradiated with 4000 J/m2 UV in 1 ml of ice-cold HBSS. The cells were collected in 1.5 ml microtubes and pelleted by centrifugation. The cell pellets were resuspended with 200 μl SDS buffer (50 mM Tris-HCl [pH 8.0], 1 mM EDTA, 150 mM NaCl, 1 mM DTT, 1% SDS, 1% Triton X-100) and were incubated for 10 min on ice. The cells were then gently sonicated (UR-20P, Tomy Seiko Co., Ltd.) and diluted ten times with a dilution buffer (50 mM Tris-HCl [pH 8.0], 1 mM EDTA, 150 mM NaCl, 1 mM DTT, 1% Triton X-100, supplemented with 1× protease inhibitor cocktail [Nacalai, Japan] and RNase inhibitor [TOYOBO, Japan]). The soluble fraction was recovered after centrifugation at 15,000 rpm for 30 min at 4°C, and subsequently used for immunoprecipitation analysis. For immunoprecipitation, 50 μl of anti-FLAG M2-Agarose (Sigma) were added to the extract and incubated for 2 hr at 4°C with continuous rotation. The beads were washed once with high salt buffer (20 mM Tris-HCl [pH 8.0], 1 mM EDTA, 500 mM NaCl, 1 mM DTT, 0.1% SDS, 1% Triton X-100), and four times with low salt buffer (20 mM Tris-HCl [pH 8.0] 1 mM EDTA, 150 mM NaCl, 1 mM DTT, 0.1% SDS, 1% Triton X-100). The beads were suspended in 200 μl of TE containing 0.5% SDS and 150 ng/ml of Proteinase K (PCR grade, Roche) and incubated for 1 hr at 37°C. RNAs were extracted with Trizol Reagent (Invitrogen). One-tenth of coprecipitated RNA was used as a template for subsequent cDNA synthesis. cDNAs were synthesized using Rever Tra Ace (TOYOBO) and random (N6) oligonucleotide according to the manufacturer's instructions, and RT-PCR was performed with ExTaq (Takara) using the following conditions; denaturation (96°C, 3 min), 32 cycles (27 cycles for Gapdh) of denaturation (96°C, 30 s), annealing (60°C, 30 s), and extension (72°C, 30 s).
|